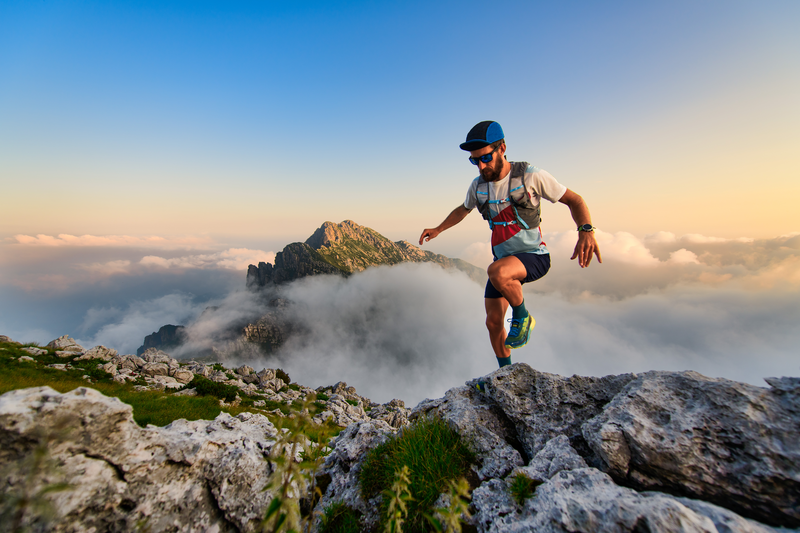
95% of researchers rate our articles as excellent or good
Learn more about the work of our research integrity team to safeguard the quality of each article we publish.
Find out more
REVIEW article
Front. Endocrinol. , 11 September 2024
Sec. Reproduction
Volume 15 - 2024 | https://doi.org/10.3389/fendo.2024.1427069
This article is part of the Research Topic Oxidative Stress and Male Fertility View all 11 articles
Hydrogen sulfide (H2S) is an endogenously produced signaling molecule that belongs to the group of gasotransmitters along with nitric oxide (NO) and carbon monoxide (CO). H2S plays a pivotal role in male reproductive processes. It is produced in various tissues and cells of the male reproductive system, including testicular tissue, Leydig and Sertoli cells, epididymis, seminal plasma, prostate, penile tissues, and sperm cells. This review aims to summarize the knowledge about the presence and effects of H2S in male reproductive tissues and outline possible therapeutic strategies in pathological conditions related to male fertility, e. g. spermatogenetic disorders and erectile dysfunction (ED). For instance, H2S supports spermatogenesis by maintaining the integrity of the blood-testicular barrier (BTB), stimulating testosterone production, and providing cytoprotective effects. In spermatozoa, H2S modulates sperm motility, promotes sperm maturation, capacitation, and acrosome reaction, and has significant cytoprotective effects. Given its vasorelaxant effects, it supports the erection of penile tissue. These findings suggest the importance and therapeutic potential of H2S in male reproduction, paving the way for further research and potential clinical applications.
Hydrogen sulfide (H2S) is a gasotransmitter, a gaseous signaling molecule that provides cell signaling through a series of intracellular signaling cascades. H2S is produced in mammalian tissues via the transsulfuration pathway, which involves the interconversion of cysteine and homocysteine through cystathionine. In mammals, H2S is synthesized by the enzymes cystathionine-β-synthase (CBS) and cystathionine-γ-lyase (CTH) (1), which require pyridoxal-5’-phosphate (PLP) as a cofactor and use L-cysteine to produce H2S (2). Some studies suggest that the effect of CBS and CTH on L-cysteine is the main pathway to produce endogenous H2S (3). However, several other pathways have been described (Figure 1). H2S can be produced, for example, by 3-mercaptopyruvate sulfurtransferase (3-MST), which is also one of the H2S-producing enzymes (1), which, unlike the previous two, is not dependent on PLP. The production of H2S through 3-MST also requires cysteine aminotransferase (CAT), which catalyzes the reaction of cysteine with keto acids to form 3-mercaptopyruvate and the corresponding amino acid. 3-MST then catalyzes the conversion of 3-mercaptopyruvate to pyruvate and H2S (4, 5) in the presence of reducing agents such as thioredoxin or dihydrolipoic acid (6). In 2013, Shibuya et al. (7) described another pathway of endogenous H2S production, which involves two enzymes – diamine oxidase (DAO) and 3-MST. DAO catalyzes the conversion of D-cysteine to 3-mercaptopyruvate, a substrate for 3-MST (7).
Figure 1. Enzymatic production of endogenous H2S. The main producers of H2S are the enzymes CBS and CTH, which use L-cysteine to produce H2S. Another possible route of H2S formation from L-cysteine is the activity of 3-MST and CAT. In addition to L-cysteine, D-cysteine can also be another substrate.
The H2S production has been described in several mammalian tissues in which H2S has a specific function. Endogenous H2S uses several signaling pathways to engage in physiological processes. In many mammalian systems, the effect of H2S is mediated by ATP-sensitive potassium channels (KATP) (8, 9). Other signaling pathways involve T- and L-type calcium channels (10, 11), large conductance calcium-activated potassium channels (BKCa) (12, 13), MAPK signaling cascade (14), mitochondrial cytochrome c oxidase (15) and transient receptor potential ion channel 1 (TRPA 1) (16). In addition to acting on various signaling pathways, H2S is a potent reducing agent that protects sulfhydryl groups of proteins from oxidation thanks to its reactivity with oxygen and nitrogen radicals (17–20). In some tissues, H2S deficiency or excess can affect the pathogenesis of some diseases. An insufficient concentration of H2S has been described, for example, in Alzheimer’s or Huntington’s disease (17, 21), whereas overexpression of hydrogen sulfide-producing enzymes, such as CTH, is often associated with the presence of testicular neoplasms, embryonic carcinoma (22), or prostate cancer (23, 24). At the same time, this higher expression of CTH correlates with higher aggressiveness of cancer (22, 25, 26). Since early detection is crucial for cancer treatment, new methods have been developed in recent years that can detect H2S in these tissues and could also help in the early diagnosis of cancer (27, 28).
H2S and its synthases have also been demonstrated in the male reproductive tract. For example, CBS has been reported in testicular germ cells, Sertoli and Leydig cells, and CTH has been described in immature testicular germ cells and Sertoli cells (29). H2S-producing enzymes have also been detected in spermatozoa (30), seminal plasma (31), epididymis (32), vas deferens (13, 33), prostate (24, 34) and penile tissue (35–37). The presence of H2S and its synthases in particular parts of the male reproductive tract in different species are listed in Table 1. H2S is currently considered to be a modulator of physiological sexual function in both sexes (45–48), so it is the reason why many scientific publications have been published in recent years dealing with the function of H2S in different parts of the male reproductive tract (Figure 2). It has been described that H2S increases the antioxidant capacity of sperm (49), has anti-inflammatory and antioxidant effects on testicular cells (50, 56), promotes testosterone production (57, 58) and can support (31, 59) and suppress (31, 32, 60, 61) sperm motility. One of the most critical functions of H2S in the male reproductive system is vasorelaxation of smooth muscle, where it helps to relax the smooth muscles of the vas deferens (33), prostate (34) or corpus cavernosum (CC) (35, 53, 62). Smooth muscle relaxation of CC with H2S is a highly discussed topic because the treatment of ED in some patients is not sufficient with conventional medications, and H2S-based compounds could, therefore, be another possible treatment used for ED patients (42, 54).
Figure 2. Summary of H2S functions in different parts of the male reproductive tract. H2S has an impact on various sperm functions, it enhances sperm and testicular antioxidant capacity (38, 49, 50) and inhibits superoxide generation in penile tissue (51, 52). H2S effectively promotes erectile function (53), and this gasotransmitter is a promising therapeutic agent for the treatment of erectile dysfunction (42, 54). A key function of H2S is protein persulfidation, through which H2S can modify proteins and regulate various signaling pathways (55). H2S also plays a crucial role in facilitating ion transport across the epididymal epithelium (32) and significantly enhances BTB constitutive protein expression in the testis (31), thereby promoting spermatogenesis and testosterone biosynthesis. H2S can also effectively regulate smooth muscle relaxation in vas deferens (33) and prostate tissue (34).
H2S activates several signaling pathways in spermatozoa (Figure 3) which participate in the development of sperm motility, capacitation, and acrosome reaction, such as the activating MAPK pathway involving four central cascades (ERK 1/2, JNK, p38, and ERK5) (31, 63). H2S also affects sperm ion channels (Ca2+, K+, Na+), which are also concerned with the physiological processes of spermatozoa (64). An example of the Ca2+ channel regulation by H2S is the cation channels of sperm (CatSper) engaged in capacitation, hyperactivation, acrosome response, and sperm chemotaxis ability (65). In addition, the opening of K+ channels by H2S regulates ATP production in the mitochondria, which supports progressive sperm motility and hyperactivation (38, 66). Other molecular targets of H2S are some of the subfamilies of transient vanilloid receptor proteins (TRPVs) (64). The TRPV1 channel has been described in the acrosome and flagellum of bull spermatozoa, where it promotes progressive motility, capacitation, including hyperactivity, and acrosome reaction (67). Similarly, the TRPV4 channel has been detected in the flagellum and acrosome of human spermatozoa and is involved in sperm capacitation associated with motility hyperactivation. In addition, TRPV1 mediates Na+ influx and subsequent membrane depolarization, activating other important ion channels related to sperm capacitation (e.g., CatSper) (68, 69).
Figure 3. H2S signaling cascades in spermatozoa. H2S activates the MAPK signaling cascade, further affecting ion channels (mainly potassium and calcium) and some TRPV channels (TRPV1, TRPV4). All these signaling cascades affect sperm motility, capacitation, hyperactivation, or acrosome reaction, and CatSper channels can also engage sperm chemotaxis ability.
H2S-producing enzymes are mainly found in the midpiece of the human, boar, and mouse sperm flagellum, but during epididymal maturation and capacitation, their sequential disappearance occurs probably due to plasma membrane remodeling (30). These results suggest that H2S is not involved in oocyte fertilization alone but in the preceding processes. Given the presence of H2S-producing enzymes in the midpiece of the flagellum, it seems likely that H2S could be involved primarily in the development of sperm motility by promoting ATP production in mitochondria. The effect of H2S on sperm motility is the subject of many research studies describing both the positive and negative effects of H2S on this important functional sperm parameter. One of the negative impacts may be the action of the sodium sulfide (Na2S) donor, which releases H2S rapidly in high concentrations, in vitro reducing sperm motility (70). Reduction of sperm motility by H2S includes decreasing Na+/K+ ATPase activity, which is known to affect spermatogenesis, metabolism (71), and sperm motility of various mammalian species (e.g., mice, stallions, humans) (72–75), and protein kinase B (Akt) levels, activating adenosine 5’-monophosphate (AMP)-activated protein kinase (AMPK) and phosphatase and tensin homolog deleted on chromosome ten (PTEN), and increasing reactive oxygen species (ROS) (70). This result has also been confirmed by Wang et al. (61) in an in vivo study on mice, which described the same negative effect of a donor-different gasotransmitter (NaHS) on sperm chemotactic abilities when treated at a dose of 50 mg/kg daily. The reason for the negative impact of H2S donors on sperm motility is probably the fact that some H2S donors release this gasotransmitter in concentrations that are supraphysiological and, therefore, unfavorable for sperm function, which can cause a decrease in sperm motility (70).
Compared to these results, a positive effect of H2S donors on sperm motility has also been described in oligoasthenozoospermic (ejaculate with reduced sperm concentration and motility) and asthenospermic (ejaculate with reduced sperm motility) ejaculates, in which lower activity of CBS in seminal plasma was measured in comparison to healthy men. Spermatozoa of these patients were in vitro exposed to two H2S donors – GYY4137 (10 µM), releasing H2S slowly in low concentrations, and NaHS (5 µM), rapidly releasing H2S in high concentrations. The first of the mentioned donors (GYY4137) led to improved sperm motility in contrast to NaHS (31). However, improvement in sperm motility occurred only in patients with lower levels of H2S (< 18 µM) in seminal plasma, which is probably related to the activation of the MAPK signaling pathway (31), which fundamentally affects sperm motility, morphology, and capacitation (76, 77). Other effective molecules in terms of sperm motility and concentration are the H2S precursor SG1002 (in vivo study on men, 750 mg of SG1002 daily) (78) and finally, the amino acid-derived N-thiocarboxyanhydrides (NTAs), which releases H2S in the presence of carbonic anhydrase, in vitro increasing progressive sperm motility and prolonging sperm survival through supported mitochondrial activity (59). These results suggest that H2S concentration probably plays a crucial role in the effect on sperm motility.
One of the leading causes of male infertility is oxidative stress, so experiments have been conducted focusing on using various H2S donors, which are considered potent antioxidants (49, 55). The donor GYY4137 releasing H2S slowly in small concentrations can maintain sperm motility in vitro, even under conditions of increased oxidative stress. Conversely, the in vitro effect of NaHS, which releases H2S rapidly in high concentrations, preserves sperm motility only in lower concentrations, while in higher concentrations (300 μM), it appears to be cytotoxic. However, both mentioned donors and amino acid NTAs can mitigate damage caused by oxidative stress, thereby increasing sperm antioxidant capacity (49, 59). In addition to its direct ability to neutralize ROS (like O2H, O2-, OH, etc.), H2S also enhances the expression of antioxidant enzymes, for example, glutathione peroxidase (GSH-Px) or superoxide dismutase (SOD), through nuclear factor erythroid-derived 2 (Nrf2) activation and translocation, which regulates the expression of antioxidant proteins (79).
H2S facilitates the persulfidation (S-sulfhydratation) (55, 80), posttranslational modification converting thiols (RSH) to persulfides (RSSH, RSSSH, etc.) (Figure 4) (81) when H2S modifies proteins by attaching a sulfhydryl group to specific cysteine residues (82). This protein modification is a redox process, and it is significant in many signaling pathways relevant to sperm development (48). This modification can be reversible and dynamic, allowing precise regulations of protein functions (83, 84). Persulfidation prevents protein damage caused by irreversible cysteine hyperoxidation, which can be detrimental to protein function (30). Persulfidation protects proteins from irreversible oxidative damage, particularly in environments with high oxidative stress (83, 84). In the context of male reproductive health, this protective mechanism is crucial for maintaining the integrity of sperm proteins, which are vital for sperm motility and function. Persulfidation has been shown to be relatively common in the testes (30), with almost 244 identified persulfidated proteins in human sperm. Interestingly, in asthenozoospermic patients, histones H3.1 and H3.3 on cysteine 111 exhibit significantly lower levels of persulfidation, potentially leading to reduced sperm motility and contributing to infertility (55). Proteins like GADPH, tubulin, and anchor protein A-kinase, which are involved in flagellum structure and sperm motility, are identified as persulfidated (30, 83). The interaction of H2S with GADPH, a critical enzyme for sperm motility, enhances cysteine reactivity, indicating that persulfidation could be responsible for the beneficial effects of H2S on sperm movement (80).
Figure 4. Various reaction mechanisms of persulfidation. In solutions, H2S dissociates into H+ and HS−. Protein persulfidation can result from sulfide anion reactions on oxidized protein thiol, including S-OH, S-N=O, S-SG, and S-SR.
Endogenous production of H2S has been confirmed in testicular tissue, and it is assumed that its production is necessary for spermatogenesis (30, 31, 38). Reduced H2S production results in impaired spermatogenesis, decreased MAPK phosphorylation, and disruption of the BTB, which plays a critical role in spermatogenesis. Conversely, the presence of H2S in vivo increases the resistance and expression of the BTB constitutive protein under conditions of increased oxidative stress (31). However, the effect of H2S in this tissue is highly dependent on its concentration, as is the case in spermatozoa. On the contrary, in vivo, elevated H2S levels induced by some donors (NaHS, Na2S) can damage BTB integrity, decrease BTB-related gene expression rates, reduce testosterone levels, damage seminiferous tubules, and increase p38 MAPK phosphorylation, a signaling pathway, which regulates cell proliferation, differentiation, apoptosis, and stress response (31). These effects are thought to be due to inhibiting ATP production, specifically mitochondrial Complex IV (85).
Antioxidant, antiapoptotic, and anti-inflammatory effects of H2S have been observed in various systems (38, 86–92). Therefore, the H2S-producing enzymes CBS and CTH play an essential role in the testis by synthesizing the antioxidant GSH-Px in the transsulfuration pathway, promoting male fertility. Decreased expression of CBS and CTH in testicular tissue leads to a reduced ability to respond to oxidative stress, which may cause a decrease in sperm concentration and motility (56). The antioxidant capacity of H2S consists in the activation of SOD, which reduces the level of ROS in testicular germ cells. H2S can further promote mitochondrial function, increase ATP production, and decrease ROS production (38, 50). In addition, Mao et al. (93) described a beneficial effect of H2S in attenuating acrolein-induced Sertoli cell and germ cell damage in vitro, which underlies several reproductive injuries (94–97), suggesting that H2S might be used in the future to prevent and treat acrolein-related reproductive injury.
The antioxidant effect of H2S is often associated with reducing ischemia-reperfusion injury in treating testicular torsion. By measuring the levels of some antioxidant substances (SOD, reduced glutathione) and oxidative stress, it was concluded that H2S contributes to anti-inflammatory, antioxidant, antiapoptotic, and antifibrotic activities in the treatment of testicular torsion (90, 92) via inhibition of inflammatory cytokines (92). H2S donor GYY4137 has been confirmed to in vivo protect against ischemia-reperfusion injury and attenuate histopathological changes after testicular torsion/detorsion, as well as mediate an increase in antioxidant capacity (89, 92), reduce apoptosis of spermatogenic cells, and increase the expression level of heat shock protein 70 (89), which helps prevent cell apoptosis during heat stress in testicular cells (98), preserve sperm motility in cryopreserved bull sperm (99), and protect proteins and DNA under stress conditions (100). According to the results of these studies, H2S treatment has beneficial effects on biochemical and histopathological damage in testicular torsion. Another study focusing on the protective function of H2S investigated the in vivo effect of H2S on the heat stress of testicular germ cells. Heat exposure significantly reduced endogenous H2S production and CBS and CTH expression in testes. NaHS application (5,6 mg/kg) alleviated heat stress in testicular germ cells and induced cell death and apoptosis. The number of apoptotic cells was significantly lower, suggesting that H2S may protect testicular germ cells through its anti-apoptotic effects (38), likely mediated by the inactivation of the intrinsic apoptosis pathway, as the Bax/Bcl-2 protein expression ratio was reduced but caspase activity was unchanged (38, 89). NaHS also improved mitochondrial function by reducing oxygen consumption and increasing ATP production, SOD activity was stimulated, and ROS production was reduced. Consequently, exogenous H2S may protect germ cells by preserving mitochondrial function and stimulating antioxidant activity (38).
The protective effect of H2S has also been studied in vivo concerning varicocele. It has been confirmed that the use of GYY4137 (5–20 mg/kg) alleviates damage to the testis and ductus epididymis and reduces the number of apoptotic epithelial cells in the epididymis, likely due to a reduction in the levels of caspase-3 and Bax (88, 101). GYY4137 also reduces oxidative stress markers and increases this tissue’s antioxidant activity. In addition, it likely activates the phosphatidylinositol 3-kinase/protein kinase B (PI3K/Akt) pathway, which regulates the cell cycle and is associated with increased sperm motility and may counteract the effects of oxidative stress (101).
Due to the protective effects of H2S, its effects have also been investigated about testicular toxicity and male infertility induced by anticancer drugs. Several studies focusing on this topic have described a positive effect of H2S against testicular toxicity. As an example, the in vivo experiment by Azarbarz et al. (102) confirmed that NaHS administration (200 µg/kg/day) provides a significant improvement in biochemical, histological, and morphometric changes (decrease in testicular weight, plasma testosterone concentration, seminiferous tubule diameter, germinal epithelium thickness, Sertoli cell count, spermatogonia and spermatocytes, Johnsen testicular score and testicular antioxidant enzymes induced by cisplatin (102). Cisplatin is a potent anticancer drug, but its use is limited due to its ability to generate free radicals that are highly toxic to specific organs, such as the kidney and testis (103). A similar in vivo experiment was performed by Özatik et al. (91), who investigated the effect of NaHS on testicular dysfunction induced by cyclophosphamide, a drug used to treat many malignancies but which can also cause serious side effects such as hemorrhagic cystitis and male infertility. NaHS (25–100 µmol/kg) has been found to prevent the increase in interleukin 6 and 10 levels, decrease in cGMP, increase in luteinizing hormone (LH), and decrease in testosterone that has been related to the effects of cyclophosphamide (91). Testicular toxicity can also be caused by the carcinogenic water-soluble acrylamide, which is used in paper and plastics manufacturing (104) and has been detected in some cosmetic products, creams, and lotions (105). In addition, acrylamide is also formed naturally during frying or baking at temperatures above 120°C and low humidity (106). Mokhlis et al. (107) described the reversal of the adverse effects of acrylamide-induced testicular toxicity using NaHS in vivo and described the protective effects of H2S in this exposure (200 µg/kg NaHS) (107). Testicular toxicity can be further induced by nanoplasts, which accumulate in the testes, cause seminiferous tubular degeneration, and induce ROS-dependent mitochondrial apoptosis. Therefore, Li et al. (50) conducted in vitro experiments that confirmed the protective function of H2S in testicular toxicity. NaHS (250 µM) improved the antioxidant capacity by increasing the protein levels of NAD(P)H dehydrogenase quinone 1 (NQO1) and heme oxygenase-1 (HO-1), which synthesizes CO, another gasotransmitter involved in the regulation of various physiological processes in the body (108). These results suggest that H2S donors may be a promising therapy not only for treating varicocele and its symptoms but also for mitigating the adverse effects of anticancer drugs and other agents that cause testicular toxicity.
Low testosterone levels and hypogonadism occur in 2.1–5.7% of men aged 40–79 years and may be a cause of male infertility (109). However, the crucial role of H2S in testosterone biosynthesis has recently been described, the clarification of which could help in the treatment of these disorders associated with low levels of testosterone (58, 61). H2S has been described to increase steroid production in Leydig cells and the expression of genes associated with testicular testosterone biosynthesis (StAR, p450c17, 3beta-HSD, P450scc) in vivo. H2S further enhanced SOD and GSH-Px activity, again pointing to its antioxidant effects (58). Another research team looked at the potential solutions to testosterone secretion disorders using H2S in vivo and in vitro (110). Overexpression of CBS was found to inhibit phosphodiesterase 4A (PDE4A) and phosphodiesterase 8A (PDE8A) via persulfidation, implicating that it is possible that by inhibiting PDE expression via persulfidation and activating the cAMP/PKA pathway that regulates testosterone synthesis, which could be restored (Figure 5). Furthermore, H2S may play an important role in testicular testosterone secretion in vivo, which is influenced by LH secretion, because the sulfides contained in garlic support the secretion of testosterone in the testicles precisely by controlling the secretion of LH (111).
Figure 5. Effect of H2S on testosterone biosynthesis. (A) Signaling cascade of testosterone secretion; (B) Disruption of the signaling cascade of testosterone secretion by overproduction of PDE, which degrades cAMP, which leads to a decrease in the activity of the PKA signaling pathway; (C) Persulfidation of PDE by H2S and its subsequent inhibition, which ensures activation of the PKA/cAMP signaling pathway and subsequent testosterone synthesis.
The role of H2S in epididymis is likely to be in regulating epididymal transepithelial ion transport. In rats, H2S has been found to induce transepithelial K+ secretion via KATP and BKCa channels in vivo. The activation of BKCa channels by H2S is mediated via TRPV4 channels and subsequent Ca2+ influx (32). The expression of the H2S-producing enzymes CBS and CTH increases in the epididymis from the caput to the cauda epididymis, corresponding to the increasing production of endogenous H2S (32). Moreover, the K+ concentration in rat intraluminal fluid also increases from the caput to the cauda, which supports the idea that H2S increases K+ secretion (32, 112). In the caput epididymis, the function of H2S is probably in reducing sperm motility by increasing the extracellular concentration of K+ ions, keeping them in a quiescent state before ejaculation (32).
H2S production has also been described in vas deferens (13, 33), and all three H2S-producing enzymes have been detected in the prostate, with the highest abundance of CTH, which is likely the main H2S producer in this tissue (23, 24, 113, 114). The main role of H2S in the vas deferens and prostate is primarily to relax smooth muscle (33, 34). In addition, H2S also plays an important role in the detection and treatment of prostate cancer. Prostate cancer is the second most frequent malignancy in men and the fifth leading cause of death worldwide (115). As early as 40 years ago, clinical practice demonstrated that water treatment with H2S improves prostate blood flow in patients with chronic prostatitis, indicating a beneficial role of H2S in prostate tissue in the pathological state (116). Endogenous H2S plays a vital role in tumor growth in a variety of cancers through induction of angiogenesis, regulation of mitochondrial bioenergetics, cell cycle acceleration, and antiapoptosis (117), and regulation of cell proliferation (23). In vivo and in vitro studies have described that H2S, its donors, or some sulfocompounds have anticancer effects (23, 118–125). The results suggest that a diet or drug containing H2S-releasing substances could be beneficial in the treatment of prostate cancer (23). However, some authors have described that excessive levels of CTH/H2S in the prostate, on the contrary, may promote prostate cancer progression and that their inhibition leads to suppression of tumor growth. This suggests that CTH and H2S could be potential therapeutic targets in intervening in prostate cancer progression (26). Moreover, the detection of elevated CTH/H2S levels could help early prostate cancer detection (27, 28).
The first effect of H2S on penile physiology was described in 2006 when intracavernous injection of NaHS (1–10µmol/kg) significantly increased penile length and cavernous pressure in primates (53). A key event during penile erection is the relaxation of the CC smooth muscle, leading to an increase in arterial flow and restriction of venous outflow, thereby resulting in an erection (126–129). Later both in vitro and in vivo studies suggest that H2S functions here as a vasodilator mediator, thereby contributing to blood pressure regulation (39, 130–136). H2S is produced in this tissue by both vascular smooth muscle and endothelial cells, on which it has vasodilatory effects (137). Vasodilation is mediated by KATP (138–142), BKCa channels (13, 35, 142), TRPVA1 channels, and through the beta3 adrenoceptor signaling pathway (44, 143). The vasodilatory effect of H2S is probably enhanced by testosterone, which promotes the production of H2S from its precursor, L-cysteine (144), and induces CC smooth muscle relaxation via KATP channels (145). H2S vasodilatory effect has also been demonstrated in vitro using its precursor (L-cysteine), donors (GYY4137), and inhibitors (DL-propargylglycine, aminooxyacetic acid) (53, 146, 147). Inhibition of CTH and CBS leads to contraction of the CC and a reduction in the normal intracavernous pressure response to electrical stimulation (35, 43, 53, 148, 149).
In addition, another gasotransmitter contributes to the erection response. A key mechanism of erection lies in the NO signaling pathway, which stimulates the guanylyl cyclase/cyclic guanosine monophosphate (GC/cGMP) pathway (Figure 6) (128, 150, 151). H2S likely exerts its pro-erectile effects precisely by enhancing the NO signaling pathway (70, 152–155). Indeed, endogenous H2S production has been described to significantly enhance the vasorelaxant effect of the NO donor sodium nitroprusside (13, 138, 153, 156–160), and conversely, the H2S donor NaHS upregulates endothelial NO synthase (eNOS), thereby relaxing and promoting erectile function (161). Conversely, a decrease in H2S levels causes dysregulation of the NO/sGC/cGMP signaling pathway, leading to ED. However, this dysregulation can be reversed by H2S donors (162). The pro-erectile effect of H2S and its donors likely involves the inhibition of PDEs, resulting in the accumulation of cGMP (163–166). Since PDE proteins contain many cysteine residues, H2S is thought to inhibit PDE activity by modifying sulfhydryl sulfides (164). H2S alone can enhance cGMP signaling like a PDE5 inhibitor (PDE5i) (Figure 6) (164). In addition, H2S increased eNOS phosphorylation and xanthine oxidase activity, leading to NO production and increasing NO bioavailability, hence increasing cGMP concentration (166–170). Conversely, NO can modulate endogenous H2S production (171). Indeed, it has been found that NO can not only increase the expression of CTH at the transcriptional level but also increase the activity of this enzyme itself, for example, by NO stimulating the uptake of cysteine, which is a substrate for CTH (172). The role of both gasotransmitters in regulating erectile function is likely to be synergistic (153, 155, 173). Taken together, H2S acts in CC by at least two mechanisms: inhibiting PDE and activating KATP channels modulated by testosterone. However, what can be inferred from the available data is that there is an interplay between H2S and NO/cGMP in CC and that the on/off switching of signaling may be reciprocally regulated and influenced by testosterone (174). These data imply a strong link between aging, testosterone, H2S, and ED (164).
Figure 6. Potential use of H2S in the treatment of ED. (A) NO/cGMP signaling pathway involved in penile erection; (B) Reduction of NO/cGMP signaling pathway activity in patients with ED in whom PDE5i is used as a therapeutic agent, which subsequently preserves the functionality of the signaling pathway; (C) Potential use of H2S in patients with ED who, due to disruption of the endothelium and the NO/cGMP signaling pathway, do not respond adequately to PDE5i treatment.
The occurrence of ED is most often related to a disorder of the blood supply to the penile arteries. The development of ED usually occurs during aging (148) or due to other diseases such as diabetes (37, 175), hypertension (175, 176), or metabolic syndrome (177). PDE5i are widely used to treat ED (178) due to its capability to increase cGMP and/or cAMP levels, leading to activation of PKG/PKA and smooth muscle relaxation in erectile tissues (179–181). Some patients, though, specifically elderly, diabetics, and hypertensives, respond inadequately or not at all to PDE5i (182–184). In addition, they often have impaired H2S and testosterone signaling (37, 42, 148, 185, 186). In patients who do not respond to PDE5i therapy, a possible clinical treatment for ED could be the use of a combination of PDE5i, testosterone, and/or H2S donors/substrates, which could lead to cGMP levels maintenance (35, 40, 43, 154, 157, 177, 186, 187). The advantage of H2S-induced CC relaxation is that it is independent of the endothelium (157, 188, 189), which NO/cGMP pathway is not, and it is one of the reasons why, for example, diabetic patients develop ED (Figure 6) (190–193). As a result, it can be said that H2S has a compensatory role in the absence of NO without altering the downstream mechanisms of the signaling pathway. However, the potential therapeutic use of H2S could lie not only in its acute effect on erection but also in its longer-term effect in reducing oxidative stress in erectile tissue, both of which have been described in another H2S donor – a sildenafil derivative ACS6 (52, 157, 194–196).
Endogenous production of H2S, which plays a crucial role in the processes of male reproduction, has been confirmed in most tissues of the male reproductive system. H2S is essential in the early phase of male fertility, as it maintains the integrity of BTB during spermatogenesis, promotes testosterone production, and has cytoprotective effects. Cytoprotective effects may be used, for example, in the treatment of testicular cancer as a means of mitigating the impact of testicular toxicity induced by anticancer drugs. The vasodilatory effects of H2S and the subsequent improvement in tissue blood flow could support the treatment of chronic prostatitis. Cytoprotective effects may, in turn, help in the treatment of prostate cancer. At the same time, H2S could also aid in the early detection of prostate cancer as a marker for which supraphysiological levels of H2S are typical.
In spermatozoa, H2S both inhibits sperm motility during epididymal maturation and supports its hyperactivation during capacitation. H2S is also involved in the acrosome response of sperm and protects sperm from oxidative stress throughout the reproductive maturation process. These effects are mediated primarily by the action of H2S on mitochondria, ion channels (Ca2+, K+, TRPV), the MAPK signaling pathway, or the persulfidation of sperm proteins. The effect of H2S on sperm motility could be exploited in treating patients with reduced sperm motility, for example, by providing an exogenous H2S donor. The physiological concentration of H2S in the body is relatively low, so donors would be needed for possible treatment that releases H2S in low concentrations, such as GYY4137, SG1002, or NTAs.
The most researched area of this topic is the penile tissue, as H2S can cause or promote pro-erectile effects. The latter through its relaxant effects on the CC, mediated by KATP channels, or by inhibiting PDE or upregulating eNOS, leading to relaxation of CC mediated by the NO/GC/cGMP signaling pathway. The synergy of H2S and NO in penile tissue works both ways, promoting CTH activity through NO. Due to its pro-erectile effects, H2S is a potential therapeutic agent for treating ED patients who do not respond to PDE5i therapy or have impaired endothelial NO production in the CC, such as diabetic patients.
The H2S concentration is a crucial factor in its tissue effects. In previous studies, two main types of H2S donors have been investigated. Namely, some donors release H2S rapidly and in high concentrations (e.g., NaHS) or release H2S slowly in low concentrations (GYY4137). While the second group of donors better mimics the physiological concentrations, it is worthwhile for future research to investigate the physiological effects of H2S using these donors. The first group of donors, such as NaHS, forming supraphysiological levels of H2S in cells may cause tissue or cellular toxicity or promote cancer.
In conclusion, H2S may be a valuable tool in the treatment of many pathological conditions of male reproduction, such as impaired spermatogenesis, lack of sperm motility, or ED. However, for the successful application of H2S in clinical settings, it is important to determine the precise concentrations that elicit the desired therapeutic effects. Furthermore, future research will likely uncover specific protein targets of persulfidation and elucidate how this posttranslational modification influences their function. This understanding will be crucial in identifying and defining critical therapeutic targets, ultimately paving the way for more targeted and effective treatments.
ZP: Writing – review & editing, Writing – original draft, Conceptualization. AP: Writing – review & editing, Writing – original draft, Conceptualization. NZ: Writing – review & editing. BK: Writing – review & editing. EC: Writing – review & editing. PP: Writing – review & editing, Supervision. MS: Writing – review & editing, Supervision.
The author(s) declare financial support was received for the research, authorship, and/or publication of this article. This manuscript was supported by the Internal Grant Agency of the Czech University of Life Sciences in Prague (SV24-21-21230), Czech Science Foundation (GA22-31156S), NAZV (QK21010327), the support of the Institute of Biotechnology (RVO:86652036), and project BIOCEV (CZ.1.05/1.1.00/02.0109) from the ERDF.
A DeepL Translator service was used to translate text into English. BioRender was used to create images and diagrams.
The authors declare that the research was conducted in the absence of any commercial or financial relationships that could be construed as a potential conflict of interest.
All claims expressed in this article are solely those of the authors and do not necessarily represent those of their affiliated organizations, or those of the publisher, the editors and the reviewers. Any product that may be evaluated in this article, or claim that may be made by its manufacturer, is not guaranteed or endorsed by the publisher.
1. Łowicka E, Bełtowski J. Hydrogen sulfide (H2S) – the third gas of interest for pharmacologists. Pharmacol Rep. (2007) 59:4–24.
2. Cavallini D, Mondovi B, de Marco C, Scioscia-Santoro A. The mechanism of desulphhydration of cysteine. Enzymologia. (1962) 24:253–66.
3. Ishigami M, Hiraki K, Umemura K, Ogasawara Y, Ishii K, Kimura H. A source of hydrogen sulfide and a mechanism of its release in the brain. Antioxid Redox Signaling. (2009) 11:205–14. doi: 10.1089/ars.2008.2132
4. Shibuya N, Tanaka M, Yoshida M, Ogasawara Y, Togawa T, Ishii K, et al. 3-mercaptopyruvate sulfurtransferase produces hydrogen sulfide and bound sulfane sulfur in the brain. Antioxid Redox Signaling. (2009) 11:703–14. doi: 10.1089/ars.2008.2253
5. Shibuya N, Mikami Y, Kimura Y, Nagahara N, Kimura H. Vascular endothelium expresses 3-mercaptopyruvate sulfurtransferase and produces hydrogen sulfide. J Biochem. (2009) 146:623–6. doi: 10.1093/jb/mvp111
6. Mikami Y, Shibuya N, Kimura Y, Nagahara N, Ogasawara Y, Kimura H. Thioredoxin and dihydrolipoic acid are required for 3-mercaptopyruvate sulfurtransferase to produce hydrogen sulfide. Biochem J. (2011) 439:479–85. doi: 10.1042/BJ20110841
7. Shibuya N, Koike S, Tanaka M, Ishigami-Yuasa M, Kimura Y, Ogasawara Y, et al. A novel pathway for the production of hydrogen sulfide from D-cysteine in mammalian cells. Nat Commun. (2013) 4:1366. doi: 10.1038/ncomms2371
8. Cheng Y, Ndisang JF, Tang G, Cao K, Wang R. Hydrogen sulfide-induced relaxation of resistance mesenteric artery beds of rats. Am J Physiology-Heart Circulatory Physiol. (2004) 287:H2316–23. doi: 10.1152/ajpheart.00331.2004
9. Tang G, Wu L, Liang W, Wang R. Direct stimulation of K ATP channels by exogenous and endogenous hydrogen sulfide in vascular smooth muscle cells. Mol Pharmacol. (2005) 68:1757–64. doi: 10.1124/mol.105.017467
10. Maeda Y, Aoki Y, Sekiguchi F, Matsunami M, Takahashi T, Nishikawa H, et al. Hyperalgesia induced by spinal and peripheral hydrogen sulfide: Evidence for involvement of Cav3.2 T-type calcium channels. Pain. (2009) 142:127–32. doi: 10.1016/j.pain.2008.12.021
11. Marques-da-Silva D, Samhan-Arias AK, Tiago T, Gutierrez-Merino C. L-type calcium channels and cytochrome b5 reductase are components of protein complexes tightly associated with lipid rafts microdomains of the neuronal plasma membrane. J Proteomics. (2010) 73:1502–10. doi: 10.1016/j.jprot.2010.02.014
12. Telezhkin V, Brazier SP, Cayzac S, Müller CT, Riccardi D, Kemp PJ. Hydrogen sulfide inhibits human BKCa channels. In: Arterial Chemoreceptors. Springer Netherlands, Dordrecht (2009). p. 65–72.
13. Li Y, Zang Y, Fu S, Zhang H, Gao L, Li J. H2S relaxes vas deferens smooth muscle by modulating the large conductance ca2+-activated K+ (BKCa) channels via a redox mechanism. J Sexual Med. (2012) 9:2806–13. doi: 10.1111/j.1743-6109.2012.02879.x
14. Peng Z, Kellenberger S. Hydrogen sulfide upregulates acid-sensing ion channels via the MAPK-erk1/2 signaling pathway. Function. (2021) 2. doi: 10.1093/function/zqab007
15. Petersen LC. The effect of inhibitors on the oxygen kinetics of cytochrome c oxidase. Biochim Biophys Acta (BBA) - Bioenerget. (1977) 460:299–307. doi: 10.1016/0005-2728(77)90216-X
16. Streng T, Axelsson HE, Hedlund P, Andersson DA, Jordt S-E, Bevan S, et al. Distribution and function of the hydrogen sulfide–sensitive TRPA1 ion channel in rat urinary bladder. Eur Urol. (2008) 53:391–400. doi: 10.1016/j.eururo.2007.10.024
17. Whiteman M, Armstrong JS, Chu SH, Jia-Ling S, Wong B-S, Cheung NS, et al. The novel neuromodulator hydrogen sulfide: an endogenous peroxynitrite ‘scavenger’? J Neurochem. (2004) 90:765–8. doi: 10.1111/j.1471-4159.2004.02617.x
18. Mitsuhashi H, Yamashita S, Ikeuchi H, Kuroiwa T, Kaneko Y, Hiromura K, et al. Oxidative stress-dependent conversion of hydrogen sulfide to sulfite by activated neutrophils. Shock. (2005) 24:529–34. doi: 10.1097/01.shk.0000183393.83272.de
19. Whiteman M, Cheung NS, Zhu Y-Z, Chu SH, Siau JL, Wong BS, et al. Hydrogen sulphide: a novel inhibitor of hypochlorous acid-mediated oxidative damage in the brain? Biochem Biophys Res Commun. (2005) 326:794–8. doi: 10.1016/j.bbrc.2004.11.110
20. Geng B, Cui Y, Zhao J, Yu F, Zhu Y, Xu G, et al. Hydrogen sulfide downregulates the aortic l -arginine/nitric oxide pathway in rats. Am J Physiology-Regulatory Integr Comp Physiol. (2007) 293:R1608–18. doi: 10.1152/ajpregu.00207.2006
21. Eto K, Asada T, Arima K, Makifuchi T, Kimura H. Brain hydrogen sulfide is severely decreased in Alzheimer’s disease. Biochem Biophys Res Commun. (2002) 293:1485–8. doi: 10.1016/S0006-291X(02)00422-9
22. Ozluk E, Patel S, Coppola D, Ghali G, Cotelingam JD, Kevil C, et al. Cystathionine gamma-lyase is increased in testicular seminomas, embryonal, and yolk sac tumors. Anticancer Res. (2021) 41:4211–4. doi: 10.21873/anticanres.15225
23. Pei Y, Wu B, Cao Q, Wu L, Yang G. Hydrogen sulfide mediates the anti-survival effect of sulforaphane on human prostate cancer cells. Toxicol Appl Pharmacol. (2011) 257:420–8. doi: 10.1016/j.taap.2011.09.026
24. Guo H, Gai J-W, Wang Y, Jin H-F, Du J-B, Jin J. Characterization of hydrogen sulfide and its synthases, cystathionine β-synthase and cystathionine γ-lyase, in human prostatic tissue and cells. Urology. (2012) 79:483.e1–5. doi: 10.1016/j.urology.2011.10.013
25. Sesterhenn IA, Davis CJ. Pathology of germ cell tumors of the testis. Cancer Control. (2004) 11:374–87. doi: 10.1177/107327480401100605
26. Wang Y-H, Huang J-T, Chen W-L, Wang R-H, Kao M-C, Pan YR, et al. Dysregulation of cystathionine γ-lyase promotes prostate cancer progression and metastasis. EMBO Rep. (2019) 20:e45986. doi: 10.15252/embr.201845986
27. Ahn YJ, Gil Y-G, Lee YJ, Jang H, Lee G-J. A dual-mode colorimetric and SERS detection of hydrogen sulfide in live prostate cancer cells using a silver nanoplate-coated paper assay. Microchem J. (2020) 155. doi: 10.1016/j.microc.2020.104724
28. Kim J-H, Lee Y-J, Ahn Y-J, Kim M, Lee G-J. In situ detection of hydrogen sulfide in 3D-cultured, live prostate cancer cells using a paper-integrated analytical device. Chemosensors. (2022) 10:27. doi: 10.3390/chemosensors10010027
29. Sugiura Y, Kashiba M, Maruyama K, Hoshikawa K, Sasaki R, Saito K, et al. Cadmium exposure alters metabolomics of sulfur-containing amino acids in rat testes. Antioxid Redox Signaling. (2005) 7:781–7. doi: 10.1089/ars.2005.7.781
30. Řimnáčová H, Moravec J, Štiavnická M, Havránková J, Monsef L, Hošek P, et al. Evidence of endogenously produced hydrogen sulfide (H2S) and persulfidation in male reproduction. Sci Rep. (2022) 12:11426. doi: 10.1038/s41598-022-15360-x
31. Wang J, Wang W, Li S, Han Y, Zhang P, Meng G, et al. Hydrogen sulfide as a potential target in preventing spermatogenic failure and testicular dysfunction. Antioxid Redox Signaling. (2018) 28:1447–62. doi: 10.1089/ars.2016.6968
32. Gao D-D, Xu J-W, Qin W-B, Peng L, Qiu Z-E, Wang L-L, et al. Cellular mechanism underlying hydrogen sulfide mediated epithelial K+ Secretion in rat epididymis. Front Physiol. (2019) 9:1886. doi: 10.3389/fphys.2018.01886
33. Li J, Li Y, Du Y, Mou K, Sun H, Zang Y, et al. Endogenous hydrogen sulfide as a mediator of vas deferens smooth muscle relaxation. Fertil Steril. (2011) 95:1833–5. doi: 10.1016/j.fertnstert.2010.11.001
34. Zou S, Shimizu T, Shimizu S, Higashi Y, Nakamura K, Ono H, et al. Possible role of hydrogen sulfide as an endogenous relaxation factor in the rat bladder and prostate. Neurourol Urodynamics. (2018) 37:2519–26. doi: 10.1002/nau.23788
35. d’Emmanuele di Villa Bianca R, Sorrentino R, Maffia P, Mirone V, Imbimbo C, Fusco F, et al. Hydrogen sulfide as a mediator of human corpus cavernosum smooth-muscle relaxation. Proc Natl Acad Sci. (2009) 106:4513–8. doi: 10.1073/pnas.0807974105
36. Huang Y-M, Xia J-Y, Jiang R. Expressions of CSE and CBS in the penile corpus cavernosum of hyperglycemia rats and their implications. Zhonghua Nan Ke Xue. (2014) 20:299–303.
37. Zhang Y, Yang J, Wang T, Wang S-G, Liu J-H, Yin C-P, et al. Decreased endogenous hydrogen sulfide generation in penile tissues of diabetic rats with erectile dysfunction. J Sexual Med. (2016) 13:350–60. doi: 10.1016/j.jsxm.2016.01.002
38. Li G, Xie Z-Z, Chua JMW, Wong PC, Bian J. Hydrogen sulfide protects testicular germ cells against heat-induced injury. Nitric Oxide. (2015) 46:165–71. doi: 10.1016/j.niox.2014.10.005
39. Yang G, Wu L, Jiang B, Yang W, Qi J, Cao K, et al. H 2 S as a physiologic vasorelaxant: hypertension in mice with deletion of cystathionine γ-lyase. Science. (2008) 322:587–90. doi: 10.1126/science.1162667
40. Yetik-Anacak G, Dikmen A, Coletta C, Mitidieri E, Dereli M, Donnarumma E, et al. Hydrogen sulfide compensates nitric oxide deficiency in murine corpus cavernosum. Pharmacol Res. (2016) 113:38–43. doi: 10.1016/j.phrs.2016.08.015
41. Aydinoglu F, Dalkir FT, Demirbag HO, Ogulener N. The interaction of l -cysteine/H 2 S pathway and muscarinic acetylcholine receptors (mAChRs) in mouse corpus cavernosum. Nitric Oxide. (2017) 70:51–8. doi: 10.1016/j.niox.2017.08.005
42. Angulo J, Sevilleja-Ortiz A, Fernández A, Pepe-Cardoso AJ, Martínez-Salamanca JI, Sánchez-Ferrer A, et al. PS-3-1 reduced expression of hydrogen sulfide synthesizing enzymes is associated with the functional impairment of L-cysteine-induced responses in human corpus cavernosum and penile arteries from ED patients. J Sexual Med. (2020) 17:S128–9. doi: 10.1016/j.jsxm.2020.04.034
43. La Fuente JM, Fernández A, Pepe-Cardoso AJ, Martínez-Salamanca JI, Louro N, Angulo J. L-cysteine/hydrogen sulfide pathway induces cGMP-dependent relaxation of corpus cavernosum and penile arteries from patients with erectile dysfunction and improves arterial vasodilation induced by PDE5 inhibition. Eur J Pharmacol. (2019) 863:172675. doi: 10.1016/j.ejphar.2019.172675
44. Mitidieri E, Tramontano T, Gurgone D, Imbimbo C, Mirone V, Fusco F, et al. [amp]]Beta; 3 adrenergic receptor activation relaxes human corpus cavernosum and penile artery through a hydrogen sulfide/cGMP-dependent mechanism. Pharmacol Res. (2017) 124:100–4. doi: 10.1016/j.phrs.2017.07.025
45. Srilatha B, Hu L, Adaikan GP, Moore PK. Initial characterization of hydrogen sulfide effects in female sexual function. J Sexual Med. (2009) 6:1875–84. doi: 10.1111/j.1743-6109.2009.01291.x
46. Pilsova A, Pilsova Z, Klusackova B, Zelenkova N, Chmelikova E, Postlerova P, et al. Hydrogen sulfide and its role in female reproduction. Front Vet Sci. (2024) 11:1378435. doi: 10.3389/fvets.2024.1378435
47. Sun X, Zhang R, Zhong Q, Song Y, Feng X. Regulatory effects of hydrogen sulfide on the female reproductive system. Eur J Pharmacol. (2024) 963:176265. doi: 10.1016/j.ejphar.2023.176265
48. Cirino G, Szabo C, Papapetropoulos A. Physiological roles of hydrogen sulfide in mammalian cells, tissues, and organs. Physiol Rev. (2022) 103:31–276. doi: 10.1152/physrev.00028.2021
49. Pintus E, Jovičić M, Kadlec M, Ros-Santaella JL. Divergent effect of fast- and slow-releasing H2S donors on boar spermatozoa under oxidative stress. Sci Rep. (2020) 10:6508. doi: 10.1038/s41598-020-63489-4
50. Li S, Ma Y, Ye S, Su Y, Hu D, Xiao F. Endogenous hydrogen sulfide counteracts polystyrene nanoplastics-induced mitochondrial apoptosis and excessive autophagy via regulating Nrf2 and PGC-1α signaling pathway in mouse spermatocyte-derived GC-2spd(ts) cells. Food Chem Toxicol. (2022) 164:113071. doi: 10.1016/j.fct.2022.113071
51. Muzaffar S, Shukla N, Bond M, Newby AC, Angelini GD, Sparatore A, et al. Exogenous hydrogen sulfide inhibits superoxide formation, NOX-1 expression and racsub1/sub activity in human vascular smooth muscle cells. J Vasc Res. (2008) 45:521–8. doi: 10.1159/000129686
52. Muzaffar S, Jeremy JY, Sparatore A, Del Soldato P, Angelini GD, Shukla N. H 2 S-donating sildenafil (ACS6) inhibits superoxide formation and gp91 phox expression in arterial endothelial cells: role of protein kinases A and G. Br J Pharmacol. (2008) 155:984–94. doi: 10.1038/bjp.2008.326
53. Srilatha B, Adaikan PG, Moore PK. Possible role for the novel gasotransmitter hydrogen sulphide in erectile dysfunction—a pilot study. Eur J Pharmacol. (2006) 535:280–2. doi: 10.1016/j.ejphar.2006.02.001
54. La Fuente JM, Sevilleja-Ortiz A, García-Rojo E, El Assar M, Fernández A, Pepe-Cardoso AJ, et al. Erectile dysfunction is associated with defective L-cysteine/hydrogen sulfide pathway in human corpus cavernosum and penile arteries. Eur J Pharmacol. (2020) 884:173370. doi: 10.1016/j.ejphar.2020.173370
55. Qi Q, Pan H, Jiang N, Zhang M, Sun S, Wan X, et al. A novel posttranslational modification of histone, H3 S-sulfhydration, is down-regulated in asthenozoospermic sperm. J Assisted Reprod Genet. (2021) 38:3175–93. doi: 10.1007/s10815-021-02314-x
56. Akbarian F, Tavalaee M, Dattilio M, Nasr-Esfahani MH. Down-regulated expression of cystathionine β-synthase and cystathionine γ-lyase in varicocele, and infertile men: A case-control study. Cell J. (2022) 24:176–81. doi: 10.22074/cellj.2022.7775
57. Eto K, Kimura H. The production of hydrogen sulfide is regulated by testosterone and S -adenosyl- l -methionine in mouse brain. J Neurochem. (2002) 83:80–6. doi: 10.1046/j.1471-4159.2002.01097.x
58. Bi Y, Li T, Pan H, Guo M, Chen L, Qi Q, et al. Elemental sulfur upregulated testicular testosterone biosynthesis by associating with altered gut microbiota in mice. Biocell. (2020) 44:301–13. doi: 10.32604/biocell.2020.011208
59. Pintus E, Chinn AF, Kadlec M, García-Vázquez FA, Novy P, Matson JB, et al. N-thiocarboxyanhydrides, amino acid-derived enzyme-activated H2S donors, enhance sperm mitochondrial activity in presence and absence of oxidative stress. BMC Vet Res. (2023) 19:52. doi: 10.1186/s12917-023-03593-5
60. Wiliński B, Wiliński J, Gajda M, Jasek E, Somogyi E, Głowacki M, et al. Sodium hydrosulfide exerts a transitional attenuating effect on spermatozoa migration in vitro. Folia Biol. (2015) 63:145–9. doi: 10.3409/fb63_2.145
61. Wang S, Chen Q, Zhang Y, Zheng F, Xue T, Ge X, et al. Omega-3 polyunsaturated fatty acids alleviate hydrogen sulfide-induced blood-testis barrier disruption in the testes of adult mice. Reprod Toxicol. (2020) 98:233–41. doi: 10.1016/j.reprotox.2020.10.007
62. Wang R. Physiological implications of hydrogen sulfide: A whiff exploration that blossomed. Physiol Rev. (2012) 92:791–896. doi: 10.1152/physrev.00017.2011
63. Almog T, Naor Z. Mitogen activated protein kinases (MAPKs) as regulators of spermatogenesis and spermatozoa functions. Mol Cell Endocrinol. (2008) 282:39–44. doi: 10.1016/j.mce.2007.11.011
64. Kolluru GK, Shen X, Yuan S, Kevil CG. Gasotransmitter heterocellular signaling. Antioxid Redox Signaling. (2017) 26:936–60. doi: 10.1089/ars.2016.6909
65. Singh AP, Rajender S. CatSper channel, sperm function and male fertility. Reprod BioMed Online. (2015) 30:28–38. doi: 10.1016/j.rbmo.2014.09.014
66. Gupta RK, Swain DK, Singh V, Anand M, Choudhury S, Yadav S, et al. Molecular characterization of voltage-gated potassium channel (Kv) and its importance in functional dynamics in bull spermatozoa. Theriogenology. (2018) 114:229–36. doi: 10.1016/j.theriogenology.2018.03.030
67. Kumar A, Mishra AK, Singh V, Yadav S, Saxena A, Garg SK, et al. Molecular and functional insights into Transient Receptor Potential Vanilloid 1 (TRPV1) in bull spermatozoa. Theriogenology. (2019) 128:207–17. doi: 10.1016/j.theriogenology.2019.01.029
68. Claudia C, Horatiu S, Iudith I, Vasile B, Constanta S. Research regarding the role of TRPV1 and capsaicin (CPS) implication for capacitation and acrosome reaction. Romanian Biotechnol Lett. (2014) 19:9437–4441.
69. Mundt N, Spehr M, Lishko PV. TRPV4 is the temperature-sensitive ion channel of human sperm. eLife. (2018) 7:e35853. doi: 10.7554/eLife.35853
70. Zhao H, Jiang R. Relaxation mechanism of smooth muscle cells and its relationship with penile erection. Zhonghua Nan Ke Xue. (2016) 22:838–42.
71. Bertoldo MJ, Faure M, Dupont J, Froment P. AMPK: a master energy regulator for gonadal function. Front Neurosci. (2015) 9:235. doi: 10.3389/fnins.2015.00235
72. Kumar L, Yadav SK, Kushwaha B, Pandey A, Sharma V, Verma V, et al. Energy utilization for survival and fertilization—Parsimonious quiescent sperm turn extravagant on motility activation in rat1. Biol Reproduction. (2016) 94:96. doi: 10.1095/biolreprod.115.137752
73. Swegen A, Lambourne SR, Aitken RJ, Gibb Z. Rosiglitazone improves stallion sperm motility, ATP content, and mitochondrial function. Biol Reproduction. (2016) 95:107–7. doi: 10.1095/biolreprod.116.142687
74. Calle-Guisado V, de Llera AH, Martin-Hidalgo D, Mijares J, Gil MC, Alvarez IS, et al. AMP-activated kinase in human spermatozoa: identification, intracellular localization, and key function in the regulation of sperm motility. Asian J Androl. (2017) 19:707–14. doi: 10.4103/1008-682X.185848
75. Shabani Nashtaei M, Amidi F, Sedighi Gilani MA, Aleyasin A, Bakhshalizadeh S, Naji M, et al. Protective features of resveratrol on human spermatozoa cryopreservation may be mediated through 5’ AMP-activated protein kinase activation. Andrology. (2017) 5:313–26. doi: 10.1111/andr.12306
76. Gangwar DK, Atreja SK. Signalling events and associated pathways related to the mammalian sperm capacitation. Reprod Domest Animals. (2015) 50:705–11. doi: 10.1111/rda.12541
77. Silva JV, Freitas MJ, Correia BR, Korrodi-Gregório L, Patrício A, Pelech S, et al. Profiling signaling proteins in human spermatozoa: biomarker identification for sperm quality evaluation. Fertil Steril. (2015) 104:845–856.e8. doi: 10.1016/j.fertnstert.2015.06.039
78. Martinez AM, Sordia-Hernández LH, Morales JA, Merino M, Vidal O, Garza MRG, et al. A randomized clinical study assessing the effects of the antioxidants, resveratrol or SG1002, a hydrogen sulfide prodrug, on idiopathic oligoasthenozoospermia. Asian Pac J Reproduction. (2015) 4:106–11. doi: 10.1016/S2305-0500(15)30005-1
79. Corsello T, Komaravelli N, Casola A. Role of hydrogen sulfide in NRF2- and sirtuin-dependent maintenance of cellular redox balance. Antioxidants. (2018) 7:129. doi: 10.3390/antiox7100129
80. Matsuura K, Huang H-W, Chen M-C, Chen Y, Cheng C-M. Relationship between porcine sperm motility and sperm enzymatic activity using paper-based devices. Sci Rep. (2017) 7. doi: 10.1038/srep46213
81. Yang C-T, Devarie-Baez NO, Hamsath A, Fu X-D, Xian M. S-persulfidation: chemistry, chemical biology, and significance in health and disease. Antioxid Redox Signaling. (2020) 33:1092–114. doi: 10.1089/ars.2019.7889
82. Mustafa AK, Gadalla MM, Sen N, Kim S, Mu W, Gazi SK, et al. H2S signals through protein S-sulfhydration. Sci Signaling. (2009) 2:ra72. doi: 10.1126/scisignal.2000464
83. Filipovic MR, Zivanovic J, Alvarez B, Banerjee R. Chemical biology of H2S signaling through persulfidation. Chem Rev. (2018) 118:1253–337. doi: 10.1021/acs.chemrev.7b00205
84. Zivanovic J, Kouroussis E, Kohl JB, Adhikari B, Bursac B, Schott-Roux S, et al. Selective persulfide detection reveals evolutionarily conserved antiaging effects of S-sulfhydration. Cell Metab. (2019) 30:1–19. doi: 10.1016/j.cmet.2019.10.007
85. Szabo C, Ransy C, Módis K, Andriamihaja M, Murghes B, Coletta C, et al. Regulation of mitochondrial bioenergetic function by hydrogen sulfide. Part I. Biochemical and physiological mechanisms. Br J Pharmacol. (2014) 171:2099–122. doi: 10.1111/bph.12369
86. Li L, Salto-Tellez M, Tan C-H, Whiteman M, Moore PK. GYY4137, a novel hydrogen sulfide-releasing molecule, protects against endotoxic shock in the rat. Free Radical Biol Med. (2009) 47:103–13. doi: 10.1016/j.freeradbiomed.2009.04.014
87. Lobb I, Zhu J, Liu W, Haig A, Lan Z, Sener A. Hydrogen sulfide treatment improves long-term renal dysfunction resulting from prolonged warm renal ischemia-reperfusion injury. Can Urol Assoc J. (2014) 8:413–8. doi: 10.5489/cuaj.1694
88. Ning JZ, Li W, Cheng F, Rao T, Yu WM, Ruan Y, et al. The protective effects of GYY4137 on ipsilateral testicular injury in experimentally varicocele-induced rats. Exp Ther Med. (2018) 15:433–9. doi: 10.3892/etm.2017.5417
89. Ning J-Z, Li W, Cheng F, Rao T, Yu W-M, Ruan Y, et al. The protective effects of GYY4137 on testicular torsion/detorsion injury in rats. Int J Clin Exp Med. (2018) 11:3387–95.
90. Bozkurt M, Degirmentepe RB, Polat EC, Yildirim F, Sonmez K, Cekmen M, et al. Protective effect of hydrogen sulfide on experimental testicular ischemia reperfusion in rats. J Pediatr Urol. (2020) 16:40.e1–8. doi: 10.1016/j.jpurol.2019.10.006
91. Özatik FY, Özatik O, Tekşen Y, Yiğitaslan S, Ari NS. Protective and therapeutic effect of hydrogen sulfide on hemorrhagic cystitis and testis dysfunction induced with cyclophosphamide. Turkish J Med Sci. (2021) 51:1530–42. doi: 10.3906/sag-2003-10
92. Yuksel S, Erginel B, Bingul I, Ozluk Y, Karatay H, Aydın F, et al. The effect of hydrogen sulfide on ischemia/reperfusion injury in an experimental testicular torsion model. J Pediatr Urol. (2022) 18:16.e1–7. doi: 10.1016/j.jpurol.2021.11.019
93. Mao Z, Li H, Zhao X-L, Zeng X-H. Hydrogen sulfide protects Sertoli cells against toxicant Acrolein-induced cell injury. Food Chem Toxicol. (2023) 176:113784. doi: 10.1016/j.fct.2023.113784
94. Iuchi Y, Kaneko T, Matsuki S, Ishii T, Ikeda Y, Uchida K, et al. Carbonyl stress and detoxification ability in the male genital tract and testis of rats. Histochem Cell Biol. (2004) 121:123–30. doi: 10.1007/s00418-003-0607-3
95. Liu F, Li X-L, Lin T, He D-W, Wei G-H, Liu J-H, et al. The cyclophosphamide metabolite, acrolein, induces cytoskeletal changes and oxidative stress in Sertoli cells. Mol Biol Rep. (2012) 39:493–500. doi: 10.1007/s11033-011-0763-9
96. Hall SE, Aitken RJ, Nixon B, Smith ND, Gibb Z. Electrophilic aldehyde products of lipid peroxidation selectively adduct to heat shock protein 90 and arylsulfatase A in stallion spermatozoa. Biol Reproduction. (2017) 96:107–21. doi: 10.1095/biolreprod.116.145292
97. Ansari MA, Khan FB, Safdari HA, Almatroudi A, Alzohairy MA, Safdari M, et al. Prospective therapeutic potential of Tanshinone IIA: An updated overview. Pharmacol Res. (2021) 164:105364. doi: 10.1016/j.phrs.2020.105364
98. Durairajanayagam D, Agarwal A, Ong C. Causes, effects and molecular mechanisms of testicular heat stress. Reprod BioMed Online. (2015) 30:14–27. doi: 10.1016/j.rbmo.2014.09.018
99. Zhang X-G, Hong J-Y, Yan G-J, Wang Y-F, Li Q-W, Hu J-H. Association of heat shock protein 70 with motility of frozen-thawed sperm in bulls. Czech J Anim Sci. (2015) 60:256–62. doi: 10.17221/8239-CJAS
100. Erata GÖ, Koçak Toker N, Durlanık Ö, Kadıoğlu A, Aktan G, Aykaç Toker G. The role of heat shock protein 70 (Hsp 70) in male infertility: is it a line of defense against sperm DNA fragmentation? Fertil Steril. (2008) 90:322–7. doi: 10.1016/j.fertnstert.2007.06.021
101. Xia Y, Ning J, Cheng F, Yu W, Rao T, Ruan Y, et al. GYY4137 a H2S donor, attenuates ipsilateral epididymis injury in experimentally varicocele-induced rats via activation of the PI3K/Akt pathway. Iran J Basic Med Sci. (2019) 22:729–35. doi: 10.22038/ijbms.2019.30588.7372
102. Azarbarz N, Shafiei Seifabadi Z, Moaiedi MZ, Mansouri E. Assessment of the effect of sodium hydrogen sulfide (hydrogen sulfide donor) on cisplatin-induced testicular toxicity in rats. Environ Sci pollut Res. (2020) 27:8119–28. doi: 10.1007/s11356-019-07266-5
103. Abdel Moneim AE, Othman MS, Aref AM. Azadirachta indica attenuates cisplatin-induced nephrotoxicity and oxidative stress. BioMed Res Int. (2014) 2014:1–11. doi: 10.1155/2014/647131
104. Braun O, Coquery C, Kieffer J, Blondel F, Favero C, Besset C, et al. Spotlight on the life cycle of acrylamide-based polymers supporting reductions in environmental footprint: review and recent advances. Molecules. (2022) 27:42. doi: 10.3390/molecules27010042
105. Lee B-M, Kwon S, Cho YM, Kim K-B, Seo K, Min CS, et al. Perspectives on trace chemical safety and chemophobia: risk communication and risk management. J Toxicol Environ Health Part A. (2019) 82:186–99. doi: 10.1080/15287394.2019.1575625
106. Paul V, Ezekiel R, Pandey R. Acrylamide in processed potato products: progress made and present status. Acta Physiol Plant. (2016) 38:276. doi: 10.1007/s11738-016-2290-8
107. Mokhlis HA, Rashed MH, Saleh IG, Eldeib MG, El-Husseiny AA, Khidr EG, et al. Hydrogen sulfide alleviates acrylamide-induced testicular toxicity in male rats. Toxicol Environ Health Sci. (2023) 15:41–51. doi: 10.1007/s13530-022-00156-3
108. Ryter SW, Alam J, Choi AMK. Heme oxygenase-1/carbon monoxide: from basic science to therapeutic applications. Physiol Rev. (2006) 86:583–650. doi: 10.1152/physrev.00011.2005
109. Sandher RK, Aning J. Diagnosing and managing androgen deficiency in men. Practitioner. (2017) 261:19–22.
110. Wang J, Wang J, Shen T, Hong R, Tang S, Zhao X. H2S catalysed by CBS regulates testosterone synthesis through affecting the sulfhydrylation of PDE. J Cell Mol Med. (2021) 25:3460–8. doi: 10.1111/jcmm.16428
111. Oi Y, Imafuku M, Shishido C, Iwai K, Kominato Y, Nishimura S. Garlic supplementation increases testicular testosterone and decreases plasma corticosterone in rats fed a high protein diet. J Nutr. (2001) 131:2150–6. doi: 10.1093/jn/131.8.2150
112. Hinton BT, Palladino MA. Epididymal epithelium: Its contribution to the formation of a luminal fluid microenvironment. Microscopy Res Technique. (1995) 30:67–81. doi: 10.1002/jemt.1070300106
113. Sen U, Sathnur PB, Kundu S, Givvimani S, Coley DM, Mishra PK, et al. Increased endogenous H 2 S generation by CBS, CSE, and 3MST gene therapy improves ex vivo renovascular relaxation in hyperhomocysteinemia. Am J Physiology-Cell Physiol. (2012) 303:C41–51. doi: 10.1152/ajpcell.00398.2011
114. Zhao K, Li S, Wu L, Lai C, Yang G. Hydrogen sulfide represses androgen receptor transactivation by targeting at the second zinc finger module. J Biol Chem. (2014) 289:20824–35. doi: 10.1074/jbc.M114.559518
115. Bray F, Ferlay J, Soerjomataram I, Siegel RL, Torre LA, Jemal A. Global cancer statistics 2018: GLOBOCAN estimates of incidence and mortality worldwide for 36 cancers in 185 countries. CA: A Cancer J Clin. (2018) 68:394–424. doi: 10.3322/caac.21492
116. Amirov RZ, Karpukhin VT, Nesterov NI. Changes in the state of the blood supply to the prostate gland in patients with chronic prostatitis under the influence of treatment with hydrogen sulfide water (according to rheovasographic findings). Vopr Kurortol Fizioter Lech Fiz Kult. (1976) 1:69–72.
117. Wu D, Si W, Wang M, Lv S, Ji A, Li Y. Hydrogen sulfide in cancer: Friend or foe? Nitric Oxide. (2015) 50:38–45. doi: 10.1016/j.niox.2015.08.004
118. Arunkumar A, Vijayababu MR, Srinivasan N, Aruldhas MM, Arunakaran J. Garlic compound, diallyl disulfide induces cell cycle arrest in prostate cancer cell line PC-3. Mol Cell Biochem. (2006) 288:107–13. doi: 10.1007/s11010-006-9126-6
119. Shankar S, Chen Q, Ganapathy S, Singh KP, Srivastava RK. Diallyl trisulfide increases the effectiveness of TRAIL and inhibits prostate cancer growth in an orthotopic model: molecular mechanisms. Mol Cancer Ther. (2008) 7:2328–38. doi: 10.1158/1535-7163.MCT-08-0216
120. Singh SV, Warin R, Xiao D, Powolny AA, Stan SD, Arlotti JA, et al. Sulforaphane inhibits prostate carcinogenesis and pulmonary metastasis in TRAMP mice in association with increased cytotoxicity of natural killer cells. Cancer Res. (2009) 69:2117–25. doi: 10.1158/0008-5472.CAN-08-3502
121. Arunkumar R, Sharmila G, Elumalai P, Senthilkumar K, Banudevi S, Gunadharini DN, et al. Effect of diallyl disulfide on insulin-like growth factor signaling molecules involved in cell survival and proliferation of human prostate cancer cells in vitro and in silico approach through docking analysis. Phytomedicine. (2012) 19:912–23. doi: 10.1016/j.phymed.2012.04.009
122. Chen M, Li B, Zhao X, Zuo H, He X, Li Z, et al. Effect of diallyl trisulfide derivatives on the induction of apoptosis in human prostate cancer PC-3 cells. Mol Cell Biochem. (2012) 363:75–84. doi: 10.1007/s11010-011-1159-9
123. Borkowska A, Knap N, Antosiewicz J. Diallyl Trisulfide Is More Cytotoxic to Prostate Cancer Cells PC-3 than to Noncancerous Epithelial Cell Line PNT1A: A Possible Role of p66Shc signaling Axis. Nutr Cancer. (2013) 65:711–7. doi: 10.1080/01635581.2013.789115
124. Traka MH, Melchini A, Mithen RF. Sulforaphane and prostate cancer interception. Drug Discovery Today. (2014) 19:1488–92. doi: 10.1016/j.drudis.2014.07.007
125. Duan F, Li Y, Chen L, Zhou X, Chen J, Chen H, et al. Sulfur inhibits the growth of androgen-independent prostate cancer in vivo. Oncology. Letters. (2015) 9:437–41. doi: 10.3892/ol.2014.2700
126. Adaikan PG, Lau LC, Ng SC, Ratnam SS. Physio-pharmacology of human penile erection: autonomic/nitrergic neurotransmissions and receptors of the human corpus cavernosum. Asia Pac J Pharmacol. (1991) 6:213–27.
127. Burnett AL. Role of nitric oxide in the physiology of erection. Biol Reproduction. (1995) 52:485–9. doi: 10.1095/biolreprod52.3.485
128. Burnett AL. Novel nitric oxide signaling mechanisms regulate the erectile response. Int J Impotence Res. (2004) 16:S15–9. doi: 10.1038/sj.ijir.3901209
129. Khan MA, Morgan RJ, Mikhailidis DP. The molecular basis of penile erection. Curr Med Res Opin. (2008) 16:s21–30. doi: 10.1185/0300799009117036
130. Ali MY, Ping CY, Mok Y-YP, Ling L, Whiteman M, Bhatia M, et al. Regulation of vascular nitric oxide in vitro and in vivo; a new role for endogenous hydrogen sulphide? Br J Pharmacol. (2006) 149:625–34. doi: 10.1038/sj.bjp.0706906
131. Ondrias K, Stasko A, Cacanyiova S, Sulova Z, Krizanova O, Kristek F, et al. H2S and HS– donor NaHS releases nitric oxide from nitrosothiols, metal nitrosyl complex, brain homogenate and murine L1210 leukaemia cells. Pflügers Archiv - Eur J Physiol. (2008) 457:271–9. doi: 10.1007/s00424-008-0519-0
132. Bertova A, Cacanyiova S, Kristek F, Krizanova O, Ondrias K, Tomaskova Z. The hypothesis of the main role of H2S in coupled sulphide-nitroso signalling pathway. Gen Physiol Biophys. (2010) 29:402–10. doi: 10.4149/gpb_2010_04_402
133. Kimura H. Hydrogen sulfide: its production, release and functions. Amino Acids. (2011) 41:113–21. doi: 10.1007/s00726-010-0510-x
134. Tomaskova Z, Bertova A, Ondrias K. On the involvement of H2S in nitroso signaling and other mechanisms of H2S action. Curr Pharm Biotechnol. (2011) 12:1394–405. doi: 10.2174/138920111798281009
135. Meng G, Ma Y, Xie L, Ferro A, Ji Y. Emerging role of hydrogen sulfide in hypertension and related cardiovascular diseases. Br J Pharmacol. (2015) 172:5501–11. doi: 10.1111/bph.12900
136. Dugbartey GJ. H2S as a possible therapeutic alternative for the treatment of hypertensive kidney injury. Nitric Oxide. (2017) 64:52–60. doi: 10.1016/j.niox.2017.01.002
137. Bełtowski J, Jamroz-Wiśniewska A. Hydrogen sulfide and endothelium-dependent vasorelaxation. Molecules. (2014) 19:21183–99. doi: 10.3390/molecules191221183
138. Zhao W. The vasorelaxant effect of H2S as a novel endogenous gaseous KATP channel opener. EMBO J. (2001) 20:6008–16. doi: 10.1093/emboj/20.21.6008
139. Brayden JE. Functional roles of K ATP channels in vascular smooth muscle. Clin Exp Pharmacol Physiol. (2002) 29:312–6. doi: 10.1046/j.1440-1681.2002.03650.x
140. Siebert N, Cantré D, Eipel C, Vollmar B. H 2 S contributes to the hepatic arterial buffer response and mediates vasorelaxation of the hepatic artery via activation of K ATP channels. Am J Physiology-Gastrointestinal Liver Physiol. (2008) 295:G1266–73. doi: 10.1152/ajpgi.90484.2008
141. Wang R. Signaling pathways for the vascular effects of hydrogen sulfide. Curr Opin Nephrol Hypertension. (2011) 20:107–12. doi: 10.1097/MNH.0b013e3283430651
142. Gai J-W, Wahafu W, Guo H, Liu M, Wang X-C, Xiao Y-X, et al. Further evidence of endogenous hydrogen sulphide as a mediator of relaxation in human and rat bladder. Asian J Androl. (2013) 15:692–6. doi: 10.1038/aja.2013.32
143. Jung JH, Kim BJ, Chae MR, Kam SC, Jeon J-H, So I, et al. Gene transfer of TRPC6DN (Dominant negative) restores erectile function in diabetic rats. J Sexual Med. (2010) 7:1126–38. doi: 10.1111/j.1743-6109.2009.01634.x
144. Bucci M, Mirone V, Di Lorenzo A, Vellecco V, Roviezzo F, Brancaleone V, et al. Hydrogen sulphide is involved in testosterone vascular effect. Eur Urol. (2009) 56:378–84. doi: 10.1016/j.eururo.2008.05.014
145. Yildiz O, Seyrek M, Irkilata HC, Yildirim I, Tahmaz L, Dayanc M. Testosterone might cause relaxation of human corpus cavernosum by potassium channel opening action. Urology. (2009) 74:229–32. doi: 10.1016/j.urology.2008.12.022
146. Srilatha B, Adaikan PG, Li L, Moore PK. Hydrogen sulphide: A novel endogenous gasotransmitter facilitates erectile function. J Sexual Med. (2007) 4:1304–11. doi: 10.1111/j.1743-6109.2007.00561.x
147. Aydinoglu F, Ogulener N. Characterization of relaxant mechanism of H 2 S in mouse corpus cavernosum. Clin Exp Pharmacol Physiol. (2016) 43:503–11. doi: 10.1111/1440-1681.12554
148. Srilatha B, Muthulakshmi P, Adaikan PG, Moore PK. Endogenous hydrogen sulfide insufficiency as a predictor of sexual dysfunction in aging rats. Aging Male. (2011) 15:153–8. doi: 10.3109/13685538.2012.668722
149. Aydinoglu F, Adıbelli EÖ, Yılmaz-Oral D, Ogulener N. Involvement of RhoA/Rho-kinase in l-cysteine/H2S pathway-induced inhibition of agonist-mediated corpus cavernosal smooth muscle contraction. Nitric Oxide. (2019) 85:54–60. doi: 10.1016/j.niox.2019.02.001
150. Hedlund P, Aszódi A, Pfeifer A, Alm P, Hofmann F, Ahmad M, et al. Erectile dysfunction in cyclic GMP-dependent kinase I-deficient mice. Proc Natl Acad Sci. (2000) 97:2349–54. doi: 10.1073/pnas.030419997
151. Andersson K-E, Michel MC. Mechanisms of penile erection and basis for pharmacological treatment of erectile dysfunction. Pharmacol Rev. (2011) 63:811–59. doi: 10.1124/pr.111.004515
152. Gur S, Kadowitz PJ, Trost L, Hellstrom WJG. Optimizing nitric oxide production by time dependent L-arginine administration in isolated human corpus cavernosum. J Urol. (2007) 178:1543–8. doi: 10.1016/j.juro.2007.05.121
153. Meng J, Ganesan Adaikan P, Srilatha B. Hydrogen sulfide promotes nitric oxide production in corpus cavernosum by enhancing expression of endothelial nitric oxide synthase. Int J Impotence Res. (2013) 25:86–90. doi: 10.1038/ijir.2012.39
154. Yilmaz-Oral D, Kaya E, Yilmaz E, Bayatli N, Cengiz T, Ozakca I, et al. PS-04-006 the beneficial effect of hydrogen sulfide donor, sodium hydrosulfide on erectile dysfunction in l-name-induced hypertensive rats. J Sexual Med. (2017) 14:e117–8. doi: 10.1016/j.jsxm.2017.03.103
155. Mostafa T, Rashed L, Nabil N, Abo-sief AF, Mohamed MM, Omar MS. Cavernosal hydrogen sulfide levels are associated with nitric oxide and hemeoxygenase levels in diabetic rats. Int J Impotence Res. (2019) 31:105–10. doi: 10.1038/s41443-018-0084-9
156. Zhao W, Wang R. H 2 S-induced vasorelaxation and underlying cellular and molecular mechanisms. Am J Physiology-Heart Circulatory Physiol. (2002) 283:H474–80. doi: 10.1152/ajpheart.00013.2002
157. Shukla N, Rossoni G, Hotston M, Sparatore A, Del Soldato P, Tazzari V, et al. Effect of hydrogen sulphide-donating sildenafil (ACS6) on erectile function and oxidative stress in rabbit isolated corpus cavernosum and in hypertensive rats. BJU Int. (2009) 103:1522–9. doi: 10.1111/j.1464-410X.2009.08415.x
158. Qiu X, Villalta J, Lin G, Lue TF. Role of hydrogen sulfide in the physiology of penile erection. J Androl. (2012) 33:529–35. doi: 10.2164/jandrol.111.014936
159. King AL, Polhemus DJ, Bhushan S, Otsuka H, Kondo K, Nicholson C, et al. Hydrogen sulfide cytoprotective signaling is endothelial nitric oxide synthase-nitric oxide dependent. Proc Natl Acad Sci. (2014) 111:3182–7. doi: 10.1073/pnas.1321871111
160. Cortese-Krott MM, Fernandez BO, Kelm M, Butler AR, Feelisch M. On the chemical biology of the nitrite/sulfide interaction. Nitric Oxide. (2015) 46:14–24. doi: 10.1016/j.niox.2014.12.009
161. Yilmaz E, Kaya-Sezginer E, Yilmaz-Oral D, Cengiz T, Bayatli N, Gur S. Effects of hydrogen sulphide donor, sodium hydrosulphide treatment on the erectile dysfunction in L-NAME-induced hypertensive rats. Andrologia. (2019) 51:e13240. doi: 10.1111/and.13240
162. Olivencia MA, Esposito E, Brancaleone V, Castaldo S, Cirino G, Pérez-Vizcaino F, et al. Hydrogen sulfide regulates the redox state of soluble guanylate cyclase in CSE-/- mice corpus cavernosum microcirculation. Pharmacol Res. (2023) 194:106834. doi: 10.1016/j.phrs.2023.106834
163. Salloum FN, Chau VQ, Hoke NN, Abbate A, Varma A, Ockaili RA, et al. Phosphodiesterase-5 inhibitor, tadalafil, protects against myocardial ischemia/reperfusion through protein-kinase G–dependent generation of hydrogen sulfide. Circulation. (2009) 120:S31–6. doi: 10.1161/CIRCULATIONAHA.108.843979
164. Bucci M, Papapetropoulos A, Vellecco V, Zhou Z, Pyriochou A, Roussos C, et al. Hydrogen sulfide is an endogenous inhibitor of phosphodiesterase activity. Arteriosclerosis Thrombosis Vasc Biol. (2010) 30:1998–2004. doi: 10.1161/ATVBAHA.110.209783
165. Burgoyne JR, Prysyazhna O, Rudyk O, Eaton P. CGMP-dependent activation of protein kinase G precludes disulfide activation. Hypertension. (2012) 60:1301–8. doi: 10.1161/HYPERTENSIONAHA.112.198754
166. Coletta C, Papapetropoulos A, Erdelyi K, Olah G, Módis K, Panopoulos P, et al. Hydrogen sulfide and nitric oxide are mutually dependent in the regulation of angiogenesis and endothelium-dependent vasorelaxation. Proc Natl Acad Sci. (2012) 109:9161–6. doi: 10.1073/pnas.1202916109
167. Cai W, Wang M, Moore P, Jin H, Yao T, Zhu Y. The novel proangiogenic effect of hydrogen sulfide is dependent on Akt phosphorylation. Cardiovasc Res. (2007) 76:29–40. doi: 10.1016/j.cardiores.2007.05.026
168. Bir SC, Kolluru GK, McCarthy P, Shen X, Pardue S, Pattillo CB, et al. Hydrogen sulfide stimulates ischemic vascular remodeling through nitric oxide synthase and nitrite reduction activity regulating hypoxia-inducible factor-1α and vascular endothelial growth factor–dependent angiogenesis. J Am Heart Assoc. (2012) 1:e004093. doi: 10.1161/JAHA.112.004093
169. Kondo K, Bhushan S, King AL, Prabhu SD, Hamid T, Koenig S, et al. H 2 S protects against pressure overload–induced heart failure via upregulation of endothelial nitric oxide synthase. Circulation. (2013) 127:1116–27. doi: 10.1161/CIRCULATIONAHA.112.000855
170. Altaany Z, Ju YJ, Yang G, Wang R. The coordination of S-sulfhydration, S-nitrosylation, and phosphorylation of endothelial nitric oxide synthase by hydrogen sulfide. Sci Signaling. (2014) 7:342. doi: 10.1126/scisignal.2005478
171. Zhao W, Ndisang JF, Wang R. Modulation of endogenous production of H 2 S in rat tissues. Can J Physiol Pharmacol. (2003) 81:848–53. doi: 10.1139/y03-077
172. Li H, Marshall ZM, Whorton AR. Stimulation of cystine uptake by nitric oxide: regulation of endothelial cell glutathione levels. Am J Physiology-Cell Physiol. (1999) 276:C803–11. doi: 10.1152/ajpcell.1999.276.4.C803
173. Yilmaz-Oral D, Kaya-Sezginer E, Oztekin CV, Bayatli N, Lokman U, Gur S. Evaluation of combined therapeutic effects of hydrogen sulfide donor sodium hydrogen sulfide and phosphodiesterase type-5 inhibitor tadalafil on erectile dysfunction in a partially bladder outlet obstructed rat model. Neurourol Urodynamics. (2020) 39:1087–97. doi: 10.1002/nau.24333
174. d’Emmanuele di Villa Bianca R, Fusco F, Mirone V, Cirino G, Sorrentino R. The role of the hydrogen sulfide pathway in male and female urogenital system in health and disease. Antioxid Redox Signaling. (2017) 27:654–68. doi: 10.1089/ars.2017.7079
175. Jain SK, Bull R, Rains JL, Bass PF, Levine SN, Reddy S, et al. Low levels of hydrogen sulfide in the blood of diabetes patients and streptozotocin-treated rats causes vascular inflammation? Antioxid Redox Signaling. (2010) 12:1333–7. doi: 10.1089/ars.2009.2956
176. Yan H, Du J, Tang C. The possible role of hydrogen sulfide on the pathogenesis of spontaneous hypertension in rats. Biochem Biophys Res Commun. (2004) 313:22–7. doi: 10.1016/j.bbrc.2003.11.081
177. Dayar E, Kara E, Yetik-Anacak G, Hocaoglu N, Bozkurt O, Gidener S, et al. Do penile haemodynamics change in the presence of hydrogen sulphide (H 2 S) donor in metabolic syndrome-induced erectile dysfunction? Andrologia. (2018) 50:e12885. doi: 10.1111/and.12885
178. Vickers MA, Satyanarayana R. Phosphodiesterase type 5 inhibitors for the treatment of erectile dysfunction in patients with diabetes mellitus. Int J Impotence Res. (2002) 14:466–71. doi: 10.1038/sj.ijir.3900910
179. Matsumoto T, Kobayashi T, Kamata K. Phosphodiesterases in the vascular system. J Smooth Muscle Res. (2003) 39:67–86. doi: 10.1540/jsmr.39.67
180. Waldkirch E, Ückert S, Yildirim H, Sohn M, Jonas U, Stief CG, et al. Cyclic AMP-specific and cyclic GMP-specific phosphodiesterase isoenzymes in human cavernous arteries—immunohistochemical distribution and functional significance. World J Urol. (2005) 23:405–10. doi: 10.1007/s00345-005-0026-2
181. Wheeler MA, Ayyagari RR, Wheeler GL, Weiss RM. Regulation of cyclic nucleotides in the urinary tract. J Smooth Muscle Res. (2005) 41:1–21. doi: 10.1540/jsmr.41.1
182. Boulton AJM, Selam J-L, Sweeney M, Ziegler D. Sildenafil citrate for the treatment of erectile dysfunction in men with Type II diabetes mellitus. Diabetologia. (2001) 44:1296–301. doi: 10.1007/s001250100656
183. Briganti A, Salonia A, Deho’ F, Zanni G, Barbieri L, Rigatti P, et al. Clinical update on phosphodiesterase type-5 inhibitors for erectile dysfunction. World J Urol. (2005) 23:374–84. doi: 10.1007/s00345-005-0022-6
184. Carson CC, Lue TF. Phosphodiesterase type 5 inhibitors for erectile dysfunction. BJU Int. (2005) 96:257–80. doi: 10.1111/j.1464-410X.2005.05614.x
185. Brancaleone V, Roviezzo F, Vellecco V, De Gruttola L, Bucci M, Cirino G. Biosynthesis of H 2 S is impaired in non-obese diabetic (NOD) mice. Br J Pharmacol. (2008) 155:673–80. doi: 10.1038/bjp.2008.296
186. Isidori AM, Buvat J, Corona G, Goldstein I, Jannini EA, Lenzi A, et al. A critical analysis of the role of testosterone in erectile function: from pathophysiology to treatment—A systematic review. Eur Urol. (2014) 65:99–112. doi: 10.1016/j.eururo.2013.08.048
187. Qabazard B, Yousif M, Mousa A, Phillips OA. GYY4137 attenuates functional impairment of corpus cavernosum and reduces fibrosis in rats with STZ-induced diabetes by inhibiting the TGF-β1/Smad/CTGF pathway. Biomed Pharmacother. (2021) 138:111486. doi: 10.1016/j.biopha.2021.111486
188. Bivalacqua TJ, Usta MF, Champion HC, Kadowitz PJ, Hellstrom WJG. Endothelial dysfunction in erectile dysfunction: role of the endothelium in erectile physiology and disease. J Androl. (2003) 24:17–37. doi: 10.1002/j.1939-4640.2003.tb02743.x
189. Gur S, Kadowitz PJ, Sikka SC, Peak TC, Hellstrom WJG. Overview of potential molecular targets for hydrogen sulfide: A new strategy for treating erectile dysfunction. Nitric Oxide. (2015) 50:65–78. doi: 10.1016/j.niox.2015.08.005
190. Goldstein I, Lue TF, Padma-Nathan H, Rosen RC, Steers WD, Wicker PA. Oral sildenafil in the treatment of erectile dysfunction. New Engl J Med. (1998) 338:1397–404. doi: 10.1056/NEJM199805143382001
191. Rendell MS, Rajfer J, Wicker PA, Smith MD. Sildenafil for treatment of erectile dysfunction in men with diabetes. JAMA. (1999) 281:421–6. doi: 10.1001/jama.281.5.421
192. Burnett AL. The role of nitric oxide in erectile dysfunction: implications for medical therapy. J Clin Hypertension. (2006) 8:53–62. doi: 10.1111/j.1524-6175.2006.06026.x
193. Palit V, Eardley I. An update on new oral PDE5 inhibitors for the treatment of erectile dysfunction. Nat Rev Urol. (2010) 7:603–9. doi: 10.1038/nrurol.2010.165
194. Azadzoi KM, Schulman RN, Aviram M, Siroky MB. Oxidative stress in arteriogenic erectile dysfunction: prophylactic role of antioxidants. J Urol. (2005) 174:386–93. doi: 10.1097/01.ju.0000161209.39959.67
195. Shukla N, Jones R, Persad R, Angelini GD, Jeremy JY. Effect of sildenafil citrate and a nitric oxide donating sildenafil derivative, NCX 911, on cavernosal relaxation and superoxide formation in hypercholesterolaemic rabbits. Eur J Pharmacol. (2005) 517:224–31. doi: 10.1111/j.1464-410X.2009.08415.x
Keywords: hydrogen sulfide, male reproduction, sperm, testes, erectile function, antioxidant, vasorelaxation
Citation: Pilsova Z, Pilsova A, Zelenkova N, Klusackova B, Chmelikova E, Postlerova P and Sedmikova M (2024) Hydrogen sulfide and its potential as a possible therapeutic agent in male reproduction. Front. Endocrinol. 15:1427069. doi: 10.3389/fendo.2024.1427069
Received: 07 May 2024; Accepted: 21 August 2024;
Published: 11 September 2024.
Edited by:
Martine Culty, University of Southern California, United StatesReviewed by:
Jasmina Zivanovic, University of Belgrade, SerbiaCopyright © 2024 Pilsova, Pilsova, Zelenkova, Klusackova, Chmelikova, Postlerova and Sedmikova. This is an open-access article distributed under the terms of the Creative Commons Attribution License (CC BY). The use, distribution or reproduction in other forums is permitted, provided the original author(s) and the copyright owner(s) are credited and that the original publication in this journal is cited, in accordance with accepted academic practice. No use, distribution or reproduction is permitted which does not comply with these terms.
*Correspondence: Zuzana Pilsova, cGlsc292YXpAYWYuY3p1LmN6 ; Aneta Pilsova, cGlsc292YUBhZi5jenUuY3o=
Disclaimer: All claims expressed in this article are solely those of the authors and do not necessarily represent those of their affiliated organizations, or those of the publisher, the editors and the reviewers. Any product that may be evaluated in this article or claim that may be made by its manufacturer is not guaranteed or endorsed by the publisher.
Research integrity at Frontiers
Learn more about the work of our research integrity team to safeguard the quality of each article we publish.