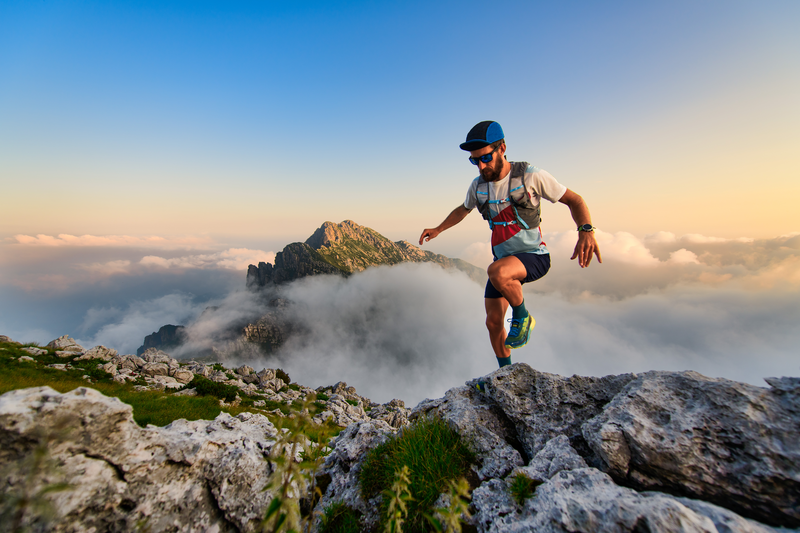
95% of researchers rate our articles as excellent or good
Learn more about the work of our research integrity team to safeguard the quality of each article we publish.
Find out more
ORIGINAL RESEARCH article
Front. Endocrinol. , 08 February 2024
Sec. Cardiovascular Endocrinology
Volume 15 - 2024 | https://doi.org/10.3389/fendo.2024.1362499
This article is part of the Research Topic Molecular biomarkers of cardiometabolic disease View all 10 articles
Objective: The effect of hypolipidemic drugs on male erectile function is still controversial. This Mendelian randomization (MR) study aimed to explore the potential impact of lipid-lowering drug targets on ED.
Methods: We collected seven genetic variants encoding lipid-lowering drug targets (LDLR, HMGCR, NPC1L1, PCSK9, APOB, APOC3 and LPL) from published genome-wide association study (GWAS) statistics, and performed drug target MR analysis. The risk of ED was defined as the primary outcome, sex hormone levels and other diseases as the secondary outcomes. Mediation analyses were performed to explore potential mediating factors.
Results: The results showed that LDLR, LPL agonists and APOC3 inhibitors were significantly associated with a reduced risk of ED occurrence. APOB inhibitors were associated with an increased risk of ED occurrence. In terms of sex hormone levels, LDLR and LPL agonists were significantly associated with increased TT levels, and HMGCR was associated with decreased TT and BT levels significantly. In terms of male-related disease, MR results showed that LDLR agonists and PCSK9 inhibitors were significantly associated with an elevated risk of PH; HMGCR, NPC1L1 inhibitors were associated with a reduced risk of PCa; and LDLR agonists were significantly associated with a reduced risk of AS and MI; in addition, HMGCR inhibitors were associated with a reduced risk of PCa.
Conclusion: After performing drug-targeted MR analysis, we found that that there was a causal relationship between lipid-lowering drug targets and ED. APOC3, APOB, LDLR and LPL may be new candidate drug targets for the treatment of ED.
Erectile dysfunction (ED), defined as the persistent inability of the penis to achieve and/or maintain an erection sufficient for a satisfactory sex life, is one of the most common diseases in urology (1). Although ED does not pose a threat to life, it poses a significant safety hazard to society. It not only affects the physical and mental health of patients, but also causes great distress to sexual partners, leading to a decrease in the quality of life for patients and their partners, disharmony in the family, and more seriously, a decrease in work productivity, an increase in domestic violence, and an increase in medical burden. ED is highly related to cardiovascular risk factors such as hyperlipidemia, diabetes and abnormal blood pressure. Previous studies have found that the pathogenesis of ED and cardiovascular disease is basically the same, both centered on vascular endothelial dysfunction, ultimately leading to vascular atherosclerosis (2–4). Therefore, ED and cardiovascular disease share common risk factors. Lipids, including total cholesterol (TC), triglycerides (TG), low-density lipoprotein (LDL), and high-density lipoprotein (HDL), play a crucial role in this process.
Nicotinic acid, statins, fibrates and novel Lipid Lowering drugs are commonly used in the treatment of hyperlipidemia (5–8). There is clinical evidence that lipid-lowering drug therapy can significantly improve erectile function in patients with organic ED caused by hyperlipidemia (9, 10). However, some scholars have found that patients with hyperlipidemia may experience a decrease in testosterone levels during the use of lipid-lowering drugs, which in turn can lead to the occurrence of ED. Several meta-analyses have also shown similar conclusions (11, 12). In addition, some studies suggest that statins may indirectly lead to the occurrence of ED by affecting autonomic nervous function or psychological factors (13). Randomized controlled trials (RCTs) are the standard methods for determining drug efficacy and adverse reactions. However, there is currently a lack of large-scale randomized controlled trials between lipid-lowering drugs and ED. The impact of lipid-lowering drugs on the occurrence of ED and sex hormone levels is still unclear, and further exploration is needed.
With the increasing popularity of genome-wide association studies (GWAS), Mendelian randomization (MR) may be an effective alternative to RCT studies for problem solving. Because genetic variants (alleles) are randomly assigned during meiosis, participants in MR studies are “randomized” based on the presence of alleles. This is similar to a randomized controlled trial, where participants are randomly assigned to either an experimental treatment group or a control group (14, 15). Thus, MR analysis has the advantage of being less susceptible to confounding factors than other research methods. In recent years, drug target MR analysis has emerged as an effective tool. It is used to infer the effect of drugs targeting protein-coding genes, antagonists, agonists, or inhibitors on disease risk (16). This tool is very helpful in deciphering the potential of drug therapy and facilitating drug development.
In this study, we performed drug-targeted MR analysis to determine the effect of lipid-lowering drugs on ED and to explore the potential impact of lipid-lowering drug targets on sex hormone levels and other common male disorders.
To explore the relationship between lipid-lowering drug target genes and male reproductive health at the genetic level, a two-sample Mendelian randomization approach was used in this study.
Based on the latest guidelines for the treatment of dyslipidemia, we selected common lipid-lowering drugs and novel therapeutic approaches such as statins, ezetimibe, PCSK9 inhibitors, mipomersen and antisense oligonucleotides targeting apolipoprotein C-III (APOC3) mRNA. The genes encoding the pharmacological targets of these drugs were identified using the DrugBank database. These target genes were further classified into LDL-c reducing target genes (i.e.; LDLR, HMGCR, NPC1L1, PCSK9 and APOB) and TG reducing target genes (i.e., LPL and APOC3) based on the primary pharmacological effect (Table 1).
The summary data of LDL-C and TG were from two GWAS summary statistics containing 440,546 and 441,016 European individuals respectively (17). By obtaining instrumental variables that target each drug target to lower LDL-C or TC, they can be used to model the effects of each lipid-lowering drug. The instrumental variables were selected to be single nucleotide polymorphisms (SNP) located within ±100kb of the drug target locus and associated with LDL-C or TG levels (P< 10×5−8). To avoid the influence of strong linkage disequilibrium (LD) on the results, a threshold for LD was set (r2 < 0.3).
We used coronary heart disease (CHD) and ED as the results of drug-targeted MR analyses, where CHD was the positive control dataset to validate the feasibility and efficacy of lipid-lowering drug targets. The CHD dataset was derived from a GWAS summary statistic with a total of 184,305 cases, which contained 60,801 cases and 123,504 controls (18). ED as the main outcome, the data were from two independent GWAS datasets, respectively from FinnGen and ebi databases (19). Total testosterone (TT), bioavailable testosterone (BT), estrogen (E2) and SHBG were used as secondary outcomes, and their gender specific genetic instruments were from previously published UKB studies (20). Prostatitis (PI), prostate hyperplasia (PH), prostate cancer (PCa), aberrant spermatogenesis (AS), and male infertility (MI) equally performed as secondary outcomes with genetic instrumentation from previously published studies and the FinnGen database (21). Detailed information can be found in Supplementary Table 1.
We used the inverse variance weighted method (IVW) to estimate the causal effect between the drug targets and ED. In addition, we performed additional analyses by weighted median method and weighted model (22–24). Sufficient evidence of a causal effect was consistently provided by statistically significant IVW results plus direction of results across all 3 analyses. We also conducted Steiger filtering to ensure directional correlation between medication and outcomes.
The ‘metafor’ package was used to analyze the data indicators, and the Risk ratio OR was used as the merging statistic (25). When I2>50%, the study was considered to have greater heterogeneity, and the results of the combined analysis were analyzed using the random effects model for Meta-analysis, and when I2<50%, the study was considered to have homogeneity, and the results of the combined analysis were analyzed using the fixed effects model.
Heterogeneity was tested using MR Egger and IVW methods. Cochrane’s Q was used to evaluate the heterogeneity of the genetic tools, and p>0.05 indicated the absence of significant heterogeneity. MR Egger regression equation was used to evaluate the horizontal multiplicity of genetic tools and p > 0.05 indicated the absence of horizontal multiplicity. To ensure that our results were not significantly affected by a particular SNP, we also removed each SNP in turn using the leave-one-out method and compared the results of the IVW method with all variants.
To determine whether there was a direct relationship between the observed associations between drugs and outcomes, we used a “two-sample” approach to assess potential intermediate effects (exposure-mediated-outcome pathways) of drugs on established outcome variables (Figure 1). This method reduces the bias of high LD correlations between genetic variants in MR analyses compared with multivariate MR methods. The method uses a “product of coefficients” approach to evaluate indirect effects and a delta method to derive standard errors for indirect effects (26).
The statistical analysis in this study was conducted using RStudio software (version 4.1.2). The resources used were mainly obtained from the TwoSampleMR R package developed by Hemani et al.
We identified 48 SNPs associated with LDLR agonists, 19 SNPs associated with HMGCR inhibitors, 6 SNPs associated with NPC1L1 inhibitors, 33 SNPs associated with PCSK9 inhibitors, and 32 SNPs associated with apoB inhibitors from the genetic tools of LDL-C; Forty SNPs associated with APOC3 inhibitors and 58 SNPs associated with LPL agonists were identified from the genetic tools of TG. In the MR analysis with CHD as the outcome, as expected, the results of IVW showed that the seven drugs significantly reduced the risk of CHD (Figure 2).
According to the IVW results of the primary analysis method, LDLR agonists showed a significant association with a reduced risk of ED occurrence in the ebi database (OR [95%] = 0.755 [0.559-0.951], p=0.005). In the FinnGen database, the APOC3 inhibitors reduced the risk of ED occurrence (OR [95%] = 0.733 [0.439-1.026], p=0.038). In contrast, APOB inhibitors showed a significant association with an elevated risk of ED occurrence (OR [95%] = 2.77 [2.289-3.251], p<0.001). Results of MR analysis are shown in Table 2.
Meta-analysis was performed on the MR results obtained from each of the two databases. The combined analysis showed that LDLR, LPL agonists and APOC3 inhibitors were significantly associated with a reduced risk of ED occurrence (OR [95%] = 0.766 [0.586 - 0.947], p=0.004 vs OR [95%] = 0.806 [0.643-0.968], p=0.009 vs OR [95%] = 0.866 [0.749-0.984], p=0.016) (Figure 3).APOB inhibitors were significantly associated with an increased risk of ED occurrence (OR[95%]=1.331 [1.087-1.574], p=0.021). Other inhibitors were not significantly associated with the risk of ED occurrence.
In the results of MR analysis with sex hormone levels as an outcome, LDLR and LPL agonists were significantly associated with increased TT levels (OR[95%] = 1.048 [1.019-1.078], p=0.001 vs OR[95%] = 2.77 [2.289-3.251], p<0.001), and HMGCR was significantly associated with decreased TT and BT levels significantly (OR[95%] = 1.08 [1.060-1.1006], p<0.001 vs OR[95%] = 0.824 [0.764-0.884], p<0.001).LDLR, LPL agonists, and PCSK9, APOC3 inhibitors were significantly correlated with elevated SHBG levels, while HMGCR was decreased significantly. In addition, no significant causal relationship was found between drugs and E2 levels (Supplementary Figures 12, 13).
In studies with male-related disease as an outcome, MR results showed that LDLR agonists and PCSK9 inhibitors were significantly associated with an elevated risk of PH occurrence (OR [95%]=1.362 [1.196-1.527], p<0.001 vs OR [95%]=1.261 [1.095-1.427], p=0.006); HMGCR, NPC1L1 inhibitors were significantly associated with a reduced risk of developing PCa (OR [95%] = 0.616 [0.399-0.833], p<0.001 vs OR [95%] = 0.427 [-0.23-1.088], p=0.01); and LDLR agonists were significantly associated with a reduced risk of developing AS and MI (OR [95%] = 0.473 [-0.028 - 0.974], p=0.003 vs OR[95%] = 0.412 [-0.192 - 1.015], p=0.003); in addition, HMGCR inhibitors were significantly associated with a reduced risk of developing PCa (OR[95%] = 7.239 [6.286-8.193], p< 0.001) (Supplementary Figures 14, 15).
Cochrane’s Q and MR Egger regression equations were used to assess levels of heterogeneity and horizontal pleiotropy. When we examined the causal relationship between APOC3 inhibitors and ED (FennGen), we found significant horizontal pleiotropy (p=0.03) (Supplementary Table 11). When we examined the causal relationship between PCSK9, APOB inhibitors and ED(ebi), we found significant horizontal pleiotropy (p=0.03 vs p=0.03). Therefore, in order to obtain more reliable results, we used more stringent criteria for selecting instrumental variables, changing the LD parameters from r2<0.3 to r2<0.2 (PCSK9 and APOC3) and r2<0.1 (APOB). MR analysis was performed again, and the updated results did not show significant heterogeneity or horizontal pleiotropy, and the updated results will be used for subsequent Meta-analysis (Supplementary Table 12).
The results of the sensitivity analysis also showed no heterogeneity or horizontal pleiotropy (p>0.05) in all other outcomes (MR analysis between drugs and hormones, MR analysis between drugs and other male diseases) (Supplementary Tables 14, 16).
The leave-one-out method showed that there would be no significant difference in the results after removing any SNP (Supplementary Figures 1–11).
Given the close relationship between sex hormones and male erectile function, reproductive function, and some male disease interest, they may mediate the effect of lipid-lowering drugs on male disease risk. We therefore used the coefficient product method of mediation analysis to examine the mediating pathway from drug to disease. The results of the mediation analyses suggest that lipid-lowering drugs do not influence the risk of disease development by altering hormone levels. Sex hormone levels were not a mediator between lipid-lowering drugs and disease occurrence (Supplementary Table 18).
Erectile dysfunction is a common disease in urology and andrology, which not only brings great pain to the patient and his family, but also is not conducive to the stability of society and family. Therefore, the epidemiology and pathogenesis of ED have attracted more and more attention from scholars all over the world. A large number of experimental and clinical data show that hyperlipidemia is an important risk factor for erectile dysfunction. Current studies have shown that hyperlipidemia induced arterial stenosis and occlusion may only be the late mechanism of hyperlipidemia induced ED. Hyperlipidemia can affect the endothelial cells, smooth muscle cells and peripheral nerves of the penis in the early stage and damage the erectile function of the penis (27–29).
Firstly this study identified two potential targets, low density lipoprotein receptor (LDLR) and Lipoprotein lipase (LPL), which are significantly associated with the development of ED by drug target MR analysis. LPL is a key enzyme regulating lipid fuel processing, and decreased levels or activity can alter LPL lipolytic function, leading to hyperlipidemia and metabolic disorders in the body, causing damage to the vascular endothelium (30). Lorentzen et al.’s study concluded that high blood lipids can increase endothelial cell activity or sensitivity (31). In vitro experiments also demonstrated that high plasma TG can damage vascular endothelial cells (32). Therefore, we hypothesized that LPL gene agonists could increase LPL enzyme activity, reduce plasma TG levels, and protect vascular endothelial function, thereby achieving a reduction in the risk of ED. Similar to LPL, LDLR is a key receptor for the body to remove LDL-C from plasma by endocytosis, and it is one of the 3 genes known to be associated with autosomal dominant inheritance of familial hypercholesterolemia (33, 34). Increased LDLR expression reduces plasma cholesterol levels. Musicki et al. found that LDLR-deficient mice fed a high cholesterol diet had a significantly reduced erectile response, and further studies found significantly increased levels of oxidative stress in the penises of mice (35). Similar results were obtained in the present study that LDLR agonists significantly reduced the risk of CHD development and were significantly associated with a reduced risk of ED development.
3-Hydroxy-3-methylglutaryl coenzyme A reductase (HMGCR) inhibitors, also known as statins, are the first-line drugs used for the prevention and treatment of cardiovascular diseases (36, 37). Currently, the main statins used in clinical practice are lovastatin, simvastatin, pravastatin, fluvastatin and atorvastatin. As early as 1996, Bruckert et al. reported the first case of statin-induced ED, and subsequently divided 678 hyperlipidemic male patients aged 30-70 years into a lipid-lowering drug group and a placebo group (339 cases in each group), and found that the incidence of ED in the lipid-lowering drug group was 12.1%, and the placebo group was 5.6%, with the difference statistically significant (P=0.0029) (38). Rizvi et al. have also reported 5 patients with ED triggered by simvastatin (10 mg/d and 20 mg/d), which returned to normal after 1 week of discontinuation of the drug (2 of them had ED recurrence in the provocation test) (11). However, in the present study, MR analysis did not reveal a significant causal relationship between HMGCR inhibitors and ED occurrence. We believe that most of the patients reported in the previous literature had ED risk factors such as hyperlipidemia and essential hypertension, and the increased incidence of ED may be a result of the combined effect of the above multifactorial factors, which should not be simply attributed to lipid-lowering drugs alone. And simvastatin listed earlier, can not completely represent other statin drugs. Some scholars believe that statins cause ED may be related to their inhibition of HMG-CoA reductase to reduce cholesterol synthesis, affecting the synthesis of steroid hormones (including testosterone).Corona et al. found that 244 cases of statin users serum total testosterone and free testosterone concentrations were significantly lower than normal values (39). In the present study, MR analysis showed that HMGCR inhibitors were associated with significantly lower levels of TT, BT, and SHBG, consistent with previous studies. In addition, the non-lipid-lowering effects of statins (e.g., antioxidant effects) have received increasing attention. The results of foreign studies in recent years have shown that statins can reduce the risk of prostate cancer, decrease the recurrence rate of prostate cancer, and delay the progression of the disease (40, 41). The results of this study are consistent with previous findings, but the specific mechanism of action is not clear.
Currently lipid-lowering drugs still recommend statins as the first-line therapeutic drugs, but there are some patients who are intolerant to statins or have poor therapeutic effects (8). With the development of new drugs, some non-statin lipid-lowering drugs have been gradually applied to clinical practice. Since the identification of the causative gene, proprotein convertase subtilisin-kexin type 9 (PCSK9), in familial hypercholesterolemia family lines in 2003, the development of PCSK9-targeted inhibitors has progressed rapidly (42, 43). PCSK9 inhibitors are now widely used as a new class of lipid-lowering drugs in a wide range of patients with cardiovascular diseases, including familial hypercholesterolemia. A study by Mostaza et al. found that the R46L variant of PCSK9 was significantly associated with the development of ED, and that the prevalence of ED was higher in carriers of the T allele of the R46L gene (44). And in a real-world study, Scicali et al. evaluated the effect of PCSK9-i on sexual function as assessed by the Men’s Sexual Health Questionnaire (MSHQ) and the International Index of Erectile Function (IIEF-5) questionnaires, and found that patients’ MSHQs improved with the addition of PCSK9-i treatment, whereas there was no significant difference in the IIEF-5 before and after use (45). In the present study, MR analysis similarly did not find a significant causal relationship between PCSK9-i and the development of ED. In addition the present study also found that PCSK9-i and SHBG levels were associated with an elevated risk of PH development, but the exact mechanism still needs to be explored subsequently.
ApoC III is a strong inhibitor of LPL, which hinders the breakdown and clearance of TRLs and leads to the aggregation of circulating pro-atherosclerotic factors such as coeliac particles and VLDL. Numerous studies at home and abroad have shown that elevated serum ApoC III levels can cause hypertriacylglycerolemia, and that inhibiting ApoC III synthesis or lowering blood (46–48). Levels of ApoC III is an effective strategy for the prevention of cardiovascular disease. In the present study, ApoC III inhibitors were found to significantly reduce the risk of ED. APOB also plays a central role in human lipoprotein metabolism. The APOB gene produces two forms of apoB through a unique post-transcriptional editing process: apoB-48 and apoB-100.ApoB-100 is an important structural component of very low-density lipoproteins (VLBIs) and their metabolites, intermediate-density lipoproteins (IDL) and ApoB-100 is an important structural component of LDL and its metabolites, intermediate density lipoprotein (IDL) and LDL, as well as a ligand for receptor-mediated endocytosis of LDL (49). Increased plasma concentrations of ApoB have been shown to be a key risk factor for the development of atherosclerosis. Mipomersen, an antisense oligodeoxynucleotide inhibitor of apolipoprotein B-100, has been approved for the treatment of familial hypercholesterolemia (50). Some studies have suggested a significant negative correlation between ApoB and testosterone levels (51, 52), but the present study did not find a significant causal relationship between ApoB inhibitors and testosterone levels. It is noteworthy that ApoB inhibitors were the only lipid-lowering agents found to be associated with an increased risk of ED development in the MR analysis results of this study, and this result and the specific mechanism still need to be verified in subsequent clinical trials.
Our study has some unavoidable limitations. First, MR analysis is only a method for analyzing causal relationships between exposures and outcomes, and it is more useful for determining the direction of the association than for quantifying the magnitude of the association. It cannot completely replace clinical trials in the objective world. Secondly, drug-targeted MR analysis may not accurately reflect the effects of short-term and different routes of administration. Finally, due to insufficient GWAS data resources, we only performed MR analysis on European populations and our findings may not be applicable to other ethnicities.
In conclusion, after performing drug-targeted MR analysis, we found that APOC3 inhibitors among lipid-lowering drugs significantly reduced the risk of ED occurrence, while on the contrary APOB inhibitors may be associated with an elevated risk of ED occurrence. In addition, the results also found that PCSK9 inhibitors increased the risk of PH, and NPC1L1, HMGCR inhibitors decreased the risk of PCa, but at the same time HMGCR inhibitors increased the risk of male infertility. Notably, this study suggests that LDLR and LPL may be new candidate drug targets for the treatment of ED, and their agonists significantly reduced the risk of ED occurrence in MR analysis, but the results still need to be further validated in basic, clinical studies.
The datasets presented in this study can be found in online repositories. The names of the repository/repositories and accession number(s) can be found in the article/Supplementary Material.
Ethical approval was not required for the study involving humans in accordance with the local legislation and institutional requirements. Written informed consent to participate in this study was not required from the participants or the participants’ legal guardians/next of kin in accordance with the national legislation and the institutional requirements.
QS: Writing – original draft, Writing – review & editing. RW: Writing – review & editing. YL: Writing – review & editing. QT: Writing – original draft. KW: Writing – review & editing.
The author(s) declare that no financial support was received for the research, authorship, and/or publication of this article.
All authors would like to acknowledge FinnGen, UKB and ebi database for their selfless public sharing of GWAS summary data.
The authors declare that the research was conducted in the absence of any commercial or financial relationships that could be construed as a potential conflict of interest.
All claims expressed in this article are solely those of the authors and do not necessarily represent those of their affiliated organizations, or those of the publisher, the editors and the reviewers. Any product that may be evaluated in this article, or claim that may be made by its manufacturer, is not guaranteed or endorsed by the publisher.
The Supplementary Material for this article can be found online at: https://www.frontiersin.org/articles/10.3389/fendo.2024.1362499/full#supplementary-material
1. Shamloul R, Ghanem H. Erectile dysfunction. Lancet (2013) 381(9861):153–65. doi: 10.1016/S0140-6736(12)60520-0
2. Miner M, Parish SJ, Billups KL, Paulos M, Sigman M, Blaha MJ. Erectile dysfunction and subclinical cardiovascular disease. Sex Med Rev (2019) 7(3):455–63. doi: 10.1016/j.sxmr.2018.01.001
3. Randrup E, Baum N, Feibus A. Erectile dysfunction and cardiovascular disease. Postgrad Med (2015) 127(2):166–72. doi: 10.1080/00325481.2015.992722
4. Mostafaei H, Mori K, Hajebrahimi S, Abufaraj M, Karakiewicz PI, Shariat SF. Association of erectile dysfunction and cardiovascular disease: an umbrella review of systematic reviews and meta-analyses. BJU Int (2021) 128(1):3–11. doi: 10.1111/bju.15313
5. Wierzbicki AS, Kim EJ, Esan O, Ramachandran R. Hypertriglyceridaemia: An update. J Clin Pathol (2022) 75(12):798–806. doi: 10.1136/jclinpath-2021-207719
6. Aguilar-Salinas CA, Gomez-Diaz RA, Corral P. New therapies for primary hyperlipidemia. J Clin Endocrinol Metab (2022) 107(5):1216–24. doi: 10.1210/clinem/dgab876
7. Parhofer KG, Laufs U. The diagnosis and treatment of hypertriglyceridemia. Dtsch Arztebl Int (2019) 116(49):825–32. doi: 10.3238/arztebl.2019.0825
8. Bosco G, Di Giacomo Barbagallo F, Spampinato S, Lanzafame L, Di Pino A, Piro S, et al. Management of statin intolerant patients in the era of novel lipid lowering therapies: A critical approach in clinical practice. J Clin Med (2023) 12(6). doi: 10.3390/jcm12062444
9. Esposito K, Giugliano F, Di Palo C, Giugliano G, Marfella R, D'Andrea F, et al. Effect of lifestyle changes on erectile dysfunction in obese men: a randomized controlled trial. JAMA (2004) 291(24):2978–84. doi: 10.1001/jama.291.24.2978
11. Rizvi K, Hampson JP, Harvey JN. Do lipid-lowering drugs cause erectile dysfunction? A systematic review. Fam Pract (2002) 19(1):95–8. doi: 10.1093/fampra/19.1.95
12. Cai X, Tian Y, Wu T, Cao CX, Bu SY, Wang KJ. The role of statins in erectile dysfunction: a systematic review and meta-analysis. Asian J Androl (2014) 16(3):461–6. doi: 10.4103/1008-682X.123678
13. Tuccori M, Montagnani S, Mantarro S, Capogrosso-Sansone A, Ruggiero E, Saporiti A, et al. Neuropsychiatric adverse events associated with statins: epidemiology, pathophysiology, prevention and management. CNS Drugs (2014) 28(3):249–72. doi: 10.1007/s40263-013-0135-1
14. Sekula P, Del Greco MF, Pattaro C, Kottgen A. Mendelian randomization as an approach to assess causality using observational data. J Am Soc Nephrol (2016) 27(11):3253–65. doi: 10.1681/ASN.2016010098
15. Birney E. Mendelian randomization. Cold Spring Harb Perspect Med (2022) 12(4). doi: 10.1101/cshperspect.a041302
16. Evans DS. Target discovery for drug development using mendelian randomization. Methods Mol Biol (2022) 2547:1–20. doi: 10.1007/978-1-0716-2573-6_1
17. Richardson TG, Sanderson E, Palmer TM, Ala-Korpela M, Ference BA, Davey Smith G, et al. Evaluating the relationship between circulating lipoprotein lipids and apolipoproteins with risk of coronary heart disease: A multivariable Mendelian randomisation analysis. PloS Med (2020) 17(3):e1003062. doi: 10.1371/journal.pmed.1003062
18. Nikpay M, Goel A, Won HH, Hall LM, Willenborg C, Kanoni S, et al. A comprehensive 1,000 Genomes-based genome-wide association meta-analysis of coronary artery disease. Nat Genet (2015) 47(10):1121–30. doi: 10.1038/ng.3396
19. Bovijn J, Jackson L, Censin J, Chen CY, Laisk T, Laber S, et al. GWAS identifies risk locus for erectile dysfunction and implicates hypothalamic neurobiology and diabetes in etiology. Am J Hum Genet (2019) 104(1):157–63. doi: 10.1016/j.ajhg.2018.11.004
20. Ruth KS, Day FR, Tyrrell J, Thompson DJ, Wood AR, Mahajan A, et al. Using human genetics to understand the disease impacts of testosterone in men and women. Nat Med (2020) 26(2):252–8. doi: 10.1038/s41591-020-0751-5
21. Sakaue S, Kanai M, Tanigawa Y, Karjalainen J, Kurki M, Koshiba S, et al. A cross-population atlas of genetic associations for 220 human phenotypes. Nat Genet (2021) 53(10):1415–24. doi: 10.1038/s41588-021-00931-x
22. Burgess S, Scott RA, Timpson NJ, Davey Smith G, Thompson SG, Consortium E-I. Using published data in Mendelian randomization: a blueprint for efficient identification of causal risk factors. Eur J Epidemiol (2015) 30(7):543–52. doi: 10.1007/s10654-015-0011-z
23. Bowden J, Davey Smith G, Haycock PC, Burgess S. Consistent estimation in mendelian randomization with some invalid instruments using a weighted median estimator. Genet Epidemiol (2016) 40(4):304–14. doi: 10.1002/gepi.21965
24. Hartwig FP, Davey Smith G, Bowden J. Robust inference in summary data Mendelian randomization via the zero modal pleiotropy assumption. Int J Epidemiol (2017) 46(6):1985–98. doi: 10.1093/ije/dyx102
25. Lortie CJ, Filazzola A. A contrast of meta and metafor packages for meta-analyses in R. . Ecol Evol (2020) 10(20):10916–21. doi: 10.1002/ece3.6747
26. Woolf B, Zagkos L, Gill D. TwoStepCisMR: A novel method and R package for attenuating bias in cis-mendelian randomization analyses. Genes (Basel) (2022) 13(9). doi: 10.3390/genes13091541
27. Gandaglia G, Briganti A, Jackson G, Kloner RA, Montorsi F, Montorsi P, et al. A systematic review of the association between erectile dysfunction and cardiovascular disease. Eur Urol (2014) 65(5):968–78. doi: 10.1016/j.eururo.2013.08.023
28. Vlachopoulos C, Rokkas K, Ioakeimidis N, Stefanadis C. Inflammation, metabolic syndrome, erectile dysfunction, and coronary artery disease: common links. Eur Urol (2007) 52(6):1590–600. doi: 10.1016/j.eururo.2007.08.004
29. Vlachopoulos C, Ioakeimidis N, Rokkas K, Angelis A, Terentes-Printzios D, Kratiras Z, et al. Central haemodynamics and prediction of cardiovascular events in patients with erectile dysfunction. Am J Hypertens (2017) 30(3):249–55. doi: 10.1093/ajh/hpw150
30. Li Y, He PP, Zhang DW, Zheng XL, Cayabyab FS, Yin WD, et al. Lipoprotein lipase: from gene to atherosclerosis. Atherosclerosis (2014) 237(2):597–608. doi: 10.1016/j.atherosclerosis.2014.10.016
31. Lorentzen B, Henriksen T. Plasma lipids and vascular dysfunction in preeclampsia. Semin Reprod Endocrinol (1998) 16(1):33–9. doi: 10.1055/s-2007-1016250
32. Birkeland KI, Kilhovd B, Thorsby P, Torjesen PA, Ganss R, Vaaler S, et al. Heterogeneity of non-insulin-dependent diabetes expressed as variability in insulin sensitivity, beta-cell function and cardiovascular risk profile. Diabetes Med (2003) 20(1):37–45. doi: 10.1046/j.1464-5491.2003.00838.x
33. Go GW, Mani A. Low-density lipoprotein receptor (LDLR) family orchestrates cholesterol homeostasis. Yale J Biol Med (2012) 85(1):19–28.
34. Santos PC, Pereira AC. Type of LDLR mutation and the pharmacogenetics of familial hypercholesterolemia treatment. Pharmacogenomics (2015) 16(15):1743–50. doi: 10.2217/pgs.15.113
35. Musicki B, Liu T, Lagoda GA, Strong TD, Sezen SF, Johnson JM, et al. Hypercholesterolemia-induced erectile dysfunction: endothelial nitric oxide synthase (eNOS) uncoupling in the mouse penis by NAD(P)H oxidase. J Sex Med (2010) 7(9):3023–32. doi: 10.1111/j.1743-6109.2010.01880.x
36. Istvan ES, Deisenhofer J. Structural mechanism for statin inhibition of HMG-CoA reductase. Science (2001) 292(5519):1160–4. doi: 10.1126/science.1059344
37. Friesen JA, Rodwell VW. The 3-hydroxy-3-methylglutaryl coenzyme-A (HMG-CoA) reductases. Genome Biol (2004) 5(11):248. doi: 10.1186/gb-2004-5-11-248
38. Bruckert E, Giral P, Heshmati HM, Turpin G. Men treated with hypolipidaemic drugs complain more frequently of erectile dysfunction. J Clin Pharm Ther (1996) 21(2):89–94. doi: 10.1111/j.1365-2710.1996.tb00006.x
39. Corona G, Boddi V, Balercia G, Rastrelli G, De Vita G, Sforza A, et al. The effect of statin therapy on testosterone levels in subjects consulting for erectile dysfunction. J Sex Med (2010) 7(4 Pt 1):1547–56. doi: 10.1111/j.1743-6109.2009.01698.x
40. Ramos JD, Yu EY. Progress in understanding what is being statin(ed) in prostate cancer. JAMA Oncol (2015) 1(4):428–30. doi: 10.1001/jamaoncol.2015.0833
41. Peltomaa AI, Raittinen P, Talala K, Taari K, Tammela TLJ, Auvinen A, et al. Prostate cancer prognosis after initiation of androgen deprivation therapy among statin users. A population-based cohort study. Prostate Cancer Prostatic Dis (2021) 24(3):917–24. doi: 10.1038/s41391-021-00351-2
42. Melendez QM, Krishnaji ST, Wooten CJ, Lopez D. Hypercholesterolemia: the role of PCSK9. Arch Biochem Biophys (2017) 625-626:39–53. doi: 10.1016/j.abb.2017.06.001
43. Pasta A, Cremonini AL, Pisciotta L, Buscaglia A, Porto I, Barra F, et al. PCSK9 inhibitors for treating hypercholesterolemia. Expert Opin Pharmacother (2020) 21(3):353–63. doi: 10.1080/14656566.2019.1702970
44. Mostaza JM, Lahoz C, Salinero-Fort MA, de Dios O, Castillo E, Gonzalez-Alegre T, et al. R46L polymorphism in the PCSK9 gene: Relationship to lipid levels, subclinical vascular disease, and erectile dysfunction. J Clin Lipidol (2018) 12(4):1039–46.e3. doi: 10.1016/j.jacl.2018.04.004
45. Scicali R, Russo GI, Di Mauro M, Manuele F, Di Marco G, Di Pino A, et al. Analysis of arterial stiffness and sexual function after adding on PCSK9 inhibitor treatment in male patients with familial hypercholesterolemia: A single lipid center real-world experience. J Clin Med (2020) 9(11). doi: 10.3390/jcm9113597
46. Jin JL, Guo YL, Li JJ. Apoprotein C-III: A review of its clinical implications. Clin Chim Acta (2016) 460:50–4. doi: 10.1016/j.cca.2016.06.016
47. de la Parra Soto LG, Gutierrez-Uribe JA, Sharma A, Ramirez-Jimenez AK. Is Apo-CIII the new cardiovascular target? An analysis of its current clinical and dietetic therapies. Nutr Metab Cardiovasc Dis (2022) 32(2):295–308. doi: 10.1016/j.numecd.2021.09.035
48. Mehta A, Shapiro MD. Apolipoproteins in vascular biology and atherosclerotic disease. Nat Rev Cardiol (2022) 19(3):168–79. doi: 10.1038/s41569-021-00613-5
49. Whitfield AJ, Barrett PH, van Bockxmeer FM, Burnett JR. Lipid disorders and mutations in the APOB gene. Clin Chem (2004) 50(10):1725–32. doi: 10.1373/clinchem.2004.038026
50. Parham JS, Goldberg AC. Mipomersen and its use in familial hypercholesterolemia. Expert Opin Pharmacother (2019) 20(2):127–31. doi: 10.1080/14656566.2018.1550071
51. Rezanezhad B, Borgquist R, Willenheimer R, Elzanaty S. Association between serum levels of testosterone and biomarkers of subclinical atherosclerosis. Aging Male (2018) 21(3):182–6. doi: 10.1080/13685538.2017.1412422
Keywords: erectile dysfunction, lipids, sex hormone, male diseases, Mendelian randomization analysis
Citation: Su Q, Wang R, Luo Y, Tang Q and Wang K (2024) Genetic association of lipid-lowering drug target genes with erectile dysfunction and male reproductive health. Front. Endocrinol. 15:1362499. doi: 10.3389/fendo.2024.1362499
Received: 28 December 2023; Accepted: 23 January 2024;
Published: 08 February 2024.
Edited by:
Aleksandra Klisic, Primary Health Care Center Podgorica, MontenegroReviewed by:
Mia Manojlovic, University of Novi Sad, SerbiaCopyright © 2024 Su, Wang, Luo, Tang and Wang. This is an open-access article distributed under the terms of the Creative Commons Attribution License (CC BY). The use, distribution or reproduction in other forums is permitted, provided the original author(s) and the copyright owner(s) are credited and that the original publication in this journal is cited, in accordance with accepted academic practice. No use, distribution or reproduction is permitted which does not comply with these terms.
*Correspondence: Qizhen Tang, bG5mc3RxekAxNjMuY29t; Kenan Wang, d2FuZ2tlbmFuODZAaG90bWFpbC5jb20=
†These authors have contributed equally to this work
Disclaimer: All claims expressed in this article are solely those of the authors and do not necessarily represent those of their affiliated organizations, or those of the publisher, the editors and the reviewers. Any product that may be evaluated in this article or claim that may be made by its manufacturer is not guaranteed or endorsed by the publisher.
Research integrity at Frontiers
Learn more about the work of our research integrity team to safeguard the quality of each article we publish.