- 1Department of Ichthyology and Fisheries Science, Rhodes University, Grahamstown, South Africa
- 2South African Institute for Aquatic Biodiversity, Grahamstown, South Africa
- 3Namibia Nature Foundation/European Union Community Conservation Fisheries in KAZA Project, Katima Mulilo, Namibia
- 4Department of Fisheries and Oceans Canada, Maurice Lamontagne Institute, Mont-Joli, QC, Canada
- 5Department of Science and Technology/National Research Foundation Research Chair in Inland Fisheries and Freshwater Ecology, South African Institute for Aquatic Biodiversity, Grahamstown, South Africa
In Africa, wetlands, such as shallow, ephemeral lakes provide ecosystem services, such as water purification, food supply, and flood control but are subject to dynamic flooding/drying cycles which vary in duration from years to decades. The stochastic nature of drying events subjects ephemeral lake fauna to persistent disturbance regimes, therefore understanding how biota respond to flooding and drying events is essential for their conservation and management. Primary production sources supporting consumer biomass in the shallow ephemeral Lake Liambezi (upper Zambezi Ecoregion), were investigated using stable isotope analysis, mixing models and stomach content analysis to investigate the following hypotheses: (1) algal primary production supports a higher consumer biomass than aquatic macrophytes; (2) the lake food chain is short, because the majority of fish fauna are detritivorous/herbivorous cichlids that are consumed by top predators; (3) fish community trophic structure will be similar between years; and (4) with short food chains and stochastic resource availability, there will be substantial competition for food among fish species. Results showed that phytoplankton production supported substantial consumer biomass in Lake Liambezi, with important contributions from macrophytes and associated detritus and/or periphyton. While particulate organic matter (POM) contributed substantially to the diet of herbivorous/detritivorous tilapiine cichlids (the backbone of Lake Liambezi's commercial fishery), considerable dietary carbon was likely also derived from aquatic plants and associated detritus and/or periphyton compared to other fishes. Three major food chains were identified in the lake. The phytoplankton-based pelagic food chain was longest, involving up to four trophic transfers. The benthic food chain based primarily on detritus of planktonic origin (but may also include macrophyte associated detritus/periphyton) was characterized by high levels of omnivory and involved up to three trophic transfers. The macrophytic detritus-based food chain was shortest, involving just two trophic transfers. Predators fed across all three food chains, but predominantly on the two benthic food chains. A combination of dietary overlap (amongst piscivores/predators, amongst insectivores), dietary specialization (tilapiine cichlids, alestids), the integration of multiple food chains and behavioral adaptation to changing dietary resources underpins the ability of Lake Liambezi's fish community to thrive under the stochastic nature of ephemeral lake ecosystems.
Introduction
Wetlands are diverse and productive systems (Ward et al., 1999, 2002) providing many economically valuable goods and services, such as water purification, food supply, and flood control (Baron et al., 2002). Among the most productive and important wetlands in Africa are large, shallow lakes (<5 m average depth, e.g., Lakes Chad and Chilwa) (Kalk et al., 1979; Carmouze et al., 1983), many of which occur in the drier tropics where surface water is scarce, making them extremely important both ecologically and economically (Kolding et al., 2016). With a high surface area relative to their volume, water replacement time in shallow lakes is generally short, making them especially sensitive to changes in water inputs (Talling, 2001). As a consequence, water level fluctuations are a dominant force controlling ecological processes in shallow lakes (Dumont, 1992; Wantzen et al., 2008; Kolding and van Zwieten, 2012). Many other environmental factors vary concurrently with fluctuations in water level (Talling, 1992), with important consequences for lake biota (Dumont, 1992; Talling, 2001).
Seasonal variation in the hydrological regime of tropical lakes becomes more pronounced with increasing latitude (Talling, 2001), and many endorheic lakes in the drier tropics experience large interannual fluctuations. In extreme cases, these are characterized by flooding and drying cycles, which can vary widely in duration from years to decades (e.g., Lake Chilwa; Kalk et al., 1979, and to some extent Lake Chad; Carmouze et al., 1983). Drying is a major disturbance in ephemeral lake ecosystems with severe consequences for populations of aquatic biota, but may be necessary for the maintenance of species diversity in ephemeral lakes as it increases habitat diversity, and promotes species coexistence by mediating biotic interactions (Dumont, 1992; Ward et al., 2002; Wantzen et al., 2008). The frequency and stochastic nature of drying events subjects ephemeral lake fauna to a persistent disturbance regime (Dumont, 1992) and thus understanding how biota respond to flooding and drying in ephemeral lakes is essential for their conservation and management.
Lake Liambezi is a shallow ephemeral floodplain lake situated in north-eastern Namibia. It is fed on an irregular basis by the Upper Zambezi and Kwando rivers, which overflow into the lake during years of extremely high flooding (Peel et al., 2015). The lake dried up in 1985 following 5 years of low floods in the inflowing rivers (Grobler and Ferreira, 1990). It remained largely dry until 2007, when it received a larger flood, followed by major flood in 2009, filling for the first time since the late 1970s. The lake reached a peak surface area of around 370 km2 during successive floods in 2010 and 2011 and remained inundated, despite receiving minimal inflow until 2017. The lake hosts a thriving fish community (Peel et al., 2015, 2019) and consequently supports an active subsistence fishery, based primarily on large cichlid species, particularly Oreochromis andersonii and Oreochromis macrochir, however, the ephemeral nature of Lake Liambezi also puts it at risk of overfishing (Simasiku et al., 2017). The lake also supports extensive areas of aquatic macrophytes (Seaman et al., 1978; Peel et al., 2015), including vast, fragmented stands of the emergent reeds Phragmites australis (Gramineae) and Typha capensis (Typhaceae), which| provide shelter from wind and wave action, enabling the submerged macrophytes Lagarosiphon ilicifolius (Hydrocharitaceae) and Najas horrida (Hydrocharitaceae) to flourish in shallower areas. Seaman et al. (1978) hypothesized that these macrophytes acted as a “nutrient sponge,” limiting the availability of nutrients to microalgae in the open lake, resulting in low algal growth potential. Seaman et al. (1978) and van der Waal (1985) thus considered macrophytes to be the most important primary producers supporting invertebrates and fishes in Lake Liambezi, a view widely held at the time (Howard-Williams and Junk, 1976; Soares et al., 1986; Bayley, 1989). Seaman et al. (1978) concluded that macrophytes must enter the food web mainly as detritus, and although there is some evidence that herbivores can remove an average of 40–48% of macrophyte biomass in aquatic ecosystems (Bakker et al., 2016), the direct consumption of macrophytes is rare among the Upper Zambezi ichthyofauna (van der Waal, 1985; Winemiller, 2004). Studies employing stable isotopes have since revealed that, despite being major primary producers in floodplain ecosystems, macrophytes, with some exceptions (e.g., Winemiller, 1996; Hoeinghaus et al., 2007; Zeug and Winemiller, 2008), generally contribute relatively little to aquatic food webs (Hamilton et al., 1992; Bunn and Boon, 1993; Forsberg et al., 1993; Thorp and Delong, 1994; Thorp et al., 1998; Lewis et al., 2001) and/or may have only very localized effects (Grosbois et al., 2017a). While these studies indicated that phytoplankton and periphyton, which usually constitute a small fraction of the potentially available carbon in floodplain ecosystems, are the principal carbon sources supporting invertebrates and fishes (Winemiller, 2004; Douglas et al., 2005).
This paper aims to investigate which primary production sources support consumer biomass in Lake Liambezi, a shallow ephemeral lake located in the upper Zambezi Ecoregion, to describe the trophic structure of the fish community and assess the trophic interactions among fish species, in order to discuss their potential influences in shaping its fish community. Using stable isotope analysis, mixing models and complimentary stomach contents analysis (fish only), this study set out to investigate the following hypotheses: (1) algal primary production (phytoplankton) supports a higher proportion of consumer biomass than aquatic macrophytes; (2) the lake food chain will be short, as a large component of the fish fauna comprises detritivorous and herbivorous fishes that may be consumed directly by top predators; (3) fish community trophic structure will be similar between years; and (4) with short food chains and the stochastic nature of resource availability in ephemeral systems, there will be substantial competition for food resources among fish species.
Methods
Site Description
Lake Liambezi is a shallow ephemeral floodplain lake situated in the Zambezi Region of Namibia in southern Africa (Figure 1). With an average depth of ~2.5 m, and a maximum depth of 7 m at its peak in 2010, the lake is relatively shallow (Peel et al., 2015) and annual temperatures range between 16.7 and 28.0°C, with a mean of 24.1°C (Seaman et al., 1978; Peel, 2016; Simasiku et al., 2017). Rains fall during austral summer from October to April, and are highly variable, averaging ~700 mm in the north-east, declining gradually to 500 mm in the south-west (Mendelsohn and Roberts, 1997). The annual average evaporation rate is as high as 2,500 mm (Mendelsohn and Roberts, 1997). The composition of the Lake Liambezi fish community underwent a series of successional changes from its full inundation in 2007 until 2010, but remained largely the same from 2011 to 2014, suggesting the fish community may have reached maturity (Peel et al., 2019) and comprises 46 species from 10 families (Supplementary Table 1; Peel et al., 2015).
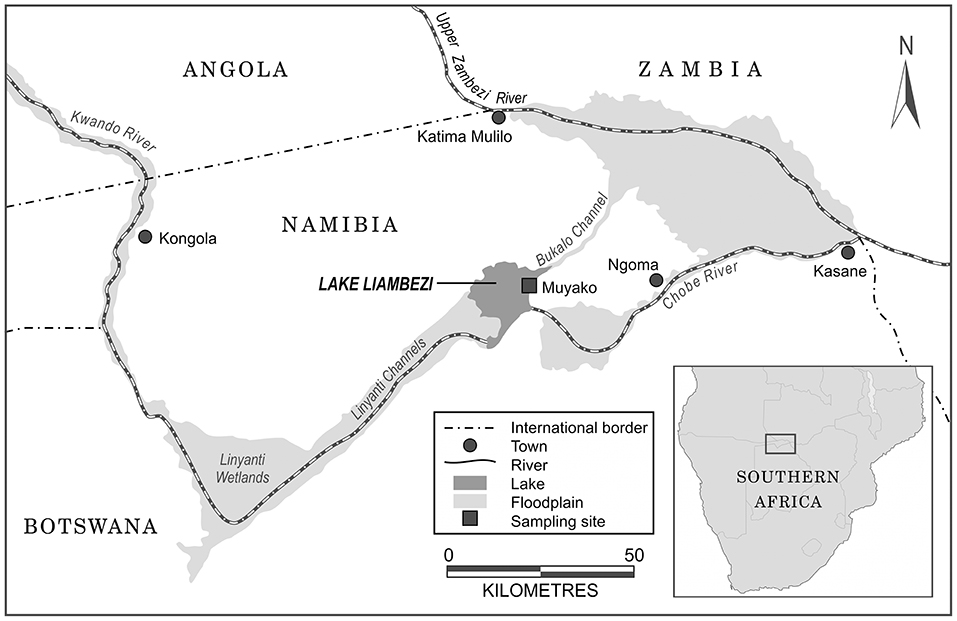
Figure 1. Map of north-eastern Namibia illustrating the rivers and floodplains feeding into Lake Liambezi, and the location of the Lake Liambezi sampling site.
Stable Isotope Analysis
Stable isotope samples were collected during August 2011 and August 2012. Limited sampling of basal resources and fishes [Oreochromis andersonii, Oreochromis macrochir, C. rendalli, and T. sparrmanii (Cichlidae), n = 11 for each species] was additionally carried out in August 2014 to investigate dietary shifts in tilapiine cichlid species between years. Fishes and their potential food sources were collected across Lake Liambezi, including littoral areas, vegetated habitats and the open lake. Fish were sampled by D-netting (3 mm mesh), electrofishing, and using standardized multifilament gill nets (110 × 2.5 m, 11 panels) with stretched mesh sizes of 12, 16, 22, 28, 35, 45, 57, 73, 93, 118, and 150 mm and baited longlines (20 m long, 20 × 9/0 circle hooks on 80 lb monofilament snoods of 1 m in length). Nets were surface set between 18:00 and 19:00 and retrieved between 06:00 and 07:00 the following morning, in the eastern half of the lake, between the inflowing Bukalo Channel and Chobe River (Figure 1), in both open water and vegetated habitats. Fish were also purchased from local fishers. For most fishes, white muscle tissue was taken above the lateral line, for fish species <30 mm (e.g., Micropanchax spp, Poeciliidae family), whole bodies were used after removing the head, intestines and scales (skin however was not removed). Aquatic invertebrates were sampled using a scoop nets and by kick sampling, and whole bodies, and/or pooled individuals of the same species, were used for each sample. Zooplankton was collected using a Bongo net (500 μm mesh) towed on the water surface at night, sorted into the broadly defined groups of herbivorous or predatory zooplankton and then analyzed as bulk, pooled (n = 10) samples. Detritus was sampled using a cone dredge and aquatic macrophytes and filamentous algae were collected by hand. Particulate organic matter (POM) was collected by filtering between 1 and 2 L surface water, onto 0.45 μm pre-combusted (at 500°C for 4 h) glass fiber filters (Advantec).
All samples were oven dried at 50°C for 24–48 h, ground to a homogenous powder and then analyzed for δ13C and δ15N using a Europa Scientific 20-20 IRMS linked to an ANCA SL Prep Unit at IsoEnvironmental cc, South African Institute for Aquatic Biodiversity, Grahamstown, South Africa. Isotope values are expressed in delta notation according to:
where X is the element and R is the ratio of the heavy over the light isotope. The δ13C and δ15N values were reported as parts per thousand (‰) relative to Vienna Pee Dee Belemnite and atmospheric nitrogen standards, respectively, and normalized to internal standards (casein and beet sugar), calibrated to the International Atomic Energy reference materials (IAEA-CH-3 and IAEA-CH-6 for δ13C, IAEA-N1 and IAEA-N2 for δ15N). Analytical precision was ± 0.07‰ for δ13C and ± 0.12‰ for δ15N for the 2011 samples and ± 0.08‰ for δ13C and ± 0.12‰ for δ15N for the 2012 samples.
Ethics approval was granted by the SAIAB Animal Ethics Committee (2013-07).
Food Web Structure
All fish samples were lipid corrected as described by Taylor et al. (2017a) and all isotope data collected in 2011 and 2012 were assessed separately, as baseline corrections for trophic positioning (e.g., Anderson and Cabana, 2007; Olsson et al., 2009) were not possible due to a lack of long-lived primary consumers living in the system. Aquatic insect and plant species were analyzed for δ13C and δ15N values and were then grouped into broad functional groups to ensure isotopically distinct resource assemblages for food-web interpretation and mixing model analyses (see below). Fishes were grouped according to a combination of taxonomic and feeding guilds as diets are often too flexible to be used solely for conclusive groupings (Yodzis and Winemiller, 1999; Jackson et al., 2001; Welcomme et al., 2006). The assessment of food web structure was based on a trophic enrichment of 1.5‰ for δ13C (Sweeting et al., 2007a), and 3.2‰ for δ15N (Sweeting et al., 2007b).
To investigate the proportional contribution of resources underpinning Lake Liambezi food webs separately for each year, Bayesian mixing models (MixSIAR; Stock and Semmens, 2016; Stock et al., 2018) were run using stable isotope data from all primary consumers (and some secondary consumers) (see Table 1) with n ≥ 10 in both 2011 and 2012 (raw data), food sources (mean ± 1 SD) and discrimination factors of 1.5 ± 0.5‰ for δ13C and 3.2 ± 0.5‰ for δ15N as described above. The model was run with Markov chain Monte Carlo (MCMC) parameters of three chains of 300,000 iterations, a burn-in phase of 200,000 and a thinning of 100. Individuals as a random effect and both residual and process error were included in the model. The model generates posterior probability distributions that can be described by average estimates of the source contributions and their associated credible intervals. Convergence and diagnostic statistics were evaluated using the Gelman–Rubin test (all variables were ≤1.05). Note that while Bayesian mixing models can include some variability in predictions through the incorporation of error terms and informative priors (Parnell et al., 2010; Stock et al., 2018), there remains a mismatch in time integration between food resources and consumers, with the latter being typically more time integrated (Phillips et al., 2014). Thus, predicted proportional resource contributions to Lake Liambezi food webs will likely display some variation over time.
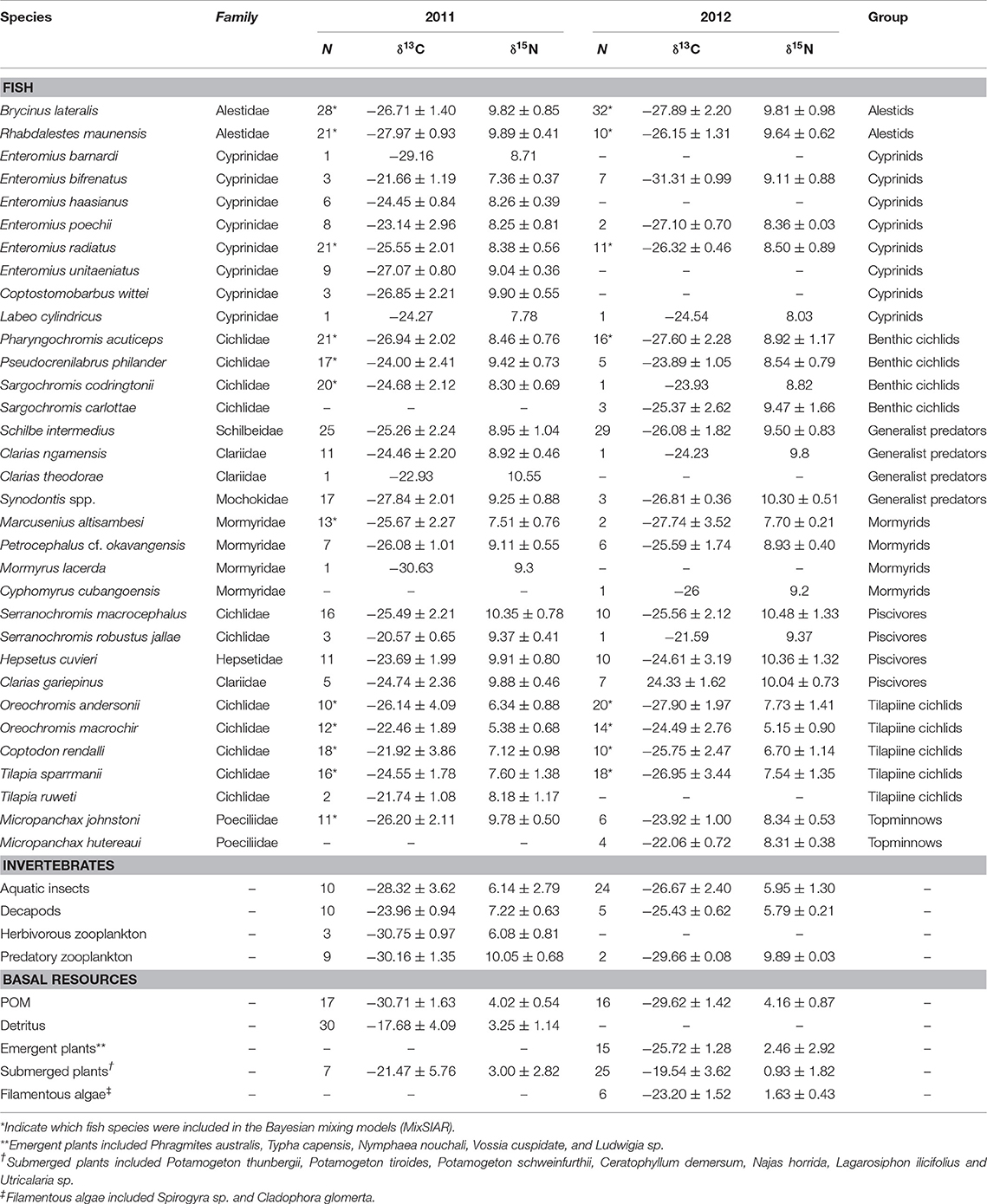
Table 1. Carbon and nitrogen stable isotope values (mean ± standard deviation) of fish species, invertebrate groups and basal carbon sources sampled in Lake Liambezi in 2011 and 2012, and the taxonomic/feeding group to which species were assigned for broad food web analysis.
Because no long lived primary consumers were found in Lake Liambezi in order to baseline our datasets, variation in the isotope values (δ13C and δ15N) of basal resources sampled in both years were assessed by multivariate statistical analyses performed using the PRIMER package, version 6 with PERMANOVA (Clarke and Gorley, 2006; Anderson et al., 2008). Analyses were performed on Euclidean distance measures of untransformed data. PERMANOVA were used to test for significant differences (α = 0.05) between years and calculated using the Type III (partial) sums of squares with 9,999 unrestricted permutations and post-hoc pair-wise PERMANOVAs (α = 0.05) were performed where appropriate. Important consumer species that showed large variation in δ13C between 2011 and 2012 were also tested for significant differences (α = 0.05) using univariate PERMANOVAs as described above. Here, the additional data collected in 2014 were used to determine whether the differences in δ13C values of the tilapiine cichlids between years represented a permanent dietary shift, or simply inter-annual dietary variation.
Community Metrics
The trophic structure of the fish community was then compared between 2011 and 2012 using six quantitative isotope metrics (Layman et al., 2007; Jackson et al., 2011); nitrogen range (NR); carbon range (CR); mean distance to centroid (CD), providing a measure of trophic diversity; mean nearest neighbor distance (MNND), which estimates the density and clustering of species within the community; standard deviation of the nearest neighbor distance (SDNND), which gives a measure of the evenness of species packing in isotopic biplot space; and the sample size corrected standard ellipse area (SEAc), which provides a bivariate measure of the mean core isotopic niche of a community. The degree of SEAc overlap between communities was calculated to give a quantitative measure of dietary similarity (Jackson et al., 2012). Only fish species collected in both years with a sample size ≥3 were included in the analyses. All metrics were calculated using the Stable Isotope Bayesian Ellipses in R (SIBER; Jackson et al., 2011) package in the R statistical computing programme (R Core Team, 2013), and bootstrapped (n = 10,000) to allow comparisons of 2011 and 2012 datasets that differed in sample size.
Trophic Structure and Species Interactions
The trophic structure of the fish community sampled in 2011 only was further resolved by calculating the SEAc of each taxonomic/feeding group, and the % of SEAc overlap between groups (as fishes were more comprehensively sampled in 2011). The SEAc and % overlap of individual species within important consumer groups were then calculated to elucidate interspecific trophic interactions and explore potential competition in the lacustrine fish community. The groups of species examined included the alestids, which have been a dominant component of the fish fauna in the lake since it filled, the tilapiine cichlids on which the fishery is based, generalist predators that include the highly abundant Schilbe intermedius (Schilbeidae), and piscivores which may influence community food web structure (van der Waal, 1985; Peel et al., 2015).
Stomach Content Analysis
To aid in interpreting the results of the stable isotope analyses, stomach contents were conducted the four most abundant, large piscivorous fish species in Lake Liambezi; S. intermedius, Clarias gariepinus (Clariidae), Hepsetus cuvieri (Hepsetidae), and Serranochromis macrocephalus (Cichlidae), which likely influenced food web structure via predation, and one small zooplanktivorous species, Brycinus lateralis (Alestidae), which was a major component of the Lake Liambezi fish fauna (Peel et al., 2015). Fish were sampled using standardized multifilament and baited longlines (see above). All fish caught were identified to species level according to Skelton (2001, 2016), measured to the nearest mm and weighed to the nearest gram. Samples of the piscivorous species were collected on five occasions between October 2013 and December 2014, and their stomach contents were examined in the field. Brycinus lateralis were sampled in February 2013, and preserved whole in the field in 10% buffered formalin. Stomach contents of B. lateralis were later examined under a dissecting microscope in the laboratory, identified to the lowest practical taxonomic level, counted and weighed to the nearest 0.1 gram after blotting dry. For B. lateralis, an indirect volumetric assessment of each food category was used (Booth and McKinlay, 2001), because food items could not be weighed with sufficient precision.
Dietary composition of the five study species was assessed by calculating the index of relative importance (IRI; Pinkas et al., 1971) of each prey item, as:
where %N and %W are the number and weight of each prey item as a percentage of all prey, and %FO is the number of stomachs containing a specific prey item as a percentage of all sampled stomachs. For B. lateralis %V, the volume of each prey item as a percentage of all prey, was used in place of %W. The IRI for each prey item was then expressed as a percentage of the sum of IRI values for all prey items (%IRI).
Results
Stable Isotope Analysis
Food Web Structure
In 2011, 424 samples were collected for stable isotope analysis, comprised of three basal carbon sources, four general invertebrate groups and 30 fish species (Table 1). In 2012, 318 samples were collected, comprising four basal carbon sources, three general invertebrate groups and 26 fish species. The δ13C values of basal resources ranged between −33 and −9‰ in 2011, and between −32 and −13‰ in 2012 (Table 1; Figure 2). POM had the lowest δ13C of all basal resources, averaging approximately −30‰ in both years. The δ13C of emergent plants and filamentous algae, both of which were only collected in 2012, averaged −26 and −23‰, respectively. Submerged plants had average δ13C values of −21 and −20‰ in 2011 and 2012, respectively. Detritus, which was only collected in 2011, was the most enriched with a δ13C value of −18‰ but was not isotopically distinct from submerged plant samples, suggesting a substantial proportion of the detritus was of submerged plant origin. The δ15N values of different basal resources sampled in 2011 were similar (~3–4‰), but in 2012, plants and filamentous algae had much lower δ15N values than POM. The isotope values of submerged plants (PERMANOVA; Pseudo-F = 2.04, P = 0.145) and POM (PERMANOVA; Pseudo-F = 3.51, P = 0.053) did not differ significantly between years. Note that although periphyton has been identified as one of the principal carbon sources supporting fish and invertebrate communities in floodplain ecosystems (Winemiller, 2004; Douglas et al., 2005), periphyton isotope data was not available for this study. However, as the isotopic values of macrophytes and associated periphyton (e.g., Neill and Cornwell, 1992; de Kluijver et al., 2015; Pettit et al., 2016) are often indistinguishable, for the purposes of interpretation, macrophytes and periphyton are considered together.
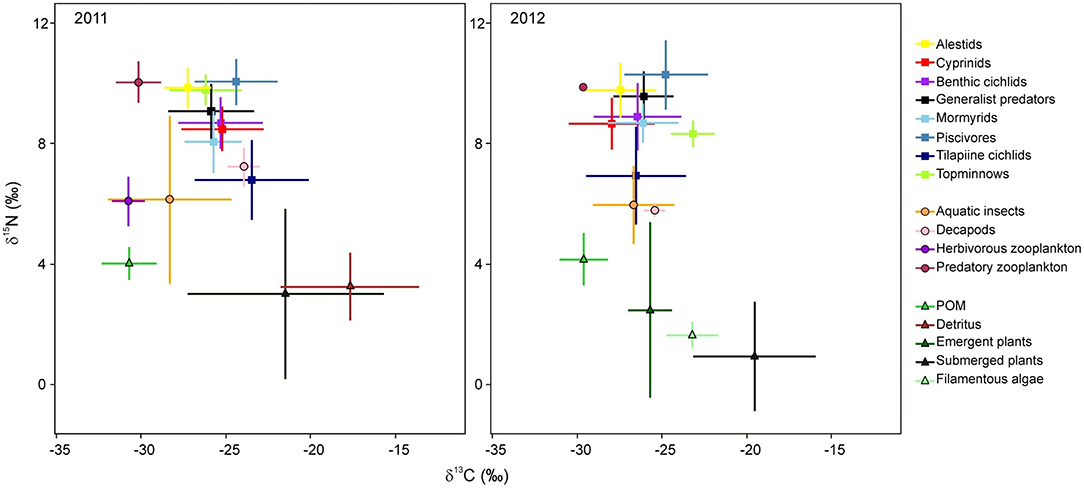
Figure 2. Stable δ13C-δ15N isotopic bi-plots of fish groups and their potential food sources from Lake Liambezi in 2011 and 2012. Data points represent the mean ± standard deviation for each group.
Overall, mixing model results for primary and secondary fish consumers showed that 58% of modeled fish species in 2011 and 87.5% in 2012 had substantial dietary POM contributions of >15%. In 2011 83% of fish species also had strong dietary contributions (>12.5%) of submerged plants. Briefly; topminnows and alestids relied almost exclusively on zooplankton and aquatic invertebrates in both years, Coptodon rendalli relied primarily on detritus and submerged plants in 2011 and decapods, POM and filamentous algae in 2012; mormyrids (data only available for 2011) were strongly opportunistic, feeding on a variety of sources including, aquatic invertebrates, zooplankton, POM, and submerged plants; tilapiine cichlids depended on submerged plants, POM and in some case zooplankton and aquatic invertebrates in 2011, vs. POM, zooplankton and filamentous algae in 2012; and benthic cichlids preferred POM, zooplankton in both years, as well as submerged plants and detritus in 2011 (Figures 3, 4, Supplementary Table 2). The low contribution values of any food source to Tilapia sparrmanii diet in 2012 suggests some basal resources may have been missed.
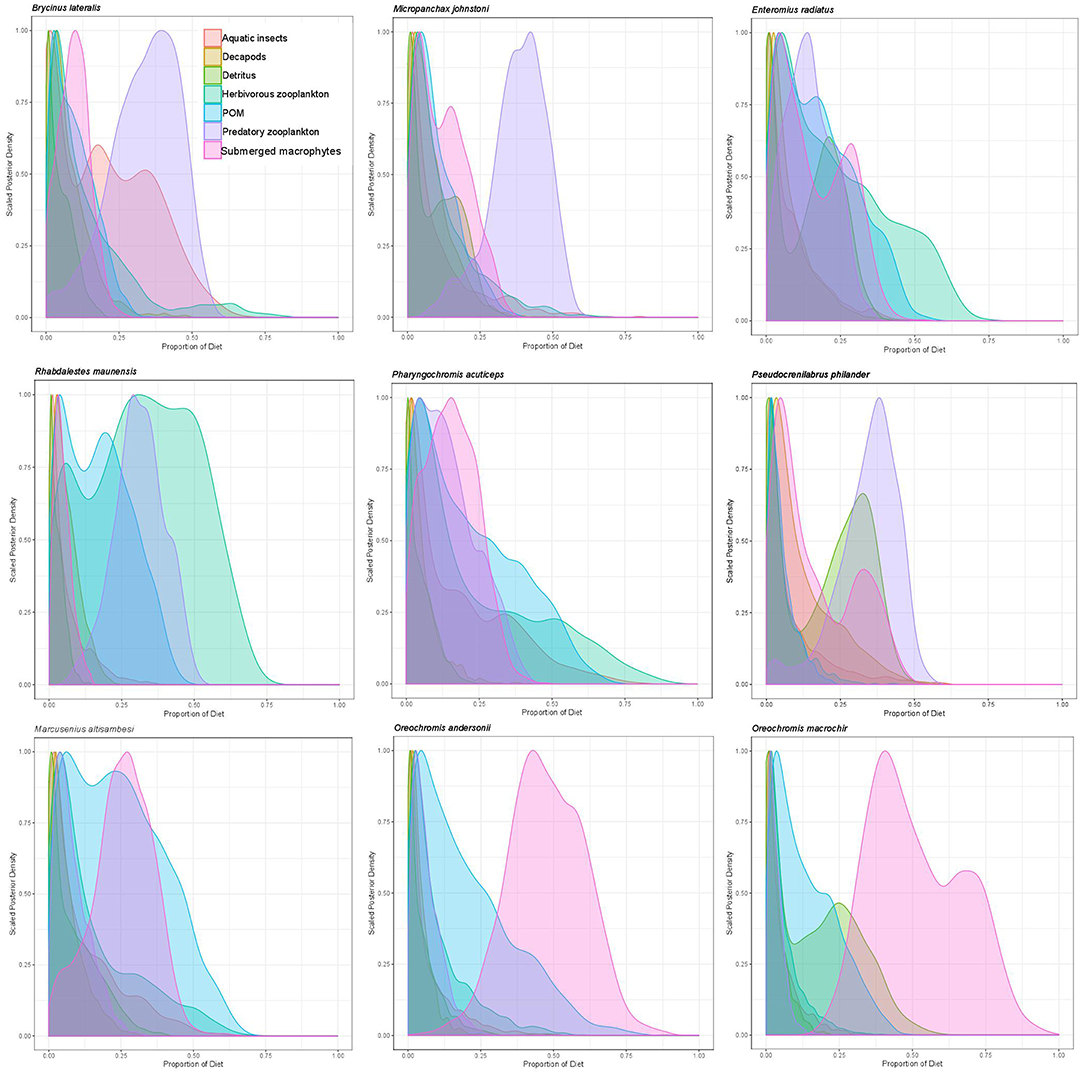
Figure 3. Proportional resource contributions to primary and secondary fish consumers as determined by Bayesian MixSIAR mixing models for 2011.
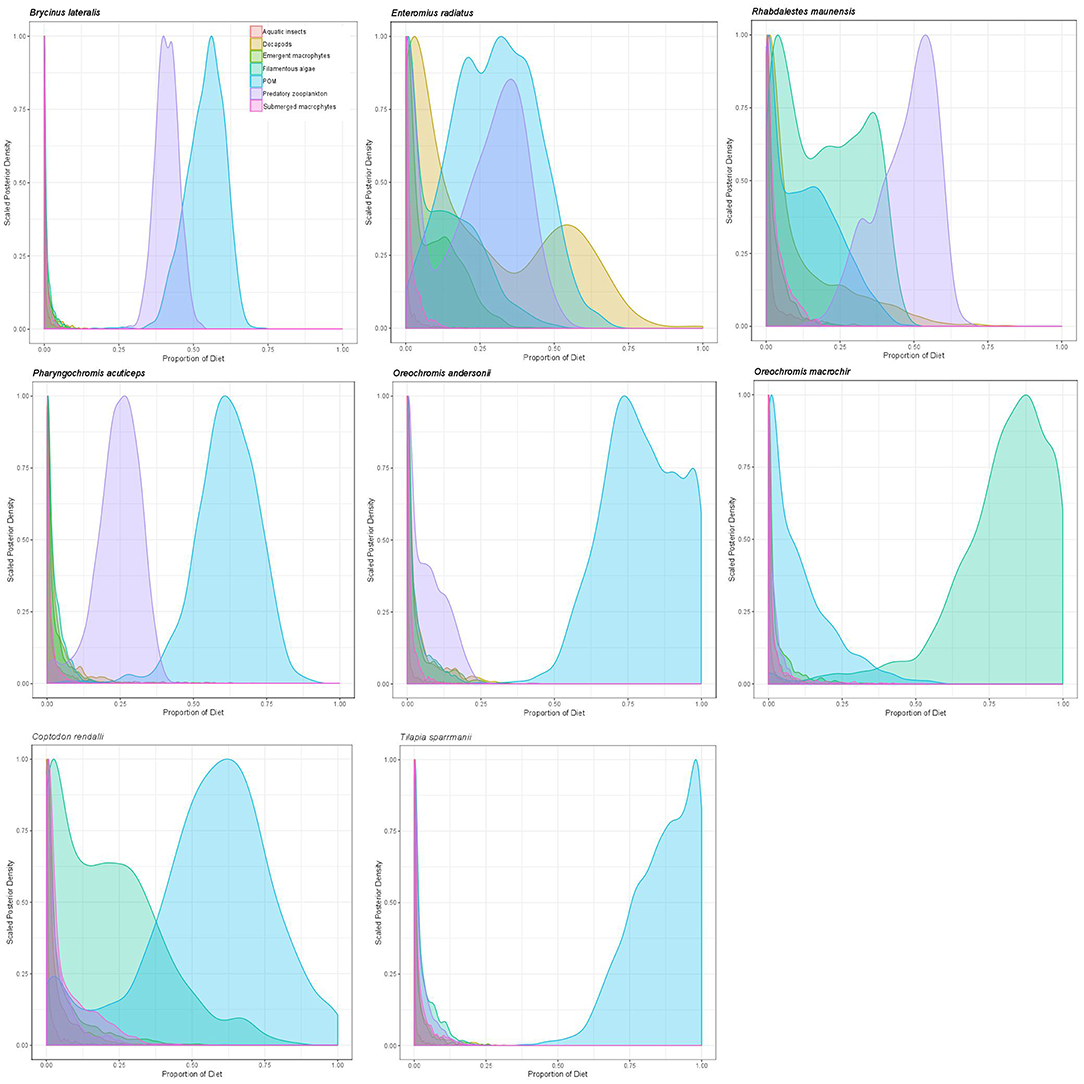
Figure 4. Proportional resource contributions to primary and secondary fish consumers as determined by Bayesian MixSIAR mixing models for 2012.
Both 2011 and 2012 data suggest that POM was the ultimate driver of the Lake Liambezi food web (either directly or indirectly), with three potential energy pathways by which basal resources were assimilated into the food web. Firstly, in 2011, the isotopic position of herbivorous zooplankton indicated that their diets likely consisted exclusively of POM. Secondly, aquatic invertebrates (including decapods in 2012) were similarly positioned, but demonstrated more variable δ13C values, indicating that while this diverse group relies primarily on POM, they likely also assimilate carbon from a range of other basal resources, including emergent plants and potentially their associated detritus and/or periphyton. The aquatic invertebrate group consisted mainly of benthic organisms, so their main source of POM likely settled out of the water column, possibly in the form of planktonic detritus (e.g., sinking phytoplankton). Thirdly, the relatively depleted values of δ15N and slightly enriched δ13C values (in 2012 only) of tilapiine cichlids relative to other fish species, suggest that although POM was a substantial contributor to tilapiine cichlid diet, considerable amounts of their dietary carbon were also derived from aquatic plants and detritus/periphyton compared to other consumers (Figure 2). However, as only Coptodon rendalli feed directly on macrophytes, this carbon was likely incorporated as detritus. As degradation does not often effect isotopic change in macrophytes (Macko and Estep, 1984; Currin et al., 1995; Fellerhoff et al., 2003; Hill and McQuaid, 2009), it is likely the sampled detritus was of submerged plant origin. All three of these primary consumers (herbivorous zooplankton; aquatic invertebrates/decapods and tilapiine cichlids) were regular dietary components for other fish species (Table 2).
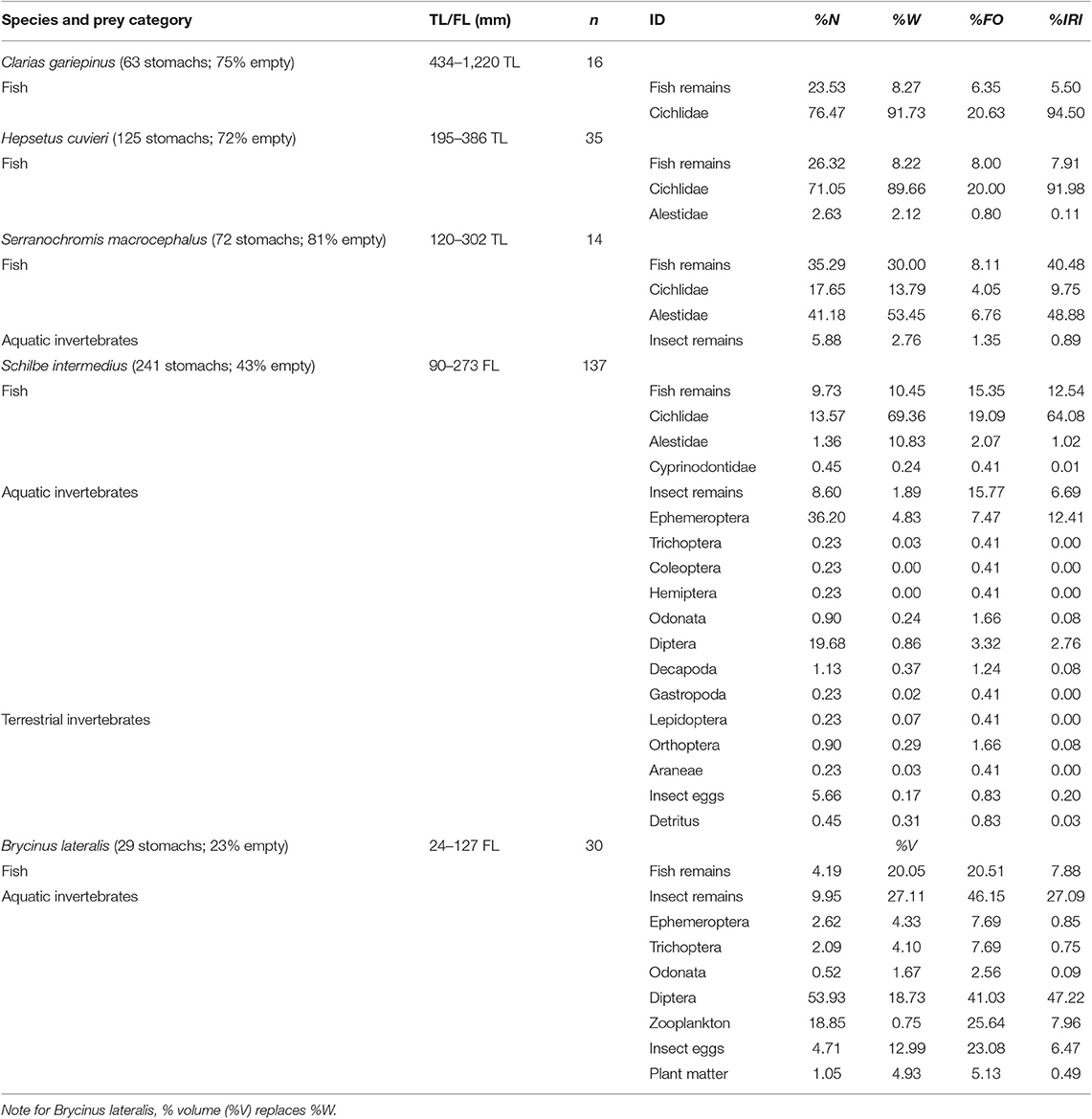
Table 2. Stomach contents of fish species (no. of stomachs; % empty) and their length, by % number (%N), % weight (%W) and % frequency of occurrence (%FO), and the % index of relative importance (%IRI) of each prey category.
The average δ13C of tilapiine cichlids showed a 3‰ depletion in 2012, possibly indicating a shift to a more POM based diet. Further sampling of the tilapiine cichlids was carried out in 2014 to establish whether the shift to a lower δ13C diet was permanent. δ13C values did not differ significantly between years for O. andersonii (PERMANOVA; Pseudo-F = 1.53, P = 0.233) or O. macrochir (PERMANOVA; Pseudo-F = 2.33, P = 0.113). However, δ13C values differed significantly between years for C. rendalli (PERMANOVA; Pseudo-F = 8.48, P = 0.001) and T. sparrmanii (PERMANOVA; Pseudo-F = 4.69, P = 0.015), with δ13C significantly lower in 2012 compared to 2011 and 2014. Two other groups of fishes showed changes in trophic position between years; cyprinids showed a depletion in δ13C, and topminnows an enrichment in δ13C coupled with decrease in δ15N between 2011 and 2012, however as minor components of the fish community, they were unlikely to alter major pathways of energy flow through the food web and were thus not investigated further. Community-wide stable isotope metrics were subsequently used to quantitatively assess the similarity of fish communities between years.
Community Metrics
The NR and CR of the fish community in 2012 were higher than in 2011, suggesting that both the trophic length and range of basal resources used by the fish community in 2012 was greater. However, the average degree of trophic diversity (CD), was similar between years. Lower MNND and SDNND in 2011 indicate greater density and evenness of species packing in bi-plot space (Table 3). For each metric, the difference between years was relatively small, and the overlap of 95% confidence intervals was substantial. The core isotopic niche area (SEAc) of the whole fish community (Figure 5A) was slightly larger in 2012 compared to 2011, corroborating results of the other metrics. The degree of overlap between years was also significant; the 2011 fish community SEAc overlapped with the 2012 fish community SEAc by 68.3%, whereas the 2012 SEAc overlapped with the 2011 SEAc by an even greater 76.8%. Overall, the tropic structure of the fish communities sampled in 2011 and 2012 were thus very similar, however, the inter-annual variations in trophic positions of the tilapiine cichlids, cyprinids and topminnows precluded the combination of both datasets. As a result, further analyses on the fish community interactions were based only on the more comprehensive 2011 dataset.
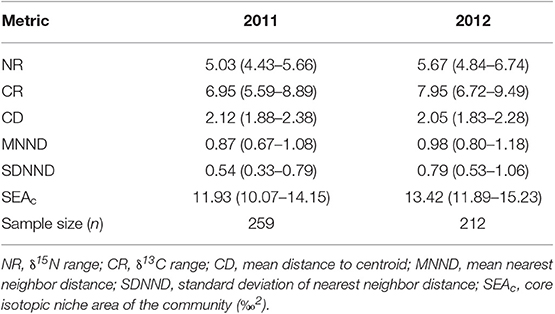
Table 3. Stable isotope community metrics (mean with 95% CI in parentheses) comparing the trophic structure of fish communities sampled from Lake Liambezi in 2011 and 2012.
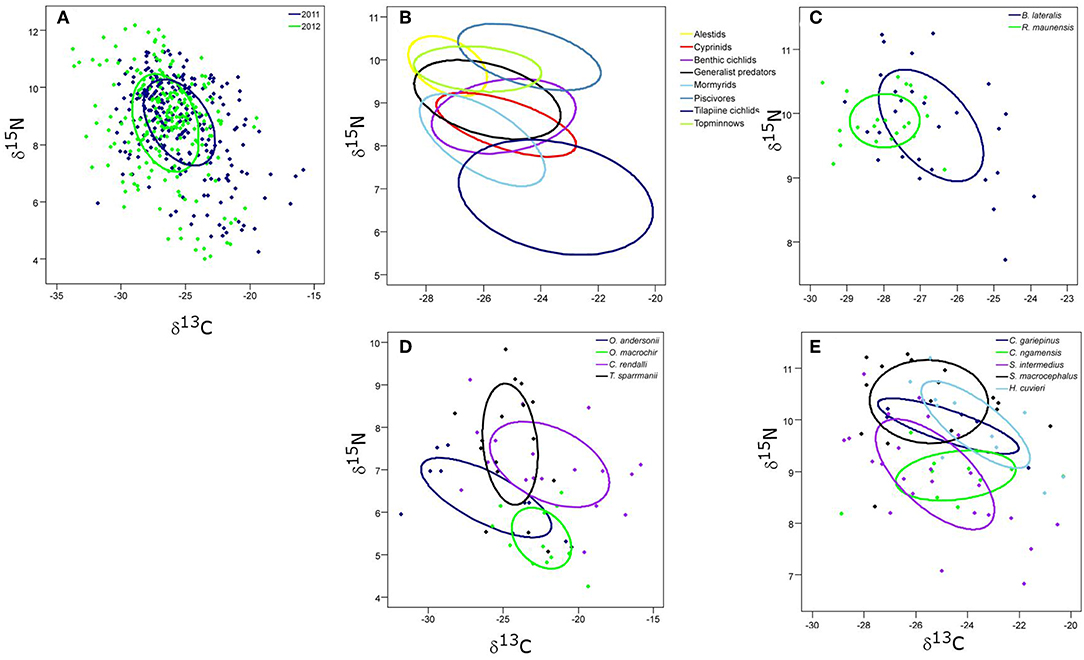
Figure 5. The core isotopic niche areas (SEAc) of (A) 2011 and 2012 complete fish communities; (B) all fish groups; (C) alestid species; (D) the four tilapiine cichlid species; and (E) the predatory fish species in Lake Liambezi in 2011.
Trophic Structure and Species Interactions
Tilapiine cichlids, despite being the backbone of the Lake Liambezi gill net fishery, made up only 4% of the CPUE (kg net−1 night−1) (Supplementary Table 1). They occupied the lowest trophic position in the overall fish community and did not show much SEAc overlap with other groups, indicating that they are the only primary consumers in the fish community. Their SEAc was large, suggesting higher trophic diversity compared to other groups. At the next trophic level there was considerable overlap in isotopic space between cyprinids, benthic cichlids, mormyrids and generalist predators, all of which had similar sized SEAc. These four groups were most likely primarily dependent upon aquatic invertebrates for their food. Alestids and topminnows occupied a slightly higher trophic level, with considerable SEAc overlap between the two groups. Alestids had the smallest SEAc of any group, indicative of dietary specialization. Their elevated trophic position and relatively low δ13C reflects a diet composed of both herbivorous and predatory zooplankton. Piscivores occupied the highest trophic level in Lake Liambezi and overlapped relatively little with other groups (Figure 5B; Table 4). The trophic positions of individual fish species within important consumer groups were examined further to elucidate interspecific trophic interactions.
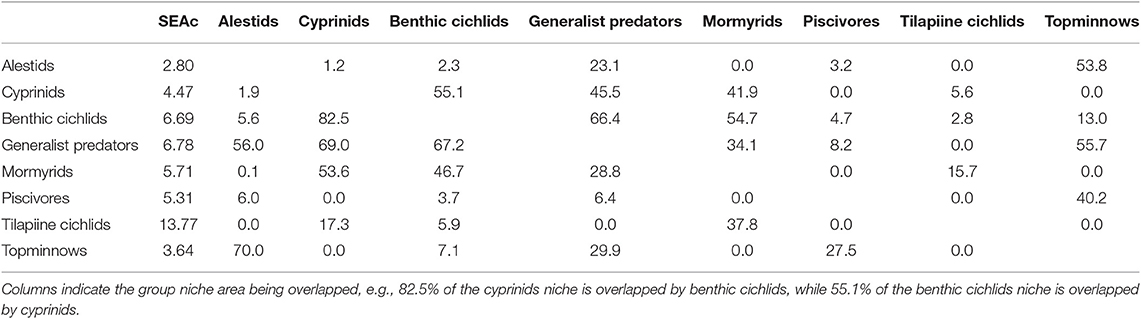
Table 4. The core isotopic niche area (SEAc) and percent overlap between fish groups in Lake Liambezi in 2011.
The trophic level of the alestids B. lateralis and Rhabdalestes maunensis was similar to that of the piscivores in Lake Liambezi. Brycinus lateralis, the larger of the two alestids, had a SEAc of 3.36‰2. Rhabdalestes maunensis had a significantly smaller SEAc of 1.24‰2, 48.9% of which was overlapped by B. lateralis. In contrast, only 18.1% of the core niche of B. lateralis was overlapped by R. maunensis (Figure 5C).
Of the four tilapiine cichlids, O. macrochir had the smallest SEAc and lowest trophic position, indicating this species was a highly specialized primary consumer (Figure 5D). The much larger isotopic niche of O. andersonii overlapped with that of O. macrochir by approximately one third (Table 5). Oreochromis andersonii generally had a lower δ13C and higher δ15N than O. macrochir. Coptodon rendalli had the largest SEAc of the four species, perhaps reflective of the extremely variable isotope values of submerged plants. Coptodon rendalli occupied a higher trophic level than both Oreochromis spp. and did not overlap with either. It did, however, overlap with T. sparrmanii which had a slightly smaller and more laterally compressed SEAc, suggesting a narrower range of basal resources, but large variation in trophic level.
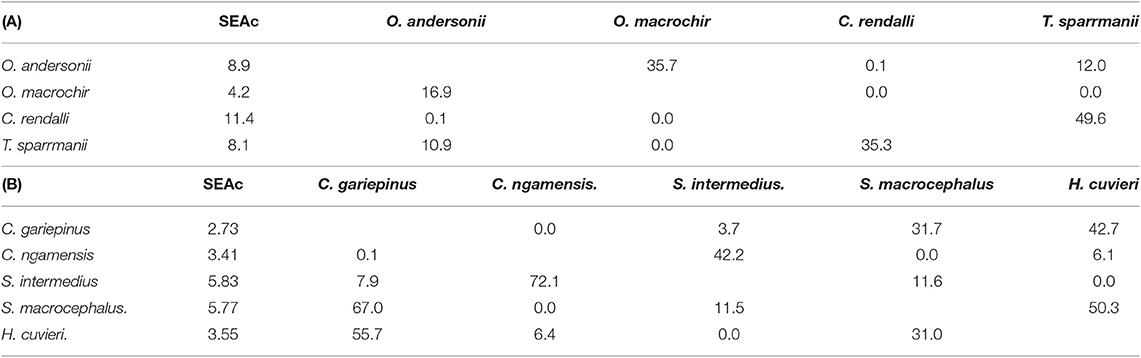
Table 5. The core isotopic niche area (SEAc) and percent overlap between (A) tilapiine cichlid species and (B) predatory fish species in Lake Liambezi in 2011.
The core niche areas of piscivores and generalist predators were examined together (Figure 5E), with the two most abundant generalist predators, S. intermedius and Clarias ngamensis occupying a lower trophic level than the top piscivores. Schilbe intermedius had the larger SEAc of the two species, which overlapped considerably with that of C. ngamensis (Table 5). There was also extensive SEAc overlap among the three piscivores. Half the SEAc of H. cuvieri, and two thirds the SEAc of C. gariepinus were overlapped by S. macrocephalus. Serranochromis macrocephalus had a similarly large SEAc size to that of S. intermedius. The large isotopic niche of S. macrocephalus revealed by stable isotope analysis is contrary to the dietary specialization inferred from stomach contents analysis. However, the smaller SEAc of H. cuvieri and C. gariepinus appear to confirm that they are relatively specialized piscivores. For both H. cuvieri and C. gariepinus, δ15N tended to decrease with increasing δ13C.
Stomach Contents Analysis
Clarias gariepinus and H. cuvieri gut contents comprised exclusively fish, while S. macrocephalus also preyed on aquatic invertebrates (Table 2). Cichlidae were the only fish identified in the stomachs of C. gariepinus. In addition to cichlids, alestids were among the prey of H. cuvieri and S. macrocephalus. Cichlidae constituted the bulk of H. cuvieri prey, while alestids were more important to S. macrocephalus. The most frequently identified cichlid prey species were Tilapia sparrmanii and Pharyngochromis acuticeps but Oreochromis spp. also contributed substantially to the diet of C. gariepinus.
A diverse range of prey were recorded from full stomachs of S. intermedius, including fish, aquatic and terrestrial invertebrates, insect eggs, and detritus (Table 2). Fish, cichlids in particular, were the most important food source for S. intermedius in Lake Liambezi, with the most commonly identified cichlid species being T. sparrmanii and P. acuticeps. Ephemeroptera were the only insect order to contribute substantially to the diet of S. intermedius.
Identifiable items in full stomachs of B. lateralis included fish, aquatic invertebrates, insect eggs and plant matter (Table 2). A large portion of the aquatic invertebrate prey were masticated and could not be identified further. Dipteran larvae, primarily Chaobrus spp., were the most important prey item identified, accounting for nearly half of the diet of B. lateralis. Planktonic crustacea and fish had similar contributions, followed by insect eggs.
Discussion
Primary Production Sources Supporting Fishes
Stable isotopic values of POM indicated that it was composed primarily of freshwater phytoplankton (see Cloern et al., 2002; O'Reilly et al., 2002; Harding and Hart, 2013; Morana et al., 2015) and was clearly the primary carbon source supporting consumer biomass in Lake Liambezi, however substantial contributions from aquatic macrophytes (including associated detritus and periphyton), the most conspicuous source of primary production in the lake, were also present. This highlights the importance of algal (here phytoplankton and/or periphyton) carbon in aquatic food webs, even when aquatic macrophytes are the dominant primary producers (Araujo-Lima et al., 1986; Forsberg et al., 1993; Lewis et al., 2001; Herwig et al., 2004). Terrestrial C3 macrophytes can also be important carbon sources in aquatic food webs (e.g., Berggren et al., 2014), particularly when algal growth is light limited (Zeug and Winemiller, 2008; Roach and Winemiller, 2015). However, the probability of a substantial contribution of terrestrial carbon in Lake Liambezi, except during the filling phase, was deemed small due to the large surface area of the lake relative to its circumference, and because benthic fish are not restricted to nearshore areas that would receive greater terrestrial carbon inputs. This differs considerably from riverine food webs which in this region are supported mainly by riparian vegetation from the floodplains and detritus from aquatic macrophytes (Taylor et al., 2017b).
Trophic Pathways
Carbon from phytoplankton and aquatic macrophytes was assimilated into the food web via three major pathways, one pelagic and two benthic. These are summarized visually in Figure 6.
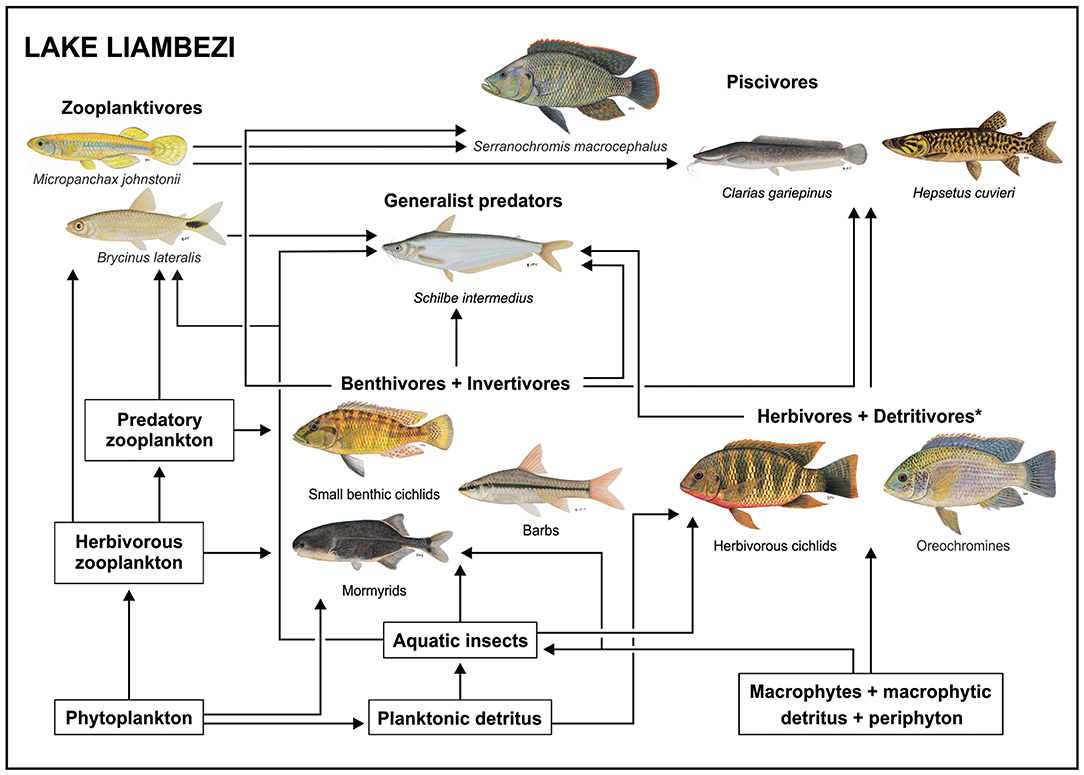
Figure 6. Conceptual diagram of the food web in Lake Liambezi, illustrating the flow of energy through three major food chains, and their integration by generalist predators and piscivores in the mature lacustrine fish community. *Herbivores and detritivores incorporate all the tilapiine cichlids. All fish images are ©NRF-SAIAB.
In the pelagic food web phytoplankton was assimilated directly by herbivorous zooplankton, such as calanoid copepods (Grosbois et al., 2017b) or cladocerans (e.g., Bosmina longirostis, Seaman et al., 1978) that are preyed upon by larger zooplankton, such as cyclopoid copepods and chaoborid larvae, as well as small zooplanktivorous fishes that include the poecilid Micropanchax johnstonii and the alestids B. lateralis and R. maunensis. Stomach content analysis showed that zooplankton and Diptera larvae (aquatic invertebrates) made up a large portion of the diet of B. lateralis, yet while isotope data generally supported these observations, the δ15N values revealed that B. lateralis and zooplankton occupied the same trophic level. This may be due to several factors including different tissue turnover rates among species, variable δ15N values among sampling periods, and seasonal variation in the abundance of zooplankton and dipteran larvae and/or their importance in the diet of B. lateralis, as seasonal variation in diet is well-documented for Brycinus species (e.g., Booth and McKinlay, 2001).
Thus, the second major pathway by which basal carbon was assimilated into the food web was via aquatic invertebrates that feed primarily on detritus of planktonic origin or potentially on emergent macrophytes and associated macrophytic detritus and/or periphyton. Among the most important aquatic invertebrates in Lake Liambezi are diptera larvae, ephemeroptera, trichoptera, and odonata nymphs (Seaman et al., 1978). These support benthic invertivorous mormyrids, cichlids, barbs, and to a lesser degree, generalist predators, such as S. intermedius. The mormyrids Petrocephalus cf. okavangensis and Marcusenius altisambesi are some of the most specialized insectivorous fishes in Lake Liambezi and feed primarily on the dominant aquatic insect groups mentioned above (van der Waal, 1985; Winemiller and Adite, 1997), but also showed a reliance on POM and submerged plants in the mixing models. The benthic invertivore cichlid group comprised four species, the small sized P. acuticeps and Pseudocrenilabrus philander, and two medium sized Sargochromis species. The diets of these cichlids were more diverse and flexible than those of the mormyrids (van der Waal, 1985; Winemiller, 1991; Zengeya and Marshall, 2007), incorporating substantial contributions from all available food resources. As members of this group are important prey species of the top piscivores (Table 2), they play an important role in moving energy up the food web.
The third major pathway involves the tilapiine cichlids which include O. andersonii, O. macrochir, T. sparrmanii and C. rendalli. This group occupies the lowest trophic level of all the fish in the lake, indicating that they were largely primary consumers. The isotopic positioning of the tilapiine cichlids indicated reliance on POM (particularly in 2012) as well as on submerged (in 2011) and emergent plants (2012) and/or detritus. Coptodon rendalli is regarded as being a specialized macrophyte feeder (van der Waal, 1985; Winemiller and Kelso-Winemiller, 2003) which is consistent with the relatively high average δ13C values and supports the mixing model which showed a reliance on submerged plants, filamentous algae, detritus and POM. Tilapia sparrmanii incorporated large contributions of POM into its diet, along with smaller amounts of zooplankton, aquatic invertebrates and submerged plants. In 2011, the isotopic niche of T. sparrmanii overlapped with that of C. rendalli by approximately one third, supporting mixing model results and previous dietary observations that T. sparrmanii consume a small amount of aquatic macrophytes (van der Waal, 1985; Winemiller and Kelso-Winemiller, 2003; Zengeya and Marshall, 2007). The higher average δ15N value over other tilapiine cichlids suggests that T. sparrmanii is likely more omnivorous, feeding at multiple trophic levels, which is supported by van der Waal (1985) who recorded a variety of aquatic and terrestrial invertebrates, fish remains and zooplankton in the diet of T. sparrmanii in the lake. Data from 2011 also showed that the larger Oreochromis species had lower δ15N values, and shared little isotopic niche space with C. rendalli and T. sparrmanii, whereas Oreochromis andersonii had a similarly wide δ13C range to that of C. rendalli but was on average 4‰ more depleted. Mixing models suggested a reliance on POM in both years, as well as submerged macrophytes in 2011. Oreochromis andersonii with lower δ13C values were probably more reliant on algal carbon sources, while those with higher δ13C values probably consumed more detritus derived from the abundant aquatic macrophytes, demonstrating some degree of individual specialization (Gu et al., 1997; Benhaïm et al., 2017). In contrast, O. macrochir occupied a small isotopic niche, and a lower trophic position than O. andersonii, although mixing models suggested they also fed on POM, detritus and submerged plants (2011) as well as POM and filamentous algae (2012). The low levels of dietary overlap support observations by van der Waal (1985) who identified algae and zooplankton as the most important food sources for O. andersonii and fine detritus as the main food source for O. macrochir. Of note is that the tilapiine cichlids, despite being the backbone of the Lake Liambezi fishery, accounted for a minimal proportion of this study's CPUE. This is likely indicative of strong fishing pressure in Lake Liambezi, where subsistence fishers are capable of removing substantial biomass (i.e., 27 00 t/year in 2011–2012; Peel et al., 2015).
Integrating Trophic Pathways
While the three trophic pathways discussed above are by no means discrete (fishes may forage across pelagic and benthic food chains), predators occupying the highest trophic levels fully integrate all pathways (Vander Zanden and Vadeboncoeur, 2002). The dominant predator in Lake Liambezi in terms of biomass was S. intermedius (Supplementary Table 1; Peel et al., 2015, 2019), a generalist predator, which consumed a wide variety of prey items including fish, aquatic and terrestrial invertebrates, decapods and gastropods. The broad, but fish-dominated diet of S. intermedius observed during the present study was consistent with previous observations from Lake Liambezi (van der Waal, 1985) and with dietary (Merron and Mann, 1995; Winemiller and Kelso-Winemiller, 1996) and trophic (Taylor et al., 2017b) assessments in neighboring rivers. Schilbe intermedius shared a significant amount of isotopic niche space with C. ngamensis, which also feeds on a wide variety of fish, insects, molluscs, and decapods (Willoughby and Tweddle, 1978; van der Waal, 1985; Winemiller and Kelso-Winemiller, 1996). These two species occupied a lower trophic position than the top predators, overlapping more with benthic cichlids, cyprinids and mormyrids.
Stomach contents and stable isotope analysis revealed a significant level of dietary overlap among the three piscivores C. gariepinus, H. cuvieri and S. macrocephalus. Clarias gariepinus and H. cuvieri fed almost exclusively on cichlids, while S. macrocephalus fed predominantly on alestids. Although C. gariepinus is known to have a broad omnivorous diet (Willoughby and Tweddle, 1978; Bruton, 1979; van der Waal, 1985; Winemiller and Kelso-Winemiller, 1996), they often specialize on a fish prey (Merron, 1993; Weyl et al., 2016). Stable isotope analysis provided strong support for the high contribution of both benthic and tilapiine cichlids to the diets of C. gariepinus and H. cuvieri, and clearly illustrated how these species integrated the two benthic trophic pathways described above. Variation in trophic position of individual C. gariepinus and H. cuvieri suggests some degree of specialization. Individuals with depleted δ13C values had high trophic positions, reflecting the greater number of trophic transfers involved in obtaining energy from omnivorous and insectivorous benthic cichlids. Individuals with enriched δ13C values had lower trophic positions, as fewer trophic transfers were involved in obtaining energy from the detritivorous and herbivorous tilapiine cichlids. Serranochromis macrocephalus were, on average, slightly more enriched in δ15N and depleted in δ13C compared to C. gariepinus and H. cuvieri, as they fed more evenly across all three trophic pathways indicating a more diverse diet than the alestid-dominated gut content analysis would suggest. As a result, B. lateralis the most abundant species in the lake (Peel et al., 2015), appears relatively poorly utilized as a prey resource and its occupation of the pelagic food web may be facilitated by predator release.
The use of the pelagic food chain, even if on a small scale, by B. lateralis may well have been facilitated by the absence of Hydrocynus vittatus, a large pursuit predator, from the lake (Peel et al., 2015). Where it occurs, H. vittatus exerts top-down control on fish communities, restricting smaller fishes to shallow littoral refugia and to macrophyte beds (Jackson, 1961). Comparing the food web of Lake Liambezi with that developed by Taylor et al. (2017b) for the upper Zambezi River demonstrates this idea (Figure 7). While the Zambezi River fish community is richer because it includes several species that were unable to colonize Lake Liambezi, zooplanktivory is only represented by M. johnstonii, a small poecilid that is strongly associated with aquatic vegetation (Skelton, 2001). In the Zambezi River B. lateralis occupies a benthic insectivore niche (Figure 7). This is different to Lake Liambezi, where we propose that, in the absence of H. vittatus resulted in predation release which facilitated the use of pelagic space by B. lateralis allowing it to make use of an unexploited niche and exit the more competitive food chain driven by benthic invertebrates.
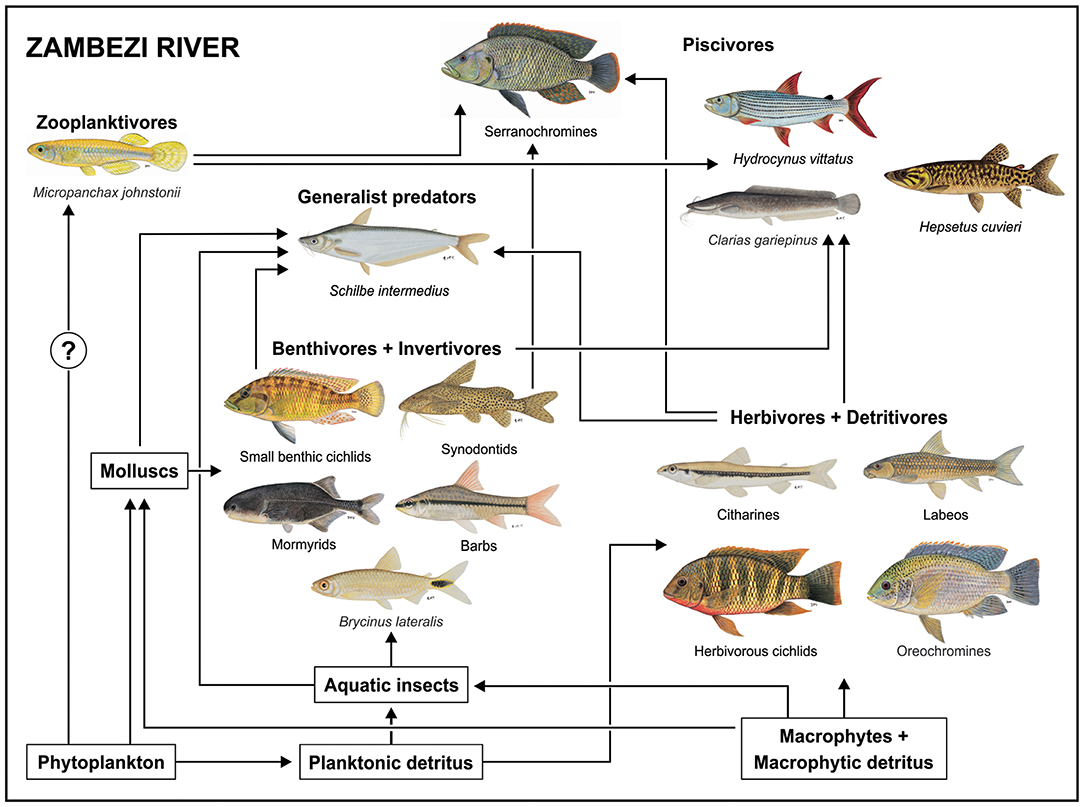
Figure 7. Conceptual diagram of the food web in the Zambezi River as described by Taylor et al. (2017b), illustrating the flow of energy through three major food chains, and their integration by generalist predators and piscivores in the riverine fish community. All fish images are ©NRF-SAIAB.
In summary, the investigations into trophic and food web structure supports the hypothesis that phytoplankton production supports a high amount of consumer biomass in Lake Liambezi, however substantial contributions by periphyton/emergent plants are also likely. These results add to the large body of evidence highlighting the importance of algal production sources to fishes, even where macrophytes appear to be the dominant source of primary production (Winemiller, 2004; Douglas et al., 2005). Submerged and emergent macrophytes (including associated detritus and potentially periphyton) do however, contribute to the assimilated diets of the tilapiine cichlids, which are the mainstay of the commercial gillnet fishery on the lake (Peel et al., 2015). Of the three major food chains, the phytoplankton based pelagic food chain was longest. The benthic food chain based on detritus of planktonic origin was characterized by high levels of omnivory and the macrophytic detritus-based food chain was shortest. Predators fed across all three food chains, but more so on the two benthic food chains. A combination of dietary overlap, dietary specialization, the integration of multiple food chains and the behavioral adaptation to changing dietary resources, underpins the ability of Lake Liambezi's fish community to thrive under the stochastic nature of ephemeral ecosystems. Unfortunately, the development of a substantial subsistence tilapiine fishery on Lake Liambezi (Peel et al., 2015, 2019) combined with the recession of lake water after 2014 resulted in the collapse of the cichlid fishery in 2016 (Peel et al., 2019; Tweddle, personal observation). The management of Lake Liambezi during its next cycle of inundation should likely focus on monitoring size and abundance of fish species involved in the longer phytoplankton based pelagic food chain, as its length and complexity would contribute to ecosystem stability. Management of catch quotas and acceptable fishing gears may also be needed in future to prevent fishery collapse (and its knock-on economic effects on subsistence livelihoods, see Simasiku et al., 2017) before the start of the subsequent drying cycle.
Data Availability Statement
The datasets generated for this study are available on request to the corresponding author.
Ethics Statement
The animal study was reviewed and approved by South African Institute for Aquatic Biodiversity Animal Ethics Committee.
Author Contributions
RP and OW: ideas, data collection, analysis, manuscript preparation, and funding. JH: ideas, analysis, and manuscript preparation. GT: ideas, data collection, and analysis.
Funding
This research collaboration was facilitated by a South African–Namibia Research Partnership Programme Grant (National Research Foundation NRF Grant No.: UID 74015). Fieldwork was funded through the Namibia Nature Foundation (NNF) managed Integrated Community-Based Ecosystem Management (ICEMA) Project, the Nedbank Namibia Go-Green Fund, the Southern African Science Service Center for Climate Change and Adaptive Land Management (SASSCAL), NNF/WWF Zambezi/Chobe Fisheries Project, the EU/NNF Community Conservation Fisheries in KAZA project, Rhodes University, Department of Environmental Affairs: National Resource Management Programme, Working for Water Programme, and the South African Research Chairs Initiative of the Department of Science and Technology (Grant No. 110507).
Conflict of Interest
The authors declare that the research was conducted in the absence of any commercial or financial relationships that could be construed as a potential conflict of interest.
Acknowledgments
We gratefully acknowledge the Ministry of Fisheries and Marine Resources, Namibia, for granting permission to carry out this research and for providing technical and logistic support. We acknowledge use of infrastructure and equipment provided by the SAIAB Research Platform and the funding channelled through the NRF-SAIAB Institutional Support system. Special thanks to Denis Tweddle for logistical, technical and intellectual support. Thank you to Susan Abraham for drawing our stylized food web diagrams
Supplementary Material
The Supplementary Material for this article can be found online at: https://www.frontiersin.org/articles/10.3389/fenvs.2019.00192/full#supplementary-material
References
Anderson, C., and Cabana, G. (2007). Estimating the trophic position of aquatic consumers in river food webs using stable nitrogen isotopes. J. North Am. Benthol. Soc. 26, 273–285. doi: 10.1899/0887-3593(2007)26[273:ETTPOA]2.0.CO;2
Anderson, M. J., Gorley, R. N., and Clarke, K. R. (2008). PERMANOVA+ for PRIMER: Guide to Software and Statistical Methods. Plymouth: PRIMER-E.
Araujo-Lima, C. A., Forsberg, B. R., Victoria, R., and Martinelli, L. (1986). Energy sources for detritivorous fishes in the Amazon. Science 234, 1256–1258. doi: 10.1126/science.234.4781.1256
Bakker, E. S., Wood, K. A, Pagès, J. F., Veen, G. F., Christianen, M. J. A., Santamaría, L., et al. (2016). Herbivory on freshwater and marine macrophytes: a review and perspective. Aquat. Bot. 135, 18–36. doi: 10.1016/j.aquabot.2016.04.008
Baron, J. S., Poff, N. L., Angermeier, P. L., Dahm, C. N., Gleick, P. H., Hairston, N. G., et al. (2002). Meeting ecological and social needs for freshwater. Ecol. Appl. 12, 1247–1260. doi: 10.1890/1051-0761(2002)012[1247:MEASNF]2.0.CO;2
Bayley, P. B. (1989). Aquatic environments in the Amazon basin, with an analysis of carbon sources, fish production, and yield. Can. Spec. Publ. Fish. Aquat. Sci. 106, 399–408.
Benhaïm, D., Akian, D. D., Ramos, M., Ferrari, S., Yao, K., and Bégout, M.-L. (2017). Self-feeding behaviour and personality traits in tilapia: a comparative study between Oreochromis niloticus and Sarotherodon melanotheron. Appl. Anim. Behav. Sci. 187, 85–92. doi: 10.1016/j.applanim.2016.12.004
Berggren, M., Ziegler, S. E., St-Gelais, N. F., Beisner, B. E., and del Giorgio, P. A. (2014). Contrasting patterns of allochthony among three major groups of crustacean zooplankton in boreal and temperate lakes. Ecology 95, 1947–1959. doi: 10.1890/13-0615.1
Booth, A. J., and McKinlay, B. W. (2001). Spatial aspects of the reproductive and feeding biology of the striped robber, Brycinus lateralis (Pisces: Characidae), in the Okavango Delta, Botswana. Afr. Zool. 36, 31–40. doi: 10.1080/15627020.2001.11657111
Bruton, M. N. (1979). The food and feeding behaviour of Clarias gariepinus (Pisces: Clariidae). in Lake Sibaya, South Africa, with emphasis on its role as a predator of cichlids. Trans. Zool. Soc. Lond. 35, 47–114. doi: 10.1111/j.1096-3642.1979.tb00057.x
Bunn, S. E., and Boon, P. I. (1993) What sources of organic carbon drive food webs in billabongs? A study based on stable isotope analysis. Oecologia 96, 85–94.
Carmouze, J. P., Durand, J. R., and Leveque, C. (1983). Lake Chad: Ecology and Productivity of a Shallow Tropical Ecosystem. The Hague: Dr. W. Junk Publishers, 575. doi: 10.1007/978-94-009-7266-7
Cloern, J. E., Canuel, E. A., and Harris, D. (2002). Stable carbon and nitrogen isotope composition of aquatic and terrestrial plants of the San Francisco Bay estuarine system. Limnol. Oceanogr. 47:713–729. doi: 10.4319/lo.2002.47.3.0713
Currin, C., Newell, S., and Paerl, H. (1995). The role of standing dead Spartina alterniflora and benthic microalgae in salt marsh food webs: considerations based on multiple stable isotope analysis. Mar. Ecol. Prog. Ser. 121, 99–116. doi: 10.3354/meps121099
de Kluijver, A., Ning, J., Liu, Z., Jeppesen, E., Gulati, R. D., and Middelburg, J. J. (2015). Macrophytes and periphyton carbon subsidies to bacterioplankton and zooplankton in a shallow eutrophic lake in tropical China. Limnol. Oceanogr. 60, 375–385. doi: 10.1002/lno.10040
Douglas, M. A., Bunn, S. E., and Davies, P. M. (2005). River and wetland food webs in Australia's wet–dry tropics : general principles and implications for management. Mar. Freshw. Res. 56, 329–342. doi: 10.1071/MF04084
Dumont, H. J. (1992). The regulation of plant and animal species and communities in African shallow lakes and wetlands. Rev. Hydrobiol. Trop. 25, 303–346.
Fellerhoff, C., Voss, M., and Wantzen, K. M. (2003). Stable carbon and nitrogen isotope signatures of decomposing tropical macrophytes. Aquatic Ecol. 37, 361–375. doi: 10.1023/B:AECO.0000007049.25535.12
Forsberg, B. R., Araujo-Lima, C. A. R. M., Martinelli, L. A., Victoria, R. L., and Bonassi, J. A. (1993). Autotrophic carbon sources for fish of the central Amazon. Ecology 74, 643–652. doi: 10.2307/1940793
Grosbois, G., Del Giorgio, P. A., and Rautio, M. (2017a). Zooplankton allochthony is spatially heterogeneous in a boreal lake. Freshw. Biol. 62, 474–490. doi: 10.1111/fwb.12879
Grosbois, G., Mariash, H., Schneider, T., and Rautio, M. (2017b). Under-ice availability of phytoplankton lipids is key to freshwater zooplankton winter survival. Sci. Rep. 7:11543. doi: 10.1038/s41598-017-10956-0
Gu, B., Schelske, C. L., and Hoyer, M. V. (1997). Intrapopulation feeding diversity in blue tilapia: evidence from stable isotope analysis. Ecology 78, 2263–2266. doi: 10.1890/0012-9658(1997)078[2263:IFDIBT]2.0.CO;2
Hamilton, S. K., Lewis, W. M., and Sippel, S. J. (1992). Energy sources for aquatic animals in the Orinoco River floodplain: evidence from stable isotopes. Oecologia 89, 324–330. doi: 10.1007/BF00317409
Harding, W. R., and Hart, R. C. (2013). Food-web structure in the hypertrophic Rietvlei Dam based on stable isotope analysis: specific and general implications for reservoir biomanipulation. Water SA 39, 611–614. doi: 10.4314/wsa.v39i5.5
Herwig, B. R., Soluk, D. A., Dettmers, J. M., and Wahl, D. H. (2004). Trophic structure and energy flow in backwater lakes of two large floodplain rivers assessed using stable isotopes. Can. J. Fish. Aquat. Sci. 61, 12–22. doi: 10.1139/f03-139
Hill, J. M., and McQuaid, C. D. (2009). Variability in the fractionation of stable isotopes during degradation of two intertidal red algae. Estuar. Coast. Shelf Sci. 82, 395–405. doi: 10.1016/j.ecss.2009.02.001
Hoeinghaus, D. J., Winemiller, K. O., and Agostinho, A. A. (2007). Landscape-scale hydrologic characteristics differentiate patterns of carbon flow in large-river food webs. Ecosystems 10, 1019–1033. doi: 10.1007/s10021-007-9075-2
Howard-Williams, C., and Junk, W. J. (1976). The decomposition of aquatic macrophytes in the floating meadows of a Central Amazonian Várzea lake. Biogeographica 7, 115–123.
Jackson, A. L., Inger, R., Parnell, A. C., and Bearhop, S. (2011). Comparing isotopic niche widths among and within communities: SIBER–Stable Isotope Bayesian Ellipses in R. J. Anim. Ecol. 80, 595–602. doi: 10.1111/j.1365-2656.2011.01806.x
Jackson, D. A., Peres-Neto, P. R., and Olden, J. D. (2001). What controls who is where in freshwater fish communities the roles of biotic, abiotic, and spatial factors. Can. J. Fish. Aquat. Sci. 58, 157–170. doi: 10.1139/cjfas-58-1-157
Jackson, M. C., Donohue, I., Jackson, A. L., Britton, J. R., Harper, D. M., and Grey, J. (2012). Population-level metrics of trophic structure based on stable isotopes and their application to invasion ecology. PLoS ONE 7:e31757. doi: 10.1371/journal.pone.0031757
Jackson, P. B. N. (1961). The impact of predation, especially by the tiger-fish (Hydrocyon vittatus Cast.). on African freshwater fishes. Proc. Zool. Soc. Lond. 136, 603–622. doi: 10.1111/j.1469-7998.1961.tb05895.x
Kalk, M., McClachlan, A.J., and Howard-Williams, C., (eds.). (1979). Lake Chilwa: Studies of Change in a Tropical Ecosystem. The Hague: Dr. W. Junk Publishers.
Kolding, J., and van Zwieten, P. A. M. (2012). Relative lake level fluctuations and their influence on productivity and resilience in tropical lakes and reservoirs. Fish. Res. 115–116, 99–109. doi: 10.1016/j.fishres.2011.11.008
Kolding, J., van Zwieten, P. A. M., Marttin, F., and Poulain, F. (2016). Fisheries in the Drylans of sub-Saharan Africa–“Fish Come With the Rains”. Building Resilience for Fisheries-Dependent Livelihoods to Enhance Food Securty and Nutrition in the Drylands. Technical Report, FAO Fisheries and Aquaculture Circular, Rome.
Layman, C. A., Arrington, D. A., Montaña, C. G., and Post, D. M. (2007). Can stable isotope ratios provide for community-wide measures of trophic structure? Ecology 88, 42–48. doi: 10.1890/0012-9658(2007)88[42:CSIRPF]2.0.CO;2
Lewis, W. M. J., Hamilton, S. K., Rodríguez, M. A., Saunders, J. F., and Lasi, M. A. (2001). Foodweb analysis of the Orinoco Floodplain based on production estimates and stable isotope data. J. North Am. Benthol. Soc. 20, 241. doi: 10.2307/1468319
Macko, S., and Estep, M. (1984). Microbial alteration of stable nitrogen and carbon isotopic compositions of organic matter. Org. Geochem. 6, 787–790. doi: 10.1016/0146-6380(84)90100-1
Mendelsohn, J., and Roberts, C. (1997). An Environmental Profile and Atlas of Caprivi. Windhoek: Ministry of Environment and Tourism.
Merron, G. S. (1993). Pack-hunting in two species of catfish, Clarias gariepinus and C. ngamensis, in the Okavango Delta, Botswana. J. Fish Biol. 43, 575–584. doi: 10.1006/jfbi.1993.1160
Merron, G. S., and Mann, B. Q. (1995). The reproductive and feeding biology of Schilbe intermedius Riippell in the Okavango Delta, Botswana. Hydrobiologia 308, 121–129. doi: 10.1007/BF00007397
Morana, C., Darchambeau, F., Roland, F. A. E., Borges, A. V., Muvundja, F., Kelemen, Z., et al. (2015). Biogeochemistry of a large and deep tropical lake (Lake Kivu, East Africa: insights from a stable isotope study covering an annual cycle. Biogeosciences 12, 4953–4963. doi: 10.5194/bg-12-4953-2015
Neill, C., and Cornwell, J. C. (1992). Stable carbon, nitrogen, and sulfur isotopes in a prairie marsh food web. Wetlands 12, 217–224. doi: 10.1007/BF03160612
Olsson, K., Stenroth, P., Nystr€om, P., and Granéli, W. (2009). Invasions and niche width: does niche width of an introduced crayfish differ from a native crayfish? Freshw. Biol. 54, 1731–1740. doi: 10.1111/j.1365-2427.2009.02221.x
O'Reilly, C. M., Hecky, R. E., Cohen, A. S., and Plisnier, P.-D. (2002). Interpreting stable isotopes in food webs: recognizing the role of time averaging at different trophic levels. Limnol. Oceanogr. 47, 306–309. doi: 10.4319/lo.2002.47.1.0306
Parnell, A. C., Inger, R., Bearhop, S., and Jackson, A. L. (2010). Source partitioning using stable isotopes: coping with too much variation. PLoS ONE 5:e9672. doi: 10.1371/journal.pone.0009672
Peel, R. A. (2016). Colonisation and succession of fishes in lake Liambezi, a shallow ephemeral floodplain lake in southern Africa. Retrieved from the Rhodes University Thesis Repository. 196.
Peel, R. A., Hill, J. M., Taylor, G. C., Tweddle, D., and Weyl, O. L. F. (2019). Species succession and the development of a lacustrine fish community in an ephemeral lake. J. Fish Biol. doi: 10.1111/jfb.14081
Peel, R. A., Tweddle, D., Simasiku, E. K., Martin, G. D., Lubanda, J., Hay, C. J., et al. (2015). Ecology, fish and fishery of Lake Liambezi, a recently refilled floodplain lake in the Zambezi Region, Namibia. Afr. J. Aquat. Sci. 40, 417–424. doi: 10.2989/16085914.2015.1105779
Pettit, N. E., Warfe, D. M., Close, P. G., Pusey, B. J., Dobbs, R., Davies, C., et al. (2016). Carbon sources for aquatic food webs of riverine and lacustrine tropical waterholes with variable groundwater influence. Mar. Freshw. Res. 68, 442–45. doi: 10.1071/MF15365
Phillips, D. L., Inger, R., Bearhop, S., Jackson, A. L., Moore, J. W., Parnell, A. C., et al. (2014). Best practices for use of stable isotope mixing models in food-web studies. Can. J. Zool. 92, 823–835. doi: 10.1139/cjz-2014-0127
Pinkas, L., Oliphant, M. S., and Iverson, I. L. K. (1971). Food habits of albacore, bluefin tuna and bonita in Calaforninan waters. Calif. Fish Game 152, 1–105.
R Core Team (2013). R: A Language and Environment for Statistical Computing. Vienna: R Foundation for Statistical Computing. Available online at: http://www.R-project.org/
Roach, K. A., and Winemiller, K. O. (2015). Hydrologic regime and turbidity influence entrance of terrestrial material into river food webs. Can. J. Fish. Aquat. Sci. 72, 1099–1112. doi: 10.1139/cjfas-2014-0459
Seaman, M. T., Scott, W. E., Walmsley, R. D., van der Waal, B. C. W., and Toerien, D. F. (1978). A limnological investigation of Lake Liambezi, Caprivi. J. Limnol. Soc. Southern Africa 4, 129–144. doi: 10.1080/03779688.1978.9633164
Simasiku, E. K., Weyl, O. L. F., Kopij, G., and Mafwila, S. K. (2017). Assessment of Lake Liambezi gillnet fishery in the Zambezi region, Namibia. Int. J. Fish. Aquat. Stud. 5, 490–495. Available online at: http://www.fisheriesjournal.com/archives/2017/vol5issue1/PartG/4-6-53-460.pdf
Skelton, P. H. (2001). A Complete Guide to the Freshwater fishes of Southern Africa. Cape Town: Struik Publisher.
Skelton, P. H. (2016). Name changes and additions to the southern African freshwater fish fauna. Afr. J. Aquat. Sci. 1–7. doi: 10.2989/16085914.2016.1186004
Soares, M. G. M., Almeida, R. G., and Junk, W. J. (1986). The trophic status of the fish fauna in Lago Camaleão, a macrophyte dominated floodplain lake in the middle Amazon. Amazoniana 9, 511–526.
Stock, B. C., Jackson, A. L., Ward, E. J., Parnell, A. C., Phillips, D. L., and Semmens, B. X. (2018). Analyzing mixing systems using a new generation of Bayesian tracer mixing models. PeerJ. 6:e5096. doi: 10.7717/peerj.5096
Stock, B. C., and Semmens, B. S. (2016). MixSIAR GUI User Manual. Version 3.1. https://github.com/brianstock/MixSIAR
Sweeting, C. J., Barry, J., Barnes, C., Polunin, N. V. C., and Jennings, S. (2007b). Effects of body size and environment on diet-tissue δ15N fractionation in fishes. J. Exp. Mar. Biol. Ecol. 340, 1–10. doi: 10.1016/j.jembe.2006.07.023
Sweeting, C. J., Barry, J. T., Polunin, N. V. C., and Jennings, S. (2007a). Effects of body size and environment on diet-tissue δ13C fractionation in fishes. J. Exp. Mar. Biol. Ecol. 352, 165–176. doi: 10.1016/j.jembe.2007.07.007
Talling, J. F. (1992). Environmental regulation in African shallow lakes and wetlands. Rev. Hydrobiol. Trop. 25, 87–144.
Talling, J. F. (2001). Environmental controls on the functioning of shallow tropical lakes. Hydrobiologia 458, 1–8. doi: 10.1023/A:1013121522321
Taylor, G. C., Hill, J. M., Jackson, M. C., Peel, R. A., and Weyl, O. L. F. (2017a). Estimating δ15N fractionation and adjusting the lipid correction equation using Southern African freshwater fishes. PLoS ONE 12:e0178047. doi: 10.1371/journal.pone.0178047
Taylor, G. C., Weyl, O. L. F., Hill, J. M., Peel, R. A., and Hay, C. J. (2017b). Comparing the fish assemblages and food-web structures of large floodplain rivers. Freshw. Biol. 62, 1891–1907. doi: 10.1111/fwb.13032
Thorp, J. H., and Delong, M. D. (1994). The riverine productivity model: an heuristic view of carbon sources and organic processing in large river ecosystems. Oikos 70, 305–308. doi: 10.2307/3545642
Thorp, J. H., Delong, M. D., Greenwood, K. S., and Casper, A. F. (1998). Isotopic analysis of three food web theories in constricted and floodplain regions of a large river. Oecologia 117, 551–563. doi: 10.1007/s004420050692
van der Waal, B. C. W. (1985). Aspects of the biology of larger fish species of Lake Liambezi, Caprivi, South West Africa. Madoqua 14, 101–144.
Vander Zanden, M. J., and Vadeboncoeur, Y. (2002). Fishes as integrators of benthic and pelagic food webs in lakes. Ecology 83, 2152–2161. doi: 10.2307/3072047
Wantzen, K. M., Junk, W. J., and Rothhaupt, K. (2008). An extension of the floodpulse concept (FPC). for lakes. Hydrobiologia 613, 151–170. doi: 10.1007/s10750-008-9480-3
Ward, J. V., Tockner, K., Arscott, D. B., and Claret, C. (2002). Riverine landscape diversity. Freshw. Biol. 47, 517–539. doi: 10.1046/j.1365-2427.2002.00893.x
Ward, J. V., Tockner, K., and Schiemer, F. (1999). Biodiversity of floodplain river ecosystems: ecotones and connectivity. Regul. Rivers Res. Manage. 15, 125–139. doi: 10.1002/(SICI)1099-1646(199901/06)15:1/3<125::AID-RRR523>3.0.CO;2-E
Welcomme, R. L., Winemiller, K. O., and Cowx, I. G. (2006). Fish environmental guilds as a tool for assessment of ecological condition of rivers. River Res. Appl. 22, 377–396. doi: 10.1002/rra.914
Weyl, O. L. F., Daga, V. S., Ellender, B. R., and Vitule, J. R. (2016). A review of Clarias gariepinus invasions in Brazil and South Africa. J. Fish Biol. 89, 386–402. doi: 10.1111/jfb.12958
Willoughby, N. G., and Tweddle, D. (1978). The ecology of the catfish Clarias gariepinus and Clarias ngamensis in the Shire Valley, Malawi. J. Zool. Soc. Lond. 184, 507–534. doi: 10.1111/j.1469-7998.1978.tb03936.x
Winemiller, K. O. (1991). Comparative ecology of Serranochromis species (Teleostei: Cichlidae). in the Upper Zambezi River floodplain. J. Fish Biol. 39, 617–639. doi: 10.1111/j.1095-8649.1991.tb04393.x
Winemiller, K. O. (1996). “Factors driving temporal and spatial variation in aquatic floodplain food webs,” in Food Webs: Integration of Patterns and Dynamics, eds G.A. Polis and K.O. Winemiller (New York, NY: Chapman and Hall), 298–312. doi: 10.1007/978-1-4615-7007-3_29
Winemiller, K. O. (2004). “Floodplain river food webs: generalizations and implications for fisheries management,” in Proceedings of the Second International Symposium on the Management of Large Rivers for Fisheries Vol. 2, eds R.L. Welcomme and T. Petr (Bangkok: FAO Regional Office for Asia and the Pacific), 285–309.
Winemiller, K. O., and Adite, A. (1997). Convergent evolution of weakly electric fishes from floodplain habitats in Africa and South America. Environ. Biol. Fishes 49, 175–186. doi: 10.1023/A:1007376826609
Winemiller, K. O., and Kelso-Winemiller, L. C. (1996). Comparative ecology of catfishes of the Upper Zambezi River floodplain. J. Fish Biol. 49, 1043–1061. doi: 10.1111/j.1095-8649.1996.tb01777.x
Winemiller, K. O., and Kelso-Winemiller, L. C. (2003). Food habits of tilapiine cichlids of the Upper Zambezi River and floodplain during the descending phase of the hydrologic cycle. J. Fish Biol. 63, 120–128. doi: 10.1046/j.1095-8649.2003.00134.x
Yodzis, P., and Winemiller, K. O. (1999). In search of operational trophospecies in a tropical aquatic food web. Oikos 87, 327–340. doi: 10.2307/3546748
Zengeya, T. A., and Marshall, B. E. (2007). Trophic interrelationships amongst cichlid fishes in a tropical African reservoir (Lake Chivero, Zimbabwe). Hydrobiologia 592, 175–182. doi: 10.1007/s10750-007-0790-7
Keywords: floodplains, stable isotopes, food webs, lakes, fish communities
Citation: Peel RA, Hill JM, Taylor GC and Weyl OLF (2019) Food Web Structure and Trophic Dynamics of a Fish Community in an Ephemeral Floodplain Lake. Front. Environ. Sci. 7:192. doi: 10.3389/fenvs.2019.00192
Received: 04 September 2019; Accepted: 21 November 2019;
Published: 05 December 2019.
Edited by:
John Pascal Simaika, IHE Delft Institute for Water Education, NetherlandsReviewed by:
Martin Paar, University of Greifswald, GermanyGuillaume Grosbois, Université du Québec à Chicoutimi, Canada
Copyright © 2019 Peel, Hill, Taylor and Weyl. This is an open-access article distributed under the terms of the Creative Commons Attribution License (CC BY). The use, distribution or reproduction in other forums is permitted, provided the original author(s) and the copyright owner(s) are credited and that the original publication in this journal is cited, in accordance with accepted academic practice. No use, distribution or reproduction is permitted which does not comply with these terms.
*Correspondence: Jaclyn M. Hill, amFjbHluLmhpbGwmI3gwMDA0MDtkZm8tbXBvLmdjLmNh
†ORCID: Jaclyn M. Hill orcid.org/0000-0003-0703-3772
Olaf L. F. Weyl orcid.org/0000-0002-8935-3296