- 1School of Life Sciences, Beijing University of Chinese Medicine, Beijing, China
- 2Department of Endocrinology, Guang’anmen Hospital, China Academy of Chinese Medical Sciences, Beijing, China
- 3School of Stomatology, Changsha Medical University, Changsha, China
- 4The Hunan Provincial Key Laboratory of the TCM Agricultural Biogenomics, Changsha Medical University, Changsha, China
- 5Hunan Key Laboratory of the Research and Development of Novel Pharmaceutical Preparations, School of Pharmaceutical Science, Changsha Medical University, Changsha, China
Background: Despite the well-established findings of a higher incidence of retina-related eye diseases in patients with diabetes, there is less investigation into the causal relationship between diabetes and non-retinal eye conditions, such as age-related cataracts and glaucoma.
Methods: We performed Mendelian randomization (MR) analysis to examine the causal relationship between type 2 diabetes mellitus (T2DM) and 111 ocular diseases. We employed a set of 184 single nucleotide polymorphisms (SNPs) that reached genome-wide significance as instrumental variables (IVs). The primary analysis utilized the inverse variance-weighted (IVW) method, with MR-Egger and weighted median (WM) methods serving as supplementary analyses.
Results: The results revealed suggestive positive causal relationships between T2DM and various ocular conditions, including “Senile cataract” (OR= 1.07; 95% CI: 1.03, 1.11; P=7.77×10-4), “Glaucoma” (OR= 1.08; 95% CI: 1.02, 1.13; P=4.81×10-3), and “Disorders of optic nerve and visual pathways” (OR= 1.10; 95% CI: 0.99, 1.23; P=7.01×10-2).
Conclusion: Our evidence supports a causal relationship between T2DM and specific ocular disorders. This provides a basis for further research on the importance of T2DM management and prevention strategies in maintaining ocular health.
Introduction
Type 2 diabetes mellitus (T2DM) accounts for approximately 90% of the global population of 537 million individuals with diabetes mellitus (DM), predominantly affecting individuals over the age of 55 (1). The prevalence of T2DM is rising due to accelerated global aging, and it is projected that the worldwide diabetic population will reach 783 million by 2045 (2). DM can give rise to a range of complications, characterized by elevated disability and mortality rates (3–8). Visual impairment stands out as a particularly severe complication (9).
A systematic review and meta-analysis of global population-based eye disease surveys conducted from 1990 to 2020 established that cataract, glaucoma, age-related macular degeneration (AMD), and diabetic retinopathy (DR) are the primary causes of blindness. Importantly, early detection and timely intervention can prevent these conditions (10). It is widely recognized that there is a strong association between DM and the development of DR, with approximately one-third of diabetic patients affected by this condition (11). Furthermore, DM has been linked to several other significant visual impairments worldwide, including cataract, AMD, and glaucoma (12). For instance, a meta-analysis indicated that DM was associated with an increased risk of AMD (13), while two other studies reported a 50% reduction in AMD prevalence among patients with DM (14, 15). Moreover, there are conflicting reports regarding the association between diabetes and glaucoma. While a meta-analysis suggested that diabetes increased the prevalence of glaucoma (16), another study revealed that DM conferred a protective effect against the development of glaucomatous optic nerve damage in patients with primary open-angle glaucoma (POAG) (17). Hence, the existing research on DM and ocular-related diseases remains inconclusive. The primary reason for these conflicting findings is that most studies examining the relationship between diabetes and ocular-related diseases are observational, making it difficult to exclude the effects of confounding variables and reverse causality.
Mendelian Randomization (MR) analysis is an epidemiological approach that utilizes genetic variants associated with a specific exposure as instrumental variables (IVs). Its primary objective is to evaluate potential causal relationships between the exposure and outcome measures (18). The methodologies employed in MR analysis are grounded in Mendel’s second law, which states that alleles are randomly allocated. This characteristic, irrespective of the individual’s illness status, helps mitigate biases induced by confounding factors and reverse causality. The objective of this study is to employ the MR method to examine the causal relationship between T2DM and 111 types of ocular conditions. Furthermore, it seeks to establish a theoretical basis for the prevention and treatment of eye diseases in individuals with diabetes.
Methods
Study design
Based on the dataset acquired from the genome-wide association study (GWAS), we identified specific single nucleotide polymorphisms (SNPs) that exhibited significant associations with T2DM as the exposure variable. We utilized these SNPs as IVs and employed a MR analysis to evaluate the causal association between T2DM and the aforementioned ocular diseases.
Data sources
The data for the exposure variable (T2DM) (including 74,124 T2DM cases and 824,006 controls of European ancestry) was obtained from Anubha Mahajan et al.’s study (19), while data for the outcome variables were obtained from all 111 eye-related diseases (detailed information can be found in Supplementary Table 1) available in the FinnGen database, which is a comprehensive biomedical research project conducted in Finland (20).
Selection of IVs
We implemented specific criteria for the selection of IVs in our MR analysis. These criteria included: (1) establishing a significant genomic-level association between the IVs and the exposure (P < 5.00×10-8), (2) ensuring the independent selection of IVs by clumping within a 10 Mb window and minimizing linkage disequilibrium (R2 < 0.001), and (3) setting a minimum minor allele frequency (MAF) threshold of 0.01. We employed F-statistics to evaluate the strength of the IVs, considering values greater than 10 as indicative of a lower probability of weak instrument bias (21).
MR analysis
Among the three MR methods employed in this study, the inverse variance-weighted (IVW) method was the primary approach. IVW primarily evaluates the results by aggregating the MR effect estimates for each individual SNP. For the weighted median (WM) method to be applicable, it is necessary for the valid variable to constitute a minimum of 50%. Furthermore, an intercept term is employed by MR-Egger to assess potential pleiotropy.
Sensitivity analysis
To detect and eliminate potential outliers, we employed pleiotropy-corrected data from MR-PRESSO. We assessed heterogeneity using Cochrane Q-values. Furthermore, we employed the Leave-one-out method by removing one SNP at a time and reanalyzing whether the remaining SNPs significantly impacted the results. Causal estimates were obtained by calculating odds ratios (ORs) and their corresponding 95% confidence intervals (CIs). To handle multiple comparisons, we applied a false discovery rate (FDR) of 5%. Causal associations were considered significant if they survived FDR correction, but suggestive associations were also discussed in our study. The two-sample MR software package in R was utilized for conducting all MR analyses.
Results
Assessment of the IVs
We identified 184 SNPs from T2DM as IVs, with F statistic values ranging from 29.47 to 1393.78 (Supplementary Table 2).
Results of the MR analysis
The findings of the IVW method indicated a potential causal association between T2DM and various ocular-related diseases, including “Vitreous haemorrhage” (OR= 1.21; 95% CI: 1.06, 1.38; P=3.77×10-3), “Senile cataract” (OR= 1.07; 95% CI: 1.03, 1.11; P=7.77×10-4), “Glaucoma” (OR= 1.08; 95% CI: 1.02, 1.13; P=4.81×10-3), and “Disorders of optic nerve and visual pathways” (OR= 1.10; 95% CI: 0.99, 1.23; P=7.01×10-2) (Figures 1, 2; Supplementary Table 3). Furthermore, significant associations persisted between T2DM and diseases of the eye and adnexa, disorders of choroid and retina, and senile cataract even after adjusting for multiple comparisons. Using the MR-Egger and WM approaches, the relationships between T2DM and these ocular-related diseases had the same direction (Figure 2; Supplementary Table 3). Figure 3 displays the scatter plot illustrating the causal relationships between T2DM and these ocular-related diseases.
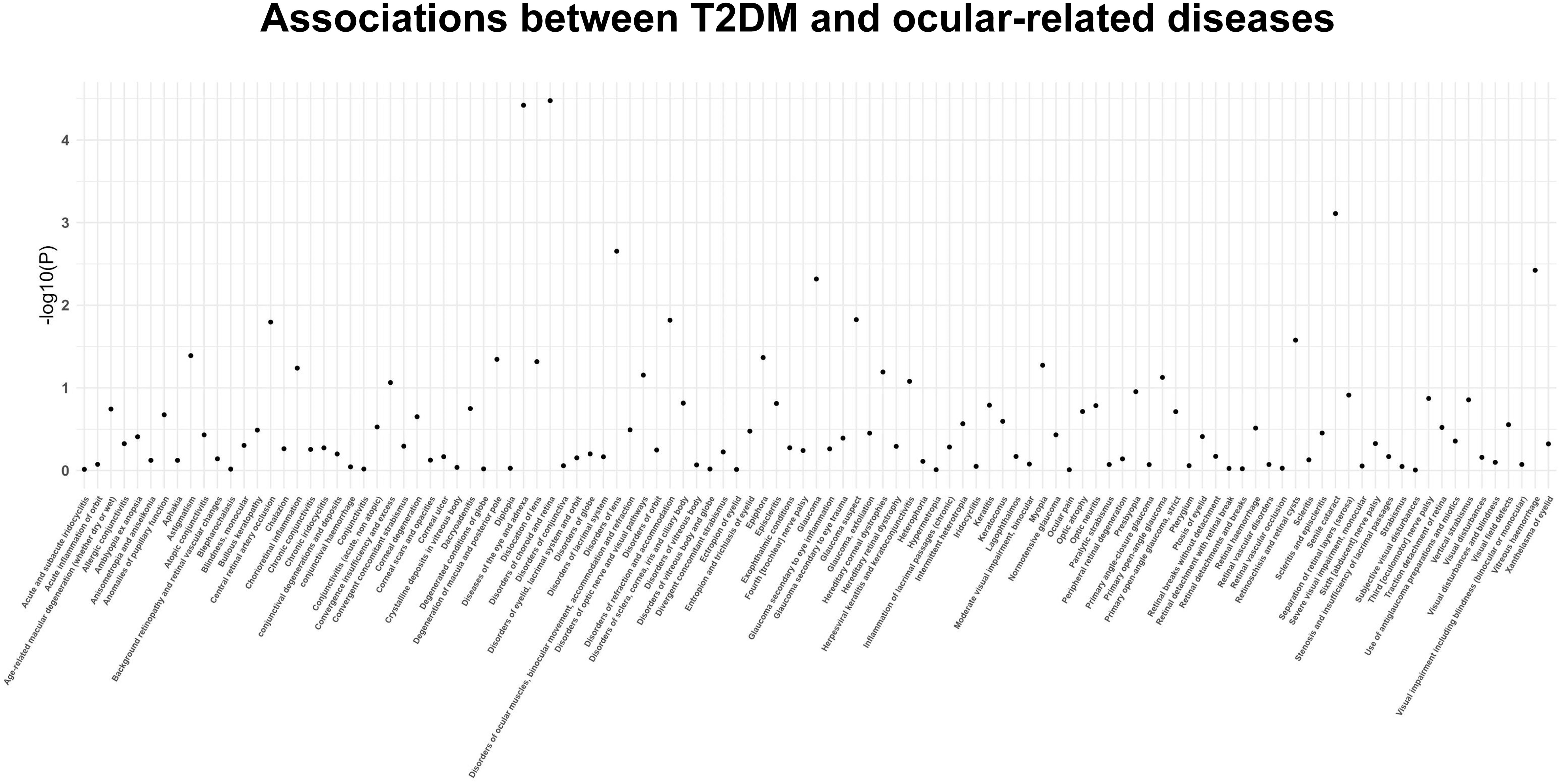
Figure 1 The P-value distribution of associations between type 2 diabetes mellitus and 111 ocular-related diseases in the Mendelian randomization analysis.
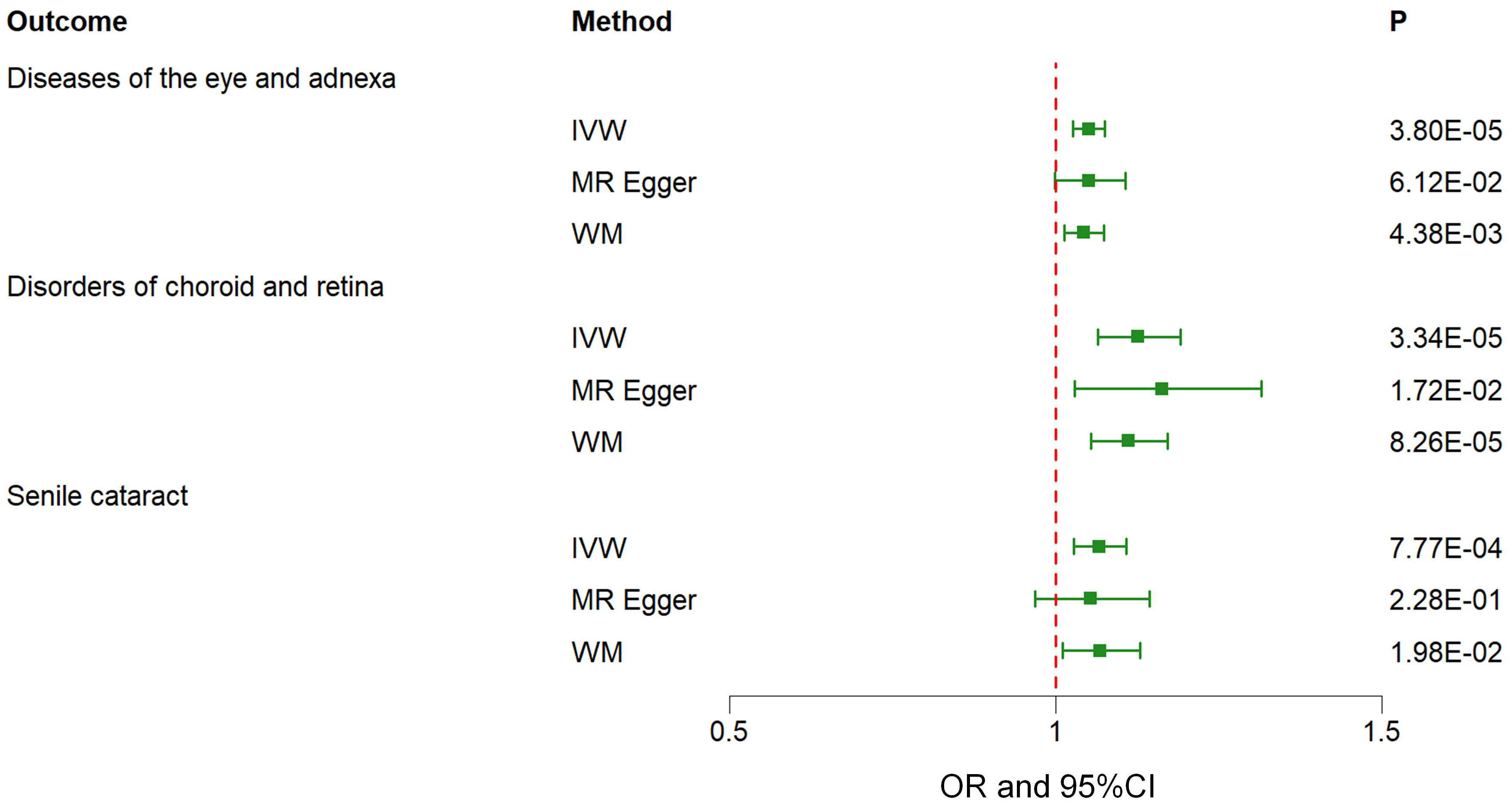
Figure 2 Associations between genetically predicted type 2 diabetes mellitus and various ocular-related diseases examined by three MR methods. MR, Mendelian randomization; IVW, inverse-variance weighted; WM, weighted median; CI, confidence interval.
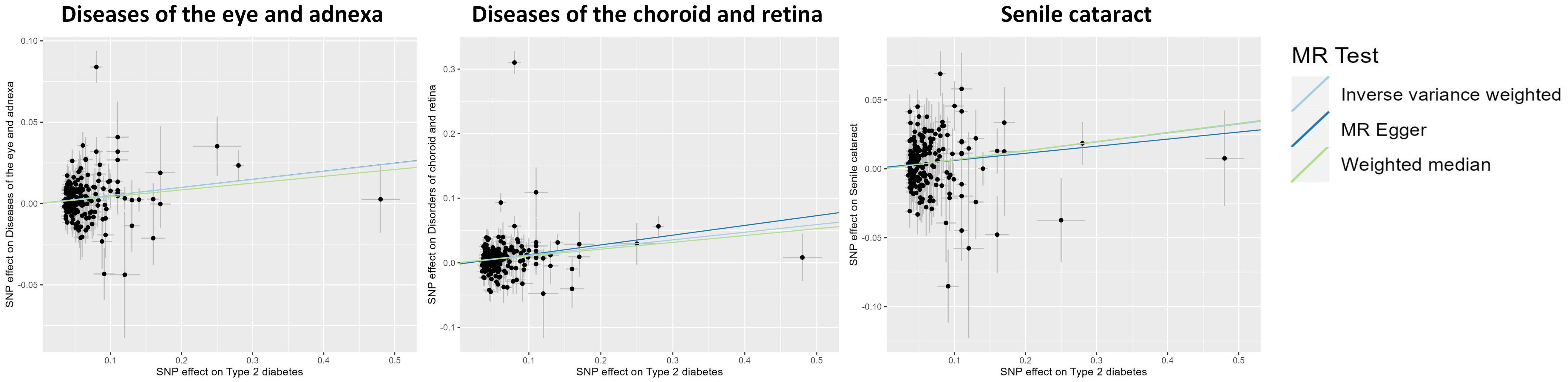
Figure 3 Scatter plot showing the causal effects of type 2 diabetes mellitus on various ocular-related diseases. SNP, single nucleotide polymorphism.
Results of the sensitivity analysis
The potential heterogeneity was evaluated (Figure 4; Supplementary Table 4). The findings presented in Supplementary Figure 1 indicate that most individual SNPs had minimal impact on the results during the leave-one-out analysis. The MR-Egger method did not detect the presence of horizontal pleiotropy (Supplementary Table 5). Despite the MR-PRESSO analysis identifying several outliers in the results, it did not significantly alter the outcomes after correction (Supplementary Table 6).
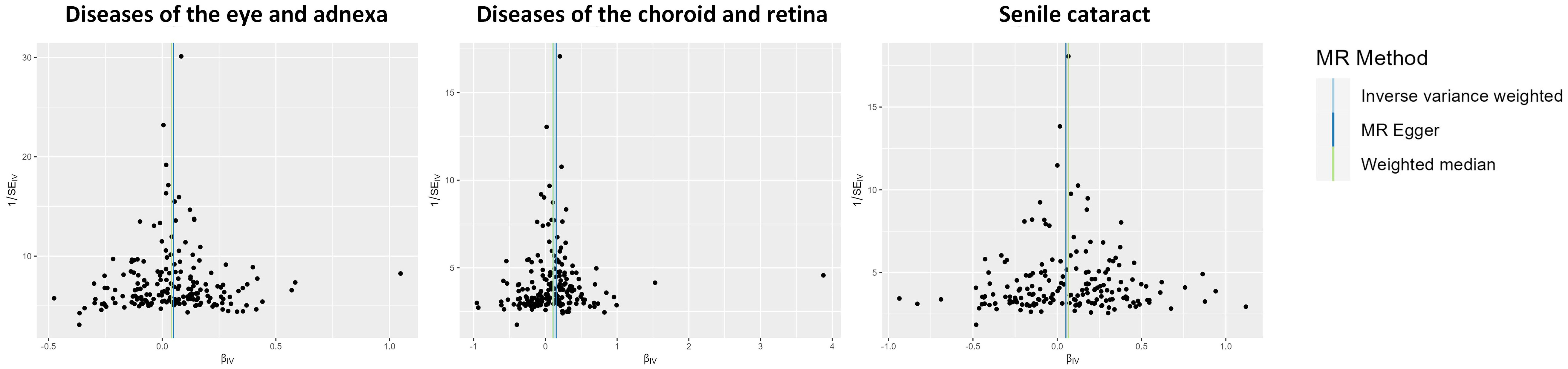
Figure 4 Funnel plot indicating the causal associations of type 2 diabetes mellitus on various ocular-related diseases. SNP, single nucleotide polymorphism; IV, instrumental variable; SE, standard error.
Discussion
In our MR analysis involving 111 ocular diseases, we identified potential causal associations between T2DM and various ocular-related diseases, such as diseases of the eye and adnexa, disorders of choroid and retina, vitreous haemorrhage, senile cataract, glaucoma, and disorders of optic nerve and visual pathways. After applying multiple corrections, the causal relationship between T2DM and diseases of the eye and adnexa, senile cataract, as well as disorders of choroid and retina, remained statistically significant. According to the definition provided by FinnGen, diseases of the eye and adnexa encompass disorders of choroid and retina (including any disease or disorder of the retina), glaucoma (characterized by increased ocular pressure due to impaired outflow), and disorders of optic nerve and visual pathways. Based on the preceding content and considering the actual clinical morbidity rate, our discussion will primarily focus on four key aspects.
Disorders of choroid and retina
Our study identified a significant causal relationship between T2DM and disorders of choroid and retina, which is consistent with prior research findings. Previous studies have shown a risk of DR ranging from 50% to 60% in patients diagnosed with T2DM (22). The underlying mechanism of T2DM causing DR may be related to oxidative stress, resulting from the formation of advanced glycation end products (AGEs). Prolonged hyperglycemia can result in the non-enzymatic glycosylation of macromolecules, such as proteins and lipids, leading to a continuous increase in the levels of AGEs (23). Previous studies have demonstrated an association between AGEs and the prevalence of DR across all stages (24). The interaction between AGEs and their receptors on the cell surface activates nicotinamide adenine dinucleotide phosphate-oxidase, promoting the generation of intracellular reactive oxygen species (ROS) (25). In turn, the enhanced ROS levels contribute to the formation of AGEs, thereby exacerbating the damage caused by AGEs (26). Oxidative stress plays a pivotal role in the pathogenesis of diabetic retinopathy. The excessive accumulation of ROS can cause damage to the retinal endovascular and surrounding tissues, leading to the development of diabetic retinopathy (27).
Disorders of optic nerve and visual pathways
The present study uncovered a potential positive causal relationship between T2DM and disorders of optic nerve and visual pathways. This finding is consistent with prior research. A cohort study found optic neuritis intensifies with the duration of diabetes and increasing glucose levels (28). This finding aligns with our research. The pathogenesis of optic nerve and visual pathway diseases in patients with T2DM may be attributed to hyperglycemia-induced oxidative stress. DM is characterized as an inflammatory disease that impacts the optic nerve through the release of inflammatory mediators resulting from oxidative stress (29). Moreover, previous research has demonstrated that elevated blood glucose levels induce the activation of Toll-like receptor 2 (TLR-2) and TLR-4 via reactive ROS, resulting in dysregulated microglial activation (30).
Glaucoma
Our study identified a potential positive causal relationship between T2DM and glaucoma. However, a MR study conducted on an East Asian population found no statistically significant association between genetically predicted type 2 diabetes and POAG risk (31). This discrepancy may be attributed to ethnic differences. Nonetheless, a cohort study carried out in South Korea reported a significant association between the incidence of T2DM and open-angle glaucoma (32). Moreover, another cohort study conducted in Korea observed that T2DM was associated with a higher risk of glaucoma incidence (33). Furthermore, another MR study focusing on glaucoma revealed an independent and causal association between T2DM and glaucoma risk (34), which aligns with our own findings. Hyperglycemia may contribute to the increased accumulation of extracellular matrix (ECM) substances, which could be an underlying mechanism of glaucoma in individuals with diabetes. The abnormal accumulation of ECM substances is a key factor contributing to elevated intraocular pressure (35). The ECM primarily consists of fibronectin and glycosaminoglycans. Studies have demonstrated that elevated glucose levels can induce the synthesis of fibronectin in trabecular meshwork cells, which plays a crucial role in regulating aqueous outflow and intraocular pressure (36). Additionally, the stiffening of the cornea due to saccharification in individuals with diabetes also may compromise the flow of aqueous humor (37, 38).
Senile cataracts
Our study has identified a significant causal relationship between T2DM and senile cataract, which is supported by previous research. A cohort study conducted in Sweden involving 35,369 women found that diabetic women had a 43% increased risk of cataract extraction (RR, 1.43; 95% CI, 1.10-1.86) (39). This finding is consistent with a meta-analysis of 20,837 subjects, which also confirmed T2DM as a risk factor for cataract (40). Additionally, Yuan et al. reported a positive correlation between genetic susceptibility to T2DM and senile cataract (41). Moreover, a previous MR study demonstrated that a higher genetic predisposition to T2DM was associated with an elevated risk of senile cataract (41). Kanishk et al. discovered that in diabetics, insufficient blood glucose control leads to increased aldose reductase (AR) levels and decreased glutathione (GSH) activity (42). AR is a critical initiator of sorbitol establishment in the lens, and abnormal sorbitol accumulation poses several risks to the lens, including lens opacity, interruption of the primary location for protein synthesis - the endoplasmic reticulum, ignition of apoptosis in lens epithelial cells, and oxidative stress damage to lens fibers (43–45). Additionally, GSH is one of the essential biochemical factors that maintain oxidative equilibrium in the lens; unusually low GSH levels can potentially affect lens transparency (46). Hence, the potential mechanism by which T2DM contributes to the development of senile cataracts may involve hyperglycemia-induced alterations in the activity of AR and GSH, thereby affecting lens metabolism.
Strengths and limitations
A major strength of this study lies in its systematic analysis using MR to examine the causal relationships between T2DM and multiple ocular-related diseases. Furthermore, our findings were reinforced through rigorous sensitivity analyses, affirming the reliability and stability of our causal conclusions. Finally, by incorporating genetic variations, we minimized confounding interference, thereby upholding the validity of our study.
The MR method itself has inherent limitations and potential problems, such as weak instruments and pleiotropy. Despite our efforts to address these issues through various methods, there are still unavoidable challenges. Firstly, we need to consider the problem of population stratification, which refers to differences in disease incidence (or trait distribution) and allele frequencies among populations. Secondly, there is a possibility of bias due to sample overlap. Although we selected two distinct samples, it is important to note that the data from these samples may not be entirely independent, as both samples consist of European populations. Lastly, while we did not find evidence of horizontal pleiotropy, it is important to acknowledge the presence of residual bias since the precise function of most SNPs remains unknown. Lastly, since our study focused on the European population, the generalizability of our findings to other ethnic groups may be limited.
Conclusion
In summary, the MR analysis results showed a significant positive causal relationship between T2DM and various ocular-related diseases, further demonstrating the adverse impact of diabetes on ocular health. As a corollary, addressing the necessity of maintaining adequate glycemic control becomes critical in protecting the ocular health of diabetics.
Data availability statement
The original contributions presented in the study are included in the article/Supplementary Material. Further inquiries can be directed to the corresponding authors.
Author contributions
RC: Writing - original draft. SX: Writing - original draft. YD: Writing - original draft. LL: Writing - original draft. CH: Writing - original draft. MB: Writing - review & editing. SL: Writing - review & editing. QW: Writing - review & editing.
Funding
The author(s) declare financial support was received for the research, authorship, and/or publication of this article. This study was supported by Young Elite Scientists Sponsorship Program by CAST (Grant No. 2019QNRC001), Special Training Program for Outstanding Young Scientific and Technological Talents (Innovation) of Chinese Academy of Chinese Medical Sciences (Grant No.ZZ14-YQ-010), the Scientific and Technological Innovation Project of China Academy of Chinese Medical Sciences (Grant No.CI2021A01612) and the BUCM Precision Cultivation Program (Grant No. JZPY-202205).
Conflict of interest
The authors declare that the research was conducted in the absence of any commercial or financial relationships that could be construed as a potential conflict of interest.
Publisher’s note
All claims expressed in this article are solely those of the authors and do not necessarily represent those of their affiliated organizations, or those of the publisher, the editors and the reviewers. Any product that may be evaluated in this article, or claim that may be made by its manufacturer, is not guaranteed or endorsed by the publisher.
Supplementary material
The Supplementary Material for this article can be found online at: https://www.frontiersin.org/articles/10.3389/fendo.2023.1307468/full#supplementary-material
Abbreviations
T2DM, type 2 diabetes mellitus; DM, diabetes mellitus; AMD, age-related macular degeneration; DR, diabetic retinopathy; POAG, primary open-angle glaucoma; MR, Mendelian randomization; IVs, instrumental variables; GWAS, genome-wide association study; SNPs, single nucleotide polymorphism; MAF, minor allele frequency; IVW, inverse-variance weighted; WM, weighted median; ORs, odds ratios; CIs, confidence intervals; FDR, false discovery rate; AGEs, advanced glycation end products; ROS, reactive oxygen species; TLR, toll-like receptor; ECM, extracellular matrix; RR, relative risk; AR, aldose reductase; GSH, glutathione.
References
1. Ahmad E, Lim S, Lamptey R, Webb DR, Davies MJ. Type 2 diabetes. Lancet (2022) 400(10365):1803–20. doi: 10.1016/S0140-6736(22)01655-5
2. Ogurtsova K, Guariguata L, Barengo NC, Ruiz PL, Sacre JW, Karuranga S, et al. IDF diabetes Atlas: Global estimates of undiagnosed diabetes in adults for 2021. Diabetes Res Clin Pract (2022) 183:109118. doi: 10.1016/j.diabres.2021.109118
3. Yang YY, Shi LX, Li JH, Yao LY, Xiang DX. Piperazine ferulate ameliorates the development of diabetic nephropathy by regulating endothelial nitric oxide synthase. Mol Med Rep (2019) 19(3):2245–53. doi: 10.3892/mmr.2019.9875
4. Xu Z, Zhang P, Chen Y, Jiang J, Zhou Z, Zhu H. Comparing SARC-calF with SARC-F for screening sarcopenia in adults with type 2 diabetes mellitus. Front Nutr (2022) 9:803924. doi: 10.3389/fnut.2022.803924
5. Luo M, Cao Q, Wang D, Tan R, Shi Y, Chen J, et al. The impact of diabetes on postoperative outcomes following spine surgery: A meta-analysis of 40 cohort studies with 2.9 million participants. Int J Surg (2022) 104:106789. doi: 10.1016/j.ijsu.2022.106789
6. Yu T, Xu B, Bao M, Gao Y, Zhang Q, Zhang X, et al. Identification of potential biomarkers and pathways associated with carotid atherosclerotic plaques in type 2 diabetes mellitus: A transcriptomics study. Front Endocrinol (Lausanne) (2022) 13:981100. doi: 10.3389/fendo.2022.981100
7. Su M, Hu R, Tang T, Tang W, Huang C. Review of the correlation between Chinese medicine and intestinal microbiota on the efficacy of diabetes mellitus. Front Endocrinol (Lausanne) (2022) 13:1085092. doi: 10.3389/fendo.2022.1085092
8. Chen J, Li X, Liu H, Zhong D, Yin K, Li Y, et al. Bone marrow stromal cell-derived exosomal circular RNA improves diabetic foot ulcer wound healing by activating the nuclear factor erythroid 2-related factor 2 pathway and inhibiting ferroptosis. Diabetes Med (2023) 40(7):e15031. doi: 10.1111/dme.15031
9. Mi W, Xia Y, Bian Y. Meta-analysis of the association between aldose reductase gene (CA)n microsatellite variants and risk of diabetic retinopathy. Exp Ther Med (2019) 18(6):4499–509. doi: 10.3892/etm.2019.8086
10. Steinmetz JD, Bourne RRA, Briant PS, Flaxman S, Taylor HR, Jonas JB. Causes of blindness and vision impairment in 2020 and trends over 30 years, and prevalence of avoidable blindness in relation to VISION 2020: the Right to Sight: an analysis for the Global Burden of Disease Study. Lancet Glob Health (2021) 9(2):e144–60. doi: 10.1016/S2214-109X(20)30489-7
11. Hou X, Wang L, Zhu D, Guo L, Weng J, Zhang M, et al. Prevalence of diabetic retinopathy and vision-threatening diabetic retinopathy in adults with diabetes in China. Nat Commun (2023) 14(1):4296. doi: 10.1038/s41467-023-39864-w
12. Feldman-Billard S, Dupas B. Eye disorders other than diabetic retinopathy in patients with diabetes. Diabetes Metab (2021) 47(6):101279. doi: 10.1016/j.diabet.2021.101279
13. Chen X, Rong SS, Xu Q, Tang FY, Liu Y, Gu H, et al. Diabetes mellitus and risk of age-related macular degeneration: a systematic review and meta-analysis. PloS One (2014) 9(9):e108196. doi: 10.1371/journal.pone.0108196
14. Bikbov MM, Zainullin RM, Gilmanshin TR, Kazakbaeva GM, Rakhimova EM, Rusakova IA, et al. Prevalence and associated factors of age-related macular degeneration in a Russian population: the ural eye and medical study. Am J Ophthalmol (2020) 210:146–57. doi: 10.1016/j.ajo.2019.10.004
15. Chakravarthy U, Bailey CC, Scanlon PH, McKibbin M, Khan RS, Mahmood S, et al. Progression from early/intermediate to advanced forms of age-related macular degeneration in a large UK cohort: rates and risk factors. Ophthalmol Retina (2020) 4(7):662–72. doi: 10.1016/j.oret.2020.01.012
16. Zhao D, Cho J, Kim MH, Friedman DS, Guallar E. Diabetes, fasting glucose, and the risk of glaucoma: a meta-analysis. Ophthalmology (2015) 122(1):72–8. doi: 10.1016/j.ophtha.2014.07.051
17. Akkaya S, Can E, Öztürk F. Comparison of the corneal biomechanical properties, optic nerve head topographic parameters, and retinal nerve fiber layer thickness measurements in diabetic and non-diabetic primary open-angle glaucoma. Int Ophthalmol (2016) 36(5):727–36. doi: 10.1007/s10792-016-0191-x
18. Burgess S, Butterworth A, Malarstig A, Thompson SG. Use of Mendelian randomisation to assess potential benefit of clinical intervention. Bmj (2012) 345:e7325. doi: 10.1136/bmj.e7325
19. Mahajan A, Taliun D, Thurner M, Robertson NR, Torres JM, Rayner NW, et al. Fine-mapping type 2 diabetes loci to single-variant resolution using high-density imputation and islet-specific epigenome maps. Nat Genet (2018) 50(11):1505–13. doi: 10.1038/s41588-018-0241-6
20. Kurki MI, Karjalainen J, Palta P, Sipilä TP, Kristiansson K, Donner KM, et al. FinnGen provides genetic insights from a well-phenotyped isolated population. Nature (2023) 613(7944):508–18. doi: 10.1038/s41586-022-05473-8
21. Burgess S, Thompson SG. Avoiding bias from weak instruments in Mendelian randomization studies. Int J Epidemiol (2011) 40(3):755–64. doi: 10.1093/ije/dyr036
22. He M, Long P, Chen T, Li K, Wei D, Zhang Y, et al. ALDH2/SIRT1 contributes to type 1 and type 2 diabetes-induced retinopathy through depressing oxidative stress. Oxid Med Cell Longev (2021) 2021:1641717. doi: 10.1155/2021/1641717
23. Shamsi A, Shahwan M, Husain FM, Khan MS. Characterization of methylglyoxal induced advanced glycation end products and aggregates of human transferrin: Biophysical and microscopic insight. Int J Biol Macromol (2019) 138:718–24. doi: 10.1016/j.ijbiomac.2019.07.140
24. Ying L, Shen Y, Zhang Y, Wang Y, Liu Y, Yin J, et al. Association of advanced glycation end products with diabetic retinopathy in type 2 diabetes mellitus. Diabetes Res Clin Pract (2021) 177:108880. doi: 10.1016/j.diabres.2021.108880
25. Guimarães ELM, Empsen C, Geerts A, van Grunsven LA. Advanced glycation end products induce production of reactive oxygen species via the activation of NADPH oxidase in murine hepatic stellate cells. J Hepatol (2010) 52(3):389–97. doi: 10.1016/j.jhep.2009.12.007
26. Moldogazieva NT, Mokhosoev IM, Mel'nikova TI, Porozov YB, Terentiev AA. Oxidative stress and advanced lipoxidation and glycation end products (ALEs and AGEs) in aging and age-related diseases. Oxid Med Cell Longev (2019) 2019:3085756. doi: 10.1155/2019/3085756
27. Kang Q, Yang C. Oxidative stress and diabetic retinopathy: Molecular mechanisms, pathogenetic role and therapeutic implications. Redox Biol (2020) 37:101799. doi: 10.1016/j.redox.2020.101799
28. Lee GI, Han K, Park KA, Oh SY. Risk of optic neuritis in type 2 diabetes mellitus: A nationwide cohort study. J Neurol Sci (2023) 450:120673. doi: 10.1016/j.jns.2023.120673
29. Sanz-Morello B, Ahmadi H, Vohra R, Saruhanian S, Freude KK, Hamann S, et al. Oxidative stress in optic neuropathies. Antioxidants (Basel) (2021) 10(10):1538. doi: 10.3390/antiox10101538
30. Altmann C, Schmidt MHH. The role of microglia in diabetic retinopathy: inflammation, microvasculature defects and neurodegeneration. Int J Mol Sci (2018) 19(1):110. doi: 10.3390/ijms19010110
31. Hu Z, Zhou F, Kaminga AC, Xu H. Type 2 diabetes, fasting glucose, hemoglobin A1c levels and risk of primary open-angle glaucoma: A mendelian randomization study. Invest Ophthalmol Vis Sci (2022) 63(5):37. doi: 10.1167/iovs.63.5.37
32. Jung Y, Han K, Park HYL, Park CK. Type 2 diabetes mellitus and risk of open-angle glaucoma development in Koreans: An 11-year nationwide propensity-score-matched study. Diabetes Metab (2018) 44(4):328–32. doi: 10.1016/j.diabet.2017.09.007
33. Jung Y, Han K, Ohn K, Kim DR, Moon JI. Association between diabetes status and subsequent onset of glaucoma in postmenopausal women. Sci Rep (2021) 11(1):18272. doi: 10.1038/s41598-021-97740-3
34. Wang K, Yang F, Liu X, Lin X, Yin H, Tang Q, et al. Appraising the effects of metabolic traits on the risk of glaucoma: A mendelian randomization study. Metabolites (2023) 13(1):109. doi: 10.3390/metabo13010109
35. Pouw AE, Greiner MA, Coussa RG, Jiao C, Han IC, Skeie JM, et al. Cell-matrix interactions in the eye: from cornea to choroid. Cells (2021) 10(3):687. doi: 10.3390/cells10030687
36. Sato T, Roy S. Effect of high glucose on fibronectin expression and cell proliferation in trabecular meshwork cells. Invest Ophthalmol Vis Sci (2002) 43(1):170–5.
37. Cui Y, Yang X, Zhang G, Guo H, Zhang M, Zhang L, et al. Intraocular pressure in general and diabetic populations from southern China: the dongguan eye study. Invest Ophthalmol Vis Sci (2019) 60(2):761–9. doi: 10.1167/iovs.18-25247
38. Gulati V, Ghate DA, Camras CB, Toris CB. Correlations between parameters of aqueous humor dynamics and the influence of central corneal thickness. Invest Ophthalmol Vis Sci (2011) 52(2):920–6. doi: 10.1167/iovs.10-5494
39. Lindblad BE, Håkansson N, Philipson B, Wolk A. Metabolic syndrome components in relation to risk of cataract extraction: a prospective cohort study of women. Ophthalmology (2008) 115(10):1687–92. doi: 10.1016/j.ophtha.2008.04.004
40. Li L, Wan XH, Zhao GH. Meta-analysis of the risk of cataract in type 2 diabetes. BMC Ophthalmol (2014) 14:94. doi: 10.1186/1471-2415-14-94
41. Yuan S, Wolk A, Larsson SC. Metabolic and lifestyle factors in relation to senile cataract: a Mendelian randomization study. Sci Rep (2022) 12(1):409. doi: 10.1038/s41598-021-04515-x
42. Khare K, Mendonca T, Rodrigues G, Kamath M, Hegde A, Nayak S, et al. Aldose reductase and glutathione in senile cataract nucleus of diabetics and non-diabetics. Int Ophthalmol (2023) 43:3673–80. doi: 10.1007/s10792-023-02776-1
43. Kumamoto Y, Takamura Y, Kubo E, Tsuzuki S, Akagi Y. Epithelial cell density in cataractous lenses of patients with diabetes: association with erythrocyte aldose reductase. Exp Eye Res (2007) 85(3):393–9. doi: 10.1016/j.exer.2007.06.007
44. Mulhern ML, Madson CJ, Danford A, Ikesugi K, Kador PF, Shinohara T. The unfolded protein response in lens epithelial cells from galactosemic rat lenses. Invest Ophthalmol Vis Sci (2006) 47(9):3951–9. doi: 10.1167/iovs.06-0193
45. Pollreisz A, Schmidt-Erfurth U. Diabetic cataract-pathogenesis, epidemiology and treatment. J Ophthalmol 2010 (2010) p:608751. doi: 10.1155/2010/608751
Keywords: type 2 diabetes, ocular diseases, diabetes complications, Mendelian randomization, causal association
Citation: Chen R, Xu S, Ding Y, Li L, Huang C, Bao M, Li S and Wang Q (2023) Dissecting causal associations of type 2 diabetes with 111 types of ocular conditions: a Mendelian randomization study. Front. Endocrinol. 14:1307468. doi: 10.3389/fendo.2023.1307468
Received: 04 October 2023; Accepted: 27 October 2023;
Published: 22 November 2023.
Edited by:
Kai Jin, Zhejiang University, ChinaReviewed by:
Zhongming Wu, Tianjin Medical University, ChinaDan-Qian Chen, Northwest University, China
Copyright © 2023 Chen, Xu, Ding, Li, Huang, Bao, Li and Wang. This is an open-access article distributed under the terms of the Creative Commons Attribution License (CC BY). The use, distribution or reproduction in other forums is permitted, provided the original author(s) and the copyright owner(s) are credited and that the original publication in this journal is cited, in accordance with accepted academic practice. No use, distribution or reproduction is permitted which does not comply with these terms.
*Correspondence: Meihua Bao, bWhiYW83OEAxNjMuY29t; Sen Li, c2VubGlAY29ubmVjdC5oa3UuaGs=; Qiuhong Wang, cWl1aG9uZ2ZvcnR1bmVAMTI2LmNvbQ==
†These authors have contributed equally to this work and share first authorship