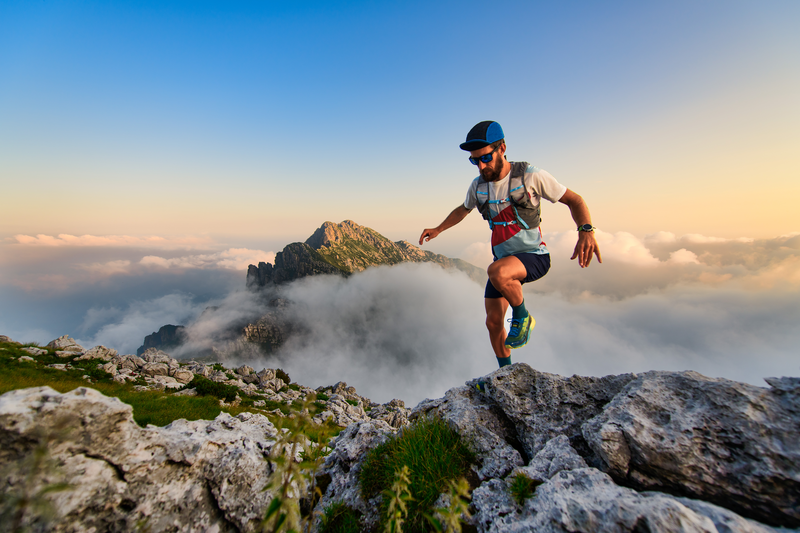
94% of researchers rate our articles as excellent or good
Learn more about the work of our research integrity team to safeguard the quality of each article we publish.
Find out more
ORIGINAL RESEARCH article
Front. Endocrinol. , 24 March 2023
Sec. Experimental Endocrinology
Volume 14 - 2023 | https://doi.org/10.3389/fendo.2023.1099832
This article is part of the Research Topic Environmental Regulation of Feeding, Growth, and Reproduction in Fish: Influence of Nutrition and Physical Parameters on the Endocrine System View all 6 articles
LPXRFa, also known as gonadotropin-inhibitory hormone (GnIH), and kisspeptin (Kiss) are two major hypothalamic peptides that modulate the reproductive axis of vertebrates, including teleosts. However, little information is available regarding the actions of nutritional status on the regulation of these two neuroendocrine systems in fish. Herein, we assessed the effects of starvation and refeeding on the expression of lpxrfa, kiss2 and their receptors (lpxrfa-r and kiss2r respectively) at the brain-pituitary level of half-smooth tongue sole (Cynoglossus semilaevis). Food deprivation for 4 weeks induced a rise in brain lpxrfa as well as brain and pituitary lpxrfa-r mRNA levels, and refeeding restored brain lpxrfa and lpxrfa-r expression back to normal. However, pituitary lpxrfa-r mRNA levels still remained high after 1 week of refeeding. Neither lpxrfa nor kiss2 transcripts in the pituitary were altered by fasting, but their mRNA levels increased significantly after 1 week of refeeding, and declined back to the control levels after 2 weeks of refeeding. None of brain kiss2 and kiss2r along with pituitary kiss2r transcripts were modified by the nutritional status. In summary, our results revealed an interaction between energy status and the elements of LPXRFa and Kiss systems in the brain-pituitary axis of half-smooth tongue sole. Food deprivation and refeeding differentially regulated the two systems, which provided additional evidence for the involvement of the LPXRFa and Kiss systems in the regulation of reproduction by energy balance in non-mammalian species.
In 2000, a novel hypothalamic neuropeptide was discovered in the Japanese quail, and was termed gonadotropin-inhibitory hormone (GnIH) based on its ability to inhibit gonadotropin release (1). Subsequently, its homologues have been identified in various vertebrates, including fish, amphibians, reptiles, birds and mammals (2–4). GnIH is also called LPXRFa in teleosts or RFamide-related peptide (RFRP) in mammals, and its precursor encompasses two, three or four putative/mature peptides depending on the species, which generally possess a common C-terminal LPXRFamide (X = L or Q) motif (3–5). There is compelling evidence that GnIH exerts an inhibitory effect on each level of the brain-pituitary-gonadal axis via its cognate receptor GPR147 (namely GnIH-R or LPXRFa-R), and it also participates in stress response, biological rhythms and social behaviors (6–8). Three different GPR147 types are found in some Cypriniform species, but only one exists in other vertebrates investigated so far (4). The molecular mechanisms of GnIH actions have been investigated in mammals (9–12), chicken (13), Nile tilapia (14), orange-spotted grouper (15), zebrafish (16), half-smooth tongue sole (17), chub mackerel (18), and European sea bass (19). It is of note that activation of GnIH receptor can interfere with signaling pathways induced by other neuroendocrine factors (9–13, 16, 19–21).
Following the discovery of GnIH, another hypothalamic neuropeptide kisspeptin (Kiss) has been recognized as an essential stimulator of reproduction in mammals (22, 23). In contrast to most mammals in which only one kiss and one receptor genes have been characterized, up to three kiss genes (kiss1, kiss2 and kiss3) and four receptor genes (kissr1, kissr2, kissr3 and kissr4) have been identified in non-mammalian species (24), which increases the complexity of the Kiss/KissR systems involved in the control of reproduction (25, 26). In most teleost species, both kiss1 and kiss2 along with kissr2 and kissr3 genes have been reported, whereas only the kiss2/kissr2 system was identified in other fish species, including half-smooth tongue sole (26, 27). There is considerable evidence supporting that Kiss exerts a stimulatory action on teleost reproduction, as in mammals (26–28). However, recent studies on gene knockout of kiss and/or kissr in zebrafish and medaka revealed that the Kiss/KissR system is dispensable for normal reproduction (29–32). Thus, much more mutant studies in various fish species are still required to clarify the reproductive role of the Kiss/KissR system in teleost.
A close association between energy balance and reproduction has been documented, and various hypothalamic neuropeptides are involved in the regulation of these two critical physiological processes, either directly or indirectly (33, 34). For example, LPXRFa stimulated food intake in chicks (35), Pekin ducks (36), sheep (37), mice (37), rats (38), jerboa (39), and cynomolgus monkeys (37). Conversely, Kiss reduced appetite in rats (40), mice (41, 42) and jerboa (39). Such comparative studies have not yet been performed in teleosts. In addition, hypothalamic lpxrfa mRNA levels were increased during depressed food intake in heat-exposed chicks (43). However, food deprivation resulted in a decrease in the number of LPXRFa-immunoreactive neurons in the hypothalamus of a female songbird, the zebra finch (44). Fasting had no effect on the number of hypothalamic LPXRFa-immunoreactive cell bodies or lpxrfa mRNA expression in zebra finch males (45). Gonadal lpxrfa mRNA levels did not differ between fasted and control males, either (45). Similarly, no changes in hypothalamic lpxrfa transcripts were noticed in hens maintained on a diet restricted to 50% of ad libitum feeding, compared to control hens allowed free access to food for 7 days (46). To the best of our knowledge, only one report is available in fish regarding the effect of feeding status on the LPXRFa/LPXRFa-R system (47), in which fasting increased lpxrfa mRNA levels in the brains of wild-type zebrafish females and Casper zebrafish males, respectively.
In addition, Kiss has emerged as a molecular switch between reproduction and energy homeostasis in vertebrates. Fasting induced a decline in kiss1 and kissr1 mRNA levels in the hypothalamus of mice (48). Interestingly, food deprivation led to a concomitant increase in hypothalamic kissr1 and decrease in kiss1 mRNA levels in prepubertal rats (49). On the contrary, starvation stimulated hypothalamic mRNA levels of kiss2 and kissr2 in Senegalese sole (Solea senegalensis) (50), and up-regulated kiss2 and kissr2_v1 expression in the hypothalamus of pejerrey (Odontesthes bonariensis) (51). Taken together, the molecular mechanisms mediating the effects of negative energy balance on reproduction may differ among various species, which merits further studies (26, 52).
Using the half-smooth tongue sole (Cynoglossus semilaevis) as a model, we have previously cloned the full-length cDNA sequences of lpxrfa, lpxrfa-r, kiss2 and kiss2r (also called kissr2), and provided evidence for their implication in the control of reproduction and the possible signaling pathways elicited by LPXRFa and Kiss2 peptides as well as their interaction on cell signaling (17, 20, 21, 53–56). Given that the way energy balance affects the reproductive axis is still poorly understood in fish, this study aimed to evaluate the effects of nutritional status on the transcript levels of both LPXRFa/LPXRFa-R and Kiss2/Kiss2R systems at the brain-pituitary levels of half-smooth tongue sole.
Approximately 2-year-old female half-smooth tongue soles were purchased from a local fishery (Qingdao, China), and maintained in an indoor concrete tank with recirculating seawater (dissolved oxygen > 5 mg/L, pH 7.8–8.2, salinity 27–31 ppt, and water temperature 24–26°C). Fish were exposed to a cyclical photoperiod (12L:12D) and fed to satiation twice daily as described in detail previously (57).
In order to investigate the effects of nutritional status on mRNA levels of lpxrfa, kiss2 and their receptors, we compared two groups of half-smooth tongue sole, one under normal feeding condition, and the other submitted to starvation followed by refeeding. The experiment was previously performed (57) where half-smooth tongue sole females with an average body weight of 530 g were divided into two groups: one (control group) was fed to satiation twice daily as mentioned above, and the other (starved group) was fasted for 4 weeks and then refed to satiation twice daily for 2 weeks. Brain and pituitary were collected from each group at 2, 4, 5 and 6 weeks, respectively, frozen in liquid nitrogen and stored in -80°C freezer. The same cDNA samples, which were used to detect spx2 gene in our previous study (57), were used to analyze lpxrfa, lpxrfa-r, kiss2, and kiss2r mRNA levels in the present study.
Total RNA from the brain and pituitary was isolated and reverse transcribed to cDNAs which were used as templates for qPCR analysis of lpxrfa, lpxrfa-r, kiss2 and kiss2r in this study. The PCR amplification was carried out on Mastercycler® ep realplex Real-time PCR System (Eppendorf), and the thermal cycling parameters were as follows: 95°C for 30 s, and 40 cycles of 95°C for 5 s and 60°C for 20 s. Data were calculated by the comparative Ct method using 18s as a reference gene (20). The specific primers and amplification size values for each gene are shown in Table 1.
The results were analyzed by Student’s t-test using SPSS17.0, and are presented as mean ± SEM. Differences were considered statistically significant when p < 0.05.
As shown in Figure 1A, there was no significant changes in the brain lpxrfa mRNA levels after 2 weeks of food deprivation when compared to the control group. However, evident increase in the brain lpxrfa expression was observed after 4 weeks of starvation (Figure 1A). The brain lpxrfa mRNA levels of starved fish were not significantly different from the corresponding controls during the refeeding period (Figure 1A). The pituitary lpxrfa gene in fish that fasted for 2-4 weeks displayed similar expression profiles compared with normal fed animals (Figure 1B). The pituitary lpxrfa transcripts displayed an evident increase at 5 weeks (1 week after refeeding), and dropped back to the levels of the control fish at 6 weeks (Figure 1B).
Figure 1 Effects of nutritional status on the brain (A) and pituitary (B) lpxrfa mRNA levels in half-smooth tongue sole. Data were normalized against 18s transcripts and are presented as mean ± SEM (n = 4). A star indicates significant difference between fed and starved/refed groups (p < 0.05).
Fasting for 4 weeks promoted brain lpxrfa-r mRNA levels, and brain lpxrfa-r expression returned to basal levels after refeeding for 1 week and 2 weeks (Figure 2A). In the pituitary (Figure 2B), lpxrfa-r mRNA levels increased markedly after starvation for 4 weeks, still keeping high at 5 weeks, and declined to the levels of control group at 6 weeks (2 weeks after refeeding).
Figure 2 Effects of nutritional status on the brain (A) and pituitary (B) lpxrfa-r mRNA levels in half-smooth tongue sole. Data were normalized against 18s transcripts and are presented as mean ± SEM (n = 4). A star indicates significant difference between fed and starved/refed groups (p < 0.05).
As shown in Figure 3A, neither food deprivation nor refeeding altered brain kiss2 mRNA levels. Similarly, pituitary kiss2 mRNA levels did not show any significant changes after fasting for 2 weeks or 4 weeks. However, pituitary kiss2 transcripts increased markedly after 1 week of refeeding, and there were no significant differences between the two groups after 2 weeks of refeeding (Figure 3B).
Figure 3 Effects of nutritional status on the brain (A) and pituitary (B) kiss2 mRNA levels in half-smooth tongue sole. Data were normalized against 18s transcripts and are presented as mean ± SEM (n = 4). A star indicates significant difference between fed and starved/refed groups (p < 0.05).
No apparent differences between the two groups in the expression of brain kiss2r were observed during the starvation and refeeding periods (Figure 4A). Similar results were obtained for pituitary kiss2r transcripts, although a tendency of increase was noticed at 4 weeks and 5 weeks with their mean values not statistically different from the corresponding controls (Figure 4B).
Figure 4 Effects of nutritional status on the brain (A) and pituitary (B) kiss2r mRNA levels in half-smooth tongue sole. Data were normalized against 18s transcripts and are presented as mean ± SEM (n = 4). A star indicates significant difference between fed and starved/refed groups (p < 0.05).
Reproduction is tightly coupled to metabolic status, and food restriction disturbs the reproductive axis by altering the signaling of some hormones or neuropeptides (34, 58, 59). It has been well demonstrated that both LPXRFa and Kiss peptides play a key role in the regulation of reproductive axis at multiple levels in vertebrates, including fish. However, the link between energy balance and reproduction via these two neuropeptides is still largely unknown (2, 26, 27, 52, 60). In the current study, we evaluated the effects of nutritional status on the transcript levels of the elements of both LPXRFa/LPXRFa-R and Kiss2/Kiss2R systems at the brain-pituitary levels of half-smooth tongue sole.
Our results showed that starvation stimulated mRNA levels of lpxrfa and lpxrfa-r in the brain along with pituitary lpxrfa-r, with no effects on pituitary lpxrfa expression in half-smooth tongue sole. Interestingly, fasting increased the brain lpxrfa transcripts in wild-type zebrafish females, but not in males (47). However, brain lpxrfa expression was higher in fasted Casper zebrafish males, but not in females (47). To our knowledge, brain and pituitary lpxrfa-r expression has not been investigated in fish under fasting conditions. Increased brain lpxrfa expression levels or LPXRFa-immunoreactive cell number in response to food deprivation was also observed in other species, including chicks (43, 61), Pekin ducks (36) and Syrian hamsters (62). Considering the orexigenic role of LPXRFa in birds and mammals (63), it is reasonable to assume that elevation of LPXRFa in the brain under fasting condition is sufficient to induce feeding behavior. Fasting did not alter lpxrfa expression in the hypothalamus of zebra finch males, in both mRNA and immunoreactivity levels (45). However, the number of LPXRFa-immunoreactive cells declined significantly in zebra finch females, showing sexual dimorphism of LPXRFa changes in response to nutritional stress (44). In addition, hypothalamic lpxrfa-r expression was lower in fasted chicks, perhaps due to receptor down-regulation in response to increased lpxrfa expression (61). Taken together, complex regulation of the LPXRFa/LPXRFa-R system exists in various species during negative metabolic state.
Similarly, the actions of negative nutritional status on the Kiss/KissR system are controversial. In the current study, neither kiss2 nor kiss2r were altered in the brain and pituitary after starvation for 2 or 4 weeks, although an evident increase in pituitary kiss2 expression was observed at the first week after refeeding. In another flatfish species, the Senegalese sole, fasting increased mRNA levels of kiss2 and kiss2r in the hypothalamus, without any effects in the stomach (50). Interestingly, in wild-type zebrafish, fasting increased the brain expressions of kiss1 in females and kiss2 in males, respectively (47). However, neither kiss1 nor kiss2 transcripts were affected by fasting in Casper zebrafish (47). In male European sea bass, hypothalamic kiss1, kiss2, kiss1r and kiss2r transcripts were elevated after a prolonged period of food restriction (64). Food deprivation also resulted in a significant increase in hypothalamic kiss2 and kissr2_v1 mRNA levels in adult pejerrey males, without affecting kissr2_v1 and kissr2_v2 expression in the testis and habenula (51). By contrast, starvation decreased kiss1 and kissr1 expression in the hypothalamus of rhesus monkeys and mice (48, 65). Fasting also reduced brain kiss1 expression in rats (66), lambs (67), and monkeys (68). Interestingly, a decrease in hypothalamic kiss1 with a concomitant rise in kissr1 mRNA levels was noticed in fasted rats (49). Altogether, kisspeptin signaling also mediates energy balance effects on the reproductive axis in fish, but the neuroendocrine mechanisms underlying the actions of undernutrition and low energy availability on the reproductive axis may differ between mammals and teleosts (69).
In summary, food deprivation differentially modulates gene expression of the components of LPXRFa and Kiss systems in half-smooth tongue sole. Combined with results from previous studies, differences of LPXRFa and Kiss in response to starvation could occur because of variations in species, sex, reproductive status, tissue and the elapsed time after treatment, indicating that LPXRFa and Kiss may provide a molecular switch between reproduction and appetite in vertebrates. The nature of starvation-elicited metabolic signals that alter LPXRFa and Kiss signaling is yet not well known, especially in teleosts (34, 59), and further studies are urgently needed to clarify how multiple signals work in concert to control reproduction during negative energy balance.
The original contributions presented in the study are included in the article/supplementary material, further inquiries can be directed to the corresponding author/s.
The animal study was reviewed and approved by Animal Care and Use Committee of Yellow Sea Fisheries Research Institute, Chinese Academy of Fishery Sciences.
BW and YX designed this study. BW, AC, YZ and YJ performed the sampling of the fish. BW and AC conducted the RT-qPCR analysis and analyzed the data. BW wrote the first draft of the manuscript. YX and XL edited the manuscript. BW and YX provided funding. All authors contributed to the article and approved the submitted version.
National Natural Science Foundation of China (32072949, 32072993), Central Public-interest Scientific Institution Basal Research Fund, YSFRI, CAFS (20603022022018), Central Public-interest Scientific Institution Basal Research Fund, CAFS (TD202047), and China Agriculture Research System of MOF and MARA (CARS-47).
The authors declare that the research was conducted in the absence of any commercial or financial relationships that could be construed as a potential conflict of interest.
All claims expressed in this article are solely those of the authors and do not necessarily represent those of their affiliated organizations, or those of the publisher, the editors and the reviewers. Any product that may be evaluated in this article, or claim that may be made by its manufacturer, is not guaranteed or endorsed by the publisher.
1. Tsutsui K, Saigoh E, Ukena K, Teranishi H, Fujisawa Y, Kikuchi M, et al. A novel avian hypothalamic peptide inhibiting gonadotropin release. Biochem Biophys Res Commun (2000) 275:661–7. doi: 10.1006/bbrc.2000.3350
2. Tsutsui K, Ubuka T, Ukena K. Advancing reproductive neuroendocrinology through research on the regulation of GnIH and on its diverse actions on reproductive physiology and behavior. Front Neuroendocrinol (2022) 64:100955. doi: 10.1016/j.yfrne.2021.100955
3. Pinelli C, Scandurra A, Tsutsui K, Falvo S, D'Aniello B. Comparative insights of the neuroanatomical distribution of the gonadotropin-inhibitory hormone (GnIH) in fish and amphibians. Front Neuroendocrinol (2022) 65:100991. doi: 10.1016/j.yfrne.2022.100991
4. Munoz-Cueto JA, Paullada-Salmeron JA, Aliaga-Guerrero M, Cowan ME, Parhar IS, Ubuka T. A journey through the gonadotropin-inhibitory hormone system of fish. Front Endocrinol (Lausanne) (2017) 8:285. doi: 10.3389/fendo.2017.00285
5. Di Yorio MP, Munoz-Cueto JA, Paullada-Salmeron JA, Somoza GM, Tsutsui K, Vissio PG. The gonadotropin-inhibitory hormone: What we know and what we still have to learn from fish. Front Endocrinol (Lausanne) (2019) 10:78. doi: 10.3389/fendo.2019.00078
6. Teo CH, Phon B, Parhar I. The role of GnIH in biological rhythms and social behaviors. Front Endocrinol (Lausanne) (2021) 12:728862. doi: 10.3389/fendo.2021.728862
7. Tobari Y, Aleksandrova Y, Fukahori Y, Tsutsui K, Meddle SL. Gonadotropin-inhibitory hormone as a regulator of social interactions in vertebrates. Front Neuroendocrinol (2022) 64:100954. doi: 10.1016/j.yfrne.2021.100954
8. Ubuka T, Parhar IS, Tsutsui K. Gonadotropin-inhibitory hormone mediates behavioral stress responses. Gen Comp Endocrinol (2018) 265:202–6. doi: 10.1016/j.ygcen.2018.03.004
9. Son YL, Ubuka T, Millar RP, Kanasaki H, Tsutsui K. Gonadotropin-inhibitory hormone inhibits GnRH-induced gonadotropin subunit gene transcriptions by inhibiting AC/cAMP/PKA-dependent ERK pathway in LbetaT2 cells. Endocrinology. (2012) 153:2332–43. doi: 10.1210/en.2011-1904
10. Son YL, Ubuka T, Soga T, Yamamoto K, Bentley GE, Tsutsui K. Inhibitory action of gonadotropin-inhibitory hormone on the signaling pathways induced by kisspeptin and vasoactive intestinal polypeptide in GnRH neuronal cell line, GT1-7. FASEB J (2016) 30:2198–210. doi: 10.1096/fj.201500055
11. Clarke IJ, Sari IP, Qi Y, Smith JT, Parkington HC, Ubuka T, et al. Potent action of RFamide-related peptide-3 on pituitary gonadotropes indicative of a hypophysiotropic role in the negative regulation of gonadotropin secretion. Endocrinology. (2008) 149:5811–21. doi: 10.1210/en.2008-0575
12. Sari IP, Rao A, Smith JT, Tilbrook AJ, Clarke IJ. Effect of RF-amide-related peptide-3 on luteinizing hormone and follicle-stimulating hormone synthesis and secretion in ovine pituitary gonadotropes. Endocrinology. (2009) 150:5549–56. doi: 10.1210/en.2009-0775
13. Shimizu M, Bedecarrats GY. Activation of the chicken gonadotropin-inhibitory hormone receptor reduces gonadotropin releasing hormone receptor signaling. Gen Comp Endocrinol (2010) 167:331–7. doi: 10.1016/j.ygcen.2010.03.029
14. Biran J, Golan M, Mizrahi N, Ogawa S, Parhar IS, Levavi-Sivan B. LPXRFa, the piscine ortholog of GnIH, and LPXRF receptor positively regulate gonadotropin secretion in tilapia (Oreochromis niloticus). Endocrinology. (2014) 155:4391–401. doi: 10.1210/en.2013-2047
15. Wang Q, Qi X, Guo Y, Li S, Zhang Y, Liu X, et al. Molecular identification of GnIH/GnIHR signal and its reproductive function in protogynous hermaphroditic orange-spotted grouper (Epinephelus coioides). Gen Comp Endocrinol (2015) 216:9–23. doi: 10.1016/j.ygcen.2015.04.016
16. Spicer OS, Zmora N, Wong TT, Golan M, Levavi-Sivan B, Gothilf Y, et al. The gonadotropin-inhibitory hormone (Lpxrfa) system's regulation of reproduction in the brain-pituitary axis of the zebrafish (Danio rerio). Biol Reprod (2017) 96:1031–42. doi: 10.1093/biolre/iox032
17. Wang B, Yang G, Liu Q, Qin J, Xu Y, Li W, et al. Characterization of LPXRFa receptor in the half-smooth tongue sole (Cynoglossus semilaevis): Molecular cloning, expression profiles, and differential activation of signaling pathways by LPXRFa peptides. Comp Biochem Physiol A Mol Integr Physiol (2018) 223:23–32. doi: 10.1016/j.cbpa.2018.05.008
18. Ohga H, Matsuyama M. Effects of LPXRFamide peptides on chub mackerel gonadotropin secretion. Biol Reprod (2021) 105:1179–88. doi: 10.1093/biolre/ioab130
19. Wang B, Paullada-Salmeron JA, Verges-Castillo A, Gomez A, Munoz-Cueto JA. Signaling pathways activated by sea bass gonadotropin-inhibitory hormone peptides in COS-7 cells transfected with their cognate receptor. Front Endocrinol (Lausanne) (2022) 13:982246. doi: 10.3389/fendo.2022.982246
20. Wang B, Yang G, Xu Y, Zhang Y, Liu X. In vitro effects of tongue sole LPXRFa and kisspeptin on relative abundance of pituitary hormone mRNA and inhibitory action of LPXRFa on kisspeptin activation in the PKC pathway. Anim Reprod Sci (2019) 203:1–9. doi: 10.1016/j.anireprosci.2019.01.009
21. Wang B, Yang G, Liu Q, Qin J, Xu Y, Li W, et al. Inhibitory action of tongue sole LPXRFa, the piscine ortholog of gonadotropin-inhibitory hormone, on the signaling pathway induced by tongue sole kisspeptin in COS-7 cells transfected with their cognate receptors. Peptides. (2017) 95:62–7. doi: 10.1016/j.peptides.2017.07.014
22. Somoza GM, Mechaly AS, Trudeau VL. Kisspeptin and GnRH interactions in the reproductive brain of teleosts. Gen Comp Endocrinol (2020) 298:113568. doi: 10.1016/j.ygcen.2020.113568
23. Pinilla L, Aguilar E, Dieguez C, Millar RP, Tena-Sempere M. Kisspeptins and reproduction: Physiological roles and regulatory mechanisms. Physiol Rev (2012) 92:1235–316. doi: 10.1152/physrev.00037.2010
24. Pasquier J, Kamech N, Lafont AG, Vaudry H, Rousseau K, Dufour S. Molecular evolution of GPCRs: Kisspeptin/kisspeptin receptors. J Mol Endocrinol (2014) 52:T101–17. doi: 10.1530/JME-13-0224
25. Sivalingam M, Ogawa S, Trudeau VL, Parhar IS. Conserved functions of hypothalamic kisspeptin in vertebrates. Gen Comp Endocrinol (2022) 317:113973. doi: 10.1016/j.ygcen.2021.113973
26. Wang B, Mechaly AS, Somoza GM. Overview and new insights into the diversity, evolution, role, and regulation of kisspeptins and their receptors in teleost fish. Front Endocrinol (Lausanne) (2022) 13:862614. doi: 10.3389/fendo.2022.862614
27. Sivalingam M, Parhar IS. Hypothalamic kisspeptin and kisspeptin receptors: Species variation in reproduction and reproductive behaviours. Front Neuroendocrinol (2022) 64:100951. doi: 10.1016/j.yfrne.2021.100951
28. Dufour S, Querat B, Tostivint H, Pasqualini C, Vaudry H, Rousseau K. Origin and evolution of the neuroendocrine control of reproduction in vertebrates, with special focus on genome and gene duplications. Physiol Rev (2020) 100:869–943. doi: 10.1152/physrev.00009.2019
29. Tang H, Liu Y, Luo D, Ogawa S, Yin Y, Li S, et al. The kiss/kissr systems are dispensable for zebrafish reproduction: Evidence from gene knockout studies. Endocrinology. (2015) 156:589–99. doi: 10.1210/en.2014-1204
30. Liu Y, Tang H, Xie R, Li S, Liu X, Lin H, et al. Genetic evidence for multifactorial control of the reproductive axis in zebrafish. Endocrinology. (2017) 158:604–11. doi: 10.1210/en.2016-1540
31. Nakajo M, Kanda S, Karigo T, Takahashi A, Akazome Y, Uenoyama Y, et al. Evolutionally conserved function of kisspeptin neuronal system is nonreproductive regulation as revealed by nonmammalian study. Endocrinology. (2018) 159:163–83. doi: 10.1210/en.2017-00808
32. Etzion T, Zmora N, Zohar Y, Levavi-Sivan B, Golan M, Gothilf Y. Ectopic over expression of kiss1 may compensate for the loss of kiss2. Gen Comp Endocrinol (2020) 295:113523. doi: 10.1016/j.ygcen.2020.113523
33. Schneider JE. Energy balance and reproduction. Physiol Behav (2004) 81:289–317. doi: 10.1016/j.physbeh.2004.02.007
34. Shahjahan M, Kitahashi T, Parhar IS. Central pathways integrating metabolism and reproduction in teleosts. Front Endocrinol (Lausanne) (2014) 5:36. doi: 10.3389/fendo.2014.00036
35. Tachibana T, Sato M, Takahashi H, Ukena K, Tsutsui K, Furuse M. Gonadotropin-inhibiting hormone stimulates feeding behavior in chicks. Brain Res (2005) 1050:94–100. doi: 10.1016/j.brainres.2005.05.035
36. Fraley GS, Coombs E, Gerometta E, Colton S, Sharp PJ, Li Q, et al. Distribution and sequence of gonadotropin-inhibitory hormone and its potential role as a molecular link between feeding and reproductive systems in the pekin duck (Anas platyrhynchos domestica). Gen Comp Endocrinol (2013) 184:103–10. doi: 10.1016/j.ygcen.2012.11.026
37. Clarke IJ, Smith JT, Henry BA, Oldfield BJ, Stefanidis A, Millar RP, et al. Gonadotropin-inhibitory hormone is a hypothalamic peptide that provides a molecular switch between reproduction and feeding. Neuroendocrinology. (2012) 95:305–16. doi: 10.1159/000332822
38. Johnson MA, Tsutsui K, Fraley GS. Rat RFamide-related peptide-3 stimulates GH secretion, inhibits LH secretion, and has variable effects on sex behavior in the adult male rat. Horm Behav (2007) 51:171–80. doi: 10.1016/j.yhbeh.2006.09.009
39. Talbi R, Laran-Chich MP, Magoul R, El Ouezzani S, Simonneaux V. Kisspeptin and RFRP-3 differentially regulate food intake and metabolic neuropeptides in the female desert jerboa. Sci Rep (2016) 6:36057. doi: 10.1038/srep36057
40. Saito R, Tanaka K, Nishimura H, Nishimura K, Sonoda S, Ueno H, et al. Centrally administered kisspeptin suppresses feeding via nesfatin-1 and oxytocin in male rats. Peptides. (2019) 112:114–24. doi: 10.1016/j.peptides.2018.12.003
41. Stengel A, Wang L, Goebel-Stengel M, Tache Y. Centrally injected kisspeptin reduces food intake by increasing meal intervals in mice. Neuroreport. (2011) 22:253–7. doi: 10.1097/WNR.0b013e32834558df
42. Dong TS, Vu JP, Oh S, Sanford D, Pisegna JR, Germano P. Intraperitoneal treatment of kisspeptin suppresses appetite and energy expenditure and alters gastrointestinal hormones in mice. Dig Dis Sci (2020) 65:2254–63. doi: 10.1007/s10620-019-05950-7
43. Chowdhury VS, Tomonaga S, Nishimura S, Tabata S, Cockrem JF, Tsutsui K, et al. Hypothalamic gonadotropin-inhibitory hormone precursor mRNA is increased during depressed food intake in heat-exposed chicks. Comp Biochem Physiol Part A: Mol Integr Physiol (2012) 162:227–33. doi: 10.1016/j.cbpa.2012.03.009
44. Wilsterman K, Alonge MM, Bao X, Conner KA, Bentley GE. Food access modifies GnIH, but not CRH, cell number in the hypothalamus in a female songbird. Gen Comp Endocrinol (2020) 292:113438. doi: 10.1016/j.ygcen.2020.113438
45. Lynn SE, Perfito N, Guardado D, Bentley GE. Food, stress, and circulating testosterone: Cue integration by the testes, not the brain, in male zebra finches (Taeniopygia guttata). Gen Comp Endocrinol (2015) 215:1–9. doi: 10.1016/j.ygcen.2015.03.010
46. Ciccone NA, Dunn IC, Sharp PJ. Increased food intake stimulates GnRH-I, glycoprotein hormone alpha-subunit and follistatin mRNAs, and ovarian follicular numbers in laying broiler breeder hens. Domest Anim Endocrinol (2007) 33:62–76. doi: 10.1016/j.domaniend.2006.04.008
47. London S, Volkoff H. Effects of fasting on the central expression of appetite-regulating and reproductive hormones in wild-type and casper zebrafish (Danio rerio). Gen Comp Endocrinol (2019) 282:113207. doi: 10.1016/j.ygcen.2019.06.011
48. Luque RM, Kineman RD, Tena-Sempere M. Regulation of hypothalamic expression of KiSS-1 and GPR54 genes by metabolic factors: Analyses using mouse models and a cell line. Endocrinology. (2007) 148:4601–11. doi: 10.1210/en.2007-0500
49. Castellano JM, Navarro VM, Fernández-Fernández R, Nogueiras R, Tovar S, Roa J, et al. Changes in hypothalamic KiSS-1 system and restoration of pubertal activation of the reproductive axis by kisspeptin in undernutrition. Endocrinology. (2005) 146:3917–25. doi: 10.1210/en.2005-0337
50. Mechaly AS, Vinas J, Piferrer F. Gene structure analysis of kisspeptin-2 (Kiss2) in the Senegalese sole (Solea senegalensis): characterization of two splice variants of Kiss2, and novel evidence for metabolic regulation of kisspeptin signaling in non-mammalian species. Mol Cell Endocrinol (2011) 339:14–24. doi: 10.1016/j.mce.2011.03.004
51. Mechaly AS, Tovar Bohorquez MO, Mechaly AE, Suku E, Perez MR, Giorgetti A, et al. Evidence of alternative splicing as a regulatory mechanism for Kissr2 in pejerrey fish. Front Endocrinol (Lausanne) (2018) 9:604. doi: 10.3389/fendo.2018.00604
52. Son YL, Ubuka T, Tsutsui K. Regulation of stress response on the hypothalamic-pituitary-gonadal axis via gonadotropin-inhibitory hormone. Front Neuroendocrinol (2022) 64:100953. doi: 10.1016/j.yfrne.2021.100953
53. Wang B, Liu Q, Liu X, Xu Y, Shi B. Molecular characterization of Kiss2 receptor and in vitro effects of Kiss2 on reproduction-related gene expression in the hypothalamus of half-smooth tongue sole (Cynoglossus semilaevis). Gen Comp Endocrinol (2017) 249:55–63. doi: 10.1016/j.ygcen.2017.04.006
54. Wang B, Liu Q, Liu X, Xu Y, Song X, Shi B. Molecular characterization of kiss2 and differential regulation of reproduction-related genes by sex steroids in the hypothalamus of half-smooth tongue sole (Cynoglossus semilaevis). Comp Biochem Physiol A Mol Integr Physiol (2017) 213:46–55. doi: 10.1016/j.cbpa.2017.08.003
55. Wang B, Liu Q, Liu X, Xu Y, Shi B. Molecular characterization and expression profiles of LPXRFa at the brain-pituitary-gonad axis of half-smooth tongue sole (Cynoglossus semilaevis) during ovarian maturation. Comp Biochem Physiol B Biochem Mol Biol (2018) 216:59–68. doi: 10.1016/j.cbpb.2017.11.016
56. Wang B, Yang G, Xu Y, Li W, Liu X. Recent studies of LPXRFa receptor signaling in fish and other vertebrates. Gen Comp Endocrinol (2019) 277:3–8. doi: 10.1016/j.ygcen.2018.11.011
57. Wang B, Cui A, Tian J, Zhang Y, Jiang Y, Xu Y, et al. Characterization of a novel spexin gene (spx2) in the half-smooth tongue sole and regulation of its expression by nutritional status. Aquac Rep (2020) 18:100544. doi: 10.1016/j.aqrep.2020.100544
58. True C, Grove KL, Smith MS. Beyond leptin: Emerging candidates for the integration of metabolic and reproductive function during negative energy balance. Front Endocrinol (Lausanne) (2011) 2:53. doi: 10.3389/fendo.2011.00053
59. Wahab F, Shahab M, Behr R. The involvement of gonadotropin inhibitory hormone and kisspeptin in the metabolic regulation of reproduction. J Endocrinol (2015) 225:R49–66. doi: 10.1530/JOE-14-0688
60. Bedecarrats GY, Hanlon C, Tsutsui K. Gonadotropin inhibitory hormone and its receptor: Potential key to the integration and coordination of metabolic status and reproduction. Front Endocrinol (Lausanne) (2022) 12:781543. doi: 10.3389/fendo.2021.781543
61. McConn BR, Yi J, Gilbert ER, Siegel PB, Chowdhury VS, Furuse M, et al. Stimulation of food intake after central administration of gonadotropin-inhibitory hormone is similar in genetically selected low and high body weight lines of chickens. Gen Comp Endocrinol (2016) 232:96–100. doi: 10.1016/j.ygcen.2016.01.004
62. Schneider JE, Benton NA, Russo KA, Klingerman CM, Williams WP, Simberlund J, et al. RFamide-related peptide-3 and the trade-off between reproductive and ingestive behavior. Integr Comp Biol (2017) 57:1225–39. doi: 10.1093/icb/icx097
63. Tsutsui K, Ubuka T. How to contribute to the progress of neuroendocrinology: Discovery of GnIH and progress of GnIH research. Front Endocrinol (Lausanne) (2018) 9:662. doi: 10.3389/fendo.2018.00662
64. Escobar S, Felip A, Zanuy S, Carrillo M. Is the kisspeptin system involved in responses to food restriction in order to preserve reproduction in pubertal male sea bass (Dicentrarchus labrax)? Comp Biochem Physiol A Mol Integr Physiol (2016) 199:38–46. doi: 10.1016/j.cbpa.2016.05.005
65. Wahab F, Ullah F, Chan YM, Seminara SB, Shahab M. Decrease in hypothalamic Kiss1 and Kiss1r expression: a potential mechanism for fasting-induced suppression of the HPG axis in the adult male rhesus monkey (Macaca mulatta). Horm Metab Res (2011) 43:81–5. doi: 10.1055/s-0030-1269852
66. Ladyman SR, Woodside B. Food restriction during lactation suppresses Kiss1 mRNA expression and kisspeptin-stimulated LH release in rats. Reproduction. (2014) 147:743–51. doi: 10.1530/REP-13-0426
67. Polkowska J, Cieslak M, Wankowska M, Wojcik-Gladysz A. The effect of short fasting on the hypothalamic neuronal system of kisspeptin in peripubertal female lambs. Anim Reprod Sci (2015) 159:184–90. doi: 10.1016/j.anireprosci.2015.06.016
68. Shamas S, Khan SU, Khan MY, Shabbir N, Zubair H, Shafqat S, et al. Fasting induced kisspeptin signaling suppression is regulated by glutamate mediated cues in adult male rhesus macaque (Macaca mulatta). Neuropeptides. (2015) 52:39–45. doi: 10.1016/j.npep.2015.06.005
Keywords: LPXRFa, LPXRFa receptor, kisspeptin, kisspeptin receptor, Cynoglossus semilaevis
Citation: Wang B, Cui A, Xu Y, Zhang Y, Jiang Y and Liu X (2023) Food deprivation differentially modulates gene expression of LPXRFa and kisspeptin systems in the brain-pituitary axis of half-smooth tongue sole (Cynoglossus semilaevis). Front. Endocrinol. 14:1099832. doi: 10.3389/fendo.2023.1099832
Received: 16 November 2022; Accepted: 15 March 2023;
Published: 24 March 2023.
Edited by:
Ayelén Melisa Blanco, University of Vigo, SpainReviewed by:
Karine Rousseau, Muséum National d’Histoire Naturelle, FranceCopyright © 2023 Wang, Cui, Xu, Zhang, Jiang and Liu. This is an open-access article distributed under the terms of the Creative Commons Attribution License (CC BY). The use, distribution or reproduction in other forums is permitted, provided the original author(s) and the copyright owner(s) are credited and that the original publication in this journal is cited, in accordance with accepted academic practice. No use, distribution or reproduction is permitted which does not comply with these terms.
*Correspondence: Yongjiang Xu, eHV5akB5c2ZyaS5hYy5jbg==
Disclaimer: All claims expressed in this article are solely those of the authors and do not necessarily represent those of their affiliated organizations, or those of the publisher, the editors and the reviewers. Any product that may be evaluated in this article or claim that may be made by its manufacturer is not guaranteed or endorsed by the publisher.
Research integrity at Frontiers
Learn more about the work of our research integrity team to safeguard the quality of each article we publish.