- Cardiovascular and Metabolic Disease, Johnson & Johnson Innovative Medicine Research & Development, Spring House, PA, United States
G protein-coupled receptors (GPCRs) have emerged as important drug targets for various chronic diseases, including obesity and diabetes. Obesity is a complex chronic disease that requires long term management predisposing to type 2 diabetes, heart disease, and some cancers. The therapeutic landscape for GPCR as targets of anti-obesity medications has undergone significant changes with the approval of semaglutide, the first peptide glucagon like peptide 1 receptor agonist (GLP-1RA) achieving double digit weight loss (≥10%) and cardiovascular benefits. The enhanced weight loss, with the expected beneficial effect on obesity-related complications and reduction of major adverse cardiovascular events (MACE), has propelled the commercial opportunity for the obesity market leading to new players entering the space. Significant progress has been made on approaches targeting GPCRs such as single peptides that simultaneously activate GIP and/or GCGR in addition to GLP1, oral tablet formulation of GLP-1, small molecules nonpeptidic oral GLP1R and fixed-dose combination as well as add-on therapy for patients already treated with a GLP-1 agonist.
Introduction
G protein-coupled receptors are the largest family of membrane proteins targeted by approved drugs (1). Ligand binding results in activation of downstream pathways including G-proteins, β-arrestins and other non-G-protein transducers. Molecules that act by directly binding GPCRs are either orthosteric (bind in the same pocket as the natural ligand) or allosteric (bind in a different pocket). Allosteric modulators cooperatively interact with the natural orthosteric ligand to potentiate or attenuate receptor signaling. β-arrestins contribute to decreases in the response of the receptor to the respective agonist by internalization of the receptors. Activated GPCRs can also promote the activation of a subset of signaling pathways resulting in ‘ligand bias’ with unique cellular responses. Drugs targeting GPCRs such as GLP-1RA, glucose-dependent insulinotropic polypeptide receptor (GIPR), and melanocortin-4 receptors (MC4R) have been approved for weight management and T2D (2, 3).
Obesity is a chronic multifactorial disease (4) increasing the risk of heart disease, diabetes, liver disease, sleep apnea and certain cancers (5). It is estimated that by 2030 nearly 1 in 2 adults will be obese in US (6). In the past 5 years, major therapeutic advances have been made with multiple mechanisms progressing to the clinic and the approval of semaglutide, the first GLP-1RA leading to over 10% weight loss, an amount known to improve many of the complications associated with obesity (7). Recently semaglutide demonstrated also cardiovascular benefits (8). The increased efficacy has reduced the gap with the ~25-30% weight loss achieved with bariatric surgery during the first 2 years (9–12) and has driven additional efforts for a weight management market projected to reach 77 billion by 2030 (Weight Loss Drugs Boost Obesity Market Value | Morgan Stanley). Novel approaches have made significant progress such as oral tablet formulation of GLP-1, oral small molecules nonpeptidic GLP1R agonists and peptides dual or tri-agonists. Additional mechanisms are being developed such as PYY, GIP, amylin as a potential second activity to be combined with GLP-1RAs.
Glucagon-like peptide-1 receptor (GLP-1R)
Processing of the preproglucagon precursor by prohormone convertase 1/3 leads to production of GLP-1, GLP-2, oxyntomodulin, glucagon and glicentin in a tissue-specific manner (13–16). GLP-1 is a peptide hormone mainly secreted from the gut L cells that improve glucose homeostasis and promote weight loss (Figure 1) (13, 15–19). Liraglutide (Saxenda) and semaglutide (Wegovy), the first and the second GLP-1RA approved for weight management, achieved mean weight change from baseline of -6.4% vs -15.8% respectively in a head-to-head trial (20–22). In a recent landmark trial (SELECT trial), in over 17,000 overweight or obese non-diabetic adults with established cardiovascular disease, semaglutide reduced major adverse cardiovascular events by 20%, compared to placebo (8, 23).
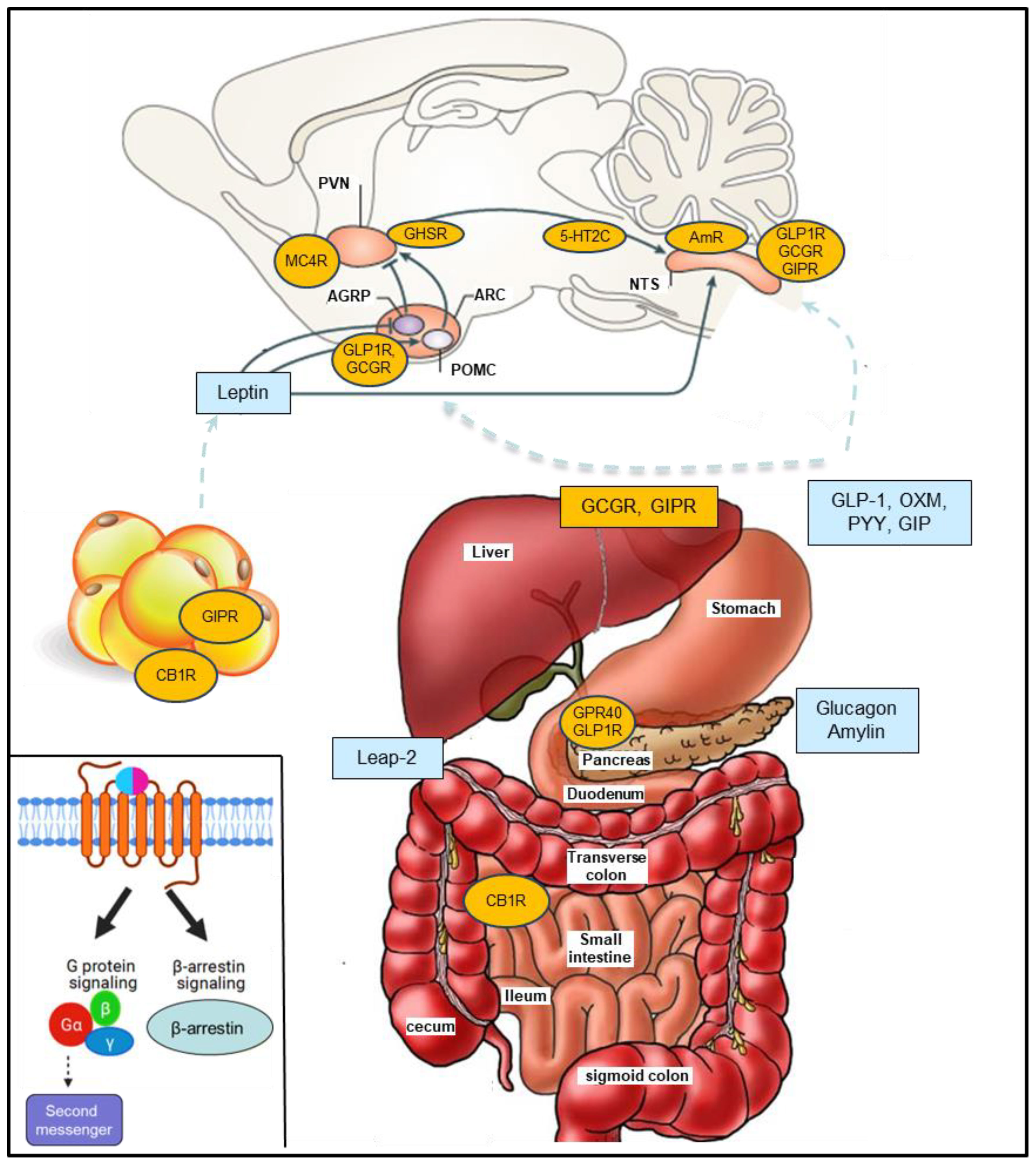
Figure 1 G-protein coupled receptors (GPCRs) and peptides discussed. The anorexigenic hormones (blue) are released from various organs mostly in the gastrointestinal system and pancreas and modulate GPCRs leading to food intake suppression, reduced gastric emptying and increased energy expenditure. Inset. GPCR signaling: Balanced agonists activate both the G protein- and β-arrestin-dependent signaling pathway while biased agonists selectively activate the GPCR-dependent signaling pathway affecting cellular response through second messenger activation or the β-arrestin-dependent signaling pathway. Glucagon-like peptide-1 (GLP-1), oxyntomodulin (OXM), gastric inhibitory polypeptide (GIP), peptide YY (PYY), cannabinoid receptor 1 (CB1R), Leap-2 (Liver-expressed antimicrobial peptide 2), melanocortin 4 receptor (MC4R), amylin receptor (AmR), 5-hydroxytryptamine receptor 2C (5-HT2CR). AgRP (Agouti-Related Protein), POMC (proopiomelanocortin); NTS, nucleus tractus solitarius; ARC, Arcuate nucleus; PVN, paraventricular nucleus.
Oral GLP-1
Although the injectable treatments have reached double digit weight loss, oral therapies are more cost effective and are more likely to achieve long term efficacy through better compliance and combination strategies (24). An oral formulation of semaglutide (14 mg) with the permeation enhancer salcaprozate sodium (SNAC) (25) has been approved as first-line therapy in adults with T2D (Rybelsus) and recent data suggest that 50 mg oral semaglutide results in an efficacy profile comparable to injectable semaglutide (26). However, to achieve sufficient absorption, Rybelsus requires food and water restrictions, an absorption enhancer and is less cost effective due to approximately 1% absorption (27). Two oral non peptidics small molecule GLP-1RA have completed phase 2 trials for weight management and T2D: Once-daily Orforglipron (LY3502970) and twice daily Danuglipron (PF-06882961). Orforglipron, a biased partial agonist of the GLP-1R with lower activation of the β-arrestin pathway (28), resulted in body weight reductions up to 12.6% (placebo adjusted) at 36 weeks in obese non-diabetic patients (29) while Danuglipron, a GLP-1R full agonist achieved approximately up to 4-5% body weight lowering at 16 weeks in T2D (30, 31).
Glucagon receptor (GCGR)
GCGR is a family B GPCR activated by glucagon mainly expressed in liver and kidney and, to a lower extent in heart, adipose tissue, pancreas, gastrointestinal tract and other tissues. Glucagon suppresses food intake and stimulates energy expenditure and hepatic glucose production (32–34). Consistently, administration of GCGR antagonists in patients with T2D resulted in decreased glucose levels (35) but also increased body weight providing human support for glucagon receptor agonism (36). Moreover, oxyntomodulin, a gut peptide co-secreted with GLP-1 with promising weight-loss and glucose-lowering properties in humans (37, 38), is a dual agonist at the GLP-1R and GCGR (39–41). Because GLP-1 improves body weight and glucose metabolism in humans (42, 43), designing single peptides co-agonists at GLP-1R and GCGR may produce superior body weight benefits to GLP-1R agonism alone and could mitigate the effect of glucagon on glucose production. Two independent papers reported for the first time the use of GLP1R/GCGR dual agonists as being of enhanced efficacy relative to pure GLP1R agonists in the treatment of rodent obesity, with simultaneous improvement in glycemic control (44, 45). Importantly, rodent models suggest that receptor balance is critical to optimize efficacy and safety (46). Translational superiority of GLP-1R/GCGR dual agonism versus GLP-1R on the body weight benefit was later demonstrated in obese rhesus monkeys (47, 48) and confirmed by other groups (49). Recently, Survodutide (BI 456906), a once weekly GLP1/GCGR dual agonist, showed up to 19% weight loss after 46 weeks in overweight and obese subjects (50). Other companies have active efforts in this space such as efinopegdutide (MK-6024) (51), pemvidutide (Altimmune, ALT-801) and mazdutide (LY3305677, IBI362, 52).
Glucose-dependent insulinotropic polypeptide receptor (GIPR)
GIP is an incretin hormone secreted from K cells in the proximal small intestine that promote nutrient-stimulated insulin release through GIPR (53–56). GIPR activation stimulates glucagon secretion, lipid accumulation in adipose tissue, bone formation and modulation of food intake and preference (57–61). Genetic and pharmacological inhibition of GIP and GIPR in mice protects from obesity (62–64). These data supported GIPR antagonism for obesity while GIP receptor agonism was thought to be ineffective for glucose lowering in T2D patients and potentially deleterious for body weight (65, 66). However, following the demonstration of superior pharmacology of GLP-1R and Glucagon receptor co-agonism to GLP-1RA (44, 45), it was discovered that also GIPR agonism can bring synergistic efficacy when combined with GLP-1 agonism (67–70). This effort resulted in the approval of Tirzepatide for T2D (Mounjaro) and obesity (Zepbound) (71–74; www.fda.gov/news-events/press-announcements/fda-approves-new-medication-chronic-weight-management). Tirzepatide favors GIPR over GLP-1R activity and it is biased for cAMP over β-arrestin recruitment at GLP-1R (74–76).
Following the initial reports of pharmacological and genetic deletion of GIPR, Killion et al. showed that an antagonist mAb to the GIPR resulted in food intake and body weight reduction in obese non-human primates and that the combination of GLP-1 agonist and GIPR antagonism resulted in additive weight loss (68, 77). This directionality was also supported by rare variants in GIPR that contribute independently to a lower body weight (78). This approach is being explored by Amgen with Maridebart cafraglutide (formerly AMG 133) which is formed by two GLP-1 agonist peptides conjugated to an anti-GIP antibody backbone (79). Killion recently reported that chronic GIP agonism desensitizes and inhibits the activity of GIPR at least in adipose tissue potentially reconciling the similar body weight directionality observed with agonists and antagonists (69).
GLP1R/GIPR/GCGR – TRI-agonists
The advancement of dual agonists for GLP-1/GIP and GLP-1/GCGR has also reinvigorated the development of triagonist peptides at the GLP-1R, GIP and GCGR (80–82). The results of a phase 2 trial of the triple agonist LY3437943 (Retatrutide) showed 24.2% weight loss at the highest dose (12 mg) in 48 weeks (23, 83). Eli Lilly recently announced initiation of 3 large phase 3 studies (TRIUMPH) of retatrutide. Multiple triple-agonists are in development including HM15211, NN9423, SAR441255.
Amylin receptor
The amylin receptor (AmR) is a GPCR composed of the calcitonin receptor (CTR) complexed with different receptor -activity -modifying proteins (RAMP) 1 or RAMP3. Amylin is a neuropeptide co-secreted with insulin by pancreatic beta-cells which slows gastric emptying, and decreases food intake (84, 85). Pramlintide is an injectable amylin analogue dosed three times a day as an adjunct for the management of T1D and T2D resulting in modest weight loss, smaller meal size and less binge eating episodes (86). CagriSema is a once-weekly injection of semaglutide and the long-acting amylin analogue cagrilintide (AM833, 87, 88) leading to nearly 16% reduction in body weight (89, 90). Recently Novo Nordisk announced the initiation of REDEFINE 4, a phase 3 trial comparing CagriSema 2.4 mg with Lilly’s Zepbound 15 mg evaluating weight loss after 72 weeks in 800 obese patients non-diabetic and is expected to be completed by October 2025 (NCT06131437). Amycretin (NNC0487-0111) is an oral formulated GLP-1 and amylin peptide being tested in phase 1 (NCT06049329). Other approaches include GUC17 (Gubra), ZP8396 (91–93), KBP-066A (94).
Neuropeptide Y receptor type 2 (Y2R)
Peptide YY (PYY) is secreted post-prandially by the L-cells of the gut together with GLP-1 (95). Secreted as PYY(1–36), it is then cleaved by the enzyme dipeptidyl peptidase 4 into PYY(3–36). PYY(3–36) activates Y2 receptors in the hypothalamus leading to appetite reduction and food reward regulation (96, 97). Bariatric surgery results in increased secretion of GLP-1 and PYY and co-infusion of PYY3–36 and GLP-1 elicit at least additive anorectic effect with ~30% reduction in food intake exceeding the 15%–20% reduction predicted to sustain a weight loss ≥10% in one year (98–101). PYY-based therapeutic development remains challenging due to emesis and low stability (102). Recent progress includes an antibody NPY2R agonist with infusion-like exposure resulting in reduction of food intake without emesis (101), Y14, a zinc-based extended-release selective Y2 receptor agonist, that demonstrated preliminary efficacy and tolerability in human subjects (103), and BI 1820237, a long acting NPY2 receptor agonist that showed decreased energy intake and delayed gastric emptying in overweight or obese men (104). Additional companies active in this space include Carmot Therapeutics and Cinfina Pharma.
Melanocortin 4 receptor (MC4R)
MC4R have been involved in food intake, metabolism, sexual behavior, and male erectile function (105, 106). Small molecules and peptide agonists of MC4R have been evaluated for obesity including MK-0493, LY2112688, MC4-NN-0453, PF-00446687 and AZD2820 and failed due to HR and BP changes, lack of human efficacy or tolerability (106). Setmelanotide (Imcivree) received FDA approval in 2020 for chronic weight management in obesity caused by genetic defects and did not result in obvious undesirable cardiovascular effects. In 2022 setmelanotide was also approved for patients with Bardet-Biedl Syndrome. Receptor selectivity, differential brain penetration and biased signaling may explain the differential profile (107, 108). Protective MC4R variants identified in the UK Biobank exhibited signaling bias toward β-arrestin recruitment and increased MAPK pathway activation suggesting that β-arrestin-biased MC4R signaling may represent an effective strategy for weight loss (109, 110). Setmelanotide has a higher potency for cAMP and a weaker effect on ERK1/2 phosphorylation when compared to alpha-MSH indicating biased agonism (110, 111).
Cannabinoid receptor 1 (CB1R)
The CB1R is a G-protein coupled receptor highly expressed in the central nervous system. CB1R antagonist/inverse agonist, rimonabant, was approved for the treatment of obesity but it was withdrawn from the market due to neuropsychiatric adverse effects (112). Multiple strategies have been proposed to circumvent the neuropsychiatric adverse effects including biased signaling, allosteric modulators, neutral antagonists, and peripherally restricted ligands since several metabolic processes appear to benefit from peripheral blockade of CB1 (113–115). Recently Novo Nordisk announced the acquisition of INV-202, an oral CB1 inverse agonist designed to preferentially block CB1R in peripheral tissues. INV-202 administration for 28 days to adult subjects resulted in an average weight loss of 3.3% (vs 0.5% gain for placebo) (https://www.novonordisk.com/news-and-media/news-and-ir-materials/news-details.html?id=166304). Zizzari demonstrated that CB1 and GLP-1 receptors modulate food intake and body weight via reciprocal functional interactions achieving greater reduction in body weight than each individual monotherapy (116).
Other GPCR targeted for the regulation of energy homeostasis
5-hydroxytryptamine receptor 2C (5-HT2CR)
Rodent studies have demonstrated that 5-HT2CR mediates most of the food intake and body weight effects of d-fenfluramine and sibutramine, but it was withdrawn from clinical use due to increased heart rate and blood pressure (117). Lorcaserin is a 5-HT2CR agonist approved for weight management, but it was withdrawn in 2020 due to a numerical imbalance in the cancers occurring in an over 12,000-participant clinical trial (118). Since then, progress has been made in 5-HT2CR research with the identification of allosteric modulators (119) acting on specific site of the receptor (120). Recently, Wagner et al. demonstrated that PPG neurons in the brainstem mediate the reduction of food intake by lorcaserin and that the combination of lorcaserin with GLP-1RA resulted in additive effects on food intake potentially providing a strategy to increase the therapeutic margin of future 5-HT2CR agonists (121).
Ghrelin receptor (GHSR)
Ghrelin administration stimulates feeding, whereas GHSR antagonists inhibit feeding (122–125). Considering the high constitutive activity of GHSR, antagonists or inverse agonists of the GHSR have been proposed as potential approaches (126). Since the GHSR system also modulates other physiological functions such as GH secretion, it is necessary to expand understanding of the pathways involved and develop biased ligands to modulate only the therapeutically relevant signaling pathway (127). LEAP-2 (Liver-expressed antimicrobial peptide 2) has been characterized as an endogenous competitive antagonist of ghrelin and proposed as a potential therapeutic target for obesity (128, 129). In a small randomized, double-blind, placebo-controlled, crossover trial, LEAP2 infusion reduced postprandial glucose excursions, growth hormone concentrations and ad libitum food intake in healthy men (130).
G protein-coupled receptor 40 (GPR40)
The orthosteric partial GPR40 agonist TAK-875 (fasiglifam) resulted in glucose improvement in T2D patients (131) but the development was halted in Phase III due to potential liver toxicity (132). Recently, GPR40 AgoPAM agonists have been identified resulting in body weight lowering and improvement of glycemic control by stimulating the secretion of insulin, glucagon, and gut peptides (133–136). Following the initial GPR40 AgoPAMs triggering rebound hyperglycemia at high doses, compounds were discovered with improved aqueous solubility that did not result in this side effect (137).
Weight loss and fat free mass
One concern among new generation drugs is the rapid weight loss and its impact on muscle mass. Sarcopenia is common among older adults with obesity and NASH patients with cirrhosis leading to increased risk for lower bone density, decreased strength and mortality. A subgroup of participants treated with Tirzepatide underwent DEXA at baseline and at week 72 in the SURMOUNT-1 trial. Tirzepatide treatment resulted in -33.9% reduction in total body fat mass (vs -8.2% placebo) and reduced lean mass by -10.9% (vs -2.6% placebo). While the percent reduction in fat mass was approximately three times greater than the reduction in lean mass, the reduction in lean mass reported is typically observed over a decade or more in elderly patients (138). Moreover, dual and triple agonists such as Retatrutide lead to chronic glucagon receptor activation that can theoretically contribute to whole-body protein catabolism (139–141). Recently Lilly announced acquisition of Versanis’ lead obesity candidate bimagrumab, a monoclonal antibody targeting activin type II receptors to inhibit atrophy and to increase muscle mass (142–144). In a 48-week phase 2 trial, intravenous bimagrumab conferred approximately a 20% reduction in fat mass and a 4.4% increase in lean mass (143). A phase 2 study is ongoing to assess if bimagrumab in addition to semaglutide is able to preserve/increase muscle mass (NCT05616013).
Discussion
The therapeutic landscape for anti-obesity medications has drastically changed in the last few years with the approval of treatments for monogenic forms of obesity and a new generation of anti-obesity medications (Figure 2) (2, 108). These new anti-obesity medications are associated with over 10% body weight loss known to improve many of the complications associated with obesity. The published data on tirzepatide suggest that second- and third-generation dual agonists and tritagonists, nicknamed “double G” or “triple G”, have the potential to be superior to semaglutide in obese patients (71, 145, 146). A head-to-head trial of tirzepatide vs. semaglutide in adults with overweight or obesity (SURMOUNT-5) was initiated in April 2023 and is expected to be completed in November 2024 (NCT05822830). Thanks to major advancements on GPCR signaling in regulating energy homeostasis, we have a better understanding of the multi-state model where the ligands’ binding affects specific downstream pathways resulting in efforts targeting selective signaling pathways (biased signaling). Interestingly, biased agonism at the GLP-1R favoring cAMP generation over β-arrestin recruitment could be involved in the efficacy of tirzepatide resulting in less receptor internalization. The clinical success of tirzepatide has revamped the attention toward GLP-1R/GCGR dual agonists and tri-agonists GLP-1R/GCGR/GIPR in which the identification of an ideal receptor balance is required to maximize efficacy and mitigate safety concerns. An important consideration for dual and tri-agonist peptides, is to monitor and clarify the clinical consequences on the cardiovascular system, especially the heart rate increase (147, 148). GLP-1R agonism causes a small rise (usually 2–3 bpm) in heart rate (149), GIP infusion increases heart rate in humans (60) and GCGR agonism appears to have a positive chronotropic and inotropic action on the heart (150). Clinical data so far have shown that retatrutide dose-dependently increases heart rate up to 6.7 bpm at 24 weeks and declined thereafter (83) while pulse rate increased with increasing doses of tirzepatide peaking (6.6. bpm) at 20 weeks and then declining (151).

Figure 2 Timeline and discoveries discussed in this minireview with therapeutic relevance in obesity.
Importantly, in the SELECT trial, differences in rates of cardiovascular events between semaglutide and placebo began to emerge within the first few months suggesting that weight loss alone may not account for the full benefits (8). SURMOUNT-MMO, an event-driven cardiovascular efficacy trial evaluating tirzepatide in adults with obesity, is expected to be completed in October 2027 (NCT05556512).
On the co-morbidities front, GLP-1R is not expressed in the liver and the data generated with semaglutide in NASH patients seems to support a lack of a direct antifibrotic effect (152). Hence, GLP-1 therapeutics that target glucagon (and GIP) may have advantages over selective GLP-1RA for liver diseases. Recently, the kidney outcome trial FLOW evaluating semaglutide in T2D and chronic kidney disease (NCT03819153; 153), was stopped early for efficacy and it is expected to read out during the first half of 2024 (154).
There is also tremendous activity in the development of non peptidic small molecules targeting the incretin system to produce drugs that are orally available. Some oral GLP-1R agonists have achieved impressive weight loss results in phase 2 but appears to have a higher rate of discontinuation due to nausea and vomiting despite an onerous titration. As with all systemic small molecules there is also the potential for off-target toxicities and drug-drug interactions that need to be evaluated. In addition to confirm comparable efficacy to injectables, oral small molecules need to show the beneficial cardiovascular effects seen with semaglutide. In the future it will be interesting to see whether a single small molecule can target multiple family B GPCR receptors. Major progress has been made in the GLP-1 combination front with long-acting amylin analogues and a better understanding of the tolerability events that have limited development of PYY therapeutics. Interestingly, GIPR agonism blocks PYY (155) and GLP-1 receptor mediated emesis and illness behaviors in preclinical species (156). Addressing the translatability and the mechanism(s) involved in the anti-emetic actions of GIP, may lead to next generation therapeutics without the onerous titration and the tolerability issues of current therapeutics.
Despite the impressive results achieved, weight reduction is still considerably lower than bariatric surgery leaving important unmet medical needs and opportunities for obesity treatments. Differences in receptor balance and signaling, tissue distribution and penetration together with a greater understanding of pathways involved in body weight regulation and tolerability leave vast opportunities available to improve upon current therapeutics.
Author contributions
AP: Writing – original draft, Writing – review & editing.
Funding
The author(s) declare that no financial support was received for the research, authorship, and/or publication of this article.
Conflict of interest
The author declares that the research was conducted in the absence of any commercial or financial relationships that could be construed as a potential conflict of interest.
Publisher’s note
All claims expressed in this article are solely those of the authors and do not necessarily represent those of their affiliated organizations, or those of the publisher, the editors and the reviewers. Any product that may be evaluated in this article, or claim that may be made by its manufacturer, is not guaranteed or endorsed by the publisher.
References
1. Sriram K, Insel PA. G protein-coupled receptors as targets for approved drugs: how many targets and how many drugs? Mol Pharmacol (2018) 93(4):251–8. doi: 10.1124/mol.117.111062
2. Ryan DH. Next generation antiobesity medications: setmelanotide, semaglutide, tirzepatide and bimagrumab: what do they mean for clinical practice? J Obes Metab Syndr (2021) 30(3):196–208. doi: 10.7570/jomes21033
3. Melson E, Miras AD, Papamargaritis D. Future therapies for obesity. Clin Med (Lond) (2023) 23(4):337–46. doi: 10.7861/clinmed.2023-0144
4. Heymsfield SB, Wadden TA. Mechanisms, pathophysiology, and management of obesity. N Engl J Med (2017) 376(3):254–66. doi: 10.1056/NEJMra1514009
5. Pantalone KM, Hobbs TM, Chagin KM, Kong SX, Wells BJ, Kattan MW, et al. Prevalence and recognition of obesity and its associated comorbidities: cross-sectional analysis of electronic health record data from a large US integrated health system. BMJ Open (2017) 7(11):e017583. doi: 10.1136/bmjopen-2017-017583
6. Ward ZJ, Bleich SN, Cradock AL, Barrett JL, Giles CM, Flax C, et al. Projected U.S. State-level prevalence of adult obesity and severe obesity. N Engl J Med (2019) 381(25):2440–50. doi: 10.1056/NEJMsa1909301
7. Ryan D, Yockey S. Weight loss and improvement in comorbidity: Differences at 5%, 10%, 15%, and over. Curr Obes Rep (2017) 6:187–94. doi: 10.1007/s13679-017-0262-y
8. Lincoff AM, Brown-Frandsen K, Colhoun HM, Deanfield J, Emerson SS, Esbjerg S, et al. Semaglutide and cardiovascular outcomes in obesity without diabetes. N Engl J Med (2023). doi: 10.1056/NEJMoa2307563. [Epub ahead of print].
9. Adams TD, Davidson LE, Litwin SE, Kolotkin RL, LaMonte MJ, Pendleton RC, et al. Health benefits of gastric bypass surgery after 6 years. JAMA (2012) 308(11):1122–31. doi: 10.1001/2012.jama.11164
10. Han Y, Jia Y, Wang H, Cao L, Zhao Y. Comparative analysis of weight loss and resolution of comorbidities between laparoscopic sleeve gastrectomy and Roux-en-Y gastric bypass: A systematic review and meta-analysis based on 18 studies. Int J Surg (2020) 76:101–10. doi: 10.1016/j.ijsu.2020.02.035
11. Syn NL, Cummings DE, Wang LZ, Lin DJ, Zhao JJ, Loh M, et al. Association of metabolic-bariatric surgery with long-term survival in adults with and without diabetes: a one-stage meta-analysis of matched cohort and prospective controlled studies with 174772 participants. Lancet (2021) 397:1830–41. doi: 10.1016/S0140-6736(21)00591-2
12. Kirwan JP, Courcoulas AP, Cummings DE, Goldfine AB, Kashyap SR, Simonson DC, et al. Diabetes remission in the alliance of randomized trials of medicine versus metabolic surgery in type 2 diabetes (ARMMS-T2D). Diabetes Care (2022) 45:1574–83. doi: 10.2337/dc21-2441
13. Holst JJ. The physiology of glucagon-like peptide 1. Physiol Rev (2007) 87(4):1409–39. doi: 10.1152/physrev.00034.2006
14. Pocai A. Unraveling oxyntomodulin, GLP1’s enigmatic brother. J Endocrinol (2012) 215(3):335–46. doi: 10.1530/JOE-12-0368
15. Drucker DJ, Habener JF, Holst JJ. Discovery, characterization, and clinical development of the glucagon-like peptides. J Clin Invest (2017) 127(12):4217–27. doi: 10.1172/JCI97233
16. Müller TD, Finan B, Bloom SR, D’Alessio D, Drucker DJ, Flatt PR, et al. Glucagon-like peptide 1 (GLP-1). Mol Metab (2019) 30:72–130. doi: 10.1016/j.molmet.2019.09.010
17. Tang-Christensen M, Larsen PJ, Göke R, Fink-Jensen A, Jessop DS, Møller M, et al. Central administration of GLP-1-(7-36) amide inhibits food and water intake in rats. Am J Physiol (1996) 271(4 Pt 2):R848–56. doi: 10.1152/ajpregu.1996.271.4.R848
18. Turton MD, O’Shea D, Gunn I, Beak SA, Edwards CM, Meeran K, et al. A role for glucagon-like peptide-1 in the central regulation of feeding. Nature (1996) 379(6560):69–72. doi: 10.1038/379069a0
19. Flint A, Raben A, Astrup A, Holst JJ. Glucagon-like peptide 1 promotes satiety and suppresses energy intake in humans. J Clin Invest (1998) 101(3):515–20. doi: 10.1172/JCI990
20. Larsen PJ, Fledelius C, Knudsen LB, Tang-Christensen M. Systemic administration of the long-acting GLP-1 derivative NN2211 induces lasting and reversible weight loss in both normal and obese rats. Diabetes (2001) 50:2530–9. doi: 10.2337/diabetes.50.11.2530
21. Lau J, Bloch P, Schäffer L, Pettersson I, Spetzler J, Kofoed J. Discovery of the once-weekly glucagon-like peptide-1 (GLP-1) analogue semaglutide. J Med Chem (2015) 58(18):7370–80. doi: 10.1021/acs.jmedchem.5b00726
22. Rubino DM, Greenway FL, Khalid U, O’Neil PM, Rosenstock J, Sørrig R, et al. STEP 8 investigators. Effect of weekly subcutaneous semaglutide vs daily liraglutide on body weight in adults with overweight or obesity without diabetes: the STEP 8 randomized clinical trial. JAMA (2022) 327(2):138–50. doi: 10.1001/jama.2021.23619
23. Harris E. Semaglutide improves heart failure and cardiovascular disease. JAMA (2023) 330(12):1127. doi: 10.1001/jama.2023.16674
24. Boye K, Ross M, Mody R, Konig M, Gelhorn H. Patients’ preferences for once-daily oral versus once-weekly injectable diabetes medications: The REVISE study. Diabetes Obes Metab (2021) 23(2):508–19. doi: 10.1111/dom.14244
25. Davies M, Pieber TR, Hartoft-Nielsen ML, Hansen OKH, Jabbour S, Rosenstock J. Effect of oral semaglutide compared with placebo and subcutaneous semaglutide on glycemic control in patients with type 2 diabetes: A randomized clinical trial. JAMA (2017) 318(15):1460–70. doi: 10.1001/jama.2017.14752
26. Knop FK, Aroda VR, do Vale RD, Holst-Hansen T, Laursen PN, Rosenstock J, et al. OASIS 1 Investigators. Oral semaglutide 50 mg taken once per day in adults with overweight or obesity (OASIS 1): a randomised, double-blind, placebo-controlled, phase 3 trial. Lancet (2023) 402(10403):705–19. doi: 10.1016/S0140-6736(23)01185-6
27. Overgaard RV, Navarria A, Ingwersen SH, Bækdal TA, Kildemoes RJ. Clinical pharmacokinet-ics of oral semaglutide: analyses of data from clinical pharmacology trials. Clin Pharmacokinet (2021) 60(10):1335–48. doi: 10.1007/s40262-021-01025-x
28. Kawai T, Sun B, Yoshino H, Feng D, Suzuki Y, Fukazawa M, et al. Structural basis for GLP-1 receptor activation by LY3502970, an orally active nonpeptide agonist. Proc Natl Acad Sci U S A. (2020) 117(47):29959–67. doi: 10.1073/pnas.2014879117
29. Wharton S, Blevins T, Connery L, Rosenstock J, Raha S, Liu R, et al. GZGI investigators. Daily oral GLP-1 receptor agonist orforglipron for adults with obesity. N Engl J Med (2023) 389(10):877–88. doi: 10.1056/NEJMoa2302392
30. Griffith DA, Edmonds DJ, Fortin JP, Kalgutkar AS, Kuzmiski JB, Loria PM, et al. A small-molecule oral agonist of the human glucagon-like peptide-1 receptor. J Med Chem (2022) 65(12):8208–26. doi: 10.1021/acs.jmedchem.1c01856
31. Saxena AR, Frias JP, Brown LS, Gorman DN, Vasas S, Tsamandouras N, et al. Efficacy and safety of oral small molecule glucagon-like peptide 1 receptor agonist danuglipron for glycemic control among patients with type 2 diabetes: A randomized clinical trial. JAMA Netw Open (2023) 6(5):e2314493. doi: 10.1001/jamanetworkopen.2023.14493
32. Habegger KM, Heppner KM, Geary N, Bartness TJ, DiMarchi R, Tschöp MH. The metabolic actions of glucagon revisited. Nat Rev Endocrinol (2010) 6(12):689–97. doi: 10.1038/nrendo.2010.187
33. Müller TD, Clemmensen C, DiMarchi RD, Tschöp MH. The new biology and pharmacology of glucagon. Physiol Rev (2017) 97(2):721–66. doi: 10.1152/physrev.00025.2016
34. Capozzi ME, D’Alessio DA, Campbell JE. The past, present, and future physiology, and pharmacology of glucagon. Cell Metab (2022) 34(11):1654–74. doi: 10.1016/j.cmet.2022.10.001
35. Bagger JI, Knop FK, Holst JJ, Vilsbøll T. Glucagon antagonism as a potential therapeutic target in type 2 diabetes. Diabetes Obes Metab (2011) 13(11):965–71. doi: 10.1111/j.1463-1326.2011.01427.x
36. Engel SS, Xu L, Andryuk PJ, Davies MJ, Amatruda J, Kaufman K, et al. Efficacy and tolerability of MK-0893, a glucagon receptor antagonist (GRA), in patients with type 2 diabetes (T2DM). Diabetes (2011) 60(Suppl. 1):A85. doi: 10.2337/db11-1-378
37. Cohen MA, Ellis SM, Le Roux CW, Batterham RL, Park A, Patterson M, et al. Oxyntomodulin suppresses appetite and reduces food in-take in humans. J Clin Endocrinol Metab (2003) 88(10):4696–701. doi: 10.1210/jc.2003-030421
38. Wynne K, Park AJ, Small CJ, Patterson M, Ellis SM, Murphy KG, et al. Subcutaneous oxyntomodulin reduces body weight in overweight and obese subjects: a double-blind, randomized, controlled trial. Diabetes (2005) 54(8):2390–5. doi: 10.2337/diabetes.54.8.2390
39. Du X, Kosinski JR, Lao J, Shen X, Petrov A, Chicchi GG. Differential effects of oxynto-modulin and GLP-1 on glucose metabolism. Am J Physiol Endocrinol Metab (2012) 303(2):E265–71. doi: 10.1152/ajpendo.00142.2012
40. Kosinski JR, Hubert J, Carrington PE, Chicchi GG, Mu J, Miller C. The glucagon receptor is involved in mediating the body weight-lowering effects of oxyntomodulin. Obes (Silver Spring) (2012) 20(8):1566–71. doi: 10.1038/oby.2012.67
41. Pocai A. Action and therapeutic potential of oxyntomodulin. Mol Metab (2013) 3(3):241–51. doi: 10.1016/j.molmet.2013.12.001
42. Gutniak M, Ørskov C, Holst JJ, Ahrén B, Efendić S. Antidiabetic effect of glucagon-like peptide-1 (7-36) amide in normal subjects and patients with diabetes mellitus. N Engl J Med (1992) 326:1316–22. doi: 10.1056/NEJM199205143262003
43. Nathan DM, Schreiber E, Fogel H, Mojsov S, Habener JF. Insulinotropic action of glucagon like peptide-I-(7-37) in diabetic and nondiabetic subjects. Diabetes Care (1992) 15:270–6. doi: 10.2337/diacare.15.2.270
44. Day JW, Ottaway N, Patterson JT, Gelfanov V, Smiley D, Gidda J, et al. A new glucagon and GLP-1 co-agonist eliminates obesity in rodents. Nat Chem Biol (2009) 5:749–57. doi: 10.1038/nchembio.209
45. Pocai A, Carrington PE, Adams JR, Wright M, Eiermann G, Zhu L, et al. Glucagon-like peptide 1/glucagon receptor dual agonism reverses obesity in mice. Diabetes (2009) 58(10):2258–66. doi: 10.2337/db09-0278
46. Day JW, Gelfanov V, Smiley D, Carrington PE, Eiermann G, Chicchi G, et al. Optimization of co-agonism at GLP-1 and glucagon receptors to safely maximize weight reduction in DIO-rodents. Biopolymers (2012) 98(5):443–50. doi: 10.1002/bip.22072
47. Lao J, Hansen BC, DiMarchi R, Pocai A. Effect of GLP1R/GCGR dual agonist in monkeys. Diabetes (2013) 62(Suppl. 1):A257. doi: 10.2337/db13-859-1394
48. Tschöp MH, Finan B, Clemmensen C, Gelfanov V, Perez-Tilve D, Müller TD, et al. Unimolecular polypharmacy for treatment of diabetes and obesity. Cell Metab (2016) 24(1):51–62. doi: 10.1016/j.cmet.2016.06.021
49. Henderson SJ, Konkar A, Hornigold DC, Trevaskis JL, Jackson R, Fritsch Fredin M. Robust anti-obesity and metabolic effects of a dual GLP-1/glucagon receptor peptide agonist in rodents and non-human primates. Diabetes Obes Metab (2016) 18(12):1176–90. doi: 10.1111/dom.12735
50. Le Roux C, Steen O, Lucas KJ, Startseva E, Unseld A, Hennige AM. A phase 2, randomized, double-blind, placebo-controlled, dose-finding study of BI 456906 in people with overweight/obesity. Diabetes (2023) 72(Supplement1):51–OR. doi: 10.2337/db23-51-OR
51. Romero-Gómez M, Lawitz E, Shankar RR, Chaudhri E, Liu J, Lam RLH, et al. MK-6024 P001 Study Group. A phase IIa active-comparator-controlled study to evaluate the efficacy and safety of efinopegdutide in patients with non-alcoholic fatty liver disease. J Hepatol (2023) 79(4):888–97. doi: 10.1016/j.jhep.2023.05.013
52. Ji L, Gao L, Jiang H, Yang J, Yu L, Wen J, et al. Safety and efficacy of a GLP-1 and glucagon receptor dual agonist mazdutide (IBI362) 9 mg and 10 mg in Chinese adults with overweight or obesity: A randomised, placebo-controlled, mul-tiple-ascending-dose phase 1b trial. EClinicalMedicine (2022) 54:101691. doi: 10.1016/j.eclinm.2022.101691
53. Seino Y, Fukushima M, Yabe D. GIP and GLP-1, the two incretin hormones: Similarities and differences. J Diabetes Investig (2010) 1(1-2):8–23. doi: 10.1111/j.2040-1124.2010.00022.x
54. McIntosh CH, Widenmaier S, Kim SJ. Glucose-dependent insulinotropic polypeptide signaling in pancreatic β-cells and adipocytes. J Diabetes Investig (2012) 3(2):96–106. doi: 10.1111/j.2040-1124.2012.00196.x
55. Chia CW, Egan JM. Incretins in obesity and diabetes. Ann N Y Acad Sci (2020) 1461(1):104–26. doi: 10.1111/nyas.14211
56. Drucker DJ, Holst JJ. The expanding incretin universe: from basic biology to clinical translation. Diabetologia (2023) 66(10):1765–79. doi: 10.1007/s00125-023-05906-7
57. Adriaenssens AE, Biggs EK, Darwish T, Tadross J, Sukthankar T, Girish M, et al. Glucose-dependent insulinotropic polypeptide receptor-expressing cells in the hypothalamus regulate food intake. Cell Metab (2019) 30(5):987–996.e6. doi: 10.1016/j.cmet.2019.07.013
58. Bergmann NC, Lund A, Gasbjerg LS, Meessen ECE, Andersen MM, Bergmann S, et al. Effects of combined GIP and GLP-1 infusion on energy intake, appetite and energy expenditure in overweight/obese individuals: a randomised, crossover study. Diabetologia (2019) 62(4):665–75. doi: 10.1007/s00125-018-4810-0
59. El K, Campbell JE. The role of GIP in α-cells and glucagon secretion. Peptides (2020) 125:170213. doi: 10.1016/j.peptides.2019.170213
60. Heimbürger SM, Bergmann NC, Augustin R, Gasbjerg LS, Christensen MB, Knop FK. Glucose-dependent insulinotropic polypeptide (GIP) and cardiovascular disease. Peptides (2020) 125:170174. doi: 10.1016/j.peptides.2019.170174
61. Zhang Q, Delessa CT, Augustin R, Bakhti M, Colldén G, Drucker DJ, et al. The glucose-dependent insulinotropic polypeptide (GIP) regulates body weight and food intake via CNS-GIPR signaling. Cell Metab (2021) 33(4):833–844.e5. doi: 10.1016/j.cmet.2021.01.015
62. Miyawaki K, Yamada Y, Ban N, Ihara Y, Tsukiyama K, Zhou H, et al. Inhibition of gastric inhibitory polypeptide signaling prevents obesity. Nat Med (2002) 8(7):738–42. doi: 10.1038/nm727
63. Gault VA, McClean PL, Cassidy RS, Irwin N, Flatt PR. Chemical gastric inhibitory polypeptide receptor antagonism protects against obesity, insulin resistance, glucose intolerance and associated disturbances in mice fed high-fat and cafeteria diets. Diabetologia (2007) 50(8):1752–62. doi: 10.1007/s00125-007-0710-4
64. McClean PL, Irwin N, Cassidy RS, Holst JJ, Gault VA, Flatt PR. GIP receptor antagonism reverses obesity, insulin re-sistance, and associated metabolic disturbances induced in mice by prolonged consumption of high-fat diet. Am J Physiol Endocrinol Metab (2007) 293(6):E1746–55. doi: 10.1152/ajpendo.00460.2007
65. Nauck M, Stöckmann F, Ebert R, Creutzfeldt W. Reduced incretin effect in Type 2 (non-insulin-dependent) diabetes. Diabetologia (1986) 29:46–52. doi: 10.1007/BF02427280
66. Nauk MA, Heimesaat MM, Ørskov C, Holst JJ, Ebert R, Creutzfeldt. W. Preserved incretin activity of glucagon-like peptide-1[7-36 amide] but not of synthetic human gastric inhibitory polypeptide in patients with type-2 diabetes mellitus. J Clin Invest (1993) 91:301–7. doi: 10.1172/JCI116186
67. Finan B, Ma T, Ottaway N, Müller TD, Habegger KM, Heppner KM, et al. Unimolecular dual incretins maximize metabolic benefits in rodents, monkeys, and humans. Sci Transl Med (2013) 5(209):209ra151. doi: 10.1126/scitranslmed.3007218
68. Killion EA, Wang J, Yie J, Shi SD, Bates D, Min X, et al. Anti-obesity effects of GIPR antagonists alone and in combination with GLP-1R agonists in preclinical models. Sci Transl Med (2018) 10(472):eaat3392. doi: 10.1126/scitranslmed.aat3392
69. Killion EA, Chen M, Falsey JR, Sivits G, Hager T, Atangan L, et al. Chronic glucose-dependent insulinotropic polypeptide recep-tor (GIPR) agonism desensitizes adipocyte GIPR activity mimicking functional GIPR antagonism. Nat Commun (2020) 11(1):4981. doi: 10.1038/s41467-020-18751-8
70. Lu SC, Chen M, Atangan L, Killion EA, Komorowski R, Cheng Y, et al. GIPR antagonist antibodies conjugated to GLP-1 peptide are bispecific molecules that decrease weight in obese mice and monkeys. Cell Rep Med (2021) 2(5):100263. doi: 10.1016/j.xcrm.2021.100263
71. Coskun T, Sloop KW, Loghin C, Alsina-Fernandez J, Urva S, Bokvist KB, et al. LY3298176, a novel dual GIP and GLP-1 receptor agonist for the treatment of type 2 diabetes mellitus: From discovery to clinical proof of concept. Mol Metab (2018) 18:3–14. doi: 10.1016/j.molmet.2018.09.009
72. Hindson J. Tirzepatide to treat obesity: phase III results. Nat Rev Gastroenterol Hepatol (2022) 19(8):488. doi: 10.1038/s41575-022-00657-z
73. Jastreboff AM, Aronne LJ, Ahmad NN, Wharton S, Connery L, Alves B, et al. SURMOUNT-1 investigators. Tirzepatide once weekly for the treatment of obesity. N Engl J Med (2022) 387(3):205–16. doi: 10.1056/NEJMoa2206038
74. Campbell JE, Müller TD, Finan B, DiMarchi RD, Tschöp MH, D’Alessio DA. GIPR/GLP-1R dual agonist therapies for diabetes and weight loss-chemistry, physiology, and clinical applications. Cell Metab (2023) 35(9):1519–29. doi: 10.1016/j.cmet.2023.07.010
75. Willard FS, Douros JD, Gabe MB, Showalter AD, Wainscott DB, Suter TM, et al. Tirzepatide is an imbalanced and biased dual GIP and GLP-1 receptor agonist. JCI Insight (2020) 5(17):e140532. doi: 10.1172/jci.insight.140532
76. Sun B, Willard FS, Feng D, Alsina-Fernandez J, Chen Q, Vieth M, et al. Structural determinants of dual incretin receptor agonism by tirzepatide. Proc Natl Acad Sci U S A. (2022) 119(13):e2116506119. doi: 10.1073/pnas.2116506119
77. Yang B, Gelfanov VM, El K, Chen A, Rohlfs R, DuBois B, et al. Discovery of a potent GIPR peptide antagonist that is effective in rodent and human systems. Mol Metab (2022) 66:101638. doi: 10.1016/j.molmet.2022.101638
78. Turcot V, Lu Y, Highland HM, Schurmann C, Justice AE, Fine RS, et al. Protein-altering variants associated with body mass index implicate pathways that control energy intake and expenditure in obesity. Nat Genet (2018) 50(1):26–41. doi: 10.1038/s41588-017-0011-x
79. Hammoud R, Drucker DJ. Beyond the pancreas: contrasting cardiometabolic actions of GIP and GLP1. Nat Rev Endocrinol (2023) 19(4):201–16. doi: 10.1038/s41574-022-00783-3
80. Finan B, Yang B, Ottaway N, Smiley DL, Ma T, Clemmensen C. A rationally designed monomeric peptide triagonist corrects obesity and diabetes in rodents. Nat Med (2015) 21(1):27–36. doi: 10.1038/nm.3761
81. Bossart M, Wagner M, Elvert R, Evers A, Hübschle T, Kloeckener T, et al. Effects on weight loss and glycemic control with SAR441255, a potent unimolecular peptide GLP-1/GIP/GCG receptor triagonist. Cell Metab (2022) 34(1):59–74.e10. doi: 10.1016/j.cmet.2021.12.005
82. Knerr PJ, Mowery SA, Douros JD, Premdjee B, Hjøllund KR, He Y, et al. Next generation GLP-1/GIP/glucagon triple agonists normalize body weight in obese mice. Mol Metab (2022) 63:101533. doi: 10.1016/j.molmet.2022.101533
83. Jastreboff AM, Kaplan LM, Frías JP, Wu Q, Du Y, Gurbuz S, et al. Retatrutide phase 2 obesity trial investigators. Triple-hormone-receptor agonist retatrutide for obesity - A phase 2 trial. N Engl J Med (2023) 389(6):514–26. doi: 10.1056/NEJMoa2301972
84. Morley JE, Morley PM, Flood JF. Anorectic effects of amylin in rats over the life span. Pharmacol Biochem Behav (1993) 44(3):577–80. doi: 10.1016/0091-3057(93)90169-t
85. Hay DL, Chen S, Lutz TA, Parkes DG, Roth JD. Amylin: pharmacology, physiology, and clinical potential. Pharmacol Rev (2015) 67(3):564–600. doi: 10.1124/pr.115.010629
86. Cao J, Belousoff MJ, Gerrard E, Danev R, Fletcher MM, Dal Maso E, et al. Structural insight into selectivity of amylin and calcitonin receptor agonists. Nat Chem Biol (2023). doi: 10.1038/s41589-023-01393-4. [Epub ahead of print].
87. Kruse T, Hansen JL, Dahl K, Schäffer L, Sensfuss U, Poulsen C, et al. Development of cagrilintide, a long-acting amylin analogue. J Med Chem (2021) 64(15):11183–94. doi: 10.1021/acs.jmedchem.1c00565
88. Lau DCW, Erichsen L, Francisco AM, Satylganova A, le Roux CW, McGowan B, et al. Once-weekly cagrilintide for weight management in people with overweight and obesity: a multicentre, randomised, double-blind, placebo-controlled and active-controlled, dose-finding phase 2 trial. Lancet (2021) 398(10317):2160–72. doi: 10.1016/S0140-6736(21)01751-7
89. Becerril S, Frühbeck G. Cagrilintide plus semaglutide for obesity management. Lancet (2021) 397(10286):1687–9. doi: 10.1016/S0140-6736(21)00944-2
90. Enebo LB, Berthelsen KK, Kankam M, Lund MT, Rubino DM, Satylganova A, et al. Safety, tolerability, pharmacokinetics, and pharmacodynamics of concomitant administration of multiple doses of cagrilintide with semaglutide 2·4 mg for weight management: a randomised, controlled, phase 1b trial. Lancet (2021) 397(10286):1736–48. doi: 10.1016/S0140-6736(21)00845-X
91. Mathiesen DS, Bagger JI, Knop FK. Long-acting amylin analogues for the management of obesity. Curr Opin Endocrinol Diabetes Obes (2022) 29(2):183–90. doi: 10.1097/MED.0000000000000716
92. Skarbaliene J, Lundqvist J, Villadsen J. ZP8396, a Novel Amylin Analogue, Induces Weight Loss in DIO Rats with a Formulation Space at Physiological pH. Diabetes (2022) 71(Supplement_1):1406–P. doi: 10.2337/db22-1406-P
93. Olsen MB, Hovelmann U, Griffin J, Knudsen KM, Johansen T, Kendall D, et al. Safety, tolerability, and clinical effects of ZP8396, a novel long-acting amylin analog—A single ascending dose trial. Diabetes (2023) 72(Supplement_1):92–LB. doi: 10.2337/db23-92-LB
94. Andreassen KV, Larsen AT, Sonne N, Mohamed KE, Karsdal MA, Henriksen K. KBP-066A, a long-acting dual amylin and calcitonin receptor agonist, induces weight loss and improves glycemic control in obese and diabetic rats. Mol Metab (2021) 53:101282. doi: 10.1016/j.molmet.2021.101282
95. Steinert RE, Feinle-Bisset C, Asarian L, Horowitz M, Beglinger C, Geary N. Ghrelin, CCK, GLP-1, and PYY(3-36): secretory controls and physiological roles in eating and glycemia in health, obesity, and after RYGB. Physiol Rev (2017) 97(1):411–63. doi: 10.1152/physrev.00031.2014
96. Batterham RL, Cowley MA, Small CJ, Herzog H, Cohen MA, Dakin CL, et al. Gut hormone PYY(3-36) physiologically inhibits food intake. Nature (2002) 418(6898):650–4. doi: 10.1038/nature00887
97. Wynne K, Bloom SR. The role of oxyntomodulin and peptide tyrosine-tyrosine (PYY) in appetite control. Nat Clin Pract Endocrinol Metab (2006) 2(11):612–20. doi: 10.1038/ncpendmet0318
98. Neary NM, Small CJ, Druce MR, Park AJ, Ellis SM, Semjonous NM, et al. Peptide YY3-36 and glucagon-like peptide-17-36 inhibit food intake additively. Endocrinology (2005) 146(12):5120–7. doi: 10.1210/en.2005-0237
99. De Silva A, Salem V, Long CJ, Makwana A, Newbould RD, Rabiner EA, et al. The gut hormones PYY 3-36 and GLP-1 7-36 amide reduce food intake and modulate brain activity in appetite centers in humans. Cell Metab (2011) 14(5):700–6. doi: 10.1016/j.cmet.2011.09.010
100. Schmidt JB, Gregersen NT, Pedersen SD, Arentoft JL, Ritz C, Schwartz TW, et al. Effects of PYY3-36 and GLP-1 on energy intake, energy expenditure, and appetite in overweight men. Am J Physiol Endocrinol Metab (2014) 306(11):E1248–56. doi: 10.1152/ajpendo.00569.2013
101. Rangwala SM, D’Aquino K, Zhang YM, Bader L, Edwards W, Zheng S, et al. A long-acting PYY3-36 analog mediates robust anorectic efficacy with minimal emesis in nonhuman primates. Cell Metab (2019) 29(4):837–843.e5. doi: 10.1016/j.cmet.2019.01.017
102. Chepurny OG, Bonaccorso RL, Leech CA, Wöllert T, Langford GM, Schwede F, et al. Chimeric peptide EP45 as a dual agonist at GLP-1 and NPY2R receptors. Sci Rep (2018) 8(1):6192. doi: 10.1038/s41598-018-24359-2
103. Tan TM, Minnion J, Khoo B, Ball LJ, Malviya R, Day E, et al. Safety and efficacy of an extended-release peptide YY analogue for obesity: A randomized, placebo-controlled, phase 1 trial. Diabetes Obes Metab (2021) 73(2):1471–83. doi: 10.1111/dom.14358
104. Kalsch B, Beetz N, Forst T, Schmid B, Schultz A, Hennige AM. A phase I study of single rising doses of BI 1820237, a novel NPY2 receptor agonist, alone or in combination with low-dose liraglutide in healthy men with overweight/obesity. Obes Facts (2023) 16(suppl 1):29. doi: 10.1159/000530456
105. Huszar D, Lynch CA, Fairchild-Huntress V, Dunmore JH, Fang Q, Berkemeier LR. Targeted disruption of the melanocortin-4 receptor results in obesity in mice. Cell (1997) 88(1):131–41. doi: 10.1016/s0092-8674(00)81865-6
106. Yeo GSH, Chao DHM, Siegert AM, Koerperich ZM, Ericson MD, Simonds SE, et al. The melanocortin pathway and energy homeostasis: From discovery to obesity therapy. Mol Metab (2021) 48:101206. doi: 10.1016/j.molmet.2021.101206
107. Collet TH, Dubern B, Mokrosinski J, Connors H, Keogh JM, Mendes, de Oliveira E, et al. Evaluation of a melanocortin-4 receptor (MC4R) agonist (Setmelanotide) in MC4R deficiency. Mol Metab (2017) 6(10):1321–9. doi: 10.1016/j.molmet.2017.06.015
108. Lazareva J, Brady SM, Yanovski JA. An evaluation of setmelanotide injection for chronic weight management in adult and pediatric patients with obesity due to Bardet-Biedl syndrome. Expert Opin Pharmacother (2023) 24(6):667–74. doi: 10.1080/14656566.2023.2199152
109. Lotta LA, Mokrosiński J, Mendes de Oliveira E, Li C, Sharp SJ, Luan J. Human gain-of-function MC4R variants show signaling bias and protect against obesity. Cell (2019) 177(3):597–607.e9. doi: 10.1016/j.cell.2019.03.044
110. Liu Z, Hruby VJ. MC4R biased signalling and the conformational basis of biological function selections. J Cell Mol Med (2022) 26(15):4125–36. doi: 10.1111/jcmm.17441
111. Hammad MM, Mohammad A, Alam-Eldin N, Madhu D, Al-Mulla F, Abu-Farha M, et al. Structural analysis of setmelanotide binding to MC4R variants in comparison to wild-type receptor. Life Sci (2022) 307:120857. doi: 10.1016/j.lfs.2022.120857
112. Boekholdt SM, Peters RJ. Rimonabant: obituary for a wonder drug. Lancet (2010) 376(9740):489–90. doi: 10.1016/S0140-6736(10)61080-X
113. Nogueiras R, Veyrat-Durebex C, Suchanek PM, Klein M, Tschöp J, Caldwell C, et al. Peripheral, but not central, CB1 antagonism provides food intake-independent metabolic benefits in diet-induced obese rats. Diabetes (2008) 57(11):2977–91. doi: 10.2337/db08-0161
114. Quarta C, Cota D. Anti-obesity therapy with peripheral CB1 blockers: from promise to safe()? practice. Int J Obes (Lond) (2020) 44(11):2179–93. doi: 10.1038/s41366-020-0577-8
115. Leo LM, Abood ME. CB1 cannabinoid receptor signaling and biased signaling. Molecules (2021) 26(17):5413. doi: 10.3390/molecules26175413
116. Zizzari P, He R, Falk S, Bellocchio L, Allard C, Clark S, et al. CB1 and GLP-1 receptors cross talk provides new therapies for obesity. Diabetes (2021) 70(2):415–22. doi: 10.2337/db20-0162
117. D’Agostino G, Lyons D, Cristiano C, Lettieri M, Olarte-Sanchez C, Burke LK. Nucleus of the solitary tract serotonin 5-HT2C receptors modulate food intake. Cell Metab (2018) 28(4):619–630.e5. doi: 10.1016/j.cmet.2018.07.017
118. Kumar R, Ryan D. Lorcaserin departs, leaving more questions than answers. Obesity (2020) 28(7):1167. doi: 10.1002/oby.22789
119. Wold EA, Wild CT, Cunningham KA, Zhou J. Targeting the 5-HT2C receptor in biological context and the current state of 5-HT2C receptor ligand development. Curr Top Med Chem (2019) 19(16):1381–98. doi: 10.2174/1568026619666190709101449
120. Wold EA, Garcia EJ, Wild CT, Miszkiel JM, Soto CA, Chen J. Discovery of 4-phenylpiperidine-2-carboxamide analogues as serotonin 5-HT2C receptor-positive allosteric modulators with enhanced drug-like properties. J Med Chem (2020) 63(14):7529–44. doi: 10.1021/acs.jmedchem.9b01953
121. Wagner S, Brierley DI, Leeson-Payne A, Jiang W, Chianese R, Lam BYH, et al. Obesity medication lorcaserin activates brainstem GLP-1 neurons to reduce food intake and augments GLP-1 receptor agonist induced appetite suppression. Mol Metab (2023) 68:101665. doi: 10.1016/j.molmet.2022.101665
122. Tschöp M, Smiley DL, Heiman ML. Ghrelin induces adiposity in rodents. Nature (2000) 407:908–13. doi: 10.1038/35038090
123. Cowley MA, Smith RG, Diano S, Tschöp M, Pronchuk N, Grove KL, et al. The distribution and mechanism of action of ghrelin in the CNS demonstrates a novel hypothalamic circuit regulating energy homeostasis. Neuron (2003) 37(4):649–61. doi: 10.1016/s0896-6273(03)00063-1
124. Horvath TL, Diano S, Tschöp M. Ghrelin in hypothalamic regulation of energy balance. Curr Top Med Chem (2003) 3(8):921–7. doi: 10.2174/1568026033452230
125. Yin Y, Li Y, Zhang W. The growth hormone secretagogue receptor: its intracellular signaling and regulation. Int J Mol Sci (2014) 15(3):4837–55. doi: 10.3390/ijms15034837
126. Holst B, Schwartz TW. Constitutive ghrelin receptor activity as a signaling set-point in appetite regulation. Trends Pharmacol Sci (2004) 25(3):113–7. doi: 10.1016/j.tips.2004.01.010
127. Sivertsen B, Holliday N, Madsen AN, Holst B. Functionally biased signalling properties of 7TM receptors - opportunities for drug development for the ghrelin receptor. Br J Pharmacol (2013) 170(7):1349–62. doi: 10.1111/bph.12361
128. Al-Massadi O, Müller T, Tschöp M, Diéguez C, Nogueiras R. Ghrelin and LEAP-2: rivals in en-ergy metabolism. Trends Pharmacol Sci (2018) 39(8):685–94. doi: 10.1016/j.tips.2018.06.004
129. Liu Z, Iyer MR, Godlewski G, Jourdan T, Liu J, Coffey NJ. Functional selectivity of a biased cannabinoid-1 receptor (CB1R) antagonist. ACS Pharmacol Transl Sci (2021) 4(3):1175–87. doi: 10.1021/acsptsci.1c00048
130. Hagemann CA, Jensen MS, Holm S, Gasbjerg LS, Byberg S, Skov-Jeppesen K, et al. LEAP2 reduces postprandial glucose excursions and ad libitum food intake in healthy men. Cell Rep Med (2022) 3(4):100582. doi: 10.1016/j.xcrm.2022.100582
131. Negoro N, Sasaki S, Mikami S, Ito M, Suzuki M, Tsujihata Y, et al. Discovery of TAK-875: A potent, selective, and orally bioavailable GPR40 agonist. ACS Med Chem Lett (2010) 1(6):290–4. doi: 10.1021/ml1000855
132. Kaku K, Enya K, Nakaya R, Ohira T, Matsuno R. Long-term safety and efficacy of fasiglifam (TAK-875), a G-protein-coupled receptor 40 agonist, as monotherapy and combination therapy in Japanese patients with type 2 diabetes: a 52-week open-label phase III study. Diabetes Obes Metab (2016) 18(9):925–9. doi: 10.1111/dom.12693
133. Itoh Y, Kawamata Y, Harada M, Kobayashi M, Fujii R, Fukusumi S, et al. Free fatty acids regulate insulin secretion from pancreatic beta cells through GPR40. Nature (2003) 422(6928):173–6. doi: 10.1038/nature01478
134. Hauge M, Vestmar MA, Husted AS, Ekberg JP, Wright MJ, Di Salvo J. GPR40 (FFAR1) - Combined Gs and Gq signaling in vitro is associated with robust incretin secretagogue action ex vivo and in vivo. Mol Metab (2014) 4(1):3–14. doi: 10.1016/j.molmet.2014.10.002
135. Ueno H, Ito R, Abe SI, Ookawara M, Miyashita H, Ogino H, et al. SCO-267, a GPR40 full agonist, improves glycemic and body weight control in rat models of diabetes and obesity. J Pharmacol Exp Ther (2019) 370(2):172–81. doi: 10.1124/jpet.118.255885
136. Rady B, Liu J, Huang H, Bakaj I, Qi J, Lee SP, et al. A FFAR1 full agonist restores islet function in models of impaired glucose-stimulated insulin secretion and diabetic non-human primates. Front Endocrinol (Lausanne) (2022) 13:1061688. doi: 10.3389/fendo.2022.1061688
137. Jurica EA, Wu X, Williams KN, Haque LE, Rampulla RA, Mathur A, et al. Optimization of physicochemical properties of pyrrolidine GPR40 AgoPAMs results in a differentiated profile with improved pharmacokinetics and reduced off-target activities. Bioorg Med Chem (2023) 85:117273. doi: 10.1016/j.bmc.2023.117273
138. Volpi E, Nazemi R, Fujita S. Muscle tissue changes with aging. Curr Opin Clin Nutr Metab Care (2004) 7(4):405–10. doi: 10.1097/01.mco.0000134362.76653.b2
139. Boden G, Rezvani I, Owen OE. Effects of glucagon on plasma amino acids. J Clin Invest (1984) 73:785–93. doi: 10.1172/JCI111272
140. Nair KS, Halliday D, Matthews DE, Welle SL. Hyperglucagonemia during insulin deficiency accelerates protein catabolism. Am J Physiol (1987) 253:E208–13. doi: 10.1152/ajpendo.1987.253.2.E208
141. Barazzoni R, Zanetti M, Tiengo A, Tessari P. Protein metabolism in glucagonoma. Diabetologia (1999) 42:326–9. doi: 10.1007/s001250051158
142. Rooks D, Petricoul O, Praestgaard J, Bartlett M, Laurent D, Roubenoff R. Safety and pharmacokinetics of bimagrumab in healthy older and obese adults with body composition changes in the older cohort. J Cachexia Sarcopenia Muscle (2020) 11(6):1525–34. doi: 10.1002/jcsm.12639
143. Heymsfield SB, Coleman LA, Miller R, Rooks DS, Laurent D, Petricoul O. Effect of bimagrumab vs placebo on body fat mass among adults with type 2 diabetes and obesity: A phase 2 randomized clinical trial. JAMA Netw Open (2021) 4(1):e2033457. doi: 10.1001/jamanetworkopen.2020.33457
144. Petricoul O, Nazarian A, Schuehly U, Schramm U, David OJ, Laurent D, et al. Pharmacokinetics and pharmacodynamics of bimagrumab (BYM338). Clin Pharmacokinet (2023) 62(1):141–55. doi: 10.1007/s40262-022-01189-0
145. Frias JP, Bastyr EJ, Vignati L, Tschoep MH, Schmitt C, Owen K, et al. The sustained effects of a dual GIP/GLP-1 receptor agonist, NNC0090-2746, in patients with type 2 diabetes. Cell Metab (2017) 26:343–352.e2. doi: 10.1016/j.cmet.2017.07.011
146. Frías JP, Davies MJ, Rosenstock J, Pérez Manghi FC, Fernández Landó L, Bergman BK, et al. SURPASS-2 investigators. Tirzepatide versus semaglutide once weekly in patients with type 2 diabetes. N Engl J Med (2021) 385(6):503–15. doi: 10.1056/NEJMoa2107519
147. Hillis GS, Woodward M, Rodgers A, Chow CK, Li Q, Zoungas S, et al. Resting heart rate and the risk of death and cardiovascular complications in patients with type 2 diabetes mellitus. Diabetologia (2012) 55(5):1283–90. doi: 10.1007/s00125-012-2471-y
148. Seviiri M, Lynch BM, Hodge AM, Yang Y, Liew D, English DR. Resting heart rate, temporal changes in resting heart rate, and overall and cause-specific mortality. Heart (2018) 104(13):1076–85. doi: 10.1136/heartjnl-2017-312251
149. Nauck MA, Meier JJ, Cavender MAA, El Aziz M, Drucker DJ. Cardiovascular actions and clinical outcomes with glucagon-like peptide-1 receptor agonists and dipeptidyl peptidase-4 inhibitors. Circulation (2017) 136(9):849–70. doi: 10.1161/CIRCULATIONAHA.117.028136
150. Petersen KM, Bøgevig S, Holst JJ, Knop FK, Christensen MB. Hemodynamic effects of glucagon: A literature review. J Clin Endocrinol Metab (2018) 103(5):1804–12. doi: 10.1210/jc.2018-00050
151. Wadden TA, Chao AM, Machineni S, Kushner R, Ard J, Srivastava G, et al. Tirzepatide after intensive lifestyle intervention in adults with overweight or obesity: the SURMOUNT-3 phase 3 trial. Nat Med (2023) 29(11):2909–18. doi: 10.1038/s41591-023-02597-w
152. Newsome PN, Buchholtz K, Cusi K, Linder M, Okanoue T, Ratziu V, et al. NN9931-4296 investigators. A placebo-controlled trial of subcutaneous semaglutide in nonalcoholic steatohepatitis. N Engl J Med (2021) 384(12):1113–24. doi: 10.1056/NEJMoa2028395
153. Rossing P, Baeres FMM, Bakris G, Bosch-Traberg H, Gislum M, Gough SCL, et al. The rationale, design and baseline data of FLOW, a kidney outcomes trial with once-weekly semaglutide in people with type 2 diabetes and chronic kidney disease. Nephrol Dial Transplant (2023) 38(9):2041–51. doi: 10.1093/ndt/gfad009
154. Gragnano F, De Sio V, Calabrò P. FLOW trial stopped early due to evidence of renal protection with semaglutide. Eur Heart J - Cardiovasc Pharmacother (2023), pvad080. doi: 10.1093/ehjcvp/pvad080
155. Samms RJ, Cosgrove R, Snider BM, Furber EC, Droz BA, Briere DA, et al. GIPR agonism inhibits PYY-induced nausea-like behavior. Diabetes (2022) 71(7):1410–23. doi: 10.2337/db21-0848
Keywords: GLP-1, GIP, semaglutide, tirzepatide, glucagon, amylin, obesity
Citation: Pocai A (2023) G protein-coupled receptors and obesity. Front. Endocrinol. 14:1301017. doi: 10.3389/fendo.2023.1301017
Received: 24 September 2023; Accepted: 29 November 2023;
Published: 14 December 2023.
Edited by:
Yi Wang, Baker Heart and Diabetes Institute, AustraliaReviewed by:
Nigel Irwin, Ulster University, United KingdomCopyright © 2023 Pocai. This is an open-access article distributed under the terms of the Creative Commons Attribution License (CC BY). The use, distribution or reproduction in other forums is permitted, provided the original author(s) and the copyright owner(s) are credited and that the original publication in this journal is cited, in accordance with accepted academic practice. No use, distribution or reproduction is permitted which does not comply with these terms.
*Correspondence: Alessandro Pocai, QWxlc3NhbmRyb19wb2NhaUB5YWhvby5jb20=