- 1Hull IVF Unit, Women and Children's Hospital, Hull Royal Infirmary, Hull, United Kingdom
- 2Centre for Diabetes and Metabolic Research, Hull York Medical School, University of Hull, Hull, United Kingdom
- 3Department of Statistics, Hull York Medical School, University of Hull, Hull, United Kingdom
- 4Weill Cornell Medicine Qatar, Doha, Qatar
- 5Sidra Medical and Research Centre, Doha, Qatar
- 6Antidoping Laboratory Qatar, Doha, Qatar
Objective: The relationship between fertilization rates and 1,25-dihydroxyvitamin D (1,25(OH)2D3), 25-hydroxyvitamin D2 (25(OH)D2), 25-hydroxyvitamin D3 (25(OH)D3), 24,25-dihydroxyvitamin D (24,25(OH)2D3), and 25-hydroxy-3epi-Vitamin D3 (3epi25(OH)D3) concentrations in age and weight matched women with and without PCOS was studied.
Methods: Fifty nine non-obese women, 29 with PCOS, and 30 non-PCOS undergoing IVF, matched for age and weight were included. Serum vitamin D metabolites were taken the menstrual cycle prior to commencing controlled ovarian hyperstimulation.
Results: Vitamin D metabolites did not differ between PCOS and controls; however, 25(OH)D3 correlated with embryo fertilization rates in PCOS patients alone (p = 0.03). For all subjects, 3epi25(OH)D3 correlated with fertilization rate (p < 0.04) and negatively with HOMA-IR (p < 0.02); 25(OH)D2 correlated with cleavage rate, G3D3 and blastocyst (p < 0.05; p < 0.009; p < 0.002, respectively). 24,25(OH)2D3 correlated with AMH, antral follicle count, eggs retrieved and top quality embryos (G3D3) (p < 0.03; p < 0.003; p < 0.009; p < 0.002, respectively), and negatively with HOMA-IR (p < 0.01). 1,25(OH)2D3 did not correlate with any of the metabolic or embryo parameters. In slim PCOS, 25(OH)D3 correlated with increased fertilization rates in PCOS, but other vitamin D parameters did not differ to matched controls.
Conclusion: 3epi25(OH)D3, 25(OH)D2, and 24,25(OH)2D3, but not 1,25(OH)2D3, were associated with embryo parameters suggesting that vitamin D metabolites other than 1,25(OH)2D3 are important in fertility.
Introduction
Polycystic ovarian syndrome (PCOS) is one of the most common endocrine disorders amongst women of reproductive age affecting 9–21% of the female population and is the main cause of anovulatory infertility (1). It is associated with clinical and biochemical hyperandrogenism, and insulin resistance (IR) in PCOS is associated with obesity, type 2 diabetes, and hypercholestrolemia (2). Vitamin D levels are low in 67–85% of women with PCOS (3), which are suggested to exacerbate IR and the free androgen index (FAI) in PCOS (4, 5). IR itself is both independent of and exacerbated by obesity and is present in 65–80% of women with PCOS (6) and may be improved by vitamin D replacement (7). In a recent meta-analysis, it was shown that in weight matched PCOS women, vitamin D was negatively predicted by weight hip ratio, glucose and LH (8).
Vitamin D deficiency has become the most common nutritional deficiency throughout the world (9). Studies of sub-fertile women have demonstrated that vitamin D deficiency is present in between 58 and 91% of cases (9–12). Obesity can exacerbate vitamin D deficiency, as a result of decreased bioavailability from cutaneous and dietary sources because of deposition in the body fat compartments (13).
Vitamin D3 (cholecalciferol) is endogenously produced or taken as a dietary supplement, whist vitamin D2 (ergocalciferol) is derived from the diet (primarily from mushrooms and fungi), though both are hydroxylated to 25(OH)D3 or 25(OH)D2 by multiple 25-hydroxylases (14, 15) (Figure 1). 25(OH)D is transported to the kidney and converted to either the active 1,25-dihydroxyvitamin D (1,25(OH)2D3) by 1 alpha hydroxylase, or to 24,25-dihydroxyvitamin D (24,25(OH)2D3), that is also active, by the 24 alpha hydroxylase, in the renal tubular and other cells widely in the body (Figure 1) (16). It has been recently reported that extrarenal tissues may also convert 25(OH)D to 1,25(OH)2D (17). 1,25(OH)2D binds to the vitamin D receptor (VDR) subsequently heterodimerizes with the retinoid X receptor for its action that may be effected in several hours (16); however, a more rapid action has been reported with binding membrane VDR or through the 1,25D3-membrane-associated, rapid response steroid-binding protein receptor with activation of protein kinases A and C (18). Vitamin D receptors have been located within structures of the female reproductive tract, including the ovary and endometrium (19, 20).
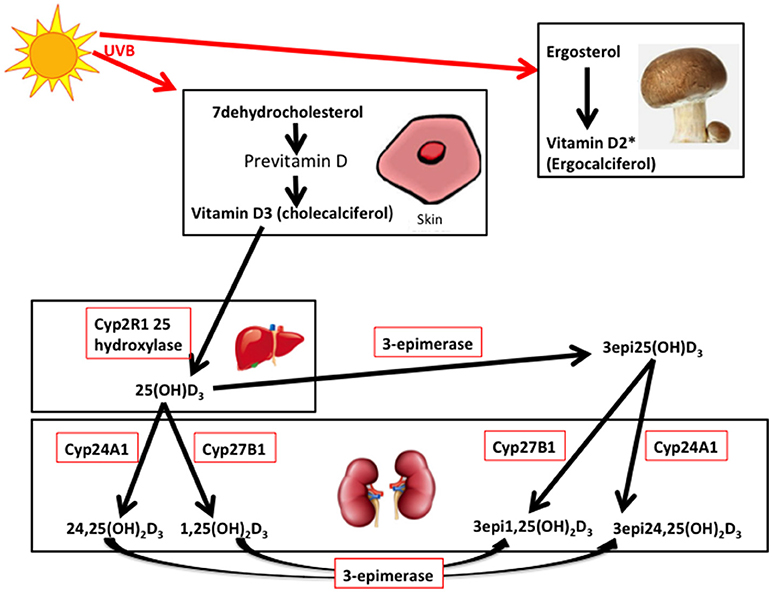
Figure 1. 7-dehydrocholesterol in the skin is converted to previtamin D3 and then is thermally isomerize to vitamin D3. Transport of vitamin D3 from the skin to the liver is via the Vitamin D binding protein (DBP) transports 25 hydroxy vitamin D (25(OH)D3) to the kidney. 25(OH)D3/DBP is filtered by the glomeruli and 25(OH)D3 is taken up into the tubular cells, following DBP binding to megalin, a transmembrane protein. 25(OH)D3 undergoes a second hydroxylation step by the 1-alpha-hydroxylase Cyp27B1, converting to the active 1α,25 (OH)2D3, whilst 24 hydroxylase Cyp27A1 converts to 24,25(OH)2D3. Keratinocytes contribute to the 3-epimerase activity converting 25(OH)D3 to 3-epi-25(OH)D3, and 1α,25 (OH)2D3 to 3-epi-1α,25 (OH)2D3, but the exact sites of activity remain unknown. 3epi25(OH)D3 is equally converted by Cyp27A1 and Cyp27B1 as 25(OH)D3. *Vitamin D2 derived from yeasts and fungi (mushrooms) is converted to 25(OH)D2 in the liver and to 1,25(OH)2D2 and 24,25(OH)2D2 in the kidney (adapted from 14, 16, and 21). It is unclear if the 3-epimers may be back converted.
Vitamin D2 is derived from the diet as ergocalciferol that has lower binding efficacy to VDR resulting in greater serum clearance, limiting the formation of 25(OH)D2, though 1,25(OH)2D2 has a high an affinity for VDR as 1,25(OH)2D3 (14, 16). In the United States and other countries vitamin D2 is available both as a supplement and as a pharmaceutical to treat vitamin D deficiency.
3- epimerase isomerizes the C-3 hydroxy group of the natural vitamin D from the α to the β orientation leading to 3epi25(OH)D3 (14, 21) that may be measured inadvertently whilst measuring 25(OH)D3 (22). 3epi25(OH)D3 is thought to be less potent physiologically as 25(OH)D3, and 1,25(OH)2-3-epi-D3 has less affinity to VDR thus less biologically active; however, the 3-epimer may be as potent as 1,25(OH)2D3 in other circumstances such as PTH suppression (14, 23) however, data is sparse on the biological potency of the C3 epimers.
Two IVF cohort studies have suggested that clinical pregnancy rates were significantly lower in women who were vitamin D deficient (24, 25), but no differences in the embryological data have been associated with 25(OH)D3 levels (25). However, it remains unknown if there is a relationship of baseline 1,25(0H)2D3, 25(OH)D3, 25(OH)D2, 24,25(0H)2D3, or its epimer 3epi25(OH)D3 to fertilization in non-obese PCOS subjects when age and weight are matched to control subjects, and therefore this study was undertaken.
Materials and Methods
This prospective cohort study was performed within the Hull IVF Unit, UK following approval by the Yorkshire and The Humber NRES ethical committee, UK and all gave their written informed consent. The PCOS subjects were recruited using the revised 2003 criteria (26), namely any 2 out of 3 criteria were met; menstrual disturbance (oligo or amenorrhoea), clinical and/or biochemical signs of androgenism and polycystic ovaries on ultrasound, with the exclusion of other conditions. All women were on folic acid 400 mcg daily but no other medication. Exclusion criteria were patients with diabetes, renal or liver insufficiency, acute or chronic infections, systemic inflammatory diseases, age < 20, age >45, known Immunological disease.
Sample Collection
A fasting blood sample was taken in the luteal phase of the cycle before commencing IVF treatment. The bloods were centrifuged at 3,500 g for 15 min and placed into aliquots and frozen at −80°C until analysis. The bloods were analyzed for FSH (Architect analyser, Abbott laboratories, Maidenhead, United Kingdom), SHBG, insulin (DPC Immulite 200 analyser, Euro/DPC, Llanberis United Kingdom), and plasma glucose (Synchron LX20 analyser, Beckman-Coulter, High Wycombe, United Kingdom). Free androgen index (FAI) was calculated by dividing the total testosterone by SHBG, and then multiplying by one hundred. Insulin resistance (IR) was calculated using the homeostasis model assessment (HOMA-IR). Serum vitamin D levels and testosterone were quantified using isotope-dilution liquid chromatography tandem mass spectrometry (LC-MS/MS). Vitamin D metabolites (1,25(OH)2D3), 25(OH)D2, 25(OH)D3, 24,25(OH)2D3 and 3epi25(OH)D3 and three labeled internal standards (d6-25(OH)D3, d6-1,25(OH)2D3 and d6-3-epi-25(OH)D3) were simultaneously extracted from 250 μL serum using supportive liquid-liquid extraction and Diels-Alder derivatization prior to LC-MS/MS analysis. Chromatographic separations were achieved using Hypersil Gold C18 column (150 × 2.1 mm; 1.9 μ) at flow rate 0.2 ml/min, operated in Electrospray Ionization (ESI) positive mode and analyzed by multiple reaction monitoring (MRM) method. The limit of quantification (LOQ) for 1,25(OH)2D were 10 pg/mL, 3-epi-25(OH)D, and 24,25(OH)2D were 50 pg/mL while 25(OH)D3 and 25(OH)D2 were 0.5 and 0.25 ng/mL, respectively. All methods employed were performed in accordance with the relevant guidelines and regulations.
All patients underwent a standard IVF antagonist protocol. The patients commenced their rFSH stimulation on day 2 of their menstrual cycle using either Merional (Pharmasure) or Gonal-F (Merck Serono). A GnRH antagonist (Cetrotide: Merck Serono) was used to prevent a premature LH surge.
The patients underwent ultrasound scans from day 7 to observe the ovarian response to stimulation and were repeated every 48 h. The scans were used to measure the diameters of the follicles thus observing response and follicle numbers. Final maturation was triggered when two or more leading follicles were ≥18 mm using human chorionic gonadotrophin [hCG, Pregnyl (Merck Sharp and Dohme)].
Transcervical embryo transfer was performed and embryos were classified using standard criteria (27) at the cleavage stage (day 2–3 after egg collection) and for blastocyst stage (day 5–6 after egg collection). Top Quality embryos on Day 3 as per Alpha Consensus (28). Embryo transfers were performed on either day 3 or ideally at day 5 (blastocyst) to give the best chance for implantation as this timing is similar compared to natural cycle embryos moving into the uterus.
Data Analysis and Statistics
Statistical analysis was performed using SPSS (v22, Chicago, Illinios). Descriptive data is presented as mean ± SD for continuous data and n (%) for categorical data. t-tests or Mann Whitney tests were used to compare means/medians where appropriate, and associations used Pearson's correlation or Spearman's correlation as appropriate. A p < 0.05 was considered to indicate statistical significance. There was no comparative study on which to base a formal power calculation; therefore, power and sample size for a pilot study was performed (29); therefore, to account for a minimum of 20 degrees-of-freedom to estimate effect size and variability a minimum of 25 patients per group were required to allow covariate adjustment.
Results
Baseline characteristics of the 59 patients are shown in Table 1 where is can be seen that patients were non-obese, age, and weight matched. There were significant differences in ovarian reserve parameters antral follicle count (AFC) and anti-Mullerian Hormone, (AMH), and androgen status between the groups, however there was no significant difference in fasting insulin, HOMA-IR or the vitamin D metabolites (Table 1).
There was a correlation between the levels of 25(OH)D3, and embryo fertilization rates in PCOS patients (r = 0.44; p = 0.03) that were not seen in the control group. However, between the PCOS and control groups there were no differences for any of the metabolic or embryo parameters for 25(OH)D2, 24R,25(OH)2D3, 1,25(OH)2D3, or 3epi25(OH)D3. When all of the subjects were combined there was a correlation between the levels of 25(OH)D2 and cleavage rate (r = 0.31; p = 0.05), G3D3 (r = 0.40; p = 0.009) and blastocyst (r = 0.40; p = 0.022); there was a correlation between 3epi25(OH)D3 and fertility rate (r = 0.33; p < 0.04) and a negative correlation with HOMA-IR (r = −0.33; p < 0.02); 24R,25(0H)2D3 correlated with AMH (r = 0.1; p = 0.03) antral follicle count (r = 0.2; p = 0.003), eggs retrieved (r = 0.14; p = 0.009) and G3D3 (r = 0.22; p = 0.002), and negatively with HOMA-IR (r = −0.07; p < 0.01). There was no correlation of the active 1,25(0H)2D3 with any of the metabolic or embryo parameters.
There was a correlation between the levels of 25(OH)D3 with both 24R,25(OH)2D3 and 3epi25(OH)D3 (r = 0.91, p < 0.001; r = 0.35, p < 0.015, respectively.
As a cohort, 25-hydroxyvitamin D levels were low did not differ between the controls and the PCOS group. The Endocrine Society defines vitamin D deficiency, insufficiency and replete as (≤20 ng/mL, 20–30 ng/mL and ≥30 ng/mL, respectively (30) that was reflected in controls and PCOS as, deficient, 51 vs. 41%; insufficient, 33 vs. 35%; deficient, 10 vs. 24%.
IVF cycle characteristics are represented in Table 2 showing that the PCOS group had significantly greater numbers of follicles aspirated and eggs retrieved compared to the controls, and the mean fertilization and cleavage rates were significantly higher for the PCOS group, though embryos quality did not differ.
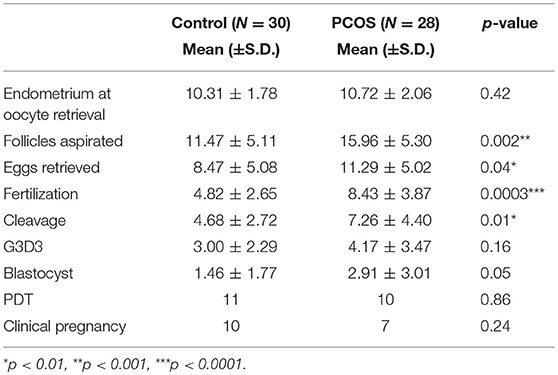
Table 2. Mean outcome data for stimulated ovarian cycle for Control and PCOS groups. G3D3: Top Quality embryos on Day 3 as per Alpha Consensus (28).
There was a significantly negative correlation between SHBG and 25-hydroxyvitamin D3 in the PCOS subjects, however after adjusting for BMI, SHBG was not significantly associated with 25-hydroxyvitamin D3.
Discussion
This study has shown that 25(OH)D3 was associated with higher fertility rates in PCOS compared to non-obese, age, and weight matched control subjects, but that this was not seen for the other vitamin D metabolites. This was surprising given that there was no difference in the 25(OH)D3 levels between the PCOS and control group; however, it is recognized that ova in PCOS may be at a less mature stage compared to normal and therefore there is a possibility that they may be more 25-hydroxyvitamin D responsive to allow those ovum within the stimulated follicles to reach a more mature stage prior to ovum retrieval, resulting in a greater capability to achieve fertilization. PCOS women typically produced more poor quality oocytes, with lower fertilization, cleavage and implantation rates (31, 32). The impaired oocyte maturation and resultant embryonic developmental competence in PCOS women is possibly due to the abnormal endocrine/paracrine functions and the environment within the follicle at the time of folliculogenesis (33, 34). No differences in the embryological data for 25(OH)D3 were found, in accord with others (25). It may have been speculated that the active 1,25(OH)2D3 may have a greater influence on fertility at higher levels, but this was not seen in this study, with no correlation with fertilization or embryo data. Vitamin D is involved in the regulation of AMH and FSH gene expression (31, 35), and high dose 25(OH)D3 has been shown to increase serum AMH levels in vitamin D insufficiency (36). In this study only the metabolite 24R,25(OH)2D3 correlated with AMH and antral follicle count; 24R,25(OH)2D3 is an active metabolite [It can be converted to 1,24,25-trihydroxyvitamin D3 through the C24 oxidation pathway (37)] as it has been shown to induce non-genomic signaling pathways and suppresses Apo A-1 in hep G cells (38), may have a physiological role in the growth plate formation (14), therefore a direct effect on the ovary cannot be excluded. However, 24,25 dihydroxyvitamin D is associated with blood levels of 25-hydroxyvitamin D and given that both were very significantly associated it is unlikely that 24R,25(OH)2D3 was having a unique biological effect and indeed was dependent on serum 25(OH)D3 levels.
There was a positive association for an increase in overall fertility rate with the 3epi25(OH)D3 that was not seen for the other vitamin D metabolites. Little is known about the epimers of vitamin D, with the assumption that they are biologically less potent (21, 23), but whilst that is likely for bone metabolism it may not be the case for ovarian effects. Epimers are compounds that have identical structure (and therefore identical molecular weight) with the exception of a stereochemical difference at one site. Other than the measurement of 3epi25(OH)D3 to enable the more accurate determination of 25(OH)D3, in children where they have been shown to be higher, there has been little research done on the C3 epimers (39) in order to know the significance of this observation on fertility, but it is of interest to note that serum lipids were discrepant for 25(OH)D epimeric forms suggesting a differential effect (40). However, 3epi25(OH)D3 is associated with blood levels of 25-hydroxyvitamin D and given that both were very significantly associated it is unlikely that 3epi25(OH)D3 was having a unique biological effect and indeed was dependent on serum 25(OH)D3 levels.
Whole group analysis for 25(OH)D2, but not 25(OH)D3 was positively associated with increased cleavage rate, G3D3 and blastocyst, though overall embryo quality did not differ. Whilst vitamin D3 supplements are better than vitamin D2 to raise vitamin D levels (41, 42), their biological effects may not be the same in different systems (43), and their differential effect in the ovary needs to be clarified. The role of vitamin D in fertilization remains controversial and the specific roles of 25-hydroxyvitamin D levels largely unknown. Observational studies have reported vitamin D levels within serum and follicular fluid to be highly correlated and that those with higher serum and follicular fluid levels of vitamin D had significantly higher clinical pregnancy rates (12). Other studies have found no correlation between serum and follicular fluid levels of vitamin D and IVF outcomes (10, 35, 44). Conversely, two cohort studies comparing serum vitamin D levels and pregnancy rates in women undergoing fresh IVF showed that clinical pregnancy rates were significantly lower in women who were vitamin D deficient (24, 25). Given the controversy, the implication of this data is that in slim PCOS women undergoing IVF that their vitamin D status should be determined, vitamin D replacement undertaken for those deficient prior to IVF may be of benefit, or at least do not harm, until future clarification becomes available.
These data suggest that vitamin D deficiency may not be a homogeneous entity but rather may depend on the different vitamin D metabolites present giving resultants effects, and therefore may account for the heterogeneity and controversy surrounding vitamin D deficiency effects and the response to replacement (42, 45). Whilst the effects of vitamin D and its metabolites on bone and calcium metabolism are well-known, vitamin D metabolite effects on other systems may not be directly equipotent for each metabolite or comparable. For example 1,25(OH)2-3-epi-D3 may be biologically less active than 1,25(OH)2D3, for increasing calcium, but may have greater PTH suppression (39). However, the kidneys produce 1,25-dihydroxyvitamin D for regulating calcium and bone metabolism (46). Therefore, measuring a blood level of 1,25-dihydroxyvitamin D may not reflect its other biologic functions, but rather local production of 1,25-dihydroxyvitamin D may have its major benefit (47). Our observation that higher blood levels of 25-hydroxyvitamin D are related to the outcome measures could also be due to the higher substrate availability for the local production of 1,25-dihydroxyvitamin D rather than 25-hydroxyvitamin D having a direct effect.
When weight and aged matched then there was no difference in vitamin D metabolite levels between PCOS and normal women. These results differ from previous studies that found Vitamin D deficiency to be more common in PCOS subjects (48); however, in this study the PCOS patients were specifically non-obese and it is well-recognized that vitamin D levels fall in obesity that may account for this observation (6). Negative correlations with HOMA-IR were seen for both 24R,25(OH)2D3, and 3epi25(OH)D3, but no association was seen for either 25(OH)D2 or 25(OH)D3, suggesting that an association with insulin resistance may depend on the metabolites present. There were no correlations between testosterone or oocyte quality in the PCOS patients in this study These observations are in accord with some studies, though not with others (4, 49); however, in those reported studies patients were not intentionally weight matched. High dose 25(OH)D3 replacement was not associated with an improvement in insulin sensitivity in PCOS subjects (50).
This study was specifically designed to look at the relationship of vitamin D levels with fertilization rates and therefore was not powered to look at pregnancy rates that would require a much larger sample population. Furthermore, the overall sample size is small and further work is needed, particularly to determine if these findings are true for the differing PCOS phenotypes within the Rotterdam criteria. However, the strengths of this study were that the patients were age and weight matched from the same ethnic background and that all of the vitamin D metabolites were measured by state of the art methods.
In conclusion, non-obese age and weight matched PCOS women showed that 25(OH)D3 was associated with the fertilization rate, compared to controls; however, vitamin D metabolites were associated with embryology parameters and HOMA-IR, suggesting a possible relationship between differing vitamin D metabolites, oocyte maturation and insulin sensitivity in non-obese PCOS patients.
Author Contributions
TC was involved in the study design, acquisition of data, analysis and interpretation of data, and paper drafting. HM, NA, and AL were involved in vitamin D analysis and drafting the manuscript. VA and SD were involved in analysis and interpretation of data, and paper drafting. SA, EK, SM, and TS were involved in the study design, supervision, paper drafting, and contributed to the interpretation of the data. All authors read and approved the final manuscript.
Funding
The publication of this article was funded by the Qatar National Library.
Conflict of Interest Statement
The authors declare that the research was conducted in the absence of any commercial or financial relationships that could be construed as a potential conflict of interest.
Acknowledgments
This work was part of an MD thesis and its publishing is in line with the University of Hull policy, and can be accessed online http://hydra.hull.ac.uk/resources/hull:14051 online (51).
References
1. Azziz R, Woods KS, Reyna R, Key TJ, Knochenhauer ES, Yildiz BO. The prevalence and features of the polycystic ovary syndrome in an unselected population. J Clin Endocrinol Metabol. (2004) 89:2745–9. doi: 10.1210/jc.2003-032046
2. Franks S. Polycystic ovary syndrome. N Engl J Med. (1995) 333:853–61. doi: 10.1056/NEJM199509283331307
3. Thomson RL, Spedding S, Buckley JD. Vitamin D in the aetiology and management of polycystic ovary syndrome. Clin Endocrinol. (2012) 77:343–50. doi: 10.1111/j.1365-2265.2012.04434.x
4. Ngo DT, Chan WP, Rajendran S, Heresztyn T, Amarasekera A, Sverdlov AL, et al. Determinants of insulin responsiveness in young women: impact of polycystic ovarian syndrome, nitric oxide, and vitamin D. Nitric Oxide (2011) 25:326–30. doi: 10.1016/j.niox.2011.06.005
5. Wehr E, Pilz S, Schweighofer N, Giuliani A, Kopera D, Pieber TR, et al. Association of hypovitaminosis D with metabolic disturbances in polycystic ovary syndrome. Eur J Endocrinol. (2009) 161:575–82. doi: 10.1530/EJE-09-0432
6. Diamanti-Kandarakis E, Dunaif A. Insulin resistance and the polycystic ovary syndrome revisited: an update on mechanisms and implications. Endocr Rev. (2012) 33:981–1030. doi: 10.1210/er.2011-1034
7. Asemi Z, Foroozanfard F, Hashemi T, Bahmani F, Jamilian M, Esmaillzadeh A. Calcium plus vitamin D supplementation affects glucose metabolism and lipid concentrations in overweight and obese vitamin D deficient women with polycystic ovary syndrome. Clin Nutr. (2015) 34:586–92. doi: 10.1016/j.clnu.2014.09.015
8. Bacopoulou F, Kolias E, Efthymiou V, Antonopoulos CN, Charmandari E. Vitamin D predictors in polycystic ovary syndrome: a meta-analysis. Eur J Clin Invest. (2017) 47:746–55. doi: 10.1111/eci.12800
9. Rudick B, Ingles S, Chung K, Stanczyk F, Paulson R, Bendikson K. Characterizing the influence of vitamin D levels on IVF outcomes. Hum Reprod. (2012) 27:3321–7. doi: 10.1093/humrep/des280
10. Anifandis GM, Dafopoulos K, Messini CI, Chalvatzas N, Liakos N, Pournaras S, et al. Prognostic value of follicular fluid 25-OH vitamin D and glucose levels in the IVF outcome. Reprod Biol Endocrinol. (2010) 8:91. doi: 10.1186/1477-7827-8-91
11. Rudick BJ, Ingles SA, Chung K, Stanczyk FZ, Paulson RJ, Bendikson KA. Influence of vitamin D levels on in vitro fertilization outcomes in donor-recipient cycles. Fertil Steril. (2014) 101:447–52. doi: 10.1016/j.fertnstert.2013.10.008
12. Ozkan S, Jindal S, Greenseid K, Shu J, Zeitlian G, Hickmon C, et al. Replete vitamin D stores predict reproductive success following in vitro fertilization. Fertil. Steril. (2010) 94:1314–9. doi: 10.1016/j.fertnstert.2009.05.019
13. Wortsman J, Matsuoka LY, Chen TC, Lu Z, Holick MF. Decreased bioavailability of vitamin D in obesity. Am J Clin Nutr. (2000) 72:690–3. doi: 10.1093/ajcn/72.3.690
14. Bikle DD. Vitamin D metabolism, mechanism of action, and clinical applications. Chem Biol. (2014) 21:319–29. doi: 10.1016/j.chembiol.2013.12.016
15. Sakaki T, Kagawa N, Yamamoto K, Inouye K. Metabolism of vitamin D3 by cytochromes P450. Front Biosci. (2005) 10:119–34. doi: 10.2741/1514
16. Christakos S, Dhawan P, Verstuyf A, Verlinden L, Carmeliet G. Vitamin D: metabolism, molecular mechanism of action, and pleiotropic effects. Physiol Rev. (2016) 96:365–408. doi: 10.1152/physrev.00014.2015
17. Adams JS, Rafison B, Witzel S, Reyes RE, Shieh A, Chun R, et al. Regulation of the extrarenal CYP27B1-hydroxylase. J Steroid Biochem Mol Biol. (2014) 144 Pt A:22–7. doi: 10.1016/j.jsbmb.2013.12.009
18. Lösel R, Wehling M. Nongenomic actions of steroid hormones. Nat Rev Mol Cell Biol. (2003) 4:46–55. doi: 10.1038/nrm1009
19. Agic A, Xu H, Altgassen C, Noack F, Wolfler MM, Diedrich K, et al. Relative expression of 1,25-dihydroxyvitamin D3 receptor, vitamin D 1 alpha-hydroxylase, vitamin D 24-hydroxylase, and vitamin D 25-hydroxylase in endometriosis and gynecologic cancers. Reprod Sci. (2007) 14:486–97. doi: 10.1177/1933719107304565
20. Viganò P, Lattuada D, Mangioni S, Ermellino L, Vignali M, Caporizzo E, et al. Cycling and early pregnant endometrium as a site of regulated expression of the vitamin D system. J Mol Endocrinol. (2006) 36:415–24. doi: 10.1677/jme.1.01946
21. Kamao M, Tatematsu S, Hatakeyama S, Sakaki T, Sawada N, Inouye K, et al. C-3 epimerization of vitamin D3 metabolites and further metabolism of C-3 epimers: 25-hydroxyvitamin D3 is metabolized to 3-epi-25-hydroxyvitamin D3 and subsequently metabolized through C-1alpha or C-24 hydroxylation. J Biol Chem. (2004) 279:15897–907. doi: 10.1074/jbc.M311473200
22. Schleicher RL, Encisco SE, Chaudhary-Webb M, Paliakov E, McCoy LF, Pfeiffer CM. Isotope dilution ultra performance liquid chromatography-tandem mass spectrometry method for simultaneous measurement of 25-hydroxyvitamin D2, 25-hydroxyvitamin D3 and 3-epi-25-hydroxyvitamin D3 in human serum. Clin Chim Acta (2011) 412:1594–9. doi: 10.1016/j.cca.2011.05.010
23. Molnár F, Sigüeiro R, Sato Y, Araujo C, Schuster I, Antony P, et al. 1alpha,25(OH)2-3-epi-vitamin D3, a natural physiological metabolite of vitamin D3: its synthesis, biological activity and crystal structure with its receptor. PLoS ONE (2011) 6:e18124. doi: 10.1371/journal.pone.0018124
24. Garbedian K, Boggild M, Moody J, Liu KE. Effect of vitamin D status on clinical pregnancy rates following in vitro fertilization. CMAJ Open (2013) 1:E77–82. doi: 10.9778/cmajo.20120032
25. Polyzos NP, Anckaert E, Guzman L, Schiettecatte J, Van Landuyt L, Camus M, et al. Vitamin D deficiency and pregnancy rates in women undergoing single embryo, blastocyst stage, transfer (SET) for IVF/ICSI. Hum Reprod. (2014) 29:2032–40. doi: 10.1093/humrep/deu156
26. Rotterdam ESHRE/ASRM-Sponsored PCOS Consensus Workshop Group. Revised 2003 consensus on diagnostic criteria and long-term health risks related to polycystic ovary syndrome (PCOS). Hum Reprod. (2004) 19:41–7. doi: 10.1093/humrep/deh098
27. Cutting R, Morroll D, Roberts SA, Pickering S, Rutherford A. Elective single embryo transfer: guidelines for practice British Fertility Society and Association of Clinical Embryologists. Hum Fertil. (2008) 11:131–46. doi: 10.1080/14647270802302629
28. ALPHA Scientists in Reproductive Medicine, ESHRE Special Interest Group Embryology. Istanbul consensus workshop on embryo assessment: proceedings of an expert meeting. Reprod Biomed Online (2011) 22:632–46. doi: 10.1016/j.rbmo.2011.02.001
29. Birkett MA, Day SJ. Internal pilot studies for estimating sample size. Stat Med. (1994) 13:2455–63. doi: 10.1002/sim.4780132309
30. Holick MF, Binkley NC, Bischoff-Ferrari HA, Gordon CM, Hanley DA, Heaney RP, et al. Evaluation, treatment, and prevention of vitamin D deficiency: an Endocrine Society clinical practice guideline. J Clin Endocrinol Metab. (2011) 96:1911–30. doi: 10.1210/jc.2011-0385
31. Irani M, Merhi Z. Role of vitamin D in ovarian physiology and its implication in reproduction: a systematic review. Fertil Steril. (2014) 102:460–8.e463. doi: 10.1016/j.fertnstert.2014.04.046
32. Sahu B, Ozturk O, Ranierri M, Serhal P. Comparison of oocyte quality and intracytoplasmic sperm injection outcome in women with isolated polycystic ovaries or polycystic ovarian syndrome. Arch Gynecol Obstet. (2008) 277:239–44. doi: 10.1007/s00404-007-0462-x
33. Barber TM, Golding SJ, Alvey C, Wass JA, Karpe F, Franks S, et al. Global adiposity rather than abnormal regional fat distribution characterizes women with polycystic ovary syndrome. J Clin Endocrinol Metab. (2008) 93:999–1004. doi: 10.1210/jc.2007-2117
34. Wood JR, Dumesic DA, Abbott DH, Strauss JF. Molecular abnormalities in oocytes from women with polycystic ovary syndrome revealed by microarray analysis. J Clin Endocrinol Metab. (2007) 92:705–13. doi: 10.1210/jc.2006-2123
35. Firouzabadi RD, Rahmani E, Rahsepar M, Firouzabadi MM. Value of follicular fluid vitamin D in predicting the pregnancy rate in an IVF program. Arch Gynecol Obstet. (2014) 289:201–6. doi: 10.1007/s00404-013-2959-9
36. Dennis NA, Houghton LA, Pankhurst MW, Harper MJ, McLennan IS. Acute supplementation with high dose vitamin D3 increases serum anti-mullerian hormone in young women. Nutrients (2017) 9:E719. doi: 10.3390/nu9070719
37. Tieu EW, Tang EK, Tuckey RC. Kinetic analysis of human CYP24A1 metabolism of vitamin D via the C24-oxidation pathway. FEBS J. (2014) 281:3280–96. doi: 10.1111/febs.12862
38. Wehmeier K, Onstead-Haas LM, Wong NC, Mooradian AD, Haas MJ. Pro-inflammatory signaling by 24,25-dihydroxyvitamin D3 in HepG2 cells. J Mol Endocrinol. (2016) 57:87–96. doi: 10.1530/JME-16-0009
39. Lensmeyer G, Poquette M, Wiebe D, Binkley N. The C-3 epimer of 25-hydroxyvitamin D is present in adult serum. J Clin Endocrinol Metab. (2012) 97:163–8. doi: 10.1210/jc.2011-0584
40. Chailurkit LO, Aekplakorn W, Srijaruskul K, Ongphiphadhanakul B. Discrepant association of serum C-3 epimer of 25-hydroxyvitamin D versus non-epimeric 25-hydroxyvitamin D with serum lipid levels. Lipids Health Dis. (2016) 15:157. doi: 10.1186/s12944-016-0333-1
41. Tripkovic L, Lambert H, Hart K, Smith CP, Bucca G, Penson S, et al. Comparison of vitamin D2 and vitamin D3 supplementation in raising serum 25-hydroxyvitamin D status: a systematic review and meta-analysis. Am J Clin Nutr. (2012) 95:1357–64. doi: 10.3945/ajcn.111.031070
42. Hammami MM, Yusuf A. Differential effects of vitamin D2 and D3 supplements on 25-hydroxyvitamin D level are dose, sex, and time dependent: a randomized controlled trial. BMC Endocrine Disorders (2017) 17:12. doi: 10.1186/s12902-017-0163-9
43. Chabas JF, Stephan D, Marqueste T, Garcia S, Lavaut MN, Nguyen C, et al. Cholecalciferol (vitamin D) improves myelination and recovery after nerve injury. PLoS ONE (2013) 8:e65034. doi: 10.1371/journal.pone.0065034
44. Aleyasin A, Hosseini MA, Mahdavi A, Safdarian L, Fallahi P, Mohajeri MR, et al. Predictive value of the level of vitamin D in follicular fluid on the outcome of assisted reproductive technology. Eur J Obstet Gynecol Reprod Biol. (2011) 159:132–7. doi: 10.1016/j.ejogrb.2011.07.006
45. Al Mheid I, Quyyumi AA. Vitamin D and cardiovascular disease: controversy unresolved. J Am Coll Cardiol. (2017) 70:89–100. doi: 10.1016/j.jacc.2017.05.031
46. Adams JS, Ramin J, Rafison B, Windon C, Windon A, Liu PT. Redefining human vitamin D sufficiency: back to the basics. Bone Res. (2013) 1:2–10. doi: 10.4248/BR201301002
47. Adams IP, Dack S, Dickinson FM, Ratledge C. The distinctiveness of ATP:citrate lyase from Aspergillus nidulans. Biochim Biophys Acta (2002) 1597:36–41. doi: 10.1016/S0167-4838(02)00276-5
48. Mahmoudi T, Gourabi H, Ashrafi M, Yazdi RS, Ezabadi Z. Calciotropic hormones, insulin resistance, and the polycystic ovary syndrome. Fertil Steril. (2010) 93:1208–14. doi: 10.1016/j.fertnstert.2008.11.031
49. Hahn S, Haselhorst U, Tan S, Quadbeck B, Schmidt M, Roesler S, et al. Low serum 25-hydroxyvitamin D concentrations are associated with insulin resistance and obesity in women with polycystic ovary syndrome. Exp Clin Endocrinol Diabetes (2006) 114:577–83. doi: 10.1055/s-2006-948308
Keywords: vitamin D, vitamin D epimers, vitamin D metabolites, fertilization rates, PCOS
Citation: Cunningham TK, Allgar V, Dargham SR, Kilpatrick E, Sathyapalan T, Maguiness S, Mokhtar Rudin HR, Abdul Ghani NM, Latiff A and Atkin SL (2019) Association of Vitamin D Metabolites With Embryo Development and Fertilization in Women With and Without PCOS Undergoing Subfertility Treatment. Front. Endocrinol. 10:13. doi: 10.3389/fendo.2019.00013
Received: 02 October 2018; Accepted: 10 January 2019;
Published: 29 January 2019.
Edited by:
Daniel David Bikle, University of California, San Francisco, United StatesReviewed by:
Carol L. Wagner, Medical University of South Carolina, United StatesMichael F. Holick, Boston Medical Center, United States
Copyright © 2019 Cunningham, Allgar, Dargham, Kilpatrick, Sathyapalan, Maguiness, Mokhtar Rudin, Abdul Ghani, Latiff and Atkin. This is an open-access article distributed under the terms of the Creative Commons Attribution License (CC BY). The use, distribution or reproduction in other forums is permitted, provided the original author(s) and the copyright owner(s) are credited and that the original publication in this journal is cited, in accordance with accepted academic practice. No use, distribution or reproduction is permitted which does not comply with these terms.
*Correspondence: Stephen L. Atkin, c2xhMjAwMkBxYXRhci1tZWQuY29ybmVsbC5lZHU=