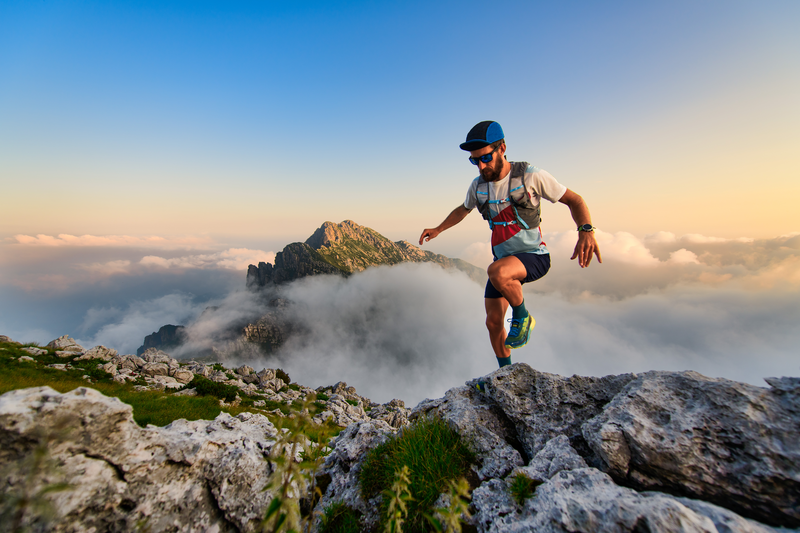
94% of researchers rate our articles as excellent or good
Learn more about the work of our research integrity team to safeguard the quality of each article we publish.
Find out more
REVIEW article
Front. Endocrinol. , 09 December 2024
Sec. Pediatric Endocrinology
Volume 15 - 2024 | https://doi.org/10.3389/fendo.2024.1449033
Central precocious puberty (CPP) is an endocrine disease in children, characterized by rapid genital development and secondary sexual characteristics before the age of eight in girls and nine in boys. The premature activation of the hypothalamic-pituitary-gonadal axis (HPGA) limits the height of patients in adulthood and is associated with a higher risk of breast cancer. How to prevent and improve the prognosis of CPP is an important problem. Vitamin D receptor (VDR) is widely expressed in the reproductive system, participates in the synthesis and function of regulatory sex hormones, and affects the development and function of gonads. In addition, gut microbiota plays an important role in human health by mainly regulating metabolites, energy homeostasis, and hormone regulation. This review aims to clarify the effect of vitamin D deficiency on the occurrence and development of CPP and explore the role of gut microbiota in it. Although evidence on the interaction between vitamin D deficiency, gut microbiota, and sexual development remains limited, vitamin D supplementation and gut microbiota interventions offer a promising, non-invasive strategy for managing CPP.
Precocious puberty (PP) is characterized by the onset of secondary sexual characteristics before age eight in girls and nine in boys, with central precocious puberty (CPP) being the most common form. CPP results from early activation of the hypothalamic-pituitary-gonadal axis (HPGA), and it affects girls 15-20 times more often than boys (1). This condition can impact adult height and is associated with an increased risk of type 2 diabetes, cardiovascular disease, breast cancer, and other complications in adulthood (2). While some causes of CPP are known—including genetic predispositions like KISS1 and MKRN3 gene variations, as well as endocrine-disrupting chemicals—many cases, particularly in girls (about 90%) and boys (up to 60%), remain idiopathic (3). There is also a global trend towards earlier puberty onset, necessitating better diagnostic and treatment approaches (4–7). While GnRH analogs remain the cornerstone of CPP treatment, novel sustained-release formulations and personalized therapeutic approaches are actively being developed.
Vitamin D plays a crucial role in children’s growth and development, maintaining calcium homeostasis, promoting bone growth, and regulating neuroendocrine and reproductive functions. The vitamin D receptor (VDR) is present throughout the hypothalamic-pituitary-gonadal axis (8–10). Adequate vitamin D levels are essential for normal pubertal progression and reproductive health, as demonstrated in mice (11). It significantly influences sex hormone secretion, gonadal development, and reproductive organ function. In humans, studies have linked vitamin D levels to the timing of menarche and overall reproductive health (12–15). Vitamin D deficiency (serum 25-hydroxyvitamin D below 50 nmol/L) is associated with various health issues, including immune dysfunction, obesity, metabolic syndrome, infections, cancer, and cardiovascular diseases (16). Recent meta-analyses indicate an inverse relationship between vitamin D levels and precocious puberty. However, findings on vitamin D deficiency prevalence in PP patients are inconsistent, possibly due to a threshold effect of vitamin D status (17–19).
The gut microbiota refers to the diverse community of microorganisms, including bacteria, archaea, viruses, and fungi, that inhabit the gastrointestinal tract. It plays a crucial role in metabolic, physiological, and immune functions and stabilizes to an adult-like composition within 1-3 years after birth (20, 21). Significant differences exist between the microbiota of children and adults, with microbial community structure, diversity, and functional potential varying by age and gender, especially during puberty (22, 23). The gut microbiota began to show gender differences during puberty and interacted with sex hormones, indicating that there was a relationship between gut microbiota and sexual maturity (24, 25). Additionally, vitamin D is vital for gastrointestinal health, influencing mucosal barriers, ILC3, and T cells, thereby affecting the microbiota (26). Both clinical and animal studies show that vitamin D can modulate the immune system through changes in gut microbiota composition and antimicrobial peptides (AMPs) regulation (27, 28). By promoting short-chain fatty acid (SCFA)-producing bacteria, vitamin D helps reduce inflammation, suggesting that vitamin D supplementation could restore gut homeostasis and offer therapeutic benefits for CPP (26).
Given the diagnostic and therapeutic challenges of CPP, there is an urgent need to explore its etiology further to possibly develop also non-invasive approaches. Recent studies highlight a potential link between PP, vitamin D, gut microbiota, and their metabolites, though the mechanisms remain unclear. This review aims to summarize the relationships between vitamin D, CPP, and gut microbiota, providing insights for future interventions through vitamin D supplementation and microbiota modulation.
A systematic search of the PubMed database was conducted up to May 2024 to evaluate the literature on the interplay between CPP, vitamin D, and gut microbiota. The review includes original articles, meta-analyses, animal studies, and clinical studies. Search terms used were “central precocious puberty”, “pubertal disorders”, “age at menarche”, “timing of puberty”, “early menarche”, “puberty time”, “vitamin D”, “vitamin D3”, “vitamin D deficiency”, “microbiota”, “gut microbiota”, “microbiome”, “dysbiosis”, “sex hormones”, “short chain fatty acid”, and “bile acids”.
Vitamin D’s role in CPP has garnered significant attention. It interacts with transcription factors, regulating vitamin D-sensitive genes critical for bone and mineral metabolism and other biological functions (29, 30). The vitamin D receptor (VDR) is widely distributed in somatic cells and organs, including bone, parathyroid glands, immune system components, and endocrine structures like the pancreas, hypothalamus, pituitary gland, and adrenal cortex. It is also present in reproductive tissues such as the testes, ovaries, and uterus (31, 32). Studies indicate that vitamin D is essential for neuroendocrine regulation, reproductive development, and immune function (33).
Studies in mammals have demonstrated that the active form of vitamin D, 1,25(OH)2D3, also known as calcitriol, stimulates the production of estradiol and estrone, while knockdown of the VDR significantly reduces testosterone synthesis and secretion in Leydig cells. This regulation may involve the expression of 3β-hydroxysteroid dehydrogenase (3β-HSD) and StAR (34–36). Vitamin D also promotes mitochondrial homeostasis, reduces oxidative stress and tissue damage, and regulates cellular health. Vitamin D deficiency, conversely, decreases mitochondrial activity and increases oxidative stress and inflammation (37). 1,25(OH)2D3 has immunomodulatory properties on T cells and can reduce pro-inflammatory cytokines such as IL-17, INF-γ, and TNF-α, exerting anti-inflammatory effects (38). Parathyroid hormone (PTH) indirectly affects vitamin D synthesis by regulating calcium levels, while vitamin D influences the secretion of pituitary gonadotropin through interactions with PTH and its receptors. Genetic factors are believed to account for 50-80% of the variation in puberty timing in the general population (39). Genome-wide association studies have identified numerous genetic loci influencing puberty timing across various ethnic groups, including loci associated with PP (40). Single nucleotide polymorphisms (SNP) are small modifications in the nucleotide sequence between individuals, and some SNPs may make subjects more susceptible to certain diseases. Recent genetic studies suggest a potential etiological role for SNPs in/near a custom list of genes related to nuclear hormone receptors including VDR during puberty (41). A case-control study by Li et al. indicated that VDR polymorphism may protect against CPP in Chinese girls by affecting the peak FSH level in the gonadotropin-releasing hormone (GnRH) stimulation test (42).
Preclinical studies show VDR expression in the hypothalamus, suggesting vitamin D may regulate reproductive system development by affecting GnRH neuron function. Vitamin D is involved in NMDA-mediated inhibition of GnRH neuronal activity, potentially delaying the onset of puberty (3, 43). Although vitamin D’s neuroprotective effects against reactive oxygen species (ROS) and inflammation are known, its specific mechanisms on HPGA remain unclear (44). Notably, vitamin D metabolic enzymes and VDR are expressed in white adipose tissue, and low-dose 1,25(OH)2-D3 inhibits apoptosis of differentiated 3T3-L1 adipocytes by regulating the expression of uncoupling protein 2 (45). Another study reports that 1,25(OH)2D3 mainly regulates the late stages of adipogenesis (46). This reveals a potential mechanism between obesity and CPP. Studies in female mice show that peripubertal vitamin D deficiency delays vaginal opening and estrus, while deficiency before weaning does not affect puberty (11, 47). VDR knockout mice exhibit gonadal dysfunction, including reduced sperm count and motility, and abnormalities in reproductive organs (48). Interaction among ANXA1, ANXA5, and VDR may influence gonadotropin secretion regulation in female rats (49).
Consistent with the “fetal programming” hypothesis, several large-sample clinical birth cohort studies have found that the season of birth and first trimester with seasonal variations in endogenous vitamin D3 synthesis has been associated with age at menarche (50, 51). In addition, a single-center, matched cohort study found a low vitamin D status during pregnancy determines the course of mini-puberty in boys (52). Other evidence also suggests that 25(OH)D modestly affects total testosterone and inhibin B levels in girls during mini-puberty, indicating that 25(OH)D may influence gonadal function in early life (53). A case-control study found that vitamin D deficiency was associated with early menarche and was an independent risk factor for idiopathic central precocious puberty (ICPP) in girls (12, 54). Female gender and puberty were negatively associated with 25(OH)D (55). However, randomized clinical trials have yielded different results from animal studies, showing no significant effect of vitamin D treatment on testosterone or other hormone levels (56–58). This discrepancy may arise from not accounting for factors such as vitamin D-binding proteins and detection methods. Vitamin D deficiency might indirectly affect hormone status by regulating the bioavailable portion of testosterone, which requires further investigation considering age and the degree of vitamin D deficiency. In summary, the exact role and effect of vitamin D in the pathogenesis of CPP need further study to determine its specific mechanisms. The possible molecular mechanisms connecting vitamin D to puberty and the findings of preclinical and clinical studies are summarized in Figure 1.
It is postulated that a complex interplay of genetic, nutritional, and environmental factors may precipitate endocrine and physiological changes leading to puberty (3). Apart from potential adjustments in overall dietary patterns and lifestyle, certain nutrients, including vitamin D, may hold promise in influencing sexual maturation. However, there is no definitive consensus regarding the correlation between vitamin D and the onset and progression of CPP. Population-based epidemiological and clinical investigations suggest that serum vitamin D levels are lower in individuals with CPP compared to subjects with normal onset of puberty, posing a risk factor for precocious puberty (17, 18, 54, 59–64). Moreover, the proportion of vitamin D deficiency in the CPP population was also higher than in the control group, as shown in Supplementary Table 1. Meanwhile, a case-control study identified a correlation between vitamin D levels and uterine volume specifically among girls with ICPP, a relationship not observed in their peers. Girls with ICPP exhibited lower vitamin D levels alongside larger uterine volumes (60). The latest meta-analysis corroborates the notion that severe vitamin D deficiency may elevate the risk of precocious puberty, with patients diagnosed with CPP exhibiting lower levels compared to other types of precocious puberty (65, 66). However, certain clinical studies have failed to establish a significant relationship between vitamin D status and CPP (67, 68). The findings from a cross-sectional study revealed that the vitamin D status among girls aged six to eight with CPP was comparable to that of preadolescent girls. While girls with CPP exhibited significantly elevated levels of parathyroid hormone (67). In the future, further high-quality clinical studies are warranted to validate the relationship between children’s vitamin D levels and CPP. Specifically, large-scale prospective cohort studies or randomized controlled trials with robust methodologies are essential to ensure the reliability of the findings.
The development of children’s gut microbiota undergoes a dynamic process influenced by various factors. Among these, the mode of delivery, breastfeeding, early exposure to antibiotics, and host-related factors exert significant influence on early life (69–71). While traditionally believed to stabilize within the first three years, recent evidence suggests gut microbiota development continues into childhood and early puberty (22, 72, 73). Puberty, influenced by early nutrition and breastfeeding, sees dynamic changes in gut microbiota, with breast milk offering a protective effect against early puberty, whereas formula feeding is linked to premature puberty (74). Additionally, gender differences in gut microbiota emerge during adolescence and persist into adulthood.
Current research indicates no significant difference in gut microbiota diversity between normal adolescents and prepubertal children, but variations in composition and metabolites exist. A Finnish population-based cohort study found that gut microbiota development during puberty is sex-specific and associated with the timing of puberty in girls. During puberty, the relative abundance of Clostridiales and Bifidobacterium increased, especially Ruminococcaceae, while Bacteroidales decreased (75). A case-control study observed enrichment of Ruminococcus bromii, Ruminococcus gnavus, and Clostridium leptum in ICPP girls, with FSH positively correlated with Fusobacterium and LH with Gemmiger (76). Another cross-sectional study showed higher alpha diversity and upregulated Bacteroides and Faecalibacterium in girls with CPP (77). Li et al. also found that CPP girls had significantly different gut microbiota from normal and overweight girls, with elevated levels of Alistipes, Klebsiella, and Sutterella (78). Functional predictions they conducted suggest peripheral precocious puberty as a transitional stage between ICPP and normal children. Gut bacteria alter sex hormone levels by modifying active-to-inactive ratios, using enzymes like β-glucuronidase (GUS), β-glucosidase, and hydroxysteroid dehydrogenases (HSD) for degradation. Furthermore, The Firmicutes to Bacteroidetes ratio decreases with higher serum hormone levels, indicating an interaction between sex hormones and gut microbes (79). Children with CPP have a higher breast cancer risk in adulthood, and gut microbial β-glucuronidase (gmGUS) inhibitors are a new approach to managing estrogen-related diseases like breast cancer (80, 81).
CPP occurs due to premature activation of the HPGA, and gut microbiota metabolites can influence hypothalamic neurotransmitters and gene expression (82). The gut-brain axis provides a two-way communication pathway via neural, hormonal, and immune pathways, influencing human physiology. Gut microbiota functional analysis suggests the neuroactive compound nitric oxide synthesis is linked to CPP progression (83). Notably, SCFAs, lipopolysaccharides (LPS), and secondary bile acids (BA) are identified as key gut microbial metabolites influencing puberty timing. Dietary carbohydrates fermented by gut microbiota produce SCFAs like acetate, butyrate, and propionate. Bacteroidetes mainly produce acetate and propionate, while Firmicutes produce butyrate (84). In vitro studies show butyrate can increase LH and FSH levels, correlating with clinical findings of increased SCFA-producing bacteria in CPP children (85, 86). This increase may promote the expression of leptin and HPG axis-related genes, leading to puberty onset (76, 77, 87). However, excessive SCFAs, particularly butyrate, are associated with promoting intestinal inflammation and insulin resistance, disrupting glucose homeostasis and intestinal mucosal barrier function, which may increase intestinal energy harvesting and promote the development of obesity (88–93). Recent studies indicate that SCFAs modulate GnRH release by the GPR54-PKC-ERK1/2 pathway in the hypothalamus, affecting puberty in female rats on a high-fat diet (HFD). Gut microbiota in precocious puberty rats show dysbiosis and reduced SCFA production. Adding SCFAs to HFD can reverse precocious puberty in rats (94). Butyrate also enhances VDR protein expression while suppressing inflammation (95). The variability between experimental and clinical findings may be due to diet interactions and confounding factors like genetics, environment, and lifestyle. LPS, derived from Gram-negative bacteria, enhances inflammation by inducing macrophage infiltration and proinflammatory cytokines and inhibiting Treg cells. Prevotella triggers TNF-α production, causing secretion of inflammatory cytokines like IL-6, via an LPS-based mechanism (96). The gut microbiome is involved in BA metabolism, regulating secondary BA metabolism, and inhibiting BA synthesis in the liver via farnesoid X receptor (FXR) signaling (97). In addition, BA functions as a signaling molecule, binding to cell receptors. FXR impairs glucose homeostasis (98). The bacterial enzyme bile salt hydrolase (BSH) can modulate FXR signaling by cleaving its antagonist tauro-β-naphthocholic acid (99). Further studies using metagenomics and metabolomics are needed to explore the associations between microbial-derived metabolites (LPS, SCFAs, BAs) and CPP. The mechanisms involving gut microbiota in CPP include differences in early feeding, LPS, SCFAs, energy homeostasis, intestinal barrier maintenance, hormone regulation, and the gut-brain axis, as shown in Figure 2.
Figure 2. Association of enterotypes in gut microbiota with puberty timing and potential mechanism for CPP. The up and down arrows indicate increase and decrease, respectively.
Vitamin D plays a crucial role in regulating the gut microbiome, and VDR is present in intestinal epithelial cells, immune cells, and gut microbiota. Vitamin D modulates gut microbiome composition and diversity by regulating antimicrobial peptides and immune responses (100). Vitamin D deficiency correlates with gut microbiota imbalance, compromised intestinal defense mechanisms, and increased intestinal permeability (101–103). A genome-wide association study involving 2029 individuals identified two VDR polymorphisms as significant contributors to gut microbiota alterations (104). Vitamin D has shown protective effects on gut microbiota in animal models of inflammatory bowel disease. It induces macrophages to produce antimicrobial peptides, enhances epithelial barrier integrity, regulates the expression of various connexins, and defensins, modulates inflammatory responses, and influences gut microbiota composition (105). Consistent evidence from mouse and human studies indicates an association between vitamin D and gut microbiota beta diversity, but not alpha diversity (106). Clinical studies show that high-dose vitamin D supplementation in adolescent girls improves vitamin D status, increases Firmicutes and Bifidobacterium levels, and decreases Bacteroidetes abundance (27). Vitamin D-deficient children exhibit higher levels of Bacteroides massillensis and Prevotella species (107). Bacteroides and Prevotella influence inflammation by releasing lipopolysaccharides, which activate macrophages. A cross-sectional study found Prevotella more prominent in individuals with high vitamin D intake and lower LPS concentrations in those with better vitamin D status (108). Chronic inflammation from vitamin D deficiency may be linked to CPP through gut microbiota changes. Obesity also impacts gut microbiota. High BMI in children is associated with decreased Bacteroidetes and increased Firmicutes (109). Obese adolescents have a gut microbiota predominantly composed of Firmicutes (94.6%), while Bacteroidetes account for 3.2% (110). However, a cohort study of 295 Dutch children found no correlation between the Firmicutes to Bacteroidetes ratio and BMI (111). Another study on HFD effects on gut microbiota and sexual development in mice found inconsistencies between obesity and precocious puberty-related microbiota changes, highlighting the need to control for obesity when analyzing the gut microbiota-CPP relationship (112).
In conclusion, the complex relationship between gut microbiota, vitamin D, and precocious puberty requires further investigation. Key mechanisms likely involve sex hormones, inflammation, immune regulation, and intestinal barrier maintenance. Understanding these interactions is crucial for elucidating CPP pathogenesis and identifying potential treatments. The relationship between vitamin D, gut microbiota, and CPP investigated in this review is shown in Figure 3.
Recent studies highlight the crucial interaction between vitamin D and gut microbiota in human health, particularly regarding metabolism and immune function. PP stands as a significant health concern in children, prompting increased scrutiny regarding the relationship between CPP and vitamin D deficiency and gut microbiota imbalance.
Previous studies have shown that vitamin D improves insulin sensitivity in adolescents with obesity (113). A recent meta-analysis suggests that combining vitamin D with drug therapy and a short-term/high-dose supplementation strategy is beneficial for sexual hormone and body indices in patients with precocious puberty (65). This observation might explain the persistently high prevalence of vitamin D deficiency despite recommended daily intakes (114, 115). Long-term, low-dose vitamin D does not significantly increase 25(OH)D levels in deficient patients, while high doses rapidly normalize these levels without adverse effects. As shown in Supplementary Table 2, clinical trials support the need for high-dose vitamin D supplementation in children with vitamin D deficiency to improve levels and address associated risks (116–130).
Despite these findings, clear and systematic vitamin D supplementation guidelines for clinical practice are lacking. More research is needed to establish guidelines for different pediatric populations. A recent study suggests that lifestyle interventions, such as the Mediterranean diet, may enhance vitamin D levels more effectively than supplementation alone (131). Current research suggests that higher doses of 1,25(OH)2D3 are needed to treat vitamin D deficiency in obese individuals (115). This may be because obesity can reduce circulating 25(OH)D by trapping this lipophilic vitamin in adipose tissue. Addressing weight management or providing vitamin D recommendations based on BMI may be necessary.
Animal studies indicate that insulin resistance linked to gut microbiota induced by HFD can promote early puberty, while microbial remodeling can prevent it (132). Clinical studies have identified Streptococcus as a potential marker for CPP therapy (83). Probiotics have shown promise in upregulating VDR expression, suggesting a synergistic effect with vitamin D. Targeted delivery of vitamins to the colon to modulate gut microbiota is also being explored (133). According to clinical studies, high-protein, complex carb diets may help protect against CPP in girls (77). Probiotic and vitamin D supplementation holds considerable promise for treating precocious puberty, but larger studies are needed to determine optimal dosages and effects. While dietary supplements, including bioactive molecules, show potential, they should never replace dietary or lifestyle modifications. Studies have shown that in addition to vitamin D, other substances like the natural sweetener glycyrrhizin may also have therapeutic potential in CPP, supporting a more comprehensive approach to management in the future (134).
This review discussed the extensive evidence demonstrating the importance of the relationship between vitamin D and the gut microbiota in CPP. Vitamin D and the gut microbiota profoundly influence the onset and progression of puberty in many different ways. For example, alterations in vitamin D/VDR signaling have been associated with microbiome dysbiosis, which in turn has been associated with CPP. On the other hand, vitamin D supplementation can also improve microbiome composition in cases of deficiency. While promising, further research is needed to fully understand the potential role of vitamin D and probiotics in modulating the risk of CPP. Correcting vitamin D deficiency and microbiota dysbiosis may offer complementary approaches to standard CPP treatment in the future.
Key questions remain: (1) What novel signal transduction pathways does vitamin D use to regulate gut microbiota and affect puberty, and what are the molecular mechanisms? (2) What are the beneficial impacts of vitamin D on CPP? (3) Why does vitamin D have varying effects on puberty timing in clinical trials versus animal studies? (4) What are the optimal dosage and concentration of vitamin D for CPP patients, and how do they compare with other treatments? More extensive clinical trials are needed to explore these questions and understand the interplay between vitamin D, gut microbiota, and CPP. Ensuring adequate vitamin D levels in CPP children, through diet or supplementation, may be key to maintaining healthy gut microbiota and supporting natural puberty onset.
DG: Writing – original draft, Writing – review & editing, Conceptualization. XN: Investigation, Validation, Visualization, Writing – review & editing. TB: Investigation, Validation, Visualization, Writing – review & editing. LT: Investigation, Validation, Visualization, Writing – review & editing. YZ: Data curation, Methodology, Writing – review & editing. ZG: Data curation, Methodology, Writing – review & editing. XL: Project administration, Supervision, Writing – review & editing.
The author(s) declare that no financial support was received for the research, authorship, and/or publication of this article.
The authors declare that the research was conducted in the absence of any commercial or financial relationships that could be construed as a potential conflict of interest.
All claims expressed in this article are solely those of the authors and do not necessarily represent those of their affiliated organizations, or those of the publisher, the editors and the reviewers. Any product that may be evaluated in this article, or claim that may be made by its manufacturer, is not guaranteed or endorsed by the publisher.
The Supplementary Material for this article can be found online at: https://www.frontiersin.org/articles/10.3389/fendo.2024.1449033/full#supplementary-material
BA, bile acids; BSH, bile salt hydrolase; CPP, central precocious puberty; FXR, farnesoid X receptor; GnRH, gonadotropin-releasing hormone; GUS, β-glucuronidase; HFD, high-fat diet; HPGA, hypothalamic-pituitary-gonadal axis; HSD, hydroxysteroid dehydrogenases; ICPP, idiopathic central precocious puberty; LPS, lipopolysaccharides; PP, precocious puberty; SCFA, short-chain fatty acid; SNP, single nucleotide polymorphisms; VDR, vitamin D receptor.
1. Cheuiche AV, Da Silveira LG, De Paula LCP, Lucena IRS, Silveiro SP. Diagnosis and management of precocious sexual maturation: an updated review. Eur J Pediatr. (2021) 180:3073–87. doi: 10.1007/s00431-021-04022-1
2. Day FR, Elks CE, Murray A, Ong KK, Perry JRB. Puberty timing associated with diabetes, cardiovascular disease and also diverse health outcomes in men and women: the UK Biobank study. Sci Rep. (2015) 5:11208. doi: 10.1038/srep11208
3. Latronico AC, Brito VN, Carel J-C. Causes, diagnosis, and treatment of central precocious puberty. Lancet Diabetes Endocrinol. (2016) 4:265–74. doi: 10.1016/S2213-8587(15)00380-0
4. Bräuner EV, Busch AS, Eckert-Lind C, Koch T, Hickey M, Juul A. Trends in the incidence of central precocious puberty and normal variant puberty among children in Denmark, 1998 to 2017. JAMA Netw Open. (2020) 3:e2015665. doi: 10.1001/jamanetworkopen.2020.15665
5. Kang S, Park MJ, Kim JM, Yuk J-S, Kim S-H. Ongoing increasing trends in central precocious puberty incidence among Korean boys and girls from 2008 to 2020. PLoS One. (2023) 18:e0283510. doi: 10.1371/journal.pone.0283510
6. Goggi G, Moro M, Chilà A, Fatti L, Cangiano B, Federici S, et al. COVID-19 lockdown and the rate of central precocious puberty. J Endocrinol Invest. (2023) 47:315–23. doi: 10.1007/s40618-023-02146-9
7. Zevin EL, Eugster EA. Central precocious puberty: a review of diagnosis, treatment, and outcomes. Lancet Child Adolesc Health. (2023) 7:886–96. doi: 10.1016/S2352-4642(23)00237-7
8. Pérez-Fernandez R, Alonso M, Segura C, Muñoz I, Garcia-Caballero T, Diéguez C. Vitamin D receptor gene expression in human pituitary gland. Life Sci. (1996) 60:35–42. doi: 10.1016/S0024-3205(96)00586-3
9. Shahbazi M, Jeddi-Tehrani M, Zareie M, Salek-Moghaddam A, Akhondi MM, Bahmanpoor M, et al. Expression profiling of vitamin D receptor in placenta, decidua and ovary of pregnant mice. Placenta. (2011) 32:657–64. doi: 10.1016/j.placenta.2011.06.013
10. Veenstra TD, Prüfer K, Koenigsberger C, Brimijoin SW, Grande JP, Kumar R. 1,25-Dihydroxyvitamin D3 receptors in the central nervous system of the rat embryo. Brain Res. (1998) 804:193–205. doi: 10.1016/S0006-8993(98)00565-4
11. Dicken CL, Israel DD, Davis JB, Sun Y, Shu J, Hardin J, et al. Peripubertal vitamin D3 deficiency delays puberty and disrupts the estrous cycle in adult female mice1. Biol Reprod. (2012) 87:1–12. doi: 10.1095/biolreprod.111.096511
12. Villamor E, Marin C, Mora-Plazas M, Baylin A. Vitamin D deficiency and age at menarche: a prospective study. Am J Clin Nutr. (2011) 94:1020–5. doi: 10.3945/ajcn.111.018168
13. Łagowska K. The relationship between vitamin D status and the menstrual cycle in young women: A preliminary study. Nutrients. (2018) 10:1729. doi: 10.3390/nu10111729
14. Jukic A, Steiner AZ, Baird DD. Lower plasma 25-hydroxyvitamin D is associated with irregular menstrual cycles in a cross-sectional study. Reprod Biol Endocrinol. (2015) 13:20. doi: 10.1186/s12958-015-0012-5
15. Chew A, Harris SS. Does vitamin D affect timing of menarche? Nutr Rev. (2013) 71:189–93. doi: 10.1111/nure.12015
16. Hu Y, Chen J, Wang R, Li M, Yun C, Li W, et al. Vitamin D nutritional status and its related factors for Chinese children and adolescents in 2010–2012. Nutrients. (2017) 9:1024. doi: 10.3390/nu9091024
17. Xu L, Li P, Yuan D. Confirming the association between low serum 25OHD levels in girls with central precocious puberty and its severity. BMC Pediatr. (2023) 23:624. doi: 10.1186/s12887-023-04447-7
18. Zhao Y, Long W, Du C, Yang H, Wu S, Ning Q, et al. Prevalence of vitamin D deficiency in girls with idiopathic central precocious puberty. Front Med. (2018) 12:174–81. doi: 10.1007/s11684-017-0544-5
19. Liu S, Zhu X, Wang Y, Yan S, Li D, Cui W. The association between vitamin D levels and precocious puberty: a meta-analysis. J Pediatr Endocrinol Metab. (2020) 33:427–9. doi: 10.1515/jpem-2019-0388
20. Koenig JE, Spor A, Scalfone N, Fricker AD, Stombaugh J, Knight R, et al. Succession of microbial consortia in the developing infant gut microbiome. Proc Natl Acad Sci USA. (2011) 108:4578–85. doi: 10.1073/pnas.1000081107
21. Bergström A, Skov TH, Bahl MI, Roager HM, Christensen LB, Ejlerskov KT, et al. Establishment of intestinal microbiota during early life: a longitudinal, explorative study of a large cohort of Danish infants. Appl Environ Microbiol. (2014) 80:2889–900. doi: 10.1128/AEM.00342-14
22. Hollister EB, Riehle K, Luna RA, Weidler EM, Rubio-Gonzales M, Mistretta T-A, et al. Structure and function of the healthy pre-adolescent pediatric gut microbiome. Microbiome. (2015) 3:36. doi: 10.1186/s40168-015-0101-x
23. Ringel-Kulka T, Cheng J, Ringel Y, Salojärvi J, Carroll I, Palva A, et al. Intestinal microbiota in healthy U.S. Young children and adults—A high throughput microarray analysis. PloS One. (2013) 8:e64315. doi: 10.1371/journal.pone.0064315
24. Yoon K, Kim N. Roles of sex hormones and gender in the gut microbiota. J Neurogastroenterol Motil. (2021) 27:314–25. doi: 10.5056/jnm20208
25. Kim YS, Unno T, Kim B-Y, Park M-S. Sex differences in gut microbiota. World J Mens Health. (2020) 38:48. doi: 10.5534/wjmh.190009
26. Cantorna MT, Snyder L, Arora J. Vitamin A and vitamin D regulate the microbial complexity, barrier function, and the mucosal immune responses to ensure intestinal homeostasis. Crit Rev Biochem Mol Biol. (2019) 54:184–92. doi: 10.1080/10409238.2019.1611734
27. Tabatabaeizadeh S-A, Fazeli M, Meshkat Z, Khodashenas E, Esmaeili H, Mazloum S, et al. The effects of high doses of vitamin D on the composition of the gut microbiome of adolescent girls. Clin Nutr ESPEN. (2020) 35:103–8. doi: 10.1016/j.clnesp.2019.10.020
28. Tabassum A, Ali A, Zahedi FD, Ismail NAS. Immunomodulatory role of vitamin D on gut microbiome in children. Biomedicines. (2023) 11:1441. doi: 10.3390/biomedicines11051441
29. Hossein-nezhad A, Holick MF. Vitamin D for health: A global perspective. Mayo Clinic Proc. (2013) 88:720–55. doi: 10.1016/j.mayocp.2013.05.011
30. Winzenberg T, Jones G. Vitamin D and bone health in childhood and adolescence. Calcif Tissue Int. (2013) 92:140–50. doi: 10.1007/s00223-012-9615-4
31. Wang Y, Zhu J, DeLuca HF. Where is the vitamin D receptor? Arch Biochem Biophysics. (2012) 523:123–33. doi: 10.1016/j.abb.2012.04.001
32. Eyles DW, Smith S, Kinobe R, Hewison M, McGrath JJ. Distribution of the Vitamin D receptor and 1α-hydroxylase in human brain. J Chem Neuroanat. (2005) 29:21–30. doi: 10.1016/j.jchemneu.2004.08.006
33. Norman AW. From vitamin D to hormone D: fundamentals of the vitamin D endocrine system essential for good health. Am J Clin Nutr. (2008) 88:491S–9S. doi: 10.1093/ajcn/88.2.491S
34. Parikh G, Varadinova M, Suwandhi P, Araki T, Rosenwaks Z, Poretsky L, et al. Vitamin D regulates steroidogenesis and insulin-like growth factor binding protein-1 (IGFBP-1) production in human ovarian cells. Horm Metab Res. (2010) 42:754–7. doi: 10.1055/s-0030-1262837
35. Yao X, Zhang G, Guo Y, EI-Samahy M, Wang S, Wan Y, et al. Vitamin D receptor expression and potential role of vitamin D on cell proliferation and steroidogenesis in goat ovarian granulosa cells. Theriogenology. (2017) 102:162–73. doi: 10.1016/j.theriogenology.2017.08.002
36. Wang L, Lu H, Wang S, Liu H, Guo M, Bai H, et al. Vitamin D Receptor affects male mouse fertility via regulation of lipid metabolism and testosterone biosynthesis in testis. Gene. (2022) 834:146589. doi: 10.1016/j.gene.2022.146589
37. Reddy AM, Iqbal M, Chopra H, Urmi S, Junapudi S, Bibi S, et al. Pivotal role of vitamin D in mitochondrial health, cardiac function, and human reproduction. EXCLI J. (2022) 21:967–90. doi: 10.17179/excli2022-4935
38. Peruzzu D, Dupuis ML, Pierdominici M, Fecchi K, Gagliardi MC, Ortona E, et al. Anti-inflammatory effects of 1,25(OH)2D/calcitriol in T cell immunity: does sex make a difference? IJMS. (2022) 23:9164. doi: 10.3390/ijms23169164
39. Gajdos ZKZ, Henderson KD, Hirschhorn JN, Palmert MR. Genetic determinants of pubertal timing in the general population. Mol Cell Endocrinol. (2010) 324:21–9. doi: 10.1016/j.mce.2010.01.038
40. Zhu J, Kusa TO, Chan Y-M. Genetics of pubertal timing. Curr Opin Pediatr. (2018) 30:532–40. doi: 10.1097/MOP.0000000000000642
41. Australian Ovarian Cancer Study, The GENICA Network, kConFab, The LifeLines Cohort Study, The InterAct Consortium, Early Growth Genetics (EGG) Consortium, Perry JRB, Day F, et al. Parent-of-origin-specific allelic associations among 106 genomic loci for age at menarche. Nature. (2014) 514:92–7. doi: 10.1038/nature13545
42. Li Y, Tao N, Chen M, Chu J, Huang X, Kong X. Gene polymorphisms associated with central precocious puberty and hormone levels in Chinese girls. Int J Endocrinol. (2022) 2022:1–9. doi: 10.1155/2022/9450663
43. Bhattarai P, Bhattarai JP, Kim MS, Han SK. Non-genomic action of vitamin D3 on N-methyl-D-aspartate and kainate receptor-mediated actions in juvenile gonadotrophin-releasing hormone neurons. Vitamin D. (2017) 29:1231–1238. doi: 10.1071/RD15357
44. Cui X, Eyles DW. Vitamin D and the central nervous system: causative and preventative mechanisms in brain disorders. Nutrients. (2022) 14:4353. doi: 10.3390/nu14204353
45. Sun X, Zemel MB. Role of uncoupling protein 2 (UCP2) expression and 1α, 25-dihydroxyvitamin D 3 in modulating adipocyte apoptosis. FASEB J. (2004) 18:1430–2. doi: 10.1096/fj.04-1971fje
46. Nimitphong H, Holick MF, Fried SK, Lee M-J. 25-hydroxyvitamin D3 and 1,25-dihydroxyvitamin D3 promote the differentiation of human subcutaneous preadipocytes. PloS One. (2012) 7:e52171. doi: 10.1371/journal.pone.0052171
47. Nicholas C, Davis J, Fisher T, Segal T, Petti M, Sun Y, et al. Maternal vitamin D deficiency programs reproductive dysfunction in female mice offspring through adverse effects on the neuroendocrine axis. Endocrinology. (2016) 157:1535–45. doi: 10.1210/en.2015-1638
48. Kinuta K, Tanaka H, Moriwake T, Aya K, Kato S, Seino Y. Vitamin D is an important factor in estrogen biosynthesis of both female and male gonads. Endocrinology. (2000) 141:1317–1324. doi: 10.1210/endo.141.4.7403
49. Murata T, Chiba S, Kawaminami M. Changes in the expressions of annexin A1, annexin A5, inhibin/activin subunits, and vitamin D receptor mRNAs in pituitary glands of female rats during the estrous cycle: correlation analyses among these factors. J Vet Med Sci. (2022) 84:1065–73. doi: 10.1292/jvms.22-0141
50. Day FR, Forouhi NG, Ong KK, Perry JRB. Season of birth is associated with birth weight, pubertal timing, adult body size and educational attainment: a UK Biobank study. Heliyon. (2015) 1:e00031. doi: 10.1016/j.heliyon.2015.e00031
51. Gaml-Sørensen A, Brix N, Ernst A, Lunddorf LLH, Lindh C, Toft G, et al. The estimated effect of season and vitamin D in the first trimester on pubertal timing in girls and boys: a cohort study and an instrumental variable analysis. Int J Epidemiol. (2023) 52:1328–40. doi: 10.1093/ije/dyad060
52. Kowalcze K, Krysiak R, Obuchowicz A. Minipuberty in sons of women with low vitamin D status during pregnancy. Nutrients. (2023) 15:4729. doi: 10.3390/nu15224729
53. Kılınç S, Atay E, Ceran Ö, Atay Z. Evaluation of vitamin D status and its correlation with gonadal function in children at mini-puberty. Clin Endocrinol. (2019) 90:122–8. doi: 10.1111/cen.13856
54. Gan D-M, Fang J, Zhang P-P, Zhao Y-D, Xu Y-N. Serum 25-hydroxyvitamin D levels and the risk of idiopathic central precocious puberty in girls. Clinics. (2023) 78:100244. doi: 10.1016/j.clinsp.2023.100244
55. Aypak C, Türedi Ö, Yüce A. The association of vitamin D status with cardiometabolic risk factors, obesity and puberty in children. Eur J Pediatr. (2014) 173:367–73. doi: 10.1007/s00431-013-2177-2
56. Wehr E, Pilz S, Boehm BO, März W, Obermayer-Pietsch B. Association of vitamin D status with serum androgen levels in men. Clin Endocrinol. (2010) 73:243–8. doi: 10.1111/j.1365-2265.2009.03777.x
57. Pilz S, Frisch S, Koertke H, Kuhn J, Dreier J, Obermayer-Pietsch B, et al. Effect of vitamin D supplementation on testosterone levels in men. Horm Metab Res. (2011) 43:223–5. doi: 10.1055/s-0030-1269854
58. Yahyavi SK, Boisen IM, Cui Z, Jorsal MJ, Kooij I, Holt R, et al. Calcium and vitamin D homoeostasis in male fertility. Proc Nutr Soc. (2023) 83:95–108. doi: 10.1017/S002966512300486X
59. Lee HS, Kim YJ, Shim YS, Jeong HR, Kwon E, Hwang JS. Associations between serum vitamin D levels and precocious puberty in girls. Ann Pediatr Endocrinol Metab. (2014) 19:91. doi: 10.6065/apem.2014.19.2.91
60. Sun J, Wang W, Xiao Y, Cao N, Wang Y, Zhang H, et al. Correlation between serum vitamin D level and uterine volume in girls with idiopathic central precocious puberty. J Pediatr Endocrinol Metab. (2024) 37:144–9. doi: 10.1515/jpem-2023-0381
61. Fu D, Li T, Zhang Y, Wang H, Wu X, Chen Y, et al. Analysis of the incidence and risk factors of precocious puberty in girls during the COVID-19 pandemic. Int J Endocrinol. (2022) 2022:9229153. doi: 10.1155/2022/9229153
62. Rafati M, Zamanfar D, Shiadeh SNR, Faramarzi F, Aarabi M, Damavandi HR. Serum vitamin D levels in girls with central precocious puberty. PBR. (2018) 4:15–9. doi: 10.18502/pbr.v4i1.141
63. Zhang J, Gao R, Jiang Y, Zhang Y, Liu C, Yu F, et al. Novel serological biomarker models composed of bone turnover markers, vitamin D, and estradiol and their auxiliary diagnostic value in girls with idiopathic central precocious puberty. Bone. (2022) 154:116221. doi: 10.1016/j.bone.2021.116221
64. Cui H, Liu P, Liu X, Du W, Wang X. The clinical value of serum-related indexes in differentiating simple premature thelarche from idiopathic central precocious puberty. Pak J Med Sci. (2023) 40:467–72. doi: 10.12669/pjms.40.3.7447
65. Wu C, Zhang X, Yan F, Cui Y, Song Y, Yan S, et al. Does vitamin D have a potential role in precocious puberty? A meta-analysis. Food Funct. (2023) 14:5301–5310. doi: 10.1039/D3FO00665D
66. Cheng H, Chen D, Gao H. An updated meta-analysis of the relationship between vitamin D levels and precocious puberty. Front Endocrinol. (2023) 14:1298374. doi: 10.3389/fendo.2023.1298374
67. Durá-Travé T, Gallinas-Victoriano F. Vitamin D status and parathyroid hormone assessment in girls with central precocious puberty. J Endocrinol Invest. (2022) 45:2069–75. doi: 10.1007/s40618-022-01838-y
68. Verrotti A, Chiarelli F, Montanaro AF, Morgese G. Bone mineral content in girls with precocious puberty treated with gonadotropin-releasing hormone analog. Gynecological Endocrinol. (1995) 9:277–81. doi: 10.3109/09513599509160459
69. Niu J, Xu L, Qian Y, Sun Z, Yu D, Huang J, et al. Evolution of the gut microbiome in early childhood: A cross-sectional study of Chinese children. Front Microbiol. (2020) 11:439. doi: 10.3389/fmicb.2020.00439
70. Stewart CJ, Ajami NJ, O’Brien JL, Hutchinson DS, Smith DP, Wong MC, et al. Temporal development of the gut microbiome in early childhood from the TEDDY study. Nature. (2018) 562:583–8. doi: 10.1038/s41586-018-0617-x
71. Dominguez-Bello MG, Costello EK, Contreras M, Magris M, Hidalgo G, Fierer N, et al. Delivery mode shapes the acquisition and structure of the initial microbiota across multiple body habitats in newborns. Proc Natl Acad Sci USA. (2010) 107:11971–5. doi: 10.1073/pnas.1002601107
72. Roswall J, Olsson LM, Kovatcheva-Datchary P, Nilsson S, Tremaroli V, Simon M-C, et al. Developmental trajectory of the healthy human gut microbiota during the first 5 years of life. Cell Host Microbe. (2021) 29:765–76.e3. doi: 10.1016/j.chom.2021.02.021
73. Derrien M, Alvarez A-S, De Vos WM. The gut microbiota in the first decade of life. Trends Microbiol. (2019) 27:997–1010. doi: 10.1016/j.tim.2019.08.001
74. Calcaterra V, Cena H, Sottotetti F, Rossi V, Loperfido F, Zuccotti G. Breast and formula milk and early puberty onset. Children. (2023) 10:1686. doi: 10.3390/children10101686
75. Korpela K, Kallio S, Salonen A, Hero M, Kukkonen AK, Miettinen PJ, et al. Gut microbiota develop towards an adult profile in a sex-specific manner during puberty. Sci Rep. (2021) 11:23297. doi: 10.1038/s41598-021-02375-z
76. Dong G, Zhang J, Yang Z, Feng X, Li J, Li D, et al. The association of gut microbiota with idiopathic central precocious puberty in girls. Front Endocrinol. (2020) 10:941. doi: 10.3389/fendo.2019.00941
77. Wang Y, Jin C, Li H, Liang X, Zhao C, Wu N, et al. Gut microbiota-metabolite interactions meditate the effect of dietary patterns on precocious puberty. iScience. (2024) 27:109887. doi: 10.1016/j.isci.2024.109887
78. Li Y, Shen L, Huang C, Li X, Chen J, Li SC, et al. Altered nitric oxide induced by gut microbiota reveals the connection between central precocious puberty and obesity. Clin Trans Med. (2021) 11:e299. doi: 10.1002/ctm2.299
79. Shin J-H. Serum level of sex steroid hormone is associated with diversity and profiles of human gut microbiome. Res Microbiol. (2019) 170:192–201. doi: 10.1016/j.resmic.2019.03.003
80. Hu S, Ding Q, Zhang W, Kang M, Ma J, Zhao L. Gut microbial beta-glucuronidase: a vital regulator in female estrogen metabolism. Gut Microbes. (2023) 15:2236749. doi: 10.1080/19490976.2023.2236749
81. Gao K, Pi Y, Mu C, Peng Y, Huang Z, Zhu W. Antibiotics-induced modulation of large intestinal microbiota altered aromatic amino acid profile and expression of neurotransmitters in the hypothalamus of piglets. J Neurochemistry. (2018) 146:219–34. doi: 10.1111/jnc.14333
82. Heiss CN, Mannerås-Holm L, Lee YS, Serrano-Lobo J, Håkansson Gladh A, Seeley RJ, et al. The gut microbiota regulates hypothalamic inflammation and leptin sensitivity in Western diet-fed mice via a GLP-1R-dependent mechanism. Cell Rep. (2021) 35:109163. doi: 10.1016/j.celrep.2021.109163
83. Huang X, Chen J, Zou H, Huang P, Luo H, Li H, et al. Gut microbiome combined with metabolomics reveals biomarkers and pathways in central precocious puberty. J Transl Med. (2023) 21:316. doi: 10.1186/s12967-023-04169-5
84. Macfarlane S, Macfarlane GT. Regulation of short-chain fatty acid production. Proc Nutr Soc. (2003) 62:67–72. doi: 10.1079/PNS2002207
85. Ghosh NK, Cox RP. Induction of human follicle-stimulating hormone in HeLa cells by sodium butyrate. Nature. (1977) 267:435–7. doi: 10.1038/267435a0
86. Liang Y, Ming Q, Liang J, Zhang Y, Zhang H, Shen T. Gut microbiota dysbiosis in polycystic ovary syndrome: association with obesity — a preliminary report. Can J Physiol Pharmacol. (2020) 98:803–9. doi: 10.1139/cjpp-2019-0413
87. Huang C, Liu H, Yang W, Li Y, Wu B, Chen J, et al. Distinct gut microbiota structure and function of children with idiopathic central and peripheral precocious puberty. Int J Endocrinol. (2022) 2022:1–11. doi: 10.1155/2022/7175250
88. Canfora EE, Jocken JW, Blaak EE. Short-chain fatty acids in control of body weight and insulin sensitivity. Nat Rev Endocrinol. (2015) 11:577–91. doi: 10.1038/nrendo.2015.128
89. Den Besten G, Van Eunen K, Groen AK, Venema K, Reijngoud D-J, Bakker BM. The role of short-chain fatty acids in the interplay between diet, gut microbiota, and host energy metabolism. J Lipid Res. (2013) 54:2325–40. doi: 10.1194/jlr.R036012
90. Vinolo MAR, Rodrigues HG, Nachbar RT, Curi R. Regulation of inflammation by short chain fatty acids. Nutrients. (2011) 3:858–76. doi: 10.3390/nu3100858
91. Tilg H, Moschen AR. Microbiota and diabetes: an evolving relationship. Gut. (2014) 63:1513–21. doi: 10.1136/gutjnl-2014-306928
92. Peng L, He Z, Chen W, Holzman IR, Lin J. Effects of butyrate on intestinal barrier function in a caco-2 cell monolayer model of intestinal barrier. Pediatr Res. (2007) 61:37–41. doi: 10.1203/01.pdr.0000250014.92242.f3
93. Tremaroli V, Bäckhed F. Functional interactions between the gut microbiota and host metabolism. Nature. (2012) 489:242–9. doi: 10.1038/nature11552
94. Wang L, Xu H, Tan B, Yi Q, Liu H, Deng H, et al. Gut microbiota and its derived SCFAs regulate the HPGA to reverse obesity-induced precocious puberty in female rats. Front Endocrinol. (2022) 13:1051797. doi: 10.3389/fendo.2022.1051797
95. Wu S, Zhang Y, Lu R, Xia Y, Zhou D, Petrof EO, et al. Intestinal epithelial vitamin D receptor deletion leads to defective autophagy in colitis. Gut. (2015) 64:1082–94. doi: 10.1136/gutjnl-2014-307436
96. Kim S-J. Leptin potentiates Prevotella intermedia lipopolysaccharide-induced production of TNF-α in monocyte-derived macrophages. J Periodontal Implant Sci. (2010) 40:119. doi: 10.5051/jpis.2010.40.3.119
97. Sayin SI, Wahlström A, Felin J, Jäntti S, Marschall H-U, Bamberg K, et al. Gut microbiota regulates bile acid metabolism by reducing the levels of tauro-beta-muricholic acid, a naturally occurring FXR antagonist. Cell Metab. (2013) 17:225–35. doi: 10.1016/j.cmet.2013.01.003
98. Prawitt J, Abdelkarim M, Stroeve JHM, Popescu I, Duez H, Velagapudi VR, et al. Farnesoid X receptor deficiency improves glucose homeostasis in mouse models of obesity. Diabetes. (2011) 60:1861–71. doi: 10.2337/db11-0030
99. Joyce SA, MacSharry J, Casey PG, Kinsella M, Murphy EF, Shanahan F, et al. Regulation of host weight gain and lipid metabolism by bacterial bile acid modification in the gut. Proc Natl Acad Sci USA. (2014) 111:7421–6. doi: 10.1073/pnas.1323599111
100. Fakhoury Hana MA, Kvietys PR, AlKattan W, Anouti FA, Elahi MA, Karras SN, et al. Vitamin D and intestinal homeostasis: Barrier, microbiota, and immune modulation. J Steroid Biochem Mol Biol. (2020) 200:105663. doi: 10.1016/j.jsbmb.2020.105663
101. Singh P, Rawat A, Alwakeel M, Sharif E, Al Khodor S. The potential role of vitamin D supplementation as a gut microbiota modifier in healthy individuals. Sci Rep. (2020) 10:21641. doi: 10.1038/s41598-020-77806-4
102. Assa A, Vong L, Pinnell LJ, Avitzur N, Johnson-Henry KC, Sherman PM. Vitamin D deficiency promotes epithelial barrier dysfunction and intestinal inflammation. J Infect Dis. (2014) 210:1296–305. doi: 10.1093/infdis/jiu235
103. Yamamoto EA, Jørgensen TN. Relationships between vitamin D, gut microbiome, and systemic autoimmunity. Front Immunol. (2020) 10:3141. doi: 10.3389/fimmu.2019.03141
104. Wang J, Thingholm LB, Skiecevičienė J, Rausch P, Kummen M, Hov JR, et al. Genome-wide association analysis identifies variation in vitamin D receptor and other host factors influencing the gut microbiota. Nat Genet. (2016) 48:1396–406. doi: 10.1038/ng.3695
105. Vernia F, Valvano M, Longo S, Cesaro N, Viscido A, Latella G. Vitamin D in inflammatory bowel diseases. Mechanisms of action and therapeutic implications. Nutrients. (2022) 14:269. doi: 10.3390/nu14020269
106. Waterhouse M. Vitamin D and the gut microbiome: a systematic review of in vivo studies. Eur J Nutr. (2019) 58:2895–910. doi: 10.1007/s00394-018-1842-7
107. Drall KM, Field CJ, Haqq AM, De Souza RJ, Tun HM, Morales-Lizcano NP, et al. Vitamin D supplementation in pregnancy and early infancy in relation to gut microbiota composition and C. difficile colonization: implications for viral respiratory infections. Gut Microbes. (2020) 12:1799734. doi: 10.1080/19490976.2020.1799734
108. Luthold RV, Fernandes GR, Franco-de-Moraes AC, Folchetti LGD, Ferreira SRG. Gut microbiota interactions with the immunomodulatory role of vitamin D in normal individuals. Metabolism. (2017) 69:76–86. doi: 10.1016/j.metabol.2017.01.007
109. Indiani CMDSP, Rizzardi KF, Castelo PM, Ferraz LFC, Darrieux M, Parisotto TM. Childhood obesity and firmicutes/bacteroidetes ratio in the gut microbiota: A systematic review. Childhood Obes. (2018) 14:501–9. doi: 10.1089/chi.2018.0040
110. Ferrer M, Ruiz A, Lanza F, Haange S, Oberbach A, Till H, et al. Microbiota from the distal guts of lean and obese adolescents exhibit partial functional redundancy besides clear differences in community structure. Environ Microbiol. (2013) 15:211–26. doi: 10.1111/j.1462-2920.2012.02845.x
111. Mbakwa CA, Hermes GDA, Penders J, Savelkoul PHM, Thijs C, Dagnelie PC, et al. Gut microbiota and body weight in school-aged children: the KOALA birth cohort study. Obesity. (2018) 26:1767–76. doi: 10.1002/oby.22320
112. Bo T, Liu M, Tang L, Lv J, Wen J, Wang D. Effects of high-fat diet during childhood on precocious puberty and gut microbiota in mice. Front Microbiol. (2022) 13:930747. doi: 10.3389/fmicb.2022.930747
113. Khayyatzadeh SS, Mirmoosavi SJ, Fazeli M, Abasalti Z, Avan A, Javandoost A, et al. High-dose vitamin D supplementation is associated with an improvement in several cardio-metabolic risk factors in adolescent girls: a nine-week follow-up study. Ann Clin Biochem. (2018) 55:227–35. doi: 10.1177/0004563217707784
114. Corsello A, Milani GP, Giannì ML, Dipasquale V, Romano C, Agostoni C. Different vitamin D supplementation strategies in the first years of life: A systematic review. Healthcare. (2022) 10:1023. doi: 10.3390/healthcare10061023
115. Chang S-W, Lee H-C. Vitamin D and health - The missing vitamin in humans. Pediatr Neonatology. (2019) 60:237–44. doi: 10.1016/j.pedneo.2019.04.007
116. Belenchia AM, Tosh AK, Hillman LS, Peterson CA. Correcting vitamin D insufficiency improves insulin sensitivity in obese adolescents: a randomized controlled trial. Am J Clin Nutr. (2013) 97:774–81. doi: 10.3945/ajcn.112.050013
117. Sacheck JM, Huang Q, Van Rompay MI, Chomitz VR, Economos CD, Eliasziw M, et al. Vitamin D supplementation and cardiometabolic risk factors among diverse schoolchildren: a randomized clinical trial. Am J Clin Nutr. (2022) 115:73–82. doi: 10.1093/ajcn/nqab319
118. Alves AGP, Cruvinel BAC, Schincaglia RM, Godoi LS, Silva MS. Vitamin D supplementation reduces serum lipids of children with hypertriacylglycerolemia: A randomized, triple-masked, placebo-controlled crossover trial. Nutrition. (2021) 89:111296. doi: 10.1016/j.nut.2021.111296
119. Al-Daghri NM, Amer OE, Khattak MNK, Sabico S, Ghouse Ahmed Ansari M, Al-Saleh Y, et al. Effects of different vitamin D supplementation strategies in reversing metabolic syndrome and its component risk factors in adolescents. J Steroid Biochem Mol Biol. (2019) 191:105378. doi: 10.1016/j.jsbmb.2019.105378
120. Shah S, Wilson DM, Bachrach LK. Large doses of vitamin D fail to increase 25-hydroxyvitamin D levels or to alter cardiovascular risk factors in obese adolescents: A pilot study. J Adolesc Health. (2015) 57:19–23. doi: 10.1016/j.jadohealth.2015.02.006
121. Hauger H, Mølgaard C, Mortensen C, Ritz C, Frøkiær H, Smith TJ, et al. Winter cholecalciferol supplementation at 55°N has no effect on markers of cardiometabolic risk in healthy children aged 4–8 years. J Nutr. (2018) 148:1261–8. doi: 10.1093/jn/nxy080
122. Smith TJ, Tripkovic L, Hauger H, Damsgaard CT, Mølgaard C, Lanham-New SA, et al. Winter cholecalciferol supplementation at 51°N has no effect on markers of cardiometabolic risk in healthy adolescents aged 14–18 years. J Nutr. (2018) 148:1269–75. doi: 10.1093/jn/nxy079
123. Putman MS, Pitts SAB, Milliren CE, Feldman HA, Reinold K, Gordon CM. A randomized clinical trial of vitamin D supplementation in healthy adolescents. J Adolesc Health. (2013) 52:592–8. doi: 10.1016/j.jadohealth.2012.10.270
124. Nader NS, Aguirre Castaneda R, Wallace J, Singh R, Weaver A, Kumar S. Effect of vitamin D3 supplementation on serum 25(OH)D, lipids and markers of insulin resistance in obese adolescents: A prospective, randomized, placebo-controlled pilot trial. Horm Res Paediatr. (2014) 82:107–12. doi: 10.1159/000362449
125. Javed A, Vella A, Balagopal PB, Fischer PR, Weaver AL, Piccinini F, et al. Cholecalciferol supplementation does not influence β-cell function and insulin action in obese adolescents: A prospective double-blind randomized trial. J Nutr. (2015) 145:284–90. doi: 10.3945/jn.114.202010
126. Kelishadi R, Salek S, Salek M, Hashemipour M, Movahedian M. Effects of vitamin D supplementation on insulin resistance and cardiometabolic risk factors in children with metabolic syndrome: a triple-masked controlled trial. Jornal Pediatria. (2014) 90:28–34. doi: 10.1016/j.jped.2013.06.006
127. Yarparvar A, Elmadfa I, Djazayery A, Abdollahi Z, Salehi F, Heshmat R. The effects of vitamin D supplementation on lipid and inflammatory profile of healthy adolescent boys: A randomized controlled trial. Nutrients. (2020) 12:1213. doi: 10.3390/nu12051213
128. Hirschler V, Maccallini G, Sanchez MS, Castaño L, Molinari C, on behalf of San Antonio de los Cobres Study Group. Improvement in high-density lipoprotein cholesterol levels in argentine Indian school children after vitamin D supplementation. Horm Res Paediatr. (2013) 80:335–42. doi: 10.1159/000355511
129. Brzeziński M, Jankowska A, Słomińska-Frączek M, Metelska P, Wiśniewski P, Socha P, et al. Long-term effects of vitamin D supplementation in obese children during integrated weight–loss programme—A double blind randomized placebo–controlled trial. Nutrients. (2020) 12:1093. doi: 10.3390/nu12041093
130. Ganmaa D, Bromage S, Khudyakov P, Erdenenbaatar S, Delgererekh B, Martineau AR. Influence of vitamin D supplementation on growth, body composition, and pubertal development among school-aged children in an area with a high prevalence of vitamin D deficiency: A randomized clinical trial. JAMA Pediatr. (2023) 177:32. doi: 10.1001/jamapediatrics.2022.4581
131. Boughanem H, Ruiz-Limón P, Pilo J, Lisbona-Montañez JM, Tinahones FJ, Moreno Indias I, et al. Linking serum vitamin D levels with gut microbiota after 1-year lifestyle intervention with Mediterranean diet in patients with obesity and metabolic syndrome: a nested cross-sectional and prospective study. Gut Microbes. (2023) 15:2249150. doi: 10.1080/19490976.2023.2249150
132. Wang M, Zhang Y, Miller D, Rehman NO, Cheng X, Yeo J-Y, et al. Microbial reconstitution reverses early female puberty induced by maternal high-fat diet during lactation. Endocrinology. (2020) 161:bqz041. doi: 10.1210/endocr/bqz041
133. Pham VT, Fehlbaum S, Seifert N, Richard N, Bruins MJ, Sybesma W, et al. Effects of colon-targeted vitamins on the composition and metabolic activity of the human gut microbiome– a pilot study. Gut Microbes. (2021) 13:1875774. doi: 10.1080/19490976.2021.1875774
Keywords: central precocious puberty, vitamin D deficiency, gut microbiota, prevention, treatment
Citation: Guo D, Ning X, Bai T, Tan L, Zhou Y, Guo Z and Li X (2024) Interaction between Vitamin D homeostasis, gut microbiota, and central precocious puberty. Front. Endocrinol. 15:1449033. doi: 10.3389/fendo.2024.1449033
Received: 14 June 2024; Accepted: 22 November 2024;
Published: 09 December 2024.
Edited by:
Valentina Chiavaroli, Pescara Public Hospital, ItalyReviewed by:
Paul B. Kaplowitz, Children’s National Hospital, United StatesCopyright © 2024 Guo, Ning, Bai, Tan, Zhou, Guo and Li. This is an open-access article distributed under the terms of the Creative Commons Attribution License (CC BY). The use, distribution or reproduction in other forums is permitted, provided the original author(s) and the copyright owner(s) are credited and that the original publication in this journal is cited, in accordance with accepted academic practice. No use, distribution or reproduction is permitted which does not comply with these terms.
*Correspondence: Xin Li, MzE0NDQwODIwQHFxLmNvbQ==
Disclaimer: All claims expressed in this article are solely those of the authors and do not necessarily represent those of their affiliated organizations, or those of the publisher, the editors and the reviewers. Any product that may be evaluated in this article or claim that may be made by its manufacturer is not guaranteed or endorsed by the publisher.
Research integrity at Frontiers
Learn more about the work of our research integrity team to safeguard the quality of each article we publish.