- 1Department of Endocrine and Metabolic Diseases, Shanghai Institute of Endocrine and Metabolic Diseases, Ruijin Hospital, Shanghai Jiao Tong University School of Medicine, Shanghai, China
- 2Shanghai National Clinical Research Center for Metabolic Diseases, Key Laboratory for Endocrine and Metabolic Diseases of the National Health Commission of the PR China, Shanghai National Center for Translational Medicine, Shanghai, China
- 3Pancreatic Disease Center, Department of General Surgery, Ruijin Hospital, Shanghai Jiao Tong University School of Medicine, Shanghai, China
- 4CAS Key Laboratory of Separation Science for Analytical Chemistry, Dalian Institute of Chemical Physics, Chinese Academy of Sciences, Dalian, China
Aims: We aimed to investigate changes of fecal short chain fatty acids (SCFAs) and their association with metabolic benefits after sleeve gastrectomy (SG). Specifically, whether pre-surgery SCFAs modify surgical therapeutic effects was determined.
Methods: 62 participants with measurements of fecal SCFAs and metabolic indices before and 1, 3, 6 months after SG were included. Changes of fecal SCFAs and their association with post-surgery metabolic benefits were calculated. Then, participants were stratified by medians of pre-surgery fecal SCFAs and modification effects of pre-surgery fecal SCFAs on surgical therapeutic effects were investigated, through calculating interaction of group by surgery.
Results: Fecal SCFAs were markedly changed by SG. Changes of propionate and acetate were positively correlated with serum triglycerides and total cholesterol, respectively. Notably, high pre-surgery fecal hexanoate group showed a better effect of SG treatment on lowering body weight (P=0.01), BMI (P=0.041) and serum triglycerides (P=0.031), and low pre-surgery fecal butyrate had a better effect of SG on lowering ALT (P=0.003) and AST (P=0.019).
Conclusion: Fecal SCFAs were changed and correlated with lipid profiles improvement after SG. Pre-surgery fecal hexanoate and butyrate were potential modifiers impacting metabolic benefits of SG.
1 Introduction
Obesity has reached pandemic proportions worldwide in recent decades, and bariatric surgery is the most effective treatment for reducing body mass index (BMI) in people with clinically severe obesity (1). Within these individuals, bariatric surgery offers a sustained weight loss, accompanied with improving obesity-related comorbidities including hyperglycemia, hypertension, and hyperlipidemia (2). However, the efficacy of bariatric surgery-induced metabolic benefits may vary considerably between individuals and the underlying factors remain largely unclear (3, 4).
Mounting evidence suggests that gut microbiota is closely related to obesity and other metabolic diseases in rodent models and humans (5, 6). In general, individuals with obesity show decreased bacterial diversity (7) and gene richness (8, 9), while these changes were largely restored after bariatric surgery (10). Predictive role of gut microbiota in therapeutic effects of bariatric surgery are also suggested by previous studies. For example, Prevotella-to-Bacteroides ratio is significantly lower in patients with excess weight loss less than 50% (4). Gut microbiota exerts its function mainly through different metabolites, however, what kind of metabolites derived from the intestinal microbiota can impact the metabolic benefits after bariatric surgery has scarcely been studied. Short chain fatty acids (SCFAs), are well-known end products of the intestinal microbial fermentation of indigestible dietary components (11), and have been demonstrated to play key functional roles in regulating metabolic homeostasis (12, 13). On the one hand, the extra energy from the SCFAs has been estimated to account for at least 10% of the overall energy intake in adult humans on a westernized diet, with acetate as the main energy source (14). Once in the liver, acetate and butyrate are converted to Acetyl-coA which enters the tricarboxylic acid cycle and eventually generate ATP and NADH (15). Propionate is metabolized to propionyl-CoA and is converted to succinyl-CoA and consequently generates glucose (15). As a matter of fact, propionate has been widely used in diets for dairy cows and sheep to increase glucose concentration in milk (16). On the other hand, SCFAs can be beneficial for metabolic health in some cases. In a human study, an acute administration with inulin-propionate ester significantly increases PYY and GLP-1 secretion, and reduces food intake by a mean reduction of 13.8% (17). In animal models of obesity and type 2 diabetes, oral administration of acetate, butyrate and propionate (18) decrease the accumulation of lipids in the liver and improve glucose tolerance. These studies suggest the multiple roles of SCFAs in the metabolic regulation. However, the influence of fecal SCFAs on the impact of bariatric surgery on metabolic benefits has not been investigated.
The two most common bariatric surgeries are Roux-en Y gastric bypass (RYGB) and sleeve gastrectomy (SG) with different gastrointestinal operations. Compared with RYGB, SG procedure is simpler with fewer operative risk and less post-operative complications and is more widely applied (19–21). Two previous studies using samples including both RYGB and SG revealed a decrease in acetate, propionate and butyrate at 4 to 12 months after surgeries (22, 23), and the absolute levels of branched SCFAs are significantly increased only in one study (23). However, both studies revealing changes in fecal SCFAs after bariatric surgery are mainly from RYGB, and small sample size of SG with limited follow-up time points (22, 23). As the two bariatric surgery types operate at different gastrointestinal parts, analysis using a combination of RYGB and SG is hard to see the effects of each surgy type individually. A comprehensive understanding of SCFAs changes at different time points after SG is still lacking. In addition, most of the participants in previous studies are European (23) or American (22), without data in Chinese people (22, 23). What’s more, no studies have discussed the impact of fecal SCFAs on metabolic response to bariatric surgery.
Here we comprehensively compared the profiles of fecal SCFAs in 62 subjects with obesity before and at 1, 3, 6 months after SG intervention and investigated the correlation between changes in fecal SCFAs and metabolic indices (body weight, BMI, lipid profiles, liver function, fasting glucose and insulin, blood pressure). Moreover, we classified the participants into two groups based on the medians according to baseline fecal concentrations of each SCFA, and analyzed the influence of pre-surgery fecal SCFAs on metabolic response to SG treatment through calculating the interaction of group by surgery, revealing that pre-surgery fecal hexanoate and butyrate can impact metabolic improvements of SG intervention, providing potential factors guiding post-surgery metabolic benefits.
2 Materials and methods
2.1 Cohorts
Participants with obesity (body mass index [BMI] ≥ 30 kg/m2) were recruited in the specialized obesity outpatient clinic of Ruijin Hospital, Shanghai Jiao Tong University School of Medicine, from the Genetics of Obesity in Chinese Young study established by Ruijin Hospital and registered at Clinical Trials. Gov (Clinical trial reg. no. NCT01084967 and NCT02653430, http://www.clinicaltrials.gov/) (10). In this study, we evaluated 62 subjects with obesity who underwent SG meeting the criteria according to “the Chinese Guidelines for Surgical Treatment of Obesity and Type 2 Diabetes Mellitus (2019 edition)” formulated by the Chinese Society for Metabolic & Bariatric Surgery (24). Only omnivorous individuals were included. Exclusion criteria were: (1) history of usage of any antibiotic consumption within 3 months before sample collection; (2) history of intake of any food containing probiotics such as yogurt within 7 days before sample collection. Surgeries were performed by the same group, with standardization of the processes and technique.
In our study, all patients were given the same advice on their diets before or after the operation, according to “the Chinese Guidelines for Surgical Treatment of Obesity and Type 2 Diabetes Mellitus (2019 edition)” formulated by the Chinese Society for Metabolic & Bariatric Surgery (24): (1) Low-calorie diets (recommended daily calorie intake: 1400 kcal/day) were given from 10 days before the operation in the hospital; (2) From 1 to 7 days after the operation, bland and liquid food was given according to the patients' condition; (3) When all the patients were discharged from the hospital, low-sugar, low-fat, decaffeinated semi-liquid and soft foods were recommended within one month after surgery. All the patients complied well with the recommendation as self-reported.
62 participants were included in our study initially, and 42, 36, 31 of the participants attended follow-up visit at 1, 3, 6 months after SG, respectively, with anthropometric data, blood and fecal samples. The study was approved by the Institutional Review Board of the Ruijin Hospital, Shanghai Jiao Tong University School of Medicine and a written informed consent was obtained from each participant. Figure 1 showed the flow diagram of the study protocol.
2.2 Fecal short-chain fatty acid analysis
The SCFA concentration was determined using ultra-high performance liquid chromatography-tandem mass spectrometry (UHPLC-MS). A Waters ACQUITY-Ultra High Performance liquid chromatography system (Waters Corp, Milford, USA) coupled with Q Exactive mass spectrometry (Thermo Fisher Scientific, Rockford, IL, USA) was used in negative ion mode. A 5 μL aliquot of each sample was injected into a BEH C18 column (2.1 mm×100 mm, 1.7 μm, Waters Corp, Milford, CT, USA). The Mobile phases were 0.1% formic acid in H2O (phase A) and 0.1% formic acid in acetonitrile (phase B). The gradient started with 15% B, increased to 27% B within 4 min and arrived to 42% at 8 min. Then it increased to 100% in 0.5 min and kept for 3 min. Finally, it returned to the initial 15% B and was stable for 2.5 minutes. The flow rate was 0.35 mL/min and the column temperature was at 40°C. The MS capillary temperature was 300°C with the auxiliary air heating temperature set at 350°C. The sheath gas and auxiliary gas flow rate were set as 45 and 10 units and the spray voltage was 3 KV. Full scan resolution was set as 70K, m/z scan range was 80-1200 Dalton. Thermo Scientific Xcalibur 4.1.31.9 was used for data acquisition.
2.3 Clinical parameter measurements
Blood samples were collected after fasting for at least 12 hours, and analyzed for fasting plasma glucose, fasting serum insulin, hemoglobin A1c (HbA1c), alanine aminotransferase (ALT), alanine aminotransferase (ALT), triglycerides, and total cholesterol. Fasting glucose, ALT, AST, triglycerides, and total cholesterol were measured using an autoanalyser (Beckman Coulter AU5800). Fasting insulin was measured using a double antibody radioimmunoassay (DSL, Webster). HbA1c was measured by high-pressure liquid chromatography. Insulin resistance index (homeostasis model assessment of insulin resistance, HOMA-IR) was defined as fasting insulin (IU/mL) × fasting glucose (mmol/L)/22.5, and pancreatic β-cell function index (homeostasis model assessment of β-cell function, HOMA-β) was defined as 20 × fasting insulin (IU/mL)/(fasting glucose (mmol/L) − 3.5).
2.4 Statistical analysis
Differences in fecal SCFAs levels and proportions before surgery and at different time-points after surgery were calculated by the paired samples Wilcoxon signed rank test. A P value < 0.05 was considered statistically significant.
We compared the correlation between percent changes ((pre-surgery values-post-surgery values)/pre-surgery values*100) 6 months after bariatric surgery in fecal SCFAs and clinical indices, analyzed by the Spearman correlation test. A P value < 0.05 was considered statistically significant. The correlation heatmap was drawn by GraphPad Prism 9.5.1.
A mixed-effect linear model was employed to discern the impact of bariatric surgery on body weight and metabolic outcomes. More specifically, metabolic measurements, encompassing body weight, BMI, lipid profiles, liver function, blood pressure, and glycemic parameters, were designated as dependent variables. The bariatric surgery was treated as the independent variable, while baseline metabolic measurements and individual basic profiles, such as age and gender, were incorporated as covariates. To mitigate bias introduced by repeated measurements of each participant pre- and post-surgery, individual IDs were treated as random effects.
The same model configured as above was employed to estimate the adjusted effect of bariatric surgery on metabolic outcomes within the population stratified by SCFA groups (with the median serving as the cutoff point for each SCFA).
A similar model with the addition of an interaction term was used to evaluate whether pre-surgery SCFA concentration, as determined by pre-surgery fecal concentration (with the median serving as the cutoff point for each SCFA), modified the effect of bariatric surgery on metabolic outcomes.
All of the calculations above were employed by IBM SPSS Statistics 25.0.
3 Results
3.1 Clinical characteristics before sleeve gastrectomy
Table 1 summarized the clinical characteristics of recruited participants before SG. In total, 21 men and 41 women with a mean age of 30.45 years old and mean BMI of 43.35 kg/m2 were included in the analyses, and 42, 36, 31 participants had a follow-up visit at 1, 3, 6 months after SG surgery, respectively.
3.2 Fecal SCFA levels and proportions before and after SG
We examined the changes in the concentrations of fecal SCFAs before and at 1, 3, 6 months after SG treatment (Table 2). Among them, acetic acid levels were significantly decreased at 3 time points after SG. Propionic acid levels were significantly decreased at 3, 6 months after SG and showed decreasing trend at 1 month after SG. Butyric acid levels were significantly decreased at 1, 3 months after SG, but rebounded at 6 months after SG. Valeric acid levels, one of minor straight SCFAs, were increased at 3 months after SG. Two main branched SCFAs, isobutyric acid and isovaleric acid, were significantly increased at 1 and 3 months after SG treatment. We also calculated the total concentration of the subgroups of SCFAs. In general, total levels of three major straight SCFAs (acetic-, propionic-, and butyric acids) were lowered at three time points after SG, while total levels of major branched SCFAs (isobutyric- and isovaleric-acids) were raised at 1 months and 3 months after SG.
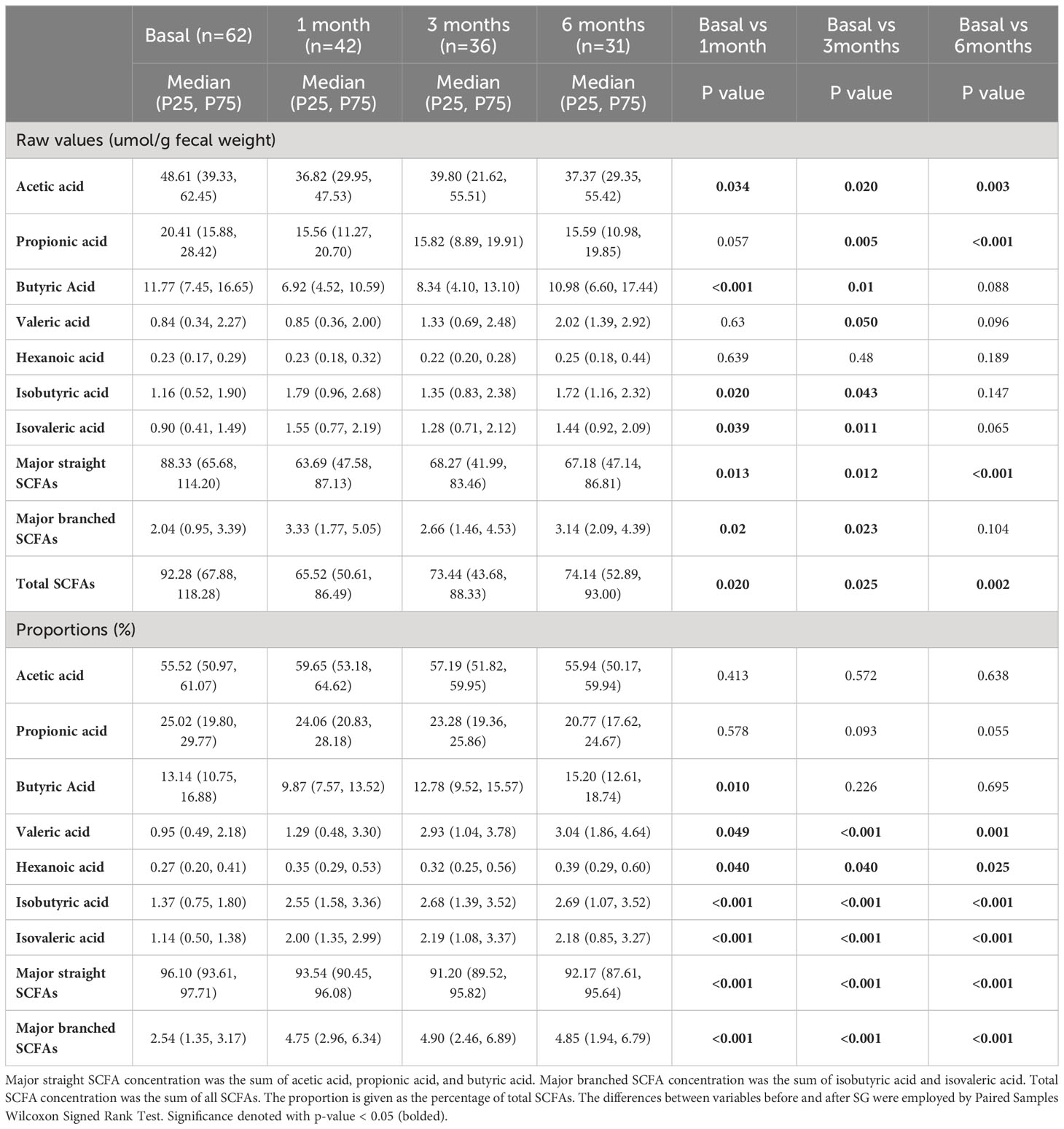
Table 2 Fecal short-chain fatty acid levels and proportions before and at 1 month, 3 months, 6 months after sleeve gastrectomy.
The proportion of each SCFA could provide additional information of the relative distribution in total SCFAs. We found that acetic acid, propionic acid and butyric acid were three major SCFAs and accounted for 55.52%, 25.02% and 13.14% of the total SCFAs in subjects before SG surgery, respectively, while the other four detected SCFAs accounted for 0.27% to 1.37% of the total SCFAs, which were overall consistent with previous reports (22, 23). After SG treatment, the proportions of acetic acid did not change significantly, and propionic acid proportions were gradually lowered, while butyric acid proportions were lowered at 1 month and rebounded at 3 and 6 months after SG, which were overall consistent with the changes in absolute concentrations. The other four minor SCFAs (including two minor straight SCFAs and two branched SCFAs) were relatively increased after SG treatment at all the 3 time points after SG. Taken together, our data demonstrate that three major SCFAs proportions were lowered, while four minor SCFAs proportions were raised at 1, 3, 6 months after SG treatment.
3.3 Correlations between changes in fecal SCFAs and clinical parameters
Figure 2 revealed the correlations between the percent changes in fecal SCFAs and clinical parameters after 6 months of SG treatment. We found that changes in propionic acid, total major straight SCFAs and total SCFAs were positively correlated with changes in serum triglycerides. Changes in acetic acid, total major straight SCFAs and total SCFAs were positively correlated with changes in serum total cholesterol.
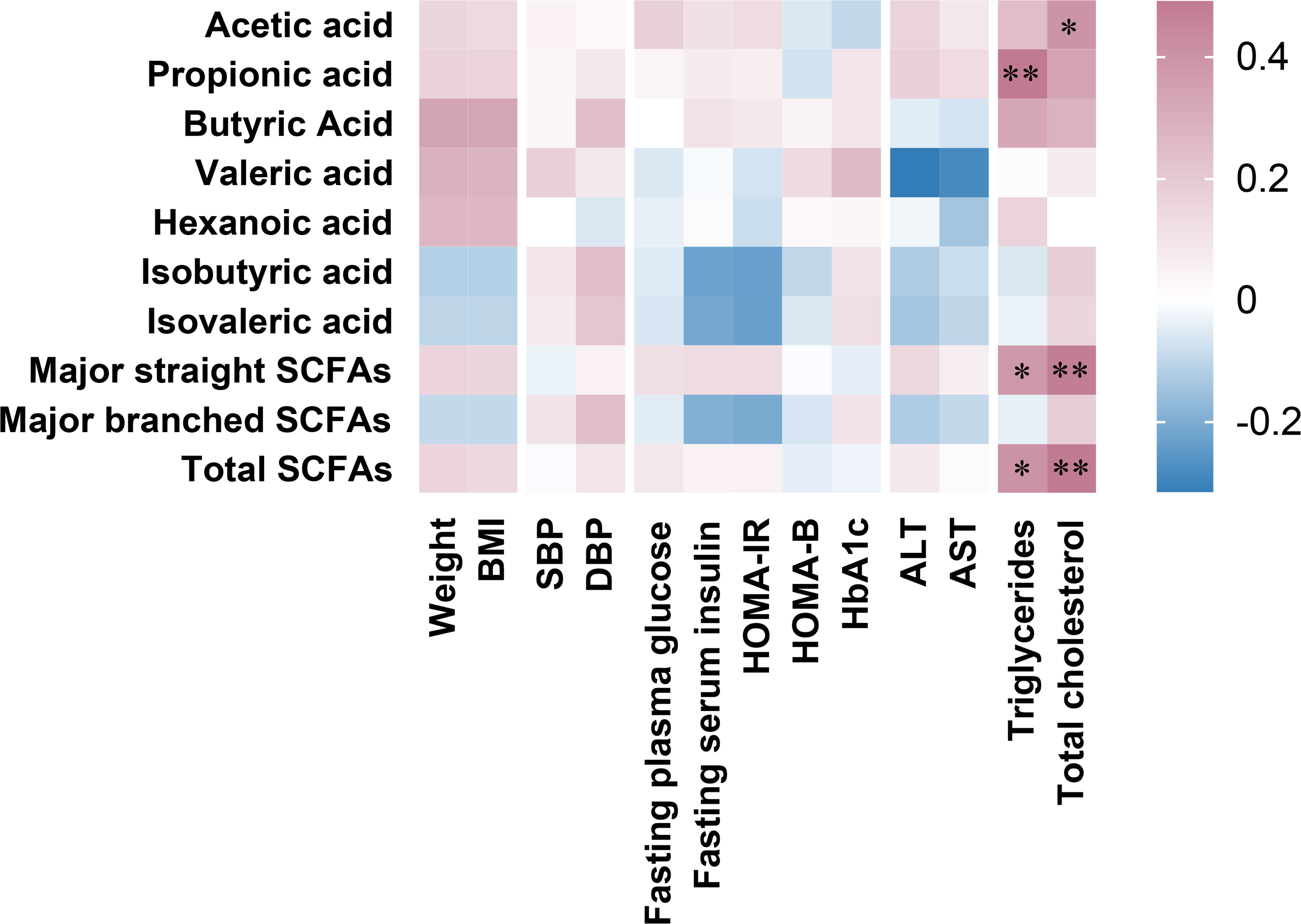
Figure 2 Associations between percent changes ((pre values-post values)/pre values*100) at 6 months after sleeve gastrectomy in fecal SCFAs and clinical parameters. Major straight SCFA concentration was the sum of acetic acid, propionic acid, and butyric acid, major branched SCFA concentration was the sum of isobutyric acid and isovaleric acid, and total SCFA concentration was the sum of all SCFAs. Spearman’s rank correlation coefficients depict positive (red) or inverse (blue) relationship with significance reported with asterisks “*” (p < 0.05) and “**” (p< 0.01).
3.4 The impact of pre-surgery fecal SCFAs on metabolic responses to SG treatment
We investigated the adjusted changes in clinical indices 6 months after SG using linear mixed model, and found that variables of obesity (Table 3), lipid profiles (Table 4), liver function (Table 5), blood pressure (Supplementary Table 1), and glucose metabolism (Supplementary Table 2) were significantly improved by SG at 6 months after surgery, indicating a sustainable metabolic improvement after SG intervention.
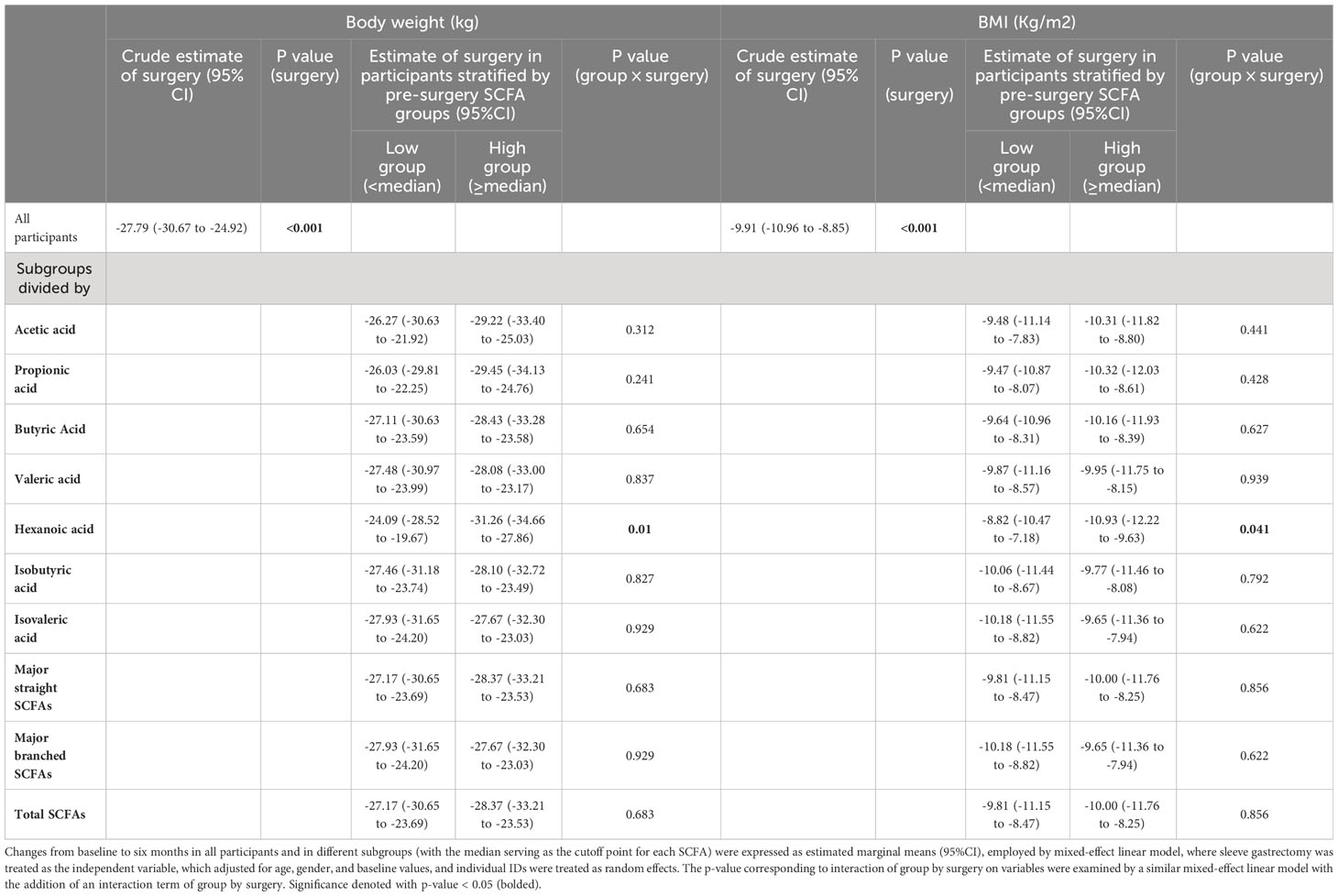
Table 3 Changes in body weight and BMI from baseline to six months after sleeve gastrectomy in all participants and in the subgroups stratified by pre-surgery SCFA levels.
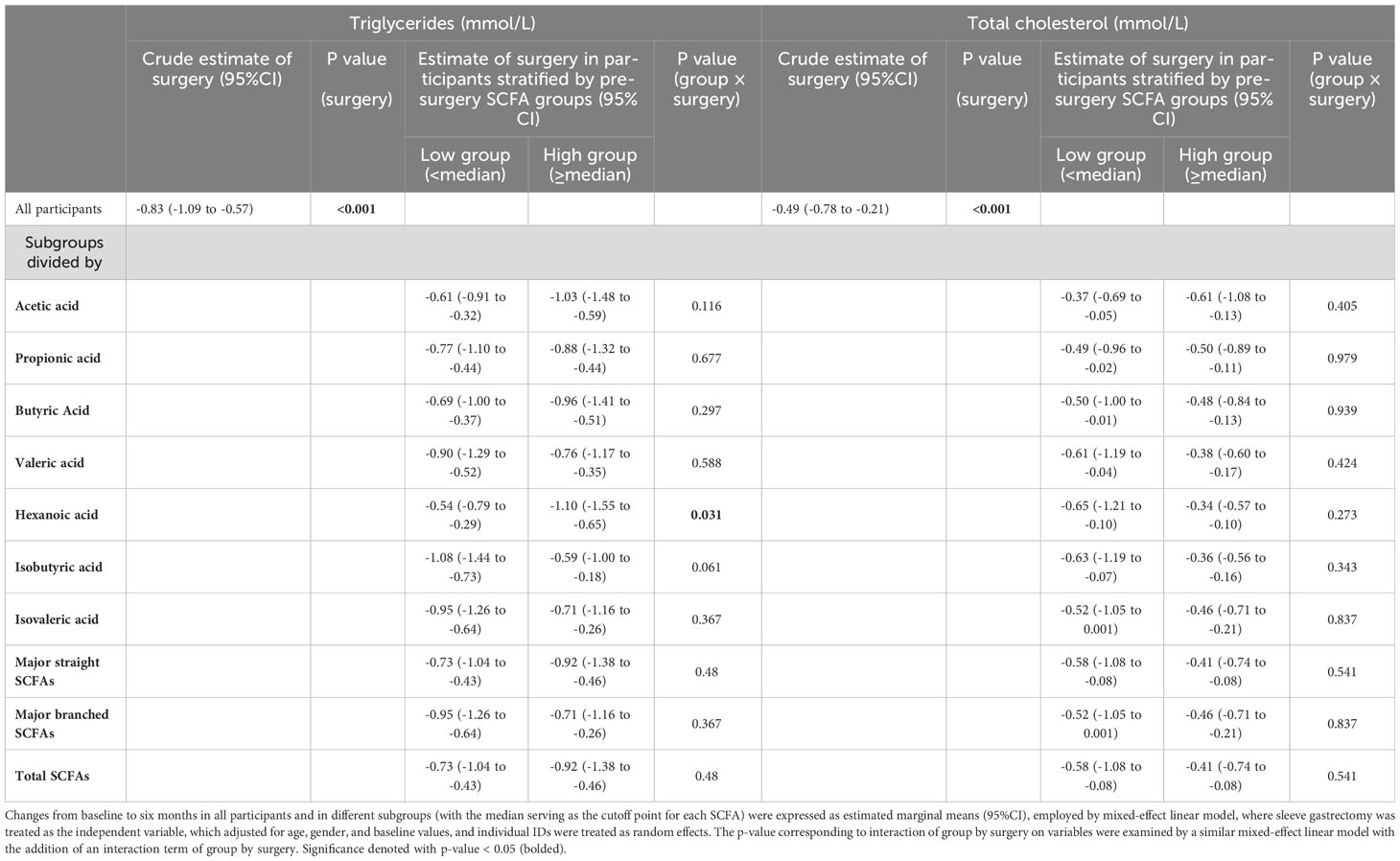
Table 4 Changes in serum triglycerides and total cholesterol from baseline to six months after sleeve gastrectomy in all participants and in the subgroups stratified by pre-surgery SCFA levels.
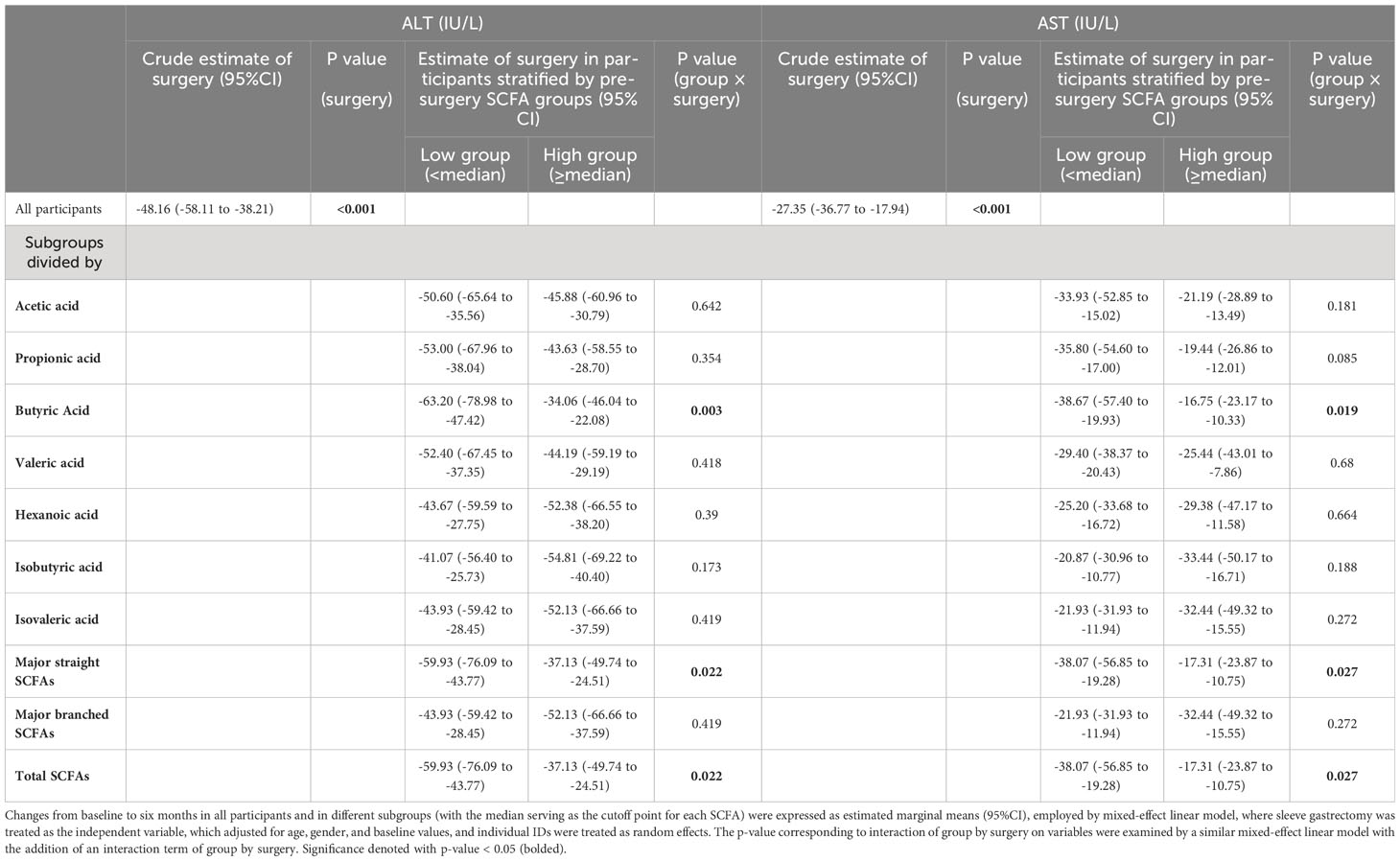
Table 5 Changes in liver function from baseline to six months after sleeve gastrectomy in all participants and in the subgroups stratified by pre-surgery SCFA levels.
To investigate whether different pre-surgery SCFAs could impact metabolic responses to SG treatment, we classified the subjects with obesity into low and high-SCFA subgroups based on the median concentrations of each SCFA. Interestingly, we found that high pre-surgery fecal hexanoic acid group showed a better effect of SG surgery on lowering body weight (low vs high -24.09 (95%CI -28.52 to -19.67) vs -31.26 (95%CI -34.66 to -27.86)kg), BMI (low vs high -8.82 (95%CI -10.47 to -7.18) vs -10.93 (95%CI -12.22 to -9.63)kg/m2) (Table 3) and serum triglycerides (low vs high -0.54 (95%CI -0.79 to -0.29) vs -1.10 (95%CI -1.55 to -0.65) mmol/L) (Table 4) than low pre-surgery fecal hexanoic acid group. Three fecal SCFA components were found to impact the effects of SG on improving liver function. Participants of lower pre-surgery fecal butyric acid or total major straight SCFAs, total SCFAs were observed a better effect of SG on lowering ALT (butyric acid, low vs high, -63.20 (95%CI -78.98 to -47.42) vs -34.06 (95%CI -46.04 to -22.08) IU/L; total major straight SCFAs, total SCFAs, low vs high -59.93(95%CI -76.09 to -43.77) vs -37.13(95%CI -49.74 to -24.51) IU/L) and AST (butyric acid, low vs high, -38.67 (95%CI -57.40 to -19.93) vs -16.75(95%CI -23.17 to -10.33) IU/L; total major straight SCFAs, total SCFAs, low vs high, -38.07 (95%CI -56.85 to -19.28) vs -17.31(95%CI -23.87 to -10.75) IU/L) (Table 5). No significance was found in the impact of baseline fecal SCFAs on blood pressure (Supplementary Table 1) or parameters of glucose metabolism (Supplementary Table 2).
4 Discussion
Metabolic benefits produced by bariatric surgery is a complex and multifactorial process, requiring studies to understand the factors involved in and impacting the therapeutic effects. The SCFAs are metabolites from fiber fermentation by gut microbiota, and contribute to the regulation of host health or diseases (25). However, whether SCFAs are affected by SG surgery and impact its therapeutic effects are largely unknown. The present study aimed to comprehensively reveal the profile changes of fecal SCFAs before and in different stages after SG surgery, and investigate the impact of fecal SCFAs’ composition on therapeutic effects of SG treatment.
SCFAs are monocarboxylic acids with one carboxyl group attached to an alkyl group, consisting of 2–6 carbons and comprise of seven types: acetic acid (C2), propionic acid (C3), butyric acid (C4), isobutyric acid (IC4), valeric acid (C5), isovaleric acid (IC5), and hexanoic acid (C6) (26). Straight SCFAs include acetic acid, propionic acid, butyric acid, valeric acid and hexanoic acid, and branched SCFAs are isobutyric acid and isovaleric acid. The most abundant straight SCFAs are acetic, propionic, and butyric acids, and are main catabolic end-products from carbohydrate bacterial fermentation (27–29). Hexanoic and valeric acids account for a small amount of fecal straight SCFAs and have not been proven to be strictly produced by the microbiota (30). Colonic fermentation of the branched-chain amino acids valine and leucine leads to the production of the branched SCFAs isobutyric and isovaleric acids (30, 31).
This study revealed for the first time the profile changes in fecal SCFAs before and in different stages (1month, 3 months and 6 months) after SG in Chinese subjects with obesity. We consistently found that the three straight SCFAs, acetate, propionate and butyrate, account for the major percentage in the gut of the subjects with obesity while after SG treatment, a decrease in the two main straight SCFAs (acetate, propionate), was detected at 3 to 6 months, which were consistent with the decreases in acetate and propionate at 4 to 12 months after surgeries in reports of the combined analysis consisting of both RYGB and SG surgery types (22, 23). Notably, butyric acids transiently decreased at 1 to 3 months after SG, but rebounded at 6 months after SG, which was different with the findings in previous studies using subjects mainly from RYGB and a small sample size of SG (22, 23). Actually, the two surgery types RYGB and SG does not have the same effects on gut microbiota in subjects with obesity (32). As butylates are derived from fiber fermentation by gut microbiota, the different impact of bariatric surgery types on gut microbiota may explain the different butyrate changes in RYGB or SG (32). Besides, an increase in the absolute or proportions of the minor SCFAs, such as isobutyric acid and isovaleric acid, were consistently observed at different time points after SG. Although few studies report the function of these minor SCFAs, our results may suggest their potential role in metabolic regulation.
We found the changes of acetic acid and propionic acid 6 months after SG were positively correlated with the changes of serum total cholesterol and triglycerides, respectively. As reported in a human study, fasting plasma acetate is positively associated with the degree of adiposity, which is interpreted as acetate increasing the source of energy that ends up being stored as lipids (33). In addition, cholesterol can be synthesized from radiolabeled acetate in rat liver (34). Therefore, the positive correlation between acetic acid and serum total cholesterol might be a result of acetate increasing the source of cholesterol biosynthesis in liver. Propionic acid has been also reported to positively correlated with total body fat, visceral fat, and subcutaneous fat in a human study (33). Another study in zebrafish reveals that sodium propionate exposure for 3 months increases triglycerides, total cholesterol and blood glucose (35). Propionic acid can promote sympathetic outflow via GPR41 (36), increasing lipolysis in WAT and enhancing VLDL-TG production by the liver (37), which support the positive relationship between changes in propionic acid and serum triglycerides in our study.
We found that high pre-surgery fecal hexanoic acid group showed a better effect of SG treatment on lowering body weight, BMI and triglycerides than low pre-surgery fecal hexanoic acid group. Hexanoic acid, a straight-chain saturated fatty acid of C6, accounts for a small amount of SCFAs and is poorly studied. In a study performed on chick embryos and HepG2 hepatocytes, hexanoic acid is found to decrease insulin and triiodothyronine-induced fatty acid synthase (FASN) expression and activity (38), which is a key enzyme in de novo lipogenesis (39). In another study performed in the HepG2 hepatoma cell line, hexanoic acid promoted basal and insulin-induced phosphorylation of the Akt-mTOR axis, maintaining a balance of lipid metabolism and potentially constituting an effective tool to manage liver steatosis and hepatic insulin resistance (40). A human study evaluated the association between fecal fungi and obesity, and found that Eurotiomycetes, which could colonize in the gastrointestinal tract of both obese and non-obese subjects, might modify metabolic phenotype in obese subjects (41). Obese subjects with Eurotiomycetes <1% had a more pronounced dyslipidemic profile, increased fasting triglycerides, increased total cholesterol and increased fasting hyperinsulinemia, compared with obese subjects with Eurotiomycetes >1%. The authors also performed a plasma metabolomics profile and found that relative abundance of Eurotiomycetes was positively associated with plasma hexanoic acid. Although it remains to be confirmed whether hexanoic acids are catabolic end-products from Eurotiomycetes and whether hexanoic acids directly modify the metabolic phenotype in obese subjects, these findings support that hexanoic acid might be a potential factor impacting SG’s effects on lowering body weight, BMI and triglycerides, and more researches on the direct effects of hexanoic acid on body weight and lipid metabolism are needed.
When it comes to the impact of pre-surgery fecal SCFAs on the liver function improvements after SG surgery, low baseline fecal butyric acid was found to have a better effect of SG on improving liver function. Fasting plasma butyrate has been proven to be positively associated with the degree of adiposity, hepatic fat and an increase in hepatic de novo lipogenesis in adolescents with obesity (33). In the liver, butyrate can be converted to acetyl-CoA, which are substrates for hepatic de novo lipogenesis (42). Hepatic fat is closely related with inflammation and hepatocyte damage (43). These findings suggest that subjects with obesity having low baseline fecal butyric acid might have a reduced potential of hepatic fat production, possibly making them more responsive to SG treatment in improving liver function. However, there are also studies reporting that butyric acid attenuated steatohepatitis (44). Daily intragastric administration with sodium butyrate at 200 mg/kg body weight for 8 weeks lowers the levels of triglycerides and cholesterol in liver and improve liver function in mice fed a high-fat diet (44). Therefore, butyric acid can be beneficial after long term and high dose application, and the mechanism underlying fecal butyrate influencing liver function improvement after bariatric surgery remains to be researched.
In our study, we revealed for the first time the impact of fecal SCFAs on therapeutic effects of SG surgery in subjects with obesity. However, there remains unresolved questions to be answered: 1) the range of fecal SCFAs that predicts a better therapeutic effect of SG should be determined in larger population; 2) only subjects with obesity undergoing SG were included in our study, and whether fecal SCFAs have an influence on therapeutic effects of RYGB is unknown, which is also an interesting topic.
The results presented in this study should also be interpreted with some limitations. For example, our study followed up to 6 months after SG surgery, and the long-term (one year or more) effects could not be determined. Second, in the current study, eating preferences or habits of participants were lacking, which, however, could be an important modifier to bariatric surgery for investigation. Third, the SCFAs levels in the blood could reflect the absorption of SCFAs from intestine and the utilization of SCFAs in vivo and likewise affect the SG surgery but were not examined in our study, which also warrants a future comprehensive investigation. Despite of these limitations, our findings may shed lights on the mechanistic researches underlying SCFAs influencing therapeutic effects of bariatric surgery. In addition, applying baseline measurement of fecal SCFAs may help to predict or assess the therapeutic capability of bariatric surgery in patients with obesity.
5 Conclusion
In conclusion, we comprehensively revealed the profile changes of fecal SCFAs before and in different stages after SG surgery, uncovered the positive correlations of acetate and propionate changes with lipid post-surgery changes, and suggested that baseline fecal butyric acid and hexanoic acid have an impact on the effect of SG surgery on metabolic improvement. To our best knowledge, this is the first study assessing the impact of fecal SCFAs on metabolic benefits after SG surgery, providing important implications for personalized management of obesity and metabolic disorders by SG surgery.
Data availability statement
Datasets generated during the current study are available from the corresponding author upon reasonable request.
Ethics statement
The studies involving humans were approved by Institutional Review Board of the Ruijin Hospital. The studies were conducted in accordance with the local legislation and institutional requirements. The participants provided their written informed consent to participate in this study.
Author contributions
CS: Investigation, Methodology, Writing – original draft. YRC: Investigation, Methodology, Writing – review & editing. QW: Investigation, Methodology, Writing – review & editing. YS: Data curation, Methodology, Writing – review & editing. HL: Resources, Writing – review & editing. MN: Resources, Writing – review & editing. YFC: Resources, Writing – review & editing. LZ: Resources, Writing – review & editing. JJ: Resources, Writing – review & editing. XY: Resources, Writing – review & editing. YYZ: Resources, Writing – review & editing. XW: Resources, Writing – review & editing. YY: Resources, Writing – review & editing. MY: Resources, Writing – review & editing. NY: Resources, Writing – review & editing. ZC: Resources, Writing – review & editing. YFZ: Writing – review & editing. WG: Writing – review & editing. WW: Writing – review & editing. GN: Writing – review & editing. JW: Writing – review & editing. SZ: Conceptualization, Project administration, Writing – review & editing, Supervision. JH: Conceptualization, Project administration, Writing – review & editing, Supervision. RL: Conceptualization, Project administration, Writing – original draft, Supervision.
Funding
The author(s) declare financial support was received for the research, authorship, and/or publication of this article. This work was supported by grants from the National Natural Science Foundation of China (92157204, 82250901, 81930021, 82070863, 82000815), the National Key Research and Development Program of China (2022YFC2505201 JW and 2021YFA1301103 GN), Shanghai Municipal Education Commission-Gaofeng Clinical Medicine Grant Support (20161306 and 20171903 Round 2), Innovative research team of high-level local universities in Shanghai, and Program of Shanghai Academic/Technology Research Leader (23XD1422400 RL).
Conflict of interest
The authors declare that the research was conducted in the absence of any commercial or financial relationships that could be construed as a potential conflict of interest.
Publisher’s note
All claims expressed in this article are solely those of the authors and do not necessarily represent those of their affiliated organizations, or those of the publisher, the editors and the reviewers. Any product that may be evaluated in this article, or claim that may be made by its manufacturer, is not guaranteed or endorsed by the publisher.
Supplementary material
The Supplementary Material for this article can be found online at: https://www.frontiersin.org/articles/10.3389/fendo.2023.1277035/full#supplementary-material
References
1. Castellana M, Procino F, Biacchi E, Zupo R, Lampignano L, Castellana F, et al. Roux-en-Y gastric bypass vs sleeve gastrectomy for remission of type 2 diabetes. J Clin Endocrinol Metab (2021) 106:922–33. doi: 10.1210/clinem/dgaa737
2. Hofsø D, Fatima F, Borgeraas H, Birkeland KI, Gulseth HL, Hertel JK, et al. Gastric bypass versus sleeve gastrectomy in patients with type 2 diabetes (Oseberg): a single-centre, triple-blind, randomised controlled trial. Lancet Diabetes Endocrinol (2019) 7:912–24. doi: 10.1016/S2213-8587(19)30344-4
3. Singh P, Adderley NJ, Hazlehurst J, Price M, Tahrani AA, Nirantharakumar K, et al. Prognostic models for predicting remission of diabetes following bariatric surgery: A systematic review and meta-analysis. Diabetes Care (2021) 44:2626–41. doi: 10.2337/dc21-0166
4. Gutiérrez-Repiso C, Garrido-Sánchez L, Alcaide-Torres J, Cornejo-Pareja I, Ocaña-Wilhelmi L, García-Fuentes E, et al. Predictive role of gut microbiota in weight loss achievement after bariatric surgery. J Am Coll Surg (2022) 234:861–71. doi: 10.1097/XCS.0000000000000145
5. Bäckhed F, Ding H, Wang T, Hooper LV, Koh GY, Nagy A, et al. The gut microbiota as an environmental factor that regulates fat storage. Proc Natl Acad Sci USA (2004) 101:15718–23. doi: 10.1073/pnas.0407076101
6. Cox AJ, West NP, Cripps AW. Obesity, inflammation, and the gut microbiota. Lancet Diabetes Endocrinol (2015) 3:207–15. doi: 10.1016/S2213-8587(14)70134-2
7. Turnbaugh PJ, Hamady M, Yatsunenko T, Cantarel BL, Duncan A, Ley RE, et al. A core gut microbiome in obese and lean twins. Nature (2009) 457:480–4. doi: 10.1038/nature07540
8. Le Chatelier E, Nielsen T, Qin J, Prifti E, Hildebrand F, Falony G, et al. Richness of human gut microbiome correlates with metabolic markers. Nature (2013) 500:541–6. doi: 10.1038/nature12506
9. Cotillard A, Kennedy SP, Kong LC, Prifti E, Pons N, Le Chatelier E, et al. Dietary intervention impact on gut microbial gene richness. Nature (2013) 500:585–8. doi: 10.1038/nature12480
10. Liu R, Hong J, Xu X, Feng Q, Zhang D, Gu Y, et al. Gut microbiome and serum metabolome alterations in obesity and after weight-loss intervention. Nat Med (2017) 23:859–68. doi: 10.1038/nm.4358
11. Topping DL, Clifton PM. Short-chain fatty acids and human colonic function: roles of resistant starch and nonstarch polysaccharides. Physiol Rev (2001) 81:1031–64. doi: 10.1152/physrev.2001.81.3.1031
12. Lin HV, Frassetto A, Kowalik EJ Jr., Nawrocki AR, Lu MM, Kosinski JR, et al. Butyrate and propionate protect against diet-induced obesity and regulate gut hormones via free fatty acid receptor 3-independent mechanisms. PloS One (2012) 7:e35240. doi: 10.1371/journal.pone.0035240
13. Hernández MAG, Canfora EE, Jocken JWE, Blaak EE. The short-chain fatty acid acetate in body weight control and insulin sensitivity. Nutrients (2019) 11:1943. doi: 10.3390/nu11081943
14. Brahe LK, Astrup A, Larsen LH. Is butyrate the link between diet, intestinal microbiota and obesity-related metabolic diseases? Obes Rev (2013) 14:950–9. doi: 10.1111/obr.12068
15. Bloemen JG, Olde Damink SW, Venema K, Buurman WA, Jalan R, Dejong CH. Short chain fatty acids exchange: Is the cirrhotic, dysfunctional liver still able to clear them? Clin Nutr (2010) 29:365–9. doi: 10.1016/j.clnu.2009.10.002
16. Aschenbach JR, Kristensen NB, Donkin SS, Hammon HM, Penner GB. Gluconeogenesis in dairy cows: the secret of making sweet milk from sour dough. IUBMB Life (2010) 62:869–77. doi: 10.1002/iub.400
17. Chambers ES, Viardot A, Psichas A, Morrison DJ, Murphy KG, Zac-Varghese SE, et al. Effects of targeted delivery of propionate to the human colon on appetite regulation, body weight maintenance and adiposity in overweight adults. Gut (2015) 64:1744–54. doi: 10.1136/gutjnl-2014-307913
18. den Besten G, Bleeker A, Gerding A, van Eunen K, Havinga R, van Dijk TH, et al. Short-chain fatty acids protect against high-fat diet-induced obesity via a PPARγ-dependent switch from lipogenesis to fat oxidation. Diabetes (2015) 64:2398–408. doi: 10.2337/db14-1213
19. Bayham BE, Greenway FL, Bellanger DE, O'Neil CE. Early resolution of type 2 diabetes seen after Roux-en-Y gastric bypass and vertical sleeve gastrectomy. Diabetes Technol Ther (2012) 14:30–4. doi: 10.1089/dia.2011.0151
20. Lupoli R, Lembo E, Saldalamacchia G, Avola CK, Angrisani L, Capaldo B. Bariatric surgery and long-term nutritional issues. World J Diabetes (2017) 8:464–74. doi: 10.4239/wjd.v8.i11.464
21. McCracken E, Wood GC, Prichard W, Bistrian B, Still C, Gerhard G, et al. Severe anemia after Roux-en-Y gastric bypass: a cause for concern. Surg Obes Relat Dis (2018) 14:902–9. doi: 10.1016/j.soard.2018.03.026
22. Meijer JL, Roderka MN, Chinburg EL, Renier TJ, McClure AC, Rothstein RI, et al. Alterations in fecal short-chain fatty acids after bariatric surgery: relationship with dietary intake and weight loss. Nutrients (2022) 14:4243. doi: 10.3390/nu14204243
23. Farup PG, Valeur J. Changes in faecal short-chain fatty acids after weight-loss interventions in subjects with morbid obesity. Nutrients (2020) 12:802. doi: 10.3390/nu12030802
24. Du Y, Zhang J, Chen G, Sun Z. Formulation and interpretation of the chinese guidelines for surgical treatment of obesity and type 2 diabetes mellitus. Biosci Trends (2021) 15:299–304. doi: 10.5582/bst.2021.01287
25. Canfora EE, Jocken JW, Blaak EE. Short-chain fatty acids in control of body weight and insulin sensitivity. Nat Rev Endocrinol (2015) 11:577–91. doi: 10.1038/nrendo.2015.128
26. Hatanaka K, Shirahase Y, Yoshida T, Kono M, Toya N, Sakasegawa SI, et al. Enzymatic measurement of short-chain fatty acids and application in periodontal disease diagnosis. PloS One (2022) 17:e0268671. doi: 10.1371/journal.pone.0268671
27. Macfarlane GT, Macfarlane S. Bacteria, colonic fermentation, and gastrointestinal health. J AOAC Int (2012) 95:50–60. doi: 10.5740/jaoacint.SGE_Macfarlane
28. Schwiertz A, Taras D, Schäfer K, Beijer S, Bos NA, Donus C, et al. Microbiota and SCFA in lean and overweight healthy subjects. Obes (Silver Spring) (2010) 18:190–5. doi: 10.1038/oby.2009.167
29. Fernandes J, Su W, Rahat-Rozenbloom S, Wolever TM, Comelli EM. Adiposity, gut microbiota and faecal short chain fatty acids are linked in adult humans. Nutr Diabetes (2014) 4:e121. doi: 10.1038/nutd.2014.23
30. Rios-Covian D, González S, Nogacka AM, Arboleya S, Salazar N, Gueimonde M, et al. An overview on fecal branched short-chain fatty acids along human life and as related with body mass index: associated dietary and anthropometric factors. Front Microbiol (2020) 11:973. doi: 10.3389/fmicb.2020.00973
31. Gutiérrez-Repiso C, Moreno-Indias I, Tinahones FJ. Shifts in gut microbiota and their metabolites induced by bariatric surgery. Impact of factors shaping gut microbiota on bariatric surgery outcomes. Rev. Endocr Metab Disord (2021) 22:1137–56. doi: 10.1007/s11154-021-09676-8
32. Chen G, Zhuang J, Cui Q, Jiang S, Tao W, Chen W, et al. Two bariatric surgical procedures differentially alter the intestinal microbiota in obesity patients. Obes Surg (2020) 30:2345–61. doi: 10.1007/s11695-020-04494-4
33. Goffredo M, Mass K, Parks EJ, Wagner DA, McClure EA, Graf J, et al. Role of gut microbiota and short chain fatty acids in modulating energy harvest and fat partitioning in youth. J Clin Endocrinol Metab (2016) 101:4367–76. doi: 10.1210/jc.2016-1797
34. Cornforth JW, Hunter GD, Popjak G. The biosynthesis of cholesterol from acetate. Arch Biochem Biophys (1953) 42:481–2. doi: 10.1016/0003-9861(53)90381-6
35. Xu YX, Zhang SH, Luan JL, Fu ZH, Sun MZ, Zhao X, et al. The food preservative sodium propionate induces hyperglycaemic state and neurological disorder in zebrafish. Neurotoxicol Teratol (2022) 93:107123. doi: 10.1016/j.ntt.2022.107123
36. Kimura I, Inoue D, Maeda T, Hara T, Ichimura A, Miyauchi S, et al. Short-chain fatty acids and ketones directly regulate sympathetic nervous system via G protein-coupled receptor 41 (GPR41). Proc Natl Acad Sci U.S.A. (2011) 108:8030–5. doi: 10.1073/pnas.1016088108
37. Geerling JJ, Boon MR, Kooijman S, Parlevliet ET, Havekes LM, Romijn JA, et al. Sympathetic nervous system control of triglyceride metabolism: novel concepts derived from recent studies. J Lipid Res (2014) 55:180–9. doi: 10.1194/jlr.R045013
38. Akpa MM, Point F, Sawadogo S, Radenne A, Mounier C. Inhibition of insulin and T3-induced fatty acid synthase by hexanoate. Lipids (2010) 45:997–1009. doi: 10.1007/s11745-010-3465-5
39. Strable MS, Ntambi JM. Genetic control of de novo lipogenesis: role in diet-induced obesity. Crit Rev Biochem Mol Biol (2010) 45:199–214. doi: 10.3109/10409231003667500
40. Rial SA, Ravaut G, Malaret TB, Bergeron KF, Mounier C. Hexanoic, octanoic and decanoic acids promote basal and insulin-induced phosphorylation of the akt-mTOR axis and a balanced lipid metabolism in the hepG2 hepatoma cell line. Molecules (2018) 23:2315. doi: 10.3390/molecules23092315
41. Mar Rodríguez M, Pérez D, Javier Chaves F, Esteve E, Marin-Garcia P, Xifra G, et al. Obesity changes the human gut mycobiome. Sci Rep (2015) 5:14600. doi: 10.1038/srep14600
42. den Besten G, van Eunen K, Groen AK, Venema K, Reijngoud DJ, Bakker BM. The role of short-chain fatty acids in the interplay between diet, gut microbiota, and host energy metabolism. J Lipid Res (2013) 54:2325–40. doi: 10.1194/jlr.R036012
43. Friedman SL, Neuschwander-Tetri BA, Rinella M, Sanyal AJ. Mechanisms of NAFLD development and therapeutic strategies. Nat Med (2018) 24:908–22. doi: 10.1038/s41591-018-0104-9
Keywords: bariatric surgery, short chain fatty acids, obesity, weight loss, sleeve gastrectomy
Citation: Shen C, Chen Y, Wang Q, Sun Y, Lin H, Ni M, Chen Y, Zhang L, Jin J, Ying X, Zou Y, Wang X, Ye Y, Yuan M, Yin N, Cao Z, Zhang Y, Gu W, Wang W, Ning G, Wang J, Zhao S, Hong J and Liu R (2023) Fecal short chain fatty acids modify therapeutic effects of sleeve gastrectomy. Front. Endocrinol. 14:1277035. doi: 10.3389/fendo.2023.1277035
Received: 13 August 2023; Accepted: 06 October 2023;
Published: 07 November 2023.
Edited by:
Evan P. Nadler, Children’s National Hospital, United StatesReviewed by:
Atte Johannes Von Wright, University of Eastern Finland, FinlandYina Huang, Anhui Polytechnic University, China
Copyright © 2023 Shen, Chen, Wang, Sun, Lin, Ni, Chen, Zhang, Jin, Ying, Zou, Wang, Ye, Yuan, Yin, Cao, Zhang, Gu, Wang, Ning, Wang, Zhao, Hong and Liu. This is an open-access article distributed under the terms of the Creative Commons Attribution License (CC BY). The use, distribution or reproduction in other forums is permitted, provided the original author(s) and the copyright owner(s) are credited and that the original publication in this journal is cited, in accordance with accepted academic practice. No use, distribution or reproduction is permitted which does not comply with these terms.
*Correspondence: Shaoqian Zhao, emhhb3NxMTk2M0AxNjMuY29t; Jie Hong, aG9uZ2ppZUBtZWRtYWlsLmNvbS5jbg==; Ruixin Liu, cnhsaXVAc2hzbXUuZWR1LmNu
†Present address: Ling Zhang, Department of Critical Care Medicine, Ruijin Hospital, Shanghai Jiao Tong University School of Medicine, Shanghai, China
‡These authors have contributed equally to this work and share first authorship