- 1Steno Diabetes Center Odense, Odense University Hospital, Odense, Denmark
- 2Department of Sports Science and Clinical Biomechanics, University of Southern Denmark, Odense, Denmark
Aims: Non-weight-bearing high-intensity interval training (HIIT) involving several muscle groups may efficiently improve metabolic health without compromising adherence in obesity and type 2 diabetes. In a non-randomized intervention study, we examined the effect of a novel HIIT-protocol, recruiting both lower and upper body muscles, on insulin sensitivity, measures of metabolic health and adherence in obesity and type 2 diabetes.
Methods: In 15 obese men with type 2 diabetes and age-matched obese (n=15) and lean (n=18) glucose-tolerant men, the effects of 8-weeks supervised HIIT combining rowing and cycling on ergometers (3 sessions/week) were examined by DXA-scan, incremental exercise test and hyperinsulinemic-euglycemic clamp combined with indirect calorimetry.
Results: At baseline, insulin-stimulated glucose disposal rate (GDR) was ~40% reduced in the diabetic vs the non-diabetic groups (all p<0.01). In response to HIIT, insulin-stimulated GDR increased ~30-40% in all groups (all p<0.01) entirely explained by increased glucose storage. These changes were accompanied by ~8-15% increases in VO2max, (all p<0.01), decreased total fat mass and increased lean body mass in all groups (all p<0.05). There were no correlations between these training adaptations and no group-differences in these responses. HbA1c showed a clinically relevant decrease in men with type 2 diabetes (4±2 mmol/mol; p<0.05). Importantly, adherence was high (>95%) in all groups and no injuries were reported.
Conclusions: A novel HIIT-protocol recruiting lower and upper body muscles efficiently improves insulin sensitivity, VO2max and body composition with intact responses in obesity and type 2 diabetes. The high adherence and lack of injuries show that non-weight-bearing HIIT involving several muscle groups is a promising mode of exercise training in obesity and type 2 diabetes.
Introduction
The global prevalence of type 2 diabetes is rapidly increasing with obesity and sedentary lifestyle as major risk factors. Type 2 diabetes and obesity are characterized by insulin resistance, which is associated with an increased risk of cardiovascular morbidity and mortality (1). However, except for strategies that aim for weight loss and increased physical activity, current treatment options that directly target insulin resistance are limited. At the metabolic level, insulin resistance in obesity and type 2 diabetes is characterized by reduced insulin-stimulated glucose uptake and glucose storage in skeletal muscle (2–4). At the molecular level, insulin resistance in skeletal muscle is associated with reduced insulin signaling to glucose transport and glycogen synthesis, accumulation of lipid metabolites and abnormalities in mitochondrial oxidative metabolism (3–7).
Physical activity plays an important role in the prevention and management of type 2 diabetes (8, 9). Studies of regular exercise training for ≥8 weeks have shown improvements in insulin sensitivity and glycemic control, together with beneficial changes in cardiorespiratory fitness (VO2max), body composition, blood pressure, and lipid profile in patients with type 2 diabetes (4, 9–11). The cellular and molecular adaptations underlying the beneficial metabolic effects of exercise training, also in obesity and type 2 diabetes, include increased mitochondrial content and function and increased abundance of proteins involved in insulin-signaling to glucose uptake and glucose storage in skeletal muscle (4, 12–16). In individuals with type 2 diabetes or obesity, the majority of studies have reported the effects of endurance training at moderate intensities on cycle ergometers or treadmills and resistance training, either separately or in combination (9). Moreover, in most studies, patients with type 2 diabetes have been compared only with weight and age-matched controls (2–4, 12, 13, 16). This precludes the opportunity to find attenuated responses in these groups compared to lean, healthy individuals, and hence the potential presence of exercise resistance (17, 18). Importantly, the most appropriate mode of exercise training for sedentary middle-aged individuals with obesity and type 2 diabetes, when it comes to beneficial effects on metabolic health, adherence and risk of injuries, remains to be established (10).
Within the last decades, high-intensity interval training (HIIT) has gained increased interest. HIIT is defined as “near maximal” efforts, and is generally performed at an intensity higher than 80% of maximal heart rate. Indeed, HIIT-protocols with shorter training durations have shown the same or better effects on measures of insulin sensitivity, glucose metabolism, VO2max, and body composition compared with endurance training not only in healthy, lean individuals (19, 20), but also in obesity and type 2 diabetes (21–23). However, in most HIIT studies, insulin sensitivity was determined as fasting or oral glucose tolerance test (OGTT) derived surrogate markers and not by the gold standard hyperinsulinemic-euglycemic clamp.
Skeletal muscle accounts for approximately 40% of total body weight and is the predominant site of insulin-stimulated glucose uptake (3). Interestingly, a recent study indicates that recruitment of both upper and lower body muscle groups could be necessary to take full advantage of the insulin-sensitizing effect of exercise training (24). Thus, while several previous studies of acute exercise using the one-leg technique have shown that insulin sensitivity is increased in the exercised muscles and not the inactive muscles (15, 25), it was recently reported that insulin-mediated glucose uptake is actually decreased in the non-exercised muscle groups after an acute exercise bout causing a reduction in whole-body insulin stimulated glucose disposal (24). Moreover, previous studies of HIIT have mainly focused on lower body activities such as walking, running and cycling (19–23). Although this mechanism remains to be demonstrated after an exercise training period, these findings suggest that the combination of rowing and cycling, which are both non-weight-bearing modes of exercise with a low risk of injuries, could enhance the beneficial effects of HIIT on insulin sensitivity and other measures of metabolic health by recruiting several lower and upper body muscle groups.
In the present study, we investigated the effects of a novel HIIT-protocol combing rowing and cycling on insulin sensitivity determined by the gold-standard hyperinsulinemic-euglycemic clamp, VO2max, body composition and substrate metabolism as well as adherence in obese men with type 2 diabetes, and whether these effects were attenuated compared with glucose-tolerant obese and lean glucose-tolerant men.
Material and methods
Study population
The study was performed at Steno Diabetes Center Odense (SDCO) and Department of Sport Science and Clinical Biomechanics at the University of Southern Denmark (SDU) in the period from January 2018 to December 2019. Middle-aged (40-65 years) obese (BMI 27-36) men with type 2 diabetes (n=15) and glucose-tolerant obese (BMI 27-36; n=15) and lean (BMI 20-25; n=18) men were included in the study (Table 1). The participants were all non-smokers and matched according to age, and the obese men with or without type 2 diabetes were further matched according to BMI. The lean and obese controls had normal glucose tolerance as evaluated by a 75 g OGTT and no family history of diabetes, and were not taking medication. Medication details and eligibility criteria are given in supporting information methods. Informed consent was obtained from all participants before inclusion. The study was approved by the Regional Scientific Ethical Committees for Southern Denmark and performed in accordance with the Helsinki Declaration II. Four participants did not complete the study. One lean person didn’t start the training period because of new knee problems, and one lean and two diabetic men withdrew their consent to continue training due to lack of time. Their baseline characteristics are included in the analysis. In this non-randomized intervention study, the changes in insulin sensitivity in the three groups response to the HIIT was the primary outcome, while HIIT-induced changes in measures of body composition, VO2max and substrate oxidation as well as adherence were secondary outcomes.
Study design
The study participants were examined on two days (Day 1 and 2) before separated by up to 2 weeks and on two days after (Day 3 and 4) an 8-week HIIT-protocol combining rowing and cycling (Figure 1). On Day 1 and 3, total fat mass and lean body mass (LBM), maximal oxygen consumption (VO2max) and maximal cycling capacity (MCC) were determined (see Supplementary Material). On Day 2 and 4, biochemical characteristics and a hyperinsulinemic-euglycemic clamp combined with indirect calorimetry were performed to examine insulin sensitivity and substrate metabolism. Day 3 was performed 60 hours after the last afternoon HIIT-session, and Day 4 was performed 48 h after the VO2max test on Day 3. The timing of the post-training examinations was planned based on the assumption that the VO2max test on Day 3 would maintain the long-lasting effects of the HIIT-protocol, and at the same time the acute effects of the VO2max test would not persist when the hyperinsulinemic-euglycemic clamp was performed 48 h later (26, 27). The participants were examined after an overnight fast and were instructed to refrain from strenuous physical activity for a period of 48-h and abstain from alcohol and caffeine for 24-h prior to experimental days. The participants were instructed not to change their dietary habits during the training intervention. In patients with type 2 diabetes, all medication was withdrawn one week prior to the clamp studies (Day 2 and 4), but was otherwise continued during the 8 weeks of HIIT.
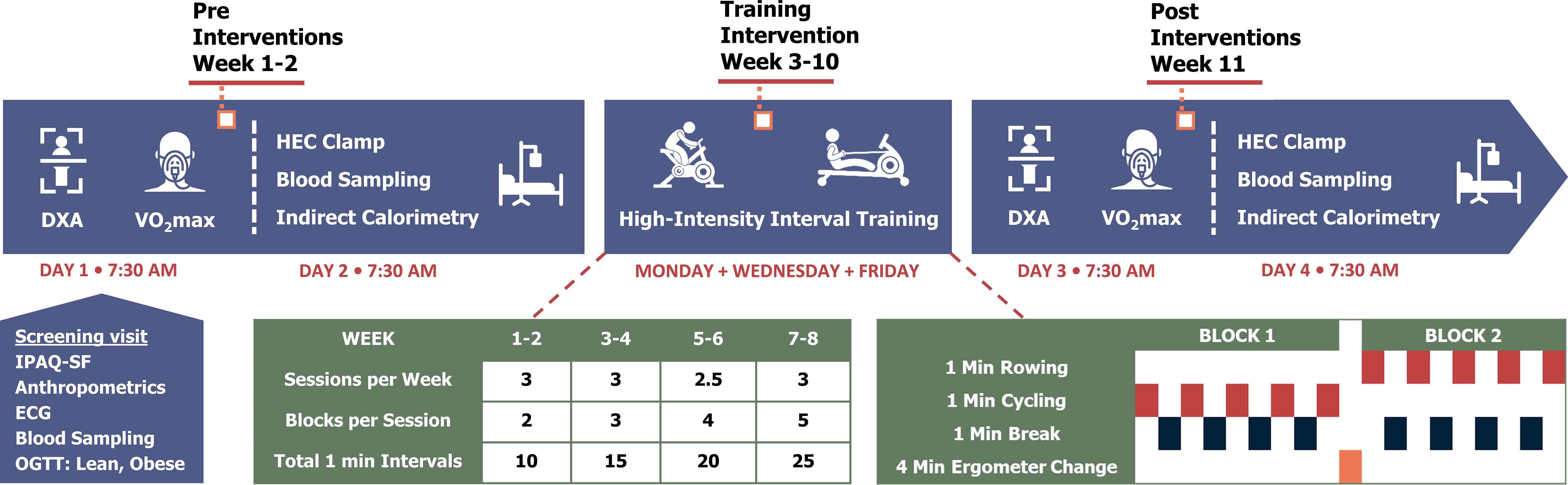
Figure 1 Study experimental design. HEC, hyperinsulinemic-euglycemic clamp; IPAQ-SF, International Physical Activity Questionnaire Short Form; OGTT, oral glucose tolerance test.
Hyperinsulinemic-euglycemic clamp and biochemical analysis
Before (Day 2) and after (Day 4) the HIIT-protocol, the participants underwent a hyperinsulinemic-euglycemic clamp with tracer glucose consisting of a 2-h basal tracer equilibration period and a 3-h insulin-stimulated period using an insulin infusion rate of 40 mU/min/m2. For further details, see Supplementary Material. This was combined with indirect calorimetry to assess glucose infusion rate (GIR), tracer-determined glucose disposal rates (GDR) and hepatic glucose production (HGP), respiratory exchange ratio (RER), as well as rates of glucose (GOX) and lipid oxidation (LOX), and non-oxidative glucose metabolism (NOX) in the basal and insulin-stimulated steady-state periods as described (see also Supplementary Material) (2). GDR was estimated both related to the body surface area and the total lean body mass. In patients with type 2 diabetes, plasma glucose was allowed to decline to 5.5 mmol/l before glucose infusion was initiated. Plasma glucose, triglycerides, and cholesterols, and serum insulin and C-peptide were measured as described (see Supplementary Material).
HIIT protocol
In all participants, the effects of 8 weeks supervised HIIT combing rowing and cycling (3 sessions per week) were investigated. Participants in all three groups trained together in small groups of up to 10 participants. There was a continuous run-in and completion of participants. The majority of the training sessions (>95%) took place in the afternoon. Each training session started with a 10-min warm-up period on rowing (Concept II Model E, Vermont, US) or cycle ergometers (WattBike Pro/Trainer, Nottingham, UK), including row-specific technique training with increasing workload and heart rate. The HIIT-protocol consisted of training blocks of 5 x 1 min high-intensity intervals each interspersed by 1 min active or resting recovery. Between the training blocks, the participants had a 4-min break in which they shifted from rowing to cycling and vice versa. The number of blocks per session was gradually increased with an extra block added every second week, going from 2 blocks in week 1-2 to 5 blocks in week 7-8. The total duration of each training session was 32 min in week 1-2, 45 min in week 3-4, 58 min in week 5-6, and 71 min in week 7-8, whereas the total duration of high-intensity intervals was 10 min in week 1-2, 15 min in week 3-4, 20 min in week 5-6, and 25 min in week 7-8.The workload was set at 100-110% of MCC during cycling intervals, while the heart rate was kept similar at 86-88% of max during rowing and cycling. Participants were encouraged by social commitment, loud music, support from supervisors, and awareness of real-time relative heart rate due to monitoring (Polar H7, Polar team, Kempele, FI) throughout the sessions. Halfway through the HIIT-protocol, the workload was adjusted according to a midway VO2max test to ensure the relative workload was maintained throughout the training period.
Deviations from protocol
Three men with type 2 diabetes and one lean man had their training period extended 1-1½ week due to illness during the training period. Two other lean and two obese men had their final DXA-scan and VO2max test (Day 3) 4-5 days after the last HIIT training session, and their final clamp (Day 4) 48-h after this.
Statistics
All statistical analyses were performed by STATA/IC 16.1. The sample size were estimated to detect a 30% reduction in baseline insulin-stimulated GDR in men with T2D compared to lean, healthy men, and a 15% increase in insulin-stimulated GDR in men with T2D in response to HIIT. This gave a power of >80% when including 13 individuals in each group. Using mixed model linear regression, analyses for the HIIT-induced responses on outcome variables within each group were performed, as well as analyses comparing pre- and post-training data and the HIIT-induced responses between the groups. Data on self-reported levels of physical activity were compared between the groups by linear regression analyses (see Supplementary Material). Normality was checked using QQ-plots of residuals and for the mixed model also by QQ-plots of random effects. When residuals were not normally distributed, either Kruskal-Wallis test or binomial regression was performed. Pearson`s correlation coefficient was used for correlation analyses. Data are presented as means ± SEM. Statistical significance was accepted at p<0.05.
Results
Baseline characteristics
At baseline, HbA1c and fasting levels of plasma glucose, triglycerides and serum insulin and C-peptide were higher in men with type 2 diabetes than in both obese and lean men (Table 1), whereas body weight, BMI, waist, waist-hip-ratio (WHR), total fat mass and LBM were higher in both obese groups compared with the lean group. VO2max per kg body weight was lower in men with type 2 diabetes compared to both obese and lean men (all p<0.001), but also lower in obese than in lean men (p=0.02) (Table 1). However, the self-reported levels of physical activity were not different between the three groups (see supplementary material (Table S1).
The levels of insulin-stimulated GDR and NOX were ~40% and ~49% lower, respectively (all P<0.01), whereas the level of insulin-suppressed LOX was ~75-90% higher (all p<0.05) in men with type 2 diabetes compared to both obese and lean men (Table 2). The level of insulin-stimulated RER was slightly lower in men with T2D compared with lean men, and the ability to increase RER in response to insulin (ΔRER) was reduced in men with T2D compared to both obese and lean men. No differences in GOX or HGP were observed between the groups.
Compliance to the HIIT-protocol
The adherence was comparable between groups (all P>0.66), with an attendance rate above 95% in all three groups (see supplementary material, Table S2). There were no injuries reported during the 8-week HIIT. Although training volume increased from 2 to 5 blocks of 5 x 1-min intervals during the 8-week intervention, the participants in all three groups trained at a comparable intensity (% of max heart rate) throughout the training period, and managed to increase the workload in both cycling (2-3%) and rowing (7-10%) from week 1-4 to 5-8 (see supplementary material, Table S2).
Effect of HIIT on VO2max and body composition
VO2max (l O2/min) increased by ~15% in the diabetic group (P<0.001), ~8% in the obese group (p=0.002) and ~10% in the lean group (p=0.001) in response to HIIT (Table 1 and Figure 2B). There was considerable heterogeneity in the response of VO2max to HIIT in all groups (Figure 2F). However, only a few participants (n=5) did not increase VO2max. In all three groups, weight, BMI, waist and WHR were slightly reduced (all p<0.05) in response to HIIT (Table 1). The relatively small HIIT-induced reduction in weight was explained by a larger average decrease in total fat mass (1.6 to 2.3 kg; all p<0.05) than the increase in LBM (0.6 to 1.5 kg; all p<0.05) in each group (Table 1 and Figures 2C, D). There were no differences in these HIIT-induced responses between the groups.
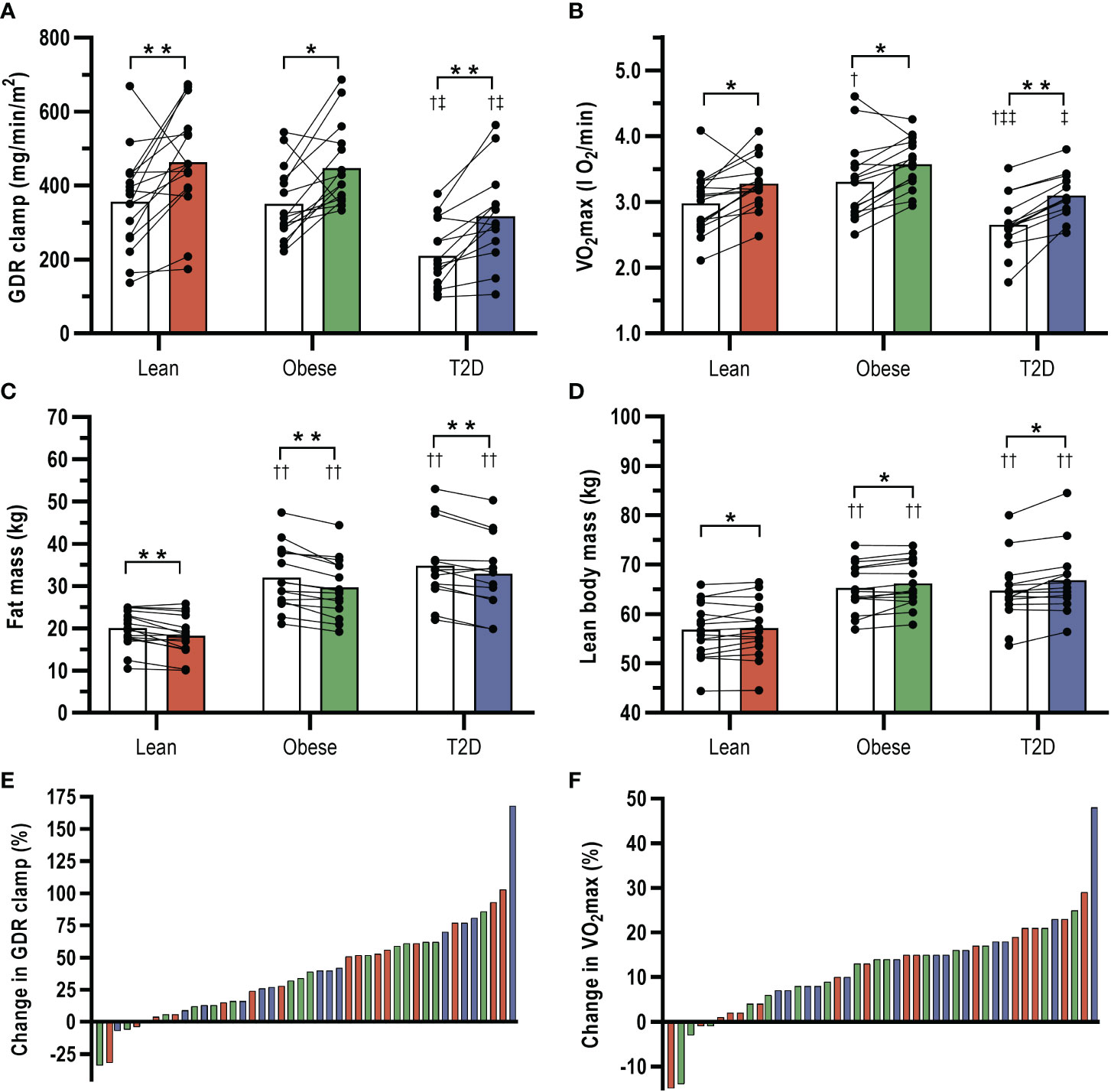
Figure 2 Effects of 8-weeks high-intensity interval training (HIIT) combining cycling and rowing on (A) insulin-stimulated glucose disposal rates (GDR clamp), (B) VO2max, (C) total fat mass, and (D) total lean body mass in patients with type 2 diabetes (T2D) and glucose tolerant obese and lean individuals. White bars show pre-training data and colored bars post-training data. (E, F) interindividual variability in the changes in GDR clamp and VO2max in response to HIIT in T2D (blue bars), obese (grey bars) and lean (red bars). Data are means ± SEM. *p<0.05 and **p<0.001 vs. pre-training, †p<0.05 and ††p<0.001 vs. lean, ‡p<0.05 and ‡‡p<0.001 vs. obese.
Glycemic control and lipid profile
HIIT induced a clinically relevant reduction in HbA1c (4 ± 2 mmol/mol; (p=0.017) and fasting plasma glucose (1.0 ± 0.4 mmol/l; p<0.001) in men with type 2 diabetes (Table 1). In obese men, HbA1c and fasting plasma glucose were also slightly reduced in response to HIIT, and a small reduction in HbA1c was also observed in lean men post-training (Table 1), but the HIIT-induced reductions in HbA1c and fasting plasma glucose were larger in the diabetic versus the non-diabetic groups (all p<0.05). In response to HIIT, plasma HDL increased (p=0.002) in the lean group, and plasma LDL decreased (p=0.016) in the obese group, whereas plasma triglycerides and total cholesterol were unaffected by HIIT in all groups. There were no differences in these responses between the groups.
Effect of HIIT on insulin sensitivity and substrate metabolism
In response to 8-week HIIT, insulin-stimulated GDR (mg/min/m2) increased by 42% in men with type 2 diabetes (p<0.001), 27% in obese men (p=0.001) and 29% in lean men (p<0.001) (Table 2 and Figure 2A). There was a large interindividual variability in the response to HIIT in all three groups, but only a few participants (n=6) did not increase insulin-stimulated GDR (Figure 2E). Post-training insulin-stimulated GDR was ~30% lower in men with type 2 diabetes compared to both obese and lean men (p<0.01). However, post-training insulin-stimulated GDR in the diabetic group was not different from pre-training insulin-stimulated GDR in either the obese (p=0.400) or the lean group (p=0.483). Similar results were observed with estimations of GIR and GDR in relation to the total lean body mass (Table 2). The magnitude of the HIIT-induced increases in insulin-stimulated GDR was entirely explained by accompanying increases in insulin-stimulated NOX in all three groups (all p<0.001) as opposed to no HIIT-induced changes in insulin-stimulated GOX (Table 2). While insulin-suppressed LOX was unaffected by HIIT in men with type 2 diabetes and lean men, it increased in obese men. However, post-training insulin-suppressed LOX was only higher in men with type 2 diabetes compared with lean men (Table 2). Basal GOX decreased and basal LOX increased in response to HITT in men with T2D, and this caused a reduction in basal RER. Therefore, the attenuated ΔRER observed in men with T2D at baseline compared to the other groups were not present after HIIT. HIIT did not cause changes in HGP or the ability of insulin to suppress HGP (ΔHGP) in any of the groups. There were no differences in any of the above HIIT-induced responses between the groups.
Correlation analyses
To test whether changes in the different exercise response variables were correlated, we examined the relationship between the HIIT-induced changes in VO2max, insulin sensitivity, body composition and HbA1c in the pooled total cohort (n=44) as well as in each group. The HIIT-induced improvement in VO2max did not correlate with the increase in insulin-stimulated GDR either in the total cohort (r=-0.14, p=0.36) or any of the groups. Moreover, no correlations were found between the HIIT-induced increases in neither VO2max nor insulin-stimulated GDR and the changes in HbA1c, BMI, fat mass or LBM in the total cohort. However, the HIIT-induced increase in insulin-stimulated GDR correlated with the reduction in BMI (r=-0.61, p=0.027) and tended to correlate with the decrease in HbA1c (r=-0.51, p=0.077) in the diabetic group, but not in the lean and obese groups.
Discussion
In the present study, we examined the effects of a novel HIIT-protocol combining rowing and cycling in men with type 2 diabetes compared with glucose-tolerant obese and lean men. Our main finding is that this HIIT-protocol markedly improved insulin sensitivity with intact responses in obese men with and without type 2 diabetes. This was accompanied by, but did not correlate with, equally improved VO2max and body composition in all groups. Moreover, we found a clinically relevant decrease in HbA1c in patients with type 2 diabetes. Importantly, we observed a high adherence rate in each group and no injuries were reported. These results provide evidence that a supervised, non-weight-bearing HIIT-protocol involving a large muscle mass and several muscle groups is a promising mode of exercise training in sedentary individuals with obesity or type 2 diabetes.
We and others have previously reported increases in insulin-stimulated GDR by ~10-20% in obese individuals with and without type 2 diabetes in response to 8-10 weeks supervised endurance training on cycle ergometers or treadmill (4, 28). However, in young, sedentary lean men a similar 10-week supervised endurance training protocol increased insulin sensitivity by ~30% (29), suggesting a lower response to exercise in obesity and type 2 diabetes as reported previously (18). Here, we show that 8-weeks supervised HIIT combining rowing and cycling improved insulin sensitivity by ~30-40% not only in lean glucose-tolerant men, but also in obesity and type 2 diabetes with the same magnitude. While comparisons of the magnitude of the effects in different exercise studies should be done with caution due to differences in methodology, our HIIT-protocol did seem to increase insulin sensitivity ~2-fold more than previously observed after endurance training in individuals with type 2 diabetes or obesity (4, 28), and, importantly, there was no evidence of exercise resistance. Interestingly, after completing the HIIT-protocol, the men with type 2 diabetes achieved a level of insulin sensitivity and also metabolic flexibility (ΔRER) similar to obese and lean men at baseline.
Recent studies have compared the effect of HIIT with endurance training on insulin sensitivity in patients with type 2 diabetes using surrogate markers of insulin sensitivity such as HOMA-IR (23, 30). In one study, 11-weeks of supervised HIIT on cycle ergometers reduced HOMA-IR by ~20-25%, whereas no change was observed after endurance training (23). In contrast, another study reported similar decreases in HOMA-IR by ~20% after 12-weeks supervised HIIT and endurance training on treadmills (30). Thus, it appears that HIIT has at least the same effect on HOMA-IR as endurance training in individuals with type 2 diabetes. However, only a few studies have investigated the effect of HIIT on insulin sensitivity using the hyperinsulinemic-euglycemic clamp. In young and older individuals, 6-12 weeks of supervised HIIT on cycle ergometers or treadmills increased insulin-stimulated glucose uptake by ~11-20% (19, 20), and in obese sedentary individuals, two weeks of sprint interval training on cycle ergometers increased insulin-stimulated GIR by ~25% (31). Taken together, these previous reports suggest that the 30-40% increase in insulin sensitivity observed in our study may not solely be ascribed to the choice of HIIT versus endurance training. Based on studies showing that insulin sensitivity is only increased in exercised muscles (15, 25), and perhaps even decreased in non-exercised muscle groups in response to an acute exercise bout (24), we designed our HIIT-protocol to activate both lower and upper body muscle groups. Indeed, our results lend support to the hypothesis that recruitment of several muscle groups is necessary to take full advantage of the insulin-sensitizing effect of exercise training, and this may to a large extent explain the marked increase in insulin sensitivity in response to our HIIT-protocol. However, we cannot exclude a contribution from other differences in the design, e.g. afternoon training sessions (32), and longer durations of the HIIT-sessions during the last weeks compared with other HIIT-protocols performed at similar intensities (19–21).
Cardiorespiratory fitness, measured as VO2max, is considered a key response variable of exercise training on cardiovascular and metabolic health (33, 34). Recent studies and meta-analyses have suggested a higher increase in VO2max in response to HIIT compared with endurance training in patients with type 2 diabetes (22, 23, 30, 35). In the present study, our HIIT-protocol increased VO2max equally in all three sedentary groups with a magnitude (8-15%) comparable to that observed in several studies of both HIIT and endurance training (13, 19, 20, 36, 37). Importantly, our findings demonstrate an intact HIIT-induced increase in VO2max in men with type 2 diabetes or obesity as compared to lean, sedentary individuals. However, in contrast to insulin sensitivity, we do not find a superior effect of HIIT or an additional effect of recruiting lower and upper body muscle groups on VO2max compared to previous endurance training studies (see above). This indicates that the metabolic response to this novel HIIT protocol goes beyond the effect on the cardiorespiratory fitness, which may be advantageous in the prevention and management of type 2 diabetes (33).
There is strong evidence that exercise training leads to variable responses or even a lack of response (non-response) in both healthy and diseased individuals (33, 34). In line, we demonstrate a high interindividual variability in the VO2max response to HIIT in each group, ranging from a 15% decrease to a 48% increase. Interestingly, the HIIT-induced changes in insulin-stimulated GDR and body composition showed a similar high degree of heterogeneity suggesting an association between these responses in the study cohort. However, except for an association between the increase in insulin sensitivity and the reduction in BMI in patients with type 2 diabetes, we found no correlations between the HIIT-induced changes in VO2max, insulin sensitivity or body composition either in the total cohort or in the individual groups. These findings are consistent with the current view that an adaptation to exercise training in one outcome does not necessarily equal responses in other outcomes (33), and emphasize that the evaluation of an exercise training program should not rely on a single exercise response variable.
Body composition plays an important role in cardiovascular and metabolic health. However, several studies of patients with type 2 diabetes have been unable to demonstrate an effect of endurance training on weight, BMI and body composition (4, 16, 18, 28). Moreover, many studies comparing the effect of HIIT versus endurance training in patients with type 2 diabetes could not demonstrate superior effects of HIIT on weight, WHR, or body composition (23, 30, 36), although one study and recent meta-analyses indicate a better effect of HIIT on BMI and WHR (22, 35, 38). A recent study of 8-weeks HIIT on cycle ergometers demonstrated reduced BMI and abdominal fat mass, but also an undesirable reduction in LBM in patients with type 2 diabetes (37). In the present study, we demonstrate clinically relevant improvements of both total fat mass and LBM in response to our HIIT-protocol in men with type 2 diabetes and glucose-tolerant obese and lean men instructed to continue their habitual food intake. These changes were accompanied by smaller decreases in weight, BMI and WHR. Compared with the previous reports mentioned above, it appears likely that the recruitment of upper and lower body muscle groups rather than HIIT per se contributed to these clear improvements in body composition. As noted above, the changes in total fat mass and LBM did not correlate with the marked increase in insulin sensitivity suggesting other factors to be involved, e.g. intrinsic adaptations in skeletal muscle and adipose tissue function, which are not necessarily reflected by changes in muscle or fat mass.
A major challenge in the prevention and management of type 2 diabetes is a low engagement in physical activity (10, 39). Although a high adherence to exercise training is critical to obtain the desired effects on metabolic health, it is often not reported or highly variable (39). In our study, we observed a very high adherence to both targeted training intensity and training sessions in all three groups and no injuries were reported. The high degree of adherence is in part explained by the supervised training and lack of injuries (39), but also by fulfillment of reported motivators for exercise including enjoyment during exercise, training in small groups, achieving weight control, and use of heart rate monitors (40). The lack of injuries could be explained by the non-weight-bearing mode of exercise, time for recovery between training sessions and a progressive training program. Supervised training of the type studied here is of course more costly than home-based training, but is most likely necessary to achieve a high adherence and thereby maintain the beneficial effects of exercise training on metabolic health.
The strengths of this study include 1) the inclusion of a lean, healthy group to rule out attenuated responses due to obesity or type 2 diabetes, 2) the application of a HIIT-protocol recruiting several lower and upper body muscle groups to maximize the insulin-sensitizing effect, 3) the supervised, group-based training sessions and the non-weight-bearing approach to ensure adherence and lack of injuries, and 4) conduction of the majority of the training sessions in the afternoon, which may enhance the metabolic benefits (32, 41). However, this study also has limitations. First, we did not register the actual food intake of the participants and, therefore, cannot exclude that potential changes in their food intake influenced the results. Second, the duration of our HIIT-protocol may not capture the long-term effects of this training intervention. Third, the design of our study does not allow us to conclude whether the marked increase in insulin sensitivity was due to the HIIT-protocol, the recruitment of lower and upper body muscle groups or both. Finally, none of the men with type 2 diabetes included were on insulin treatment, and, our study only included men, which limits the generalizability of the results to women and type 2 diabetes patients with a higher degree of beta-cell failure. Other HIIT-studies including both men and women with or without type 2 diabetes have reported beneficial effects on insulin sensitivity, VO2max and/or body composition in the pooled cohort (19, 23, 42, 43). This indicates that we can also expect beneficial effects of our HIIT-protocol in women. However, further studies are warranted to establish whether the magnitude of the beneficial effects is similar in women.
In conclusion, our results demonstrate that a novel HIIT-protocol combining rowing and cycling efficiently improves insulin sensitivity, VO2max, and body composition with preserved responses in sedentary men with obesity and type 2 diabetes. The supervised training and non-weight-bearing mode of exercise ensured a high adherence rate and absence of injuries in all groups. In addition, the men with type 2 diabetes experienced a clinically relevant decrease in HbA1c. Our results demonstrate that a supervised HIIT-protocol engaging lower and upper body muscle groups is a well-tolerated and promising mode of exercise training in sedentary individuals with obesity or type 2 diabetes. However, longer-term studies in larger cohorts including both men and women are needed to fully evaluate the metabolic and cardiovascular benefits of this HIIT protocol.
Data availability statement
The datasets for this manuscript are not publicly available but may be requested by researchers who have the relevant legal permissions from the Danish Data Protection Agency. Requests to access the datasets should be directed to the corresponding author.
Ethics statement
The studies involving human participants were reviewed and approved by Regional Scientific Ethical Committees for Southern Denmark. The patients/participants provided their written informed consent to participate in this study.
Author contributions
MP, KJ, NØ and KH contributed to the conception and design of the study. MP recruited the eligible participants and conducted the metabolic studies. MA and EW performed the supervised training, VO2max tests and DXA scanning. MP, MA, EW, NØ and KH analysed and interpreted data, and MP and KH wrote the manuscript. All authors have revised the manuscript critically for important intellectual content and given final approval of the version to be published. KH and NØ are guarantors of this work, and as such had full access to all the data in the study and takes full responsibility for the integrity of the data and the accuracy of the data analysis.
Funding
This study was supported by grants from the Region of Southern Denmark, Odense University Hospital, the Novo Nordisk Foundation, University of Southern Denmark, Christenson-Cesons Family Fund and from The Sawmill Owner Jeppe Juhl and wife Ovita Juhl Memorial Foundation. The funders had no role in the study design, data collection and analysis, decision to publish, or preparation of the manuscript.
Acknowledgments
We would like to thank L. Hansen and C. B. Olsen at the Steno Diabetes Center Odense, Odense University Hospital for their skilled technical assistance. We thank J. V. Stidsen at the Steno Diabetes Center Odense, Odense University Hospital for analytical and statistical support. The Odense Patient data Explorative Network (OPEN) is acknowledged for statistical support and access to data storage in REDCap. Figure 1 has been designed using “Rowing Machine” and “Gym Cycling Machine” icons by Gan Khoon Lay from the Noun Project and resources from Flaticon.com.
Conflict of interest
The authors declare that the research was conducted in the absence of any commercial or financial relationships that could be construed as a potential conflict of interest.
Publisher’s note
All claims expressed in this article are solely those of the authors and do not necessarily represent those of their affiliated organizations, or those of the publisher, the editors and the reviewers. Any product that may be evaluated in this article, or claim that may be made by its manufacturer, is not guaranteed or endorsed by the publisher.
Supplementary material
The Supplementary Material for this article can be found online at: https://www.frontiersin.org/articles/10.3389/fendo.2022.1032235/full#supplementary-material
Abbreviations
FFA, Free fatty acid; GDR, Glucose disposal rate; GIR, Glucose infusion rate; GOX, Glucose oxidation; HGP, Hepatic glucose production; HIIT, High-intensity interval training; LBM, Lean body mass; LOX, Lipid oxidation; MCC, Maximal cycling capacity; NOX, Non-oxidative glucose metabolism; IPAQ, International physical activity questionnaire; RER, Respiratory exchange ratio; VO2max, Maximal oxygen consumption; W, Watt.
References
1. Reaven GM. Insulin resistance: The link between obesity and cardiovascular disease. Med Clin North Am (2011) 95(5):875–92. doi: 10.1016/j.mcna.2011.06.002
2. Højlund K, Frystyk J, Levin K, Flyvbjerg A, Wojtaszewski JF, Beck-Nielsen H. Reduced plasma adiponectin concentrations may contribute to impaired insulin activation of glycogen synthase in skeletal muscle of patients with type 2 diabetes. Diabetologia (2006) 49(6):1283–91. doi: 10.1007/s00125-006-0240-5
3. Hojlund K. Metabolism and insulin signaling in common metabolic disorders and inherited insulin resistance. Dan Med J (2014) 61(7):B4890.
4. Vind BF, Pehmoller C, Treebak JT, Birk JB, Hey-Mogensen M, Beck-Nielsen H, et al. Impaired insulin-induced site-specific phosphorylation of Tbc1 domain family, member 4 (Tbc1d4) in skeletal muscle of type 2 diabetes patients is restored by endurance exercise-training. Diabetologia (2011) 54(1):157–67. doi: 10.1007/s00125-010-1924-4
5. Mogensen M, Sahlin K, Fernstrom M, Glintborg D, Vind BF, Beck-Nielsen H, et al. Mitochondrial respiration is decreased in skeletal muscle of patients with type 2 diabetes. Diabetes (2007) 56(6):1592–9. doi: 10.2337/db06-0981
6. Cusi K, Maezono K, Osman A, Pendergrass M, Patti ME, Pratipanawatr T, et al. Insulin resistance differentially affects the pi 3-kinase- and map kinase-mediated signaling in human muscle. J Clin Invest (2000) 105(3):311–20. doi: 10.1172/jci7535
7. Szendroedi J, Phielix E, Roden M. The role of mitochondria in insulin resistance and type 2 diabetes mellitus. Nat Rev Endocrinol (2011) 8(2):92–103. doi: 10.1038/nrendo.2011.138
8. Tuomilehto J, Lindstrom J, Eriksson JG, Valle TT, Hamalainen H, Ilanne-Parikka P, et al. Prevention of type 2 diabetes mellitus by changes in lifestyle among subjects with impaired glucose tolerance. N Engl J Med (2001) 344(18):1343–50. doi: 10.1056/nejm200105033441801
9. Kanaley JA, Colberg SR, Corcoran MH, Malin SK, Rodriguez NR, Crespo CJ, et al. Exercise/Physical activity in individuals with type 2 diabetes: A consensus statement from the American college of sports medicine. Med Sci Sports Exerc (2022) 54(2):353–68. doi: 10.1249/mss.0000000000002800
10. O'Hagan C, De Vito G, Boreham CA. Exercise prescription in the treatment of type 2 diabetes mellitus : Current practices, existing guidelines and future directions. Sports Med (2013) 43(1):39–49. doi: 10.1007/s40279-012-0004-y
11. Mogensen M, Vind BF, Højlund K, Beck-Nielsen H, Sahlin K. Maximal lipid oxidation in patients with type 2 diabetes is normal and shows an adequate increase in response to aerobic training. Diabetes Obes Metab (2009) 11(9):874–83. doi: 10.1111/j.1463-1326.2009.01063.x
12. Phielix E, Meex R, Moonen-Kornips E, Hesselink MK, Schrauwen P. Exercise training increases mitochondrial content and ex vivo mitochondrial function similarly in patients with type 2 diabetes and in control individuals. Diabetologia (2010) 53(8):1714–21. doi: 10.1007/s00125-010-1764-2
13. Hey-Mogensen M, Højlund K, Vind BF, Wang L, Dela F, Beck-Nielsen H, et al. Effect of physical training on mitochondrial respiration and reactive oxygen species release in skeletal muscle in patients with obesity and type 2 diabetes. Diabetologia (2010) 53(9):1976–85. doi: 10.1007/s00125-010-1813-x
14. Wang Y, Simar D, Fiatarone Singh MA. Adaptations to exercise training within skeletal muscle in adults with type 2 diabetes or impaired glucose tolerance: A systematic review. Diabetes Metab Res Rev (2009) 25(1):13–40. doi: 10.1002/dmrr.928
15. Frosig C, Rose AJ, Treebak JT, Kiens B, Richter EA, Wojtaszewski JF. Effects of endurance exercise training on insulin signaling in human skeletal muscle: Interactions at the level of phosphatidylinositol 3-kinase, akt, and As160. Diabetes (2007) 56(8):2093–102. doi: 10.2337/db06-1698
16. Christ-Roberts CY, Pratipanawatr T, Pratipanawatr W, Berria R, Belfort R, Kashyap S, et al. Exercise training increases glycogen synthase activity and Glut4 expression but not insulin signaling in overweight nondiabetic and type 2 diabetic subjects. Metabolism (2004) 53(9):1233–42. doi: 10.1016/j.metabol.2004.03.022
17. Stephens NA, Sparks LM. Resistance to the beneficial effects of exercise in type 2 diabetes: Are some individuals programmed to fail? J Clin Endocrinol Metab (2015) 100(1):43–52. doi: 10.1210/jc.2014-2545
18. Burns N, Finucane FM, Hatunic M, Gilman M, Murphy M, Gasparro D, et al. Early-onset type 2 diabetes in obese white subjects is characterised by a marked defect in beta cell insulin secretion, severe insulin resistance and a lack of response to aerobic exercise training. Diabetologia (2007) 50(7):1500–8. doi: 10.1007/s00125-007-0655-7
19. Robinson MM, Dasari S, Konopka AR, Johnson ML, Manjunatha S, Esponda RR, et al. Enhanced protein translation underlies improved metabolic and physical adaptations to different exercise training modes in young and old humans. Cell Metab (2017) 25(3):581–92. doi: 10.1016/j.cmet.2017.02.009
20. Søgaard D, Lund MT, Scheuer CM, Dehlbaek MS, Dideriksen SG, Abildskov CV, et al. High-intensity interval training improves insulin sensitivity in older individuals. Acta Physiol (Oxf) (2018) 222(4):e13009. doi: 10.1111/apha.13009
21. Ryan BJ, Schleh MW, Ahn C, Ludzki AC, Gillen JB, Varshney P, et al. Moderate-intensity exercise and high-intensity interval training affect insulin sensitivity similarly in obese adults. J Clin Endocrinol Metab (2020) 105(8):e2941–59. doi: 10.1210/clinem/dgaa345
22. De Nardi AT, Tolves T, Lenzi TL, Signori LU, Silva A. High-intensity interval training versus continuous training on physiological and metabolic variables in prediabetes and type 2 diabetes: A meta-analysis. Diabetes Res Clin Pract (2018) 137:149–59. doi: 10.1016/j.diabres.2017.12.017
23. Winding KM, Munch GW, Iepsen UW, Van Hall G, Pedersen BK, Mortensen SP. The effect on glycaemic control of low-volume high-intensity interval training versus endurance training in individuals with type 2 diabetes. Diabetes Obes Metab (2018) 20(5):1131–9. doi: 10.1111/dom.13198
24. Steenberg DE, Hingst JR, Birk JB, Thorup A, Kristensen JM, Sjøberg KA, et al. A single bout of one-legged exercise to local exhaustion decreases insulin action in nonexercised muscle leading to decreased whole-body insulin action. Diabetes (2020) 69(4):578–90. doi: 10.2337/db19-1010
25. Wojtaszewski JF, Hansen BF, Gade, Kiens B, Markuns JF, Goodyear LJ, et al. Insulin signaling and insulin sensitivity after exercise in human skeletal muscle. Diabetes (2000) 49(3):325–31. doi: 10.2337/diabetes.49.3.325
26. Devlin JT, Hirshman M, Horton ED, Horton ES. Enhanced peripheral and splanchnic insulin sensitivity in niddm men after single bout of exercise. Diabetes (1987) 36(4):434–9. doi: 10.2337/diab.36.4.434
27. Mikines KJ, Sonne B, Farrell PA, Tronier B, Galbo H. Effect of physical exercise on sensitivity and responsiveness to insulin in humans. Am J Physiol (1988) 254(3 Pt 1):E248–59. doi: 10.1152/ajpendo.1988.254.3.E248
28. De Filippis E, Cusi K, Ocampo G, Berria R, Buck S, Consoli A, et al. Exercise-induced improvement in vasodilatory function accompanies increased insulin sensitivity in obesity and type 2 diabetes mellitus. J Clin Endocrinol Metab (2006) 91(12):4903–10. doi: 10.1210/jc.2006-1142
29. Christensen B, Nellemann B, Larsen MS, Thams L, Sieljacks P, Vestergaard PF, et al. Whole body metabolic effects of prolonged endurance training in combination with erythropoietin treatment in humans: A randomized placebo controlled trial. Am J Physiol Endocrinol Metab (2013) 305(7):E879–89. doi: 10.1152/ajpendo.00269.2013
30. Mitranun W, Deerochanawong C, Tanaka H, Suksom D. Continuous vs interval training on glycemic control and macro- and microvascular reactivity in type 2 diabetic patients. Scand J Med Sci Sports (2014) 24(2):e69–76. doi: 10.1111/sms.12112
31. Richards JC, Johnson TK, Kuzma JN, Lonac MC, Schweder MM, Voyles WF, et al. Short-term sprint interval training increases insulin sensitivity in healthy adults but does not affect the thermogenic response to beta-adrenergic stimulation. J Physiol (2010) 588(Pt 15):2961–72. doi: 10.1113/jphysiol.2010.189886
32. Savikj M, Gabriel BM, Alm PS, Smith J, Caidahl K, Björnholm M, et al. Afternoon exercise is more efficacious than morning exercise at improving blood glucose levels in individuals with type 2 diabetes: A randomised crossover trial. Diabetologia (2019) 62(2):233–7. doi: 10.1007/s00125-018-4767-z
33. Sparks LM. Exercise training response heterogeneity: Physiological and molecular insights. Diabetologia (2017) 60(12):2329–36. doi: 10.1007/s00125-017-4461-6
34. Ross R, Goodpaster BH, Koch LG, Sarzynski MA, Kohrt WM, Johannsen NM, et al. Precision exercise medicine: Understanding exercise response variability. Br J Sports Med (2019) 53(18):1141–53. doi: 10.1136/bjsports-2018-100328
35. Lora-Pozo I, Lucena-Anton D, Salazar A, Galán-Mercant A, Moral-Munoz JA. Anthropometric, cardiopulmonary and metabolic benefits of the high-intensity interval training versus moderate, low-intensity or control for type 2 diabetes: Systematic review and meta-analysis. Int J Environ Res Public Health (2019) 16(22):4524. doi: 10.3390/ijerph16224524
36. Baasch-Skytte T, Lemgart CT, Oehlenschlager MH, Petersen PE, Hostrup M, Bangsbo J, et al. Efficacy of 10-20-30 training versus moderate-intensity continuous training on Hba1c, body composition and maximum oxygen uptake in Male patients with type 2 diabetes: A randomized controlled trial. Diabetes Obes Metab (2020) 22(5):767–78. doi: 10.1111/dom.13953
37. Madsen SM, Thorup AC, Overgaard K, Jeppesen PB. High intensity interval training improves glycaemic control and pancreatic beta cell function of type 2 diabetes patients. PloS One (2015) 10(8):e0133286. doi: 10.1371/journal.pone.0133286
38. Støa EM, Meling S, Nyhus LK, Glenn S, Mangerud KM, Helgerud J, et al. High-intensity aerobic interval training improves aerobic fitness and Hba1c among persons diagnosed with type 2 diabetes. Eur J Appl Physiol (2017) 117(3):455–67. doi: 10.1007/s00421-017-3540-1
39. MacDonald CS, Ried-Larsen M, Soleimani J, Alsawas M, Lieberman DE, Ismail AS, et al. A systematic review of adherence to physical activity interventions in individuals with type 2 diabetes. Diabetes Metab Res Rev (2021) 37(8):e3444. doi: 10.1002/dmrr.3444
40. Korkiakangas EE, Alahuhta MA, Husman PM, Keinanen-Kiukaanniemi S, Taanila AM, Laitinen JH. Motivators and barriers to exercise among adults with a high risk of type 2 diabetes–a qualitative study. Scand J Caring Sci (2011) 25(1):62–9. doi: 10.1111/j.1471-6712.2010.00791.x
41. Mancilla R, Brouwers B, Schrauwen-Hinderling VB, Hesselink MKC, Hoeks J, Schrauwen P. Exercise training elicits superior metabolic effects when performed in the afternoon compared to morning in metabolically compromised humans. Physiol Rep (2021) 8(24):e14669. doi: 10.14814/phy2.14669
42. Karstoft K, Winding K, Knudsen SH, James NG, Scheel MM, Olesen J, et al. Mechanisms behind the superior effects of interval vs continuous training on glycaemic control in individuals with type 2 diabetes: A randomised controlled trial. Diabetologia (2014) 57(10):2081–93. doi: 10.1007/s00125-014-3334-5
43. Sjöros TJ, Heiskanen MA, Motiani KK, Löyttyniemi E, Eskelinen JJ, Virtanen KA, et al. Increased insulin-stimulated glucose uptake in both leg and arm muscles after sprint interval and moderate-intensity training in subjects with type 2 diabetes or prediabetes. Scand J Med Sci Sports (2018) 28(1):77–87. doi: 10.1111/sms.12875
Keywords: body composition, glucose disposal rate (GDR), high-intensity interval training (HIIT), hyperinsulinemic-euglycemic clamp, obesity, type 2 diabetes, maximal oxygen consumption (VO2max)
Citation: Petersen MH, de Almeida ME, Wentorf EK, Jensen K, Ørtenblad N and Højlund K (2022) High-intensity interval training combining rowing and cycling efficiently improves insulin sensitivity, body composition and VO2max in men with obesity and type 2 diabetes. Front. Endocrinol. 13:1032235. doi: 10.3389/fendo.2022.1032235
Received: 30 August 2022; Accepted: 17 October 2022;
Published: 01 November 2022.
Edited by:
Steven K. Malin, Rutgers, The State University of New Jersey, United StatesReviewed by:
Matthew M Robinson, Oregon State University, United StatesFaidon Magkos, University of Copenhagen, Denmark
Copyright © 2022 Petersen, de Almeida, Wentorf, Jensen, Ørtenblad and Højlund. This is an open-access article distributed under the terms of the Creative Commons Attribution License (CC BY). The use, distribution or reproduction in other forums is permitted, provided the original author(s) and the copyright owner(s) are credited and that the original publication in this journal is cited, in accordance with accepted academic practice. No use, distribution or reproduction is permitted which does not comply with these terms.
*Correspondence: Kurt Højlund, a3VydC5ob2VqbHVuZEByc3lkLmRr