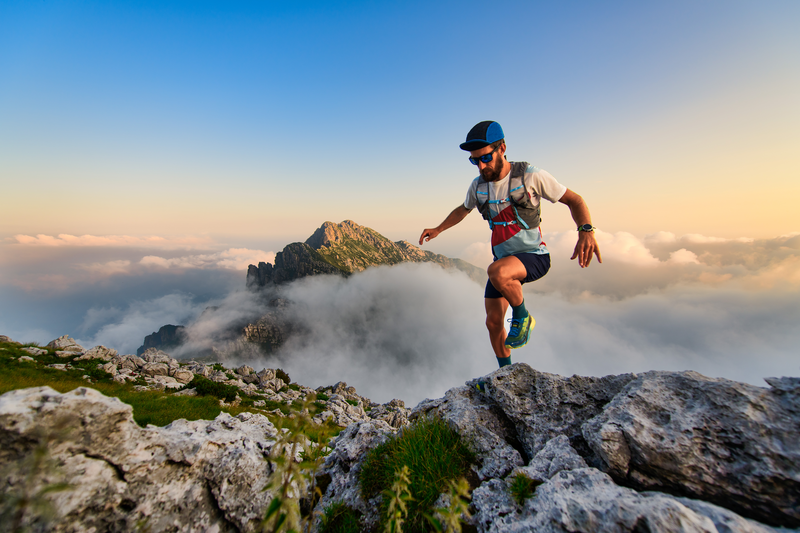
95% of researchers rate our articles as excellent or good
Learn more about the work of our research integrity team to safeguard the quality of each article we publish.
Find out more
ORIGINAL RESEARCH article
Front. Endocrinol. , 22 January 2025
Sec. Thyroid Endocrinology
Volume 15 - 2024 | https://doi.org/10.3389/fendo.2024.1369800
Background: hyperthyroidism characterized by low thyrotropin, highlighting complications and risks, including cardiac issues, osteoporosis, adverse pregnancy outcomes, unintentional weight loss, and increased mortality associated with untreated hyperthyroidism. However, the casual association between telomere length (TL) and hyperthyroidism remains unclear.
Objective: We aim to explore the casual relationship between TL and hyperthyroidism.
Methods: A two-sample bidirectional Mendelian randomization (MR) analysis employed the inverse variance weighted (IVW) method, supplemented by additional approaches such as Weighted Median (WM), and MR Egger.
Results: The summary statistics for TL were derived from the UK Biobank, comprising 472,174 individuals, while the data for hyperthyroidism were sourced from the GWAS Catalog and the FinnGen database, encompassing cohorts of 460,499 and 173,938 individuals, respectively. Utilizing 139 genome-wide significant single nucleotide polymorphisms (SNPs) as instrumental variables (IVs) for TL, forward MR analyses indicated a negative causal effect of TL on hyperthyroidism. The risk of hyperthyroidism decreased as genetically predicted TL increased by one standard deviation, as determined by the IVW form GWAS Catalog (OR:0.659,95%CI: 0.541-0.802, p <0.001) and IVW from FinnGen(OR:0.634, 95%CI: 0.479-0.840, p = 0.001). Other MR methods exhibited a consistent trend in the impact of TL on hyperthyroidism. Reverse MR analysis suggested no causal association between TL and hyperthyroidism (p > 0.05). Sensitivity analyses confirmed the robustness of these results, suggesting minimal susceptibility to confounding factors and bias.
Conclusion: The finding that longer telomeres reduce hyperthyroidism risk highlights the need to validate hyperthyroidism’s impact on telomere length, offering valuable insights for prevention and treatment.
Hyperthyroidism, a prevalent endocrine disorder, affects 0.2-1.3% of the general population (1), with women more affected than men, and increases with age (2). Given the critical role of thyroid hormones in essential physiological processes like growth (3), maturation (4), and metabolism (5), many efforts have the positive association between thyroid function and cancer development (6, 7). Without treatment, hyperthyroidism can lead to serious complications including cardiac arrhythmias (8), congestive heart failure (9), osteoporosis (10), adverse obstetric outcomes (11), and metabolic imbalances (12), such as increased resting energy demand and gluconeogenesis (13). The intricate consequences of hyperthyroidism highlight the importance of understanding its prevalence and the diverse underlying mechanisms, emphasizing the need for effective control and prevention of associated disorders (14).
Hyperthyroidism, characterized by elevated thyroid hormone levels, plays a significant role in the aging process. Age-related changes in thyroid function have important implications for longevity (3). Studies suggest that longevity in vertebrates is positive associated with low metabolic rates and TH levels (15), and thyrotoxicosis in mice has been linked to aging traits like malnutrition and immune senescence (16). Telomeres are indispensable DNA-protein complexes at the ends of chromosomes, crucial for maintaining genomic stability by protecting repeated “TTAGGG” sequences (15). However, with each cell division, telomeres shorten, leading to replicative senescence, genetic instability, and ultimately cell death when critically short (16). Telomere length (TL) has been extensively studied as a biomarker for human aging across various tissues (17), with research linking TL to increased susceptibility to conditions such as cardiovascular disease (18), type 2 diabetes (19), cancers (20), Alzheimer’s disease (21), chronic kidney disease (22), chronic obstructive pulmonary disease (23), and alcohol consumption (24). However, the casual relationship between TL and hyperthyroidism remains underexplored.
Mendelian randomization (MR) as a statistical tool is used in epidemiology to examine causal associations between exposures, biomarkers, or risk factors and outcomes. MR is particularly useful in situations where conducting randomized controlled trials is not feasible or poses ethical dilemmas (17). MR is an excellent approach for mitigating the issues of residual confounding and reverse causality, which are sometimes encountered when studying observational data using alternative approaches (18). Recent research has explored the connections between depression (19), mortality (20), Graves’ diseases (21), multiple sclerosis (22), and TL. However, no MR analysis investigated the causal relationship between TL and hyperthyroidism.
Our study investigates the causal relationship between TL and hyperthyroidism using a two-sample bidirectional Mendelian randomization analysis. Using genome-wide association study (GWAS) data, we present casual evidence that genetically predicted longer TL decreases the risk of developing hyperthyroidism. Then, we validate these findings with an independent dataset from the Finne cohort. These findings underscore the potential of telomere length as a biomarker for hyperthyroidism, offering insights that could inform novel preventive and therapeutic strategies, including personalized treatments.
We perform a conventional bidirectional MR study, using single nucleotide polymorphisms (SNPs) that are strongly linked to the target variable as instrumental variables (IVs). The GWAS datasets were used to assess the probable causal impact of the exposure on the outcomes. Genetic variants are the primary and effective IVs in a MR study. To be considered qualified IVs, they must adhere to three fundamental principles specified in MR theory. Figure 1A depicts the whole flowchart of this MR study, whereas Figure 1B represents the basic MR assumptions and acronyms. Furthermore, the data for exposure and outcomes were obtained from separate and independent samples.
Figure 1. The research design in the bidirectional MR analysis. (A) Schematic illustrating the experimental plan. Red represents the use of forward MR analysis, where TL is used as the predictor and hyperthyroidism is the outcome. The color azure represents the use of reverse MR analysis, where hyperthyroidism is used as the predictor and TL as the result. (B) The three essential assumptions of MR analysis. SNPs refer to single nucleotide polymorphisms, IVs are instrumental variables, TL stands for telomere length, MR represents Mendelian randomization, X denotes the exposure, and Y represents the outcome.
To discover SNPs substantially linked with both TL and hyperthyroidism, we used a strict significance criterion of p < 5 × 10-8. In addition, we used strict criteria to exclude any association between genetic markers, using a 10,000 kilobase aggregation window and putting the r2 threshold at 0.001. Afterwards, each SNP was carefully examined for any departures from basic assumptions ② and ③ by consulting the PhenoScanner database. To evaluate the efficacy of IVs, we calculated the F-statistic for each SNP as well as for the full set. We take the formulas (23, 24) for calculating F-statistic for single SNP and total set. A F-statistic greater than 10 suggests a significant association between the SNP and the observed phenotype.
MR also entails ascertaining the concordance between the exposure SNP and its impact on the same gene, therefore influencing the outcome. We excluded palindromic SNPs to prevent any potential biases caused by strand orientation or allele coding. We removed palindromic SNPs with intermediate allele frequency and standardized the exposure and outcome data. The MR-PRESSO (25) and MR Egger (26) methods were used to mitigate the impact of horizontal pleiotropy. The MR-PRESSO outlier test generated p-values to assess the pleiotropy of each SNP, while the global test determined an overall p-value to quantify horizontal pleiotropy. The SNPs were sorted based on their MR-PRESSO outlier test p-values. Subsequent global testing of MR-PRESSO was conducted on the remaining SNPs after the elimination of each individual SNP. The p-value surpassed 0.05, indicating a lack of statistical significance. The subsequent MR study used the remaining SNPs after excluding pleiotropic SNPs. Then inverse MR analysis has been finished.
All data was obtained from the public available IEU Open GWAS project. After a thorough and careful evaluation, we excluded unnecessary studies and individuals who were not of European descent. Our analysis utilized summary-level data from GWAS that specifically investigated the genetic factors linked to TL. The TL data we primarily relied on came from the UK Biobank (27). More precisely, genetic variations associated with the length of telomeres were obtained from GWAS that included a group of 472,174 people. This group included an almost equal proportion of men (45.8%) and females (54.2%), and all participants were of European descent (28). In the case of hyperthyroidism, SNPs were chosen as IVs from a GWAS dataset obtained from the GWAS Catalog (29) and FinnGen. The sample size of Hyperthyroidism which from the GWAS database consists of 460,499 people of European descent, consisting of 3,557 cases and 456,942 controls. The sample size of Hyperthyroidism which from the FinnGen consists of 173,938 people of European descent, consisting of 962 cases and 172,976 controls. Table 1 presents a concise summary of the results in this investigation.
In order to examine the cause-and-effect connection between TL and hyperthyroidism, we used the IVW random-effects model (30) as our main method. Combining Wald for each SNP within a meta-analysis paradigm was part of this analysis. Additionally, we utilized other two techniques, such as the WM (31), MR Egger (26) to reassure that our results would remain stable. We conducted sensitivity analyses to ensure robustness, including assessments for heterogeneity, pleiotropy, leave-one-out tests, and MR-PRESSO analysis. Heterogeneity was assessed with Cochran’s Q test (32) (< 0.05 considered significant). MR-Egger intercept analysis evaluated directional pleiotropy (p > 0.05 indicates negligible pleiotropy). MR-PRESSO assessed outliers. Leave-one-out tests confirmed stability and reliability of causal relationships. The MR analyses were performed using the TwoSampleMR, mr.raps, forestploter, and MR-PRESSO programs in the R statistical environment, version 4.3.2.
In our forward MR, we originally chose 154 SNPs as IVs to investigate the relationship between TL and hyperthyroidism. PhenoScanner analysis could not find any connections between these SNPs and established confounding factors or outcomes. After removing palindromic SNPs, there were 141 remaining SNPs that were suitable for study. During the MR-PRESSO outlier testing, in the GWAS database, two SNPs, namely rs10774624 and rs2763979, were detected as outliers. In FinnGen, two SNPs, namely rs2306646 and rs762810, were detected as outliers. Post-outlier removal, every single SNP in this revised group had an F-statistic more than 10, thereby leading to a R2 value of 3.72%, with the combined F-statistic reaching 120.
In the reverse MR analysis, we first selected 13 SNPs as instrumental factors for hyperthyroidism, with a specific emphasis on studying hyperthyroidism. The PhenoScanner inquiry, like the TL analysis, discovered no correlation between these SNPs and any confounding variables or outcomes. After eliminating palindromic SNPs, a total of 12 and13 SNPs remained for further study. MR-PRESSO verified the dependability of these SNPs, identifying four SNPs (rs1794280, rs2160215, rs4338740, rs758778) and three SNPs (rs11571297, rsrs11646791, rs1794511) as anomalies. After removing these outliers, every single one of these SNPs had an F-statistic that significantly exceeded 10. The aggregate coefficient of determination (R2) for these SNPs were 8.92 and 0.621, while their cumulative F-statistic amounted to -57625 and 13.12.
A detail list of SNPs that were excluded from the final analysis owing to not satisfying the inclusion criteria can be found in Supplementary Table S1. The complete details of all SNPs included in the final analysis can be found in Supplementary Table S2, which is included in the supplementary materials.
Our forward MR analysis indicates that genetically predicted increases in TL significantly reduce the risk of hyperthyroidism. This causal relationship is consistently supported by three analytical methods—IVW, Egger, and WM—as shown in Figure 2. In the GWAS database, IVW analysis reveals a significant negative association between TL and hyperthyroidism (OR: 0.695, 95% CI: 0.541–0.802, p < 0.001), which is corroborated by Egger (OR: 0.578, 95% CI: 0.406–0.822, p = 0.002) and WM (OR: 0.761, 95% CI: 0.570–1.016, p = 0.063). Similarly, in the FinnGen database, IVW confirms this association (OR: 0.634, 95% CI: 0.479–0.840, p = 0.001), supported by Egger (OR: 0.568, 95% CI: 0.345–0.934, p = 0.027) and WM (OR: 0.551, 95% CI: 0.371–0.820, p = 0.003).
Figure 2. Forest plot of the bidirectional two-samples MR analysis. SNP, single nucleotide polymorphism; OR, odds ratio.
In contrast, reverse MR analysis finds no significant association between hyperthyroidism and TL. In the GWAS database, IVW reports an OR of 0.999 (95% CI: 0.987–1.011, p = 0.844), Egger an OR of 0.997 (95% CI: 0.952–1.044, p = 0.901), and WM an OR of 1.002 (95% CI: 0.989–1.015, p = 0.742). Similarly, in the FinnGen database, IVW suggests a slight association (OR: 0.984, 95% CI: 0.972–0.996, p = 0.009), but Egger (OR: 0.959, 95% CI: 0.946–0.971, p < 0.001) and WM (OR: 0.991, 95% CI: 0.981–1.001, p = 0.105) do not indicate a strong causal link. These findings are summarized in Figure 2.
In our bidirectional MR analysis, leave-one-out evaluations and funnel plots were used to examine the relationship between TL and hyperthyroidism, as shown in Figure 3. The forward MR funnel plot revealed a symmetrical distribution, supported by IVW (p < 0.001, p = 0.001) and MR-Egger (p = 0.002, p = 0.027) analyses. In contrast, the reverse MR funnel plot showed asymmetry, suggesting potential bias, with GWAS IVW (p = 0.844), MR-Egger (p = 0.901), FinnGen IVW (p = 0.009), and MR-Egger (p < 0.001) results confirming this.
Leave-one-out analyses in Figure 3 validated the stability of the results, showing a consistent negative causal link in forward MR and no significant relationship in reverse MR. Scatter plots in Figure 3 further illustrate a significant negative association in forward MR and no correlation in reverse MR, reinforcing the robustness of our findings.
We investigated both the presence of heterogeneity and horizontal pleiotropy, and detailed findings are provided in Table 2. In this study, we explored the association between TL and hyperthyroidism. Utilizing 139 SNPs and 137SNPs as explanatory variables, we accounted for 3.72% and 0.9% of the variation (r2), demonstrating robust F-statistic of 120 and 125.64. Heterogeneity tests, such as MR Egger and IVW from GWAS, resulted in Q values of 189 (p = 0.002) and 190 (p = 0.002) respectively, indicating substantial heterogeneity. In FinnGen, resulted in Q values of 208 (p<0.001) and 209 (p<0.001) respectively, indicating substantial heterogeneity. The assessment of Pleiotropy by MR Egger and MR-PRESSO indicated that the results were statistically significant.
Our reverse MR study, which used hyperthyroidism as the independent variable and included 8 and 10 SNPs, produced the R2 value of 8.92 and 0.621. F-statistic are of -57625 and 13.12. The observed direction exhibited heterogeneity, as shown by Q values of 11.8 (p = 0.067) and 15.306(p = 0.053) for MR Egger, 11.8 (p = 0.108) and 56.025(p<0.001) for IVW. The results in the GWAS database showed a lack of pleiotropic effects. in the FinnGen database, a significant pleiotropic effect was shown.
By two-sample bidirectional MR analyses, we firstly have explored that increased telomere length is associated with decreased risk of hyperthyroidism. An previous investigation (33) suggested an association between genetic variants governing TL and thyroid cancer risk. Our current study expands upon this assertion by leveraging data from larger cohorts than previously employed. Through a comprehensive and focused analysis, we establish a causal relationship between TL and hyperthyroidism. Our findings demonstrated robustness and consistency across various MR methods. Collectively, the result offers compelling evidence supporting a causal connection between shortened telomeres and hyperthyroidism, hinting at distinct underlying mechanisms for the disease. We further validated the causal link between hyperthyroidism and TL, we found no significant association in GWAS (IVW, OR = 0.999, p = 0.844, 95% CI: 0.987-1.011). In FinnGen, we found association between TL and hyperthyroidism (IVW, OR = 0.984, p = 0.009, 95% CI: 0.972-0.996). This suggests a specific correlation between TL and the progression of hyperthyroidism. In our MR study, we incorporated valid IVs from the latest and most extensive GWAS database of TL. Subsequently, we established stringent criteria for IV selection, opting only for TL variants significantly associated with TL measurements and meeting the three core assumptions of MR analysis. Additionally, to mitigate bias in causal estimation, we employed three MR methods, ensuring the validity and consistency of results in sensitivity analyses. Addressing heterogeneity, horizontal pleiotropy, and outliers, our findings suggest that hyperthyroidism may result from shortened TL and a senescent immune system. Conversely, hyperthyroidism appears to have no impact on TL.
Hyperthyroidism itself is not typically considered an age-related disease, but the risk factors and underlying causes can be influenced by age-related factors (34). This is an intricate biological process that may be roughly categorized into replicative senescence, caused by inherent cellular mechanisms such as telomere shortening, and cellular senescence, which can be induced by different stressors such oxidative stress and DNA damage (35). Based on our research, we hypothesize that the process of telomere shortening may play a significant role in causing cellular senescence in hyperthyroidism. This is similar to how telomere-associated driver mutations are linked to rheumatoid arthritis (36), systemic sclerosis (37), and systemic lupus erythematosus (38). Shorter TL could also result from immune senescence (39). Currently, there is a growing recognition of the correlation between telomere and hyperthyroidism. The process of telomere length shortening is accelerated by age (40), and this is likely a component of the modified immune response seen in individuals with hyperthyroidism. To reduce the role of confounding factors, we excluded TL variations in our MR analysis that are related with features that might independently affect the risk of hyperthyroidism. prior research consistently indicates that a shorter TL is associated with an increased risk of developing immune-mediated inflammatory diseases (41, 42). Our results align with these prior studies.
The result indicating a cause-and-effect relationship between premature telomere erosion and hyperthyroidism offers many possibilities. Possible therapeutic strategies may include interventions targeting inadequate telomere maintenance, either on a broad scale or specifically in cells that play a significant role in the development of the illness (43). Replenishing telomere length is a complex process that cannot be easily achieved, since just enhancing the activity of telomerase may raise the likelihood of developing cancer (44). Current clinical studies, such as NCT04110964 (45), are examining this method in different medical diseases. Safe telomerase activation treatment is being investigated in other medical disciplines, such as cardiology, to mitigate the possible danger of increasing endogenous telomerase activity (46). This therapy involves temporarily delivering modified TERT RNA to prevent sustained elevation of telomerase activity (47). Despite encountering difficulties in achieving in vivo targeted distribution, the use of this method in hyperthyroidism may become possible in the future due to potential advancements. Androgens may also normalize telomerase levels in cells from individuals with telomere illness who have heterozygous mutations in the TERT gene (48).
Approaches that enhance general well-being while positively influencing TL promotion exist. Speculatively, these approaches could be further integrated into the clinical management of hyperthyroidism patients (49), incorporating strategies such as exercise (50), stress reduction (51), and mindfulness (52). Our findings indicate that individuals with hyperthyroidism tend to engage in reduced physical activity, likely exacerbated by poor physical health. Thoughtfully increasing exercise could yield multiple benefits, promoting chromosomal telomere length while enhancing fitness and mental health (50). Similarly, stress resulting from challenging life circumstances, like social deprivation, may be alleviated through mindfulness practices (51). Relatively straightforward measures that enhance patient well-being could fundamentally contribute to reducing telomere attrition.
Our research has many limitations. The major investigations of TL and hyperthyroidism GWAS solely involved individuals of European ethnicity. As a result, the generalizability of our findings to other populations is uncertain, emphasizing the need for stratification by various racial groups. Secondly, our MR analysis was confined to summary-level statistics, with individual-level data remaining inaccessible. This limitation restricts our ability to conduct stratified analyses based on specific factors. Thirdly, information on the subtype and severity of hyperthyroidism was not available, preventing the estimation of the relationship between TL and different hyperthyroidism subtypes and severity levels. Fourthly, there may be unidentified confounders influencing the associations between TL and hyperthyroidism that require further investigation. Lastly, it is essential to recognize that telomere length is determined by a combination of genetics, environmental factors, lifestyles, and epigenetic modifications. Hence, it is essential to acknowledge that our findings only partly clarify the causative impact of TL on hyperthyroidism.
The finding that longer telomeres reduce hyperthyroidism risk highlights the need to validate hyperthyroidism’s impact on telomere length, offering valuable insights for prevention and treatment.
The original contributions presented in the study are included in the article/Supplementary Material. Further inquiries can be directed to the corresponding author.
The analyses conducted in this study exclusively relied on publicly accessible summary data, obviating the need for institutional review board approval.
SZ: Conceptualization, Data curation, Formal analysis, Writing – original draft, Writing – review & editing. ZH: Data curation, Methodology, Writing – original draft, Writing – review & editing. QC: Data curation, Methodology, Writing – original draft. XL: Data curation, Formal analysis, Writing – original draft. WW: Data curation, Software, Writing – original draft. FZ: Conceptualization, Methodology, Project administration, Supervision, Writing – original draft, Writing – review & editing.
The author(s) declare that no financial support was received for the research, authorship, and/or publication of this article.
We would like to acknowledge the IEU Open GWAS Project for their efforts in making GWAS data accessible, which greatly contributed to our research.
The authors declare that the research was conducted in the absence of any commercial or financial relationships that could be construed as a potential conflict of interest.
All claims expressed in this article are solely those of the authors and do not necessarily represent those of their affiliated organizations, or those of the publisher, the editors and the reviewers. Any product that may be evaluated in this article, or claim that may be made by its manufacturer, is not guaranteed or endorsed by the publisher.
The Supplementary Material for this article can be found online at: https://www.frontiersin.org/articles/10.3389/fendo.2024.1369800/full#supplementary-material
1. Taylor PN, Albrecht D, Scholz A, Gutierrez-Buey G, Lazarus JH, Dayan CM, et al. Global epidemiology of hyperthyroidism and hypothyroidism. Nat Rev Endocrinol. (2018) 14(5):301–16. doi: 10.1038/nrendo.2018.18
2. Vanderpump MP. The epidemiology of thyroid disease. Br Med Bull. (2011) 99:39–51. doi: 10.1093/bmb/ldr030
3. van Heemst D. The ageing thyroid: implications for longevity and patient care. Nat Rev Endocrinol. (2024) 20:5–15. doi: 10.1038/s41574-023-00911-7
4. Lee J, Park J-W, Kim HI, Park C-B, Cho S-H. Thyroid-gonadal hormonal interplay in zebrafish exposed to sodium perchlorate: Implications for reproductive health. Chemosphere. (2024) 346:140662. doi: 10.1016/j.chemosphere.2023.140662
5. Fenneman AC, Bruinstroop E, Nieuwdorp M, van der Spek AH. amp]]amp; Boelen, A. A comprehensive review of thyroid hormone metabolism in the gut and its clinical implications. Thyroid®. (2022) 33:32–44. doi: 10.1089/thy.2022.0491
6. L’Heureux A, Wieland DR, Weng C-H, Chen Y-H, Lin C-H, Lin T-H, et al. Association between thyroid disorders and colorectal cancer risk in adult patients in Taiwan. JAMA Network Open. (2019) 2:e193755–e193755. doi: 10.1001/jamanetworkopen.2019.3755
7. Petranović Ovčariček P, Verburg FA, Hoffmann M, Iakovou I, Mihailovic J, Vrachimis A, et al. Higher thyroid hormone levels and cancer. Eur J Nucl Med Mol Imaging. (2021) 48:808–21. doi: 10.1007/s00259-020-05018-z
8. Osman F, Franklyn JA, Holder RL, Sheppard MC, Gammage MD. Cardiovascular manifestations of hyperthyroidism before and after antithyroid therapy: a matched case-control study. J Am Coll Cardiol. (2007) 49:71–81. doi: 10.1016/j.jacc.2006.08.042
9. Graettinger JS, Muenster JJ, Selverstone LA. amp]]amp; Campbell, J. A. A correlation of clinical and hemodynamic studies in patients with hyperthyroidism with and without congestive heart failure. J Clin Invest. (1959) 38:1316–27. doi: 10.1172/JCI103906
10. Baliram R, Sun L, Cao J, Li J, Latif R, Huber AK, et al. Hyperthyroid-associated osteoporosis is exacerbated by the loss of TSH signaling. J Clin Invest. (2012) 122:3737–41. doi: 10.1172/JCI63948
11. Cho GJ, Kim S-Y, Lee HC, Lee K-M, Han SW, Oh M-J, et al. Risk of adverse obstetric outcomes and the abnormal growth of offspring in women with a history of thyroid cancer. Thyroid. (2019) 29:879–85. doi: 10.1089/thy.2018.0283
12. ANDRUS EC, MCEACHERN D. Studies on the increased metabolism in hyperthyroidism. Ann Internal Med. (1935) 9:579–85. doi: 10.7326/0003-4819-9-5-579
13. Shulman G, Ladenson P, Wolfe M, Ridgway E, Wolfe R. Substrate cycling between gluconeogenesis and glycolysis in euthyroid, hypothyroid, and hyperthyroid man. J Clin Invest. (1985) 76:757–64. doi: 10.1172/JCI112032
14. Ross DS, Burch HB, Cooper DS, Greenlee MC, Laurberg P, Maia AL, et al. 2016 american thyroid association guidelines for diagnosis and management of hyperthyroidism and other causes of thyrotoxicosis. Thyroid®. (2016) 26:1343–421. doi: 10.1089/thy.2016.0229
15. Gauthier BR, Sola-García A, Cáliz-Molina M, Lorenzo PI, Cobo-Vuilleumier N, Capilla-González V, et al. Thyroid hormones in diabetes, cancer, and aging. Aging Cell. (2020) 19:e13260. doi: 10.1111/acel.13260
16. Feng Q, Xia W, Dai G, Lv J, Yang J, Liu D, et al. The aging features of thyrotoxicosis mice: malnutrition, immunosenescence and lipotoxicity. Front Immunol. (2022) 13:864929. doi: 10.3389/fimmu.2022.864929
17. Sanderson E, Glymour MM, Holmes MV, Kang H, Morrison J, Munafò MR, et al. Mendelian randomization. Nat Rev Methods Primers. (2022) 2:6. doi: 10.1038/s43586-021-00092-5
18. Lai W, Li G, Peng D, Li N, Wang W. Mendelian randomization study reveals the relationship between dietary factors and respiratory diseases. Sci Rep. (2023) 13:22601. doi: 10.1038/s41598-023-50055-x
19. Bojesen SE, Nordestgaard BG, Rode L, Wium-Andersen MK, Ørsted DD. Telomere length and depression: Prospective cohort study and Mendelian randomisation study in 67 306 individuals. Br J Psychiatry. (2017) 210:31–8. doi: 10.1192/bjp.bp.115.178798
20. Rode L, Nordestgaard BG, Bojesen SE. Peripheral blood leukocyte telomere length and mortality among 64 637 individuals from the general population. JNCI: J Natl Cancer Institute. (2015) 107:djv074. doi: 10.1093/jnci/djv074
21. Ye M, Wang Y, Zhan Y. Genetic association of leukocyte telomere length with Graves’ disease in Biobank Japan: A two-sample Mendelian randomization study. Front Immunol. (2022) 13:998102. doi: 10.3389/fimmu.2022.998102
22. Liao Q, He J, Tian F-F, Bi F-F, Huang K. A causal relationship between leukocyte telomere length and multiple sclerosis: A Mendelian randomization study. Front Immunol. (2022) 13:922922. doi: 10.3389/fimmu.2022.922922
23. Pan R, Xiao M, Wu Z, Liu J, Wan L. Associations of genetically predicted circulating levels of cytokines with telomere length: a Mendelian randomization study. Front Immunol. (2023) 14:1276257. doi: 10.3389/fimmu.2023.1276257
24. Bai C, Shen Z, Qiu B, Zhang S. Leukocyte telomere length is associated with increased risk of endometriosis: a bidirectional two-sample Mendelian randomization study. Front Endocrinol. (2023) 14. doi: 10.3389/fendo.2023.1272200
25. Verbanck M, Chen C-Y, Neale B, Do R. Detection of widespread horizontal pleiotropy in causal relationships inferred from Mendelian randomization between complex traits and diseases. Nat Genet. (2018) 50:693–8. doi: 10.1038/s41588-018-0099-7
26. Burgess S, Thompson SG. Interpreting findings from Mendelian randomization using the MR-Egger method. Eur J Epidemiol. (2017) 32:377–89. doi: 10.1007/s10654-017-0255-x
27. Sudlow C, Gallacher J, Allen N, Beral V, Burton P, Danesh J, et al. UK biobank: an open access resource for identifying the causes of a wide range of complex diseases of middle and old age. PloS Med. (2015) 12:e1001779. doi: 10.1371/journal.pmed.1001779
28. Rentsch CT, Garfield V, Mathur R, Eastwood SV, Smeeth L, Chaturvedi N, et al. Sex-specific risks for cardiovascular disease across the glycaemic spectrum: a population-based cohort study using the UK Biobank. Lancet Regional Health–Europe. (2023) 32:100693. doi: 10.1101/2023.03.16.23287310
29. Kurki MI, Karjalainen J, Palta P, Sipilä TP, Kristiansson K, Donner KM, et al. FinnGen provides genetic insights from a well-phenotyped isolated population. Nature. (2023) 613:508–18. doi: 10.1038/s41586-022-05473-8
30. Lee CH, Cook S, Lee JS, Han B. Comparison of two meta-analysis methods: inverse-variance-weighted average and weighted sum of Z-scores. Genomics Inf. (2016) 14:173. doi: 10.5808/GI.2016.14.4.173
31. Brownrigg DR. The weighted median filter. Commun ACM. (1984) 27:807–18. doi: 10.1145/358198.358222
32. Pereira TV, Patsopoulos NA, Salanti G, Ioannidis JP. Critical interpretation of Cochran’s Q test depends on power and prior assumptions about heterogeneity. Res Synth Methods. (2010) 1:149–61. doi: 10.1002/jrsm.v1:2
33. Huang L, Feng X, Yang W, Li X, Zhang K, Feng S, et al. Appraising the effect of potential risk factors on thyroid cancer: A mendelian randomization study. J Clin Endocrinol Metab. (2022) 107:e2783–91. doi: 10.1210/clinem/dgac196
34. Mariotti S, Franceschi C, Cossarizza A, Pinchera A. The aging thyroid. Endocr Rev. (1995) 16:686–715. doi: 10.1210/edrv-16-6-686
35. Schumacher B, Pothof J, Vijg J, Hoeijmakers JH. The central role of DNA damage in the ageing process. Nature. (2021) 592:695–703. doi: 10.1038/s41586-021-03307-7
36. Steer S, Williams FM, Kato B, Gardner JP, Norman PJ, Hall MA, et al. Reduced telomere length in rheumatoid arthritis is independent of disease activity and duration. Ann rheumatic Dis. (2006) 66(4):476–480. doi: 10.1136/ard.2006.059188
37. Adler BL, Boin F, Wolters PJ, Bingham CO, Shah AA, Greider C, et al. Autoantibodies targeting telomere-associated proteins in systemic sclerosis. Ann rheumatic Dis. (2021) 80:912–9. doi: 10.1136/annrheumdis-2020-218918
38. Wang XF, Xu WJ, Wang FF, Leng R, Yang XK, Ling HZ, et al. Telomere length and development of systemic lupus erythematosus: a Mendelian randomization study. Arthritis Rheumatol. (2022) 74:1984–90. doi: 10.1002/art.v74.12
39. Heba A-C, Toupance S, Arnone D, Peyrin-Biroulet L, Benetos A, Ndiaye NC. Telomeres: New players in immune-mediated inflammatory diseases? J Autoimmun. (2021) 123:102699. doi: 10.1016/j.jaut.2021.102699
40. Epel ES, Blackburn EH, Lin J, Dhabhar FS, Adler NE, Morrow JD, et al. Accelerated telomere shortening in response to life stress. Proc Natl Acad Sci. (2004) 101:17312–5. doi: 10.1073/pnas.0407162101
41. Liu X, Yuan J, Liu S, Wang X, Tang M, Meng X, et al. The causal relationship between autoimmune thyroid disorders and telomere length: A Mendelian randomization and colocalization study. Clin Endocrinol. (2024) 100(3):294–303. doi: 10.1111/cen.15004
42. Liu M, Luo P, Liu L, Wei X, Bai X, Li J, et al. Immune-mediated inflammatory diseases and leukocyte telomere length: A Mendelian randomization study. Front Genet. (2023) 14:1129247. doi: 10.3389/fgene.2023.1129247
43. Guo J, Huang X, Dou L, Yan M, Shen T, Tang W, et al. Aging and aging-related diseases: From molecular mechanisms to interventions and treatments. Signal Transduct Targeted Ther. (2022) 7:391. doi: 10.1038/s41392-022-01251-0
44. Shay JW. Role of telomeres and telomerase in aging and cancer. Cancer Discovery. (2016) 6:584–93. doi: 10.1158/2159-8290.CD-16-0062
45. Han J, Luo L, Marcelina O, Kasim V, Wu S. Therapeutic angiogenesis-based strategy for peripheral artery disease. Theranostics. (2022) 12:5015. doi: 10.7150/thno.74785
46. Hoffmann J, Richardson G, Haendeler J, Altschmied J, Andrés V, Spyridopoulos I. Telomerase as a therapeutic target in cardiovascular disease. Arterioscler Thrombosis Vasc Biol. (2021) 41:1047–61. doi: 10.1161/ATVBAHA.120.315695
47. Cao Y, Li H, Deb S, Liu J-P. TERT regulates cell survival independent of telomerase enzymatic activity. Oncogene. (2002) 21:3130–8. doi: 10.1038/sj.onc.1205419
48. Calado RT, Yewdell WT, Wilkerson KL, Regal JA, Kajigaya S, Stratakis CA, et al. Sex hormones, acting on the TERT gene, increase telomerase activity in human primary hematopoietic cells. Blood J Am Soc Hematol. (2009) 114:2236–43. doi: 10.1182/blood-2008-09-178871
49. Umbricht CB, Conrad GT, Clark DP, Westra WH, Smith DC, Zahurak M, et al. Human telomerase reverse transcriptase gene expression and the surgical management of suspicious thyroid tumors. Clin Cancer Res. (2004) 10:5762–8. doi: 10.1158/1078-0432.CCR-03-0389
50. Denham J, O’Brien BJ, Charchar FJ. Telomere length maintenance and cardio-metabolic disease prevention through exercise training. Sports Med. (2016) 46:1213–37. doi: 10.1007/s40279-016-0482-4
51. Mathur MB, Epel E, Kind S, Desai M, Parks CG, Sandler DP, et al. Perceived stress and telomere length: A systematic review, meta-analysis, and methodologic considerations for advancing the field. Brain Behav Immun. (2016) 54:158–69. doi: 10.1016/j.bbi.2016.02.002
Keywords: Mendelian randomization analysis, telomere length, hyperthyroidism, casual effect, GWAS
Citation: Zhu S, Hao Z, Chen Q, Liu X, Wu W and Zhang F (2025) A two-sample bidirectional Mendelian randomization analysis between telomere length and hyperthyroidism. Front. Endocrinol. 15:1369800. doi: 10.3389/fendo.2024.1369800
Received: 13 January 2024; Accepted: 27 December 2024;
Published: 22 January 2025.
Edited by:
Joseph V. Martin, Rutgers University Camden, United StatesReviewed by:
Abdulsamed Kükürt, Kafkas University, TürkiyeCopyright © 2025 Zhu, Hao, Chen, Liu, Wu and Zhang. This is an open-access article distributed under the terms of the Creative Commons Attribution License (CC BY). The use, distribution or reproduction in other forums is permitted, provided the original author(s) and the copyright owner(s) are credited and that the original publication in this journal is cited, in accordance with accepted academic practice. No use, distribution or reproduction is permitted which does not comply with these terms.
*Correspondence: Fang Zhang, emhhbmdmYW5nZjExQDE2My5jb20=
†These authors have contributed equally to this work and share first authorship
Disclaimer: All claims expressed in this article are solely those of the authors and do not necessarily represent those of their affiliated organizations, or those of the publisher, the editors and the reviewers. Any product that may be evaluated in this article or claim that may be made by its manufacturer is not guaranteed or endorsed by the publisher.
Research integrity at Frontiers
Learn more about the work of our research integrity team to safeguard the quality of each article we publish.