- INSERM UMR 1195, Université Paris-Saclay, Hôpital Le Kremlin Bicêtre, Val de Marne, France
Some newly described steroid-related compounds, also found in the rest of the body, are formed and active in the central nervous system, particularly in the brain. Some are of pharmacological and physiopathological interest. We specifically report on two compounds, “MAP4343,” a new neurosteroid very efficient antidepressant, and “FKBP52,” a protein component of hetero-oligomeric steroid receptors that we found involved in cerebral function, including in Alzheimer’s disease.
Introduction
Some cholesterol found in brain and spinal cord is biologically largely independent of that found in the rest of the body (Figure 1). It gives rise to “neurosteroids” (1) and we mention in this short “Perspective” some of their pharmacological and pathophysiological properties. In particular, we report the therapeutic antidepressant activity of a new neurosteroid drug “MAP4343,” derivative of pregnenolone (PREG) (2). Very differently, we mention the cloning (3) and the function of a protein, “FKBP52,” which is a component of hetero-oligomeric steroid receptors and that, with Chambraud et al. (4), we found involved in the function of the cerebral Tau protein, including in Alzheimer’s disease. Thus, we take care of two novel and distinct active steroid-related compounds of medical interest.
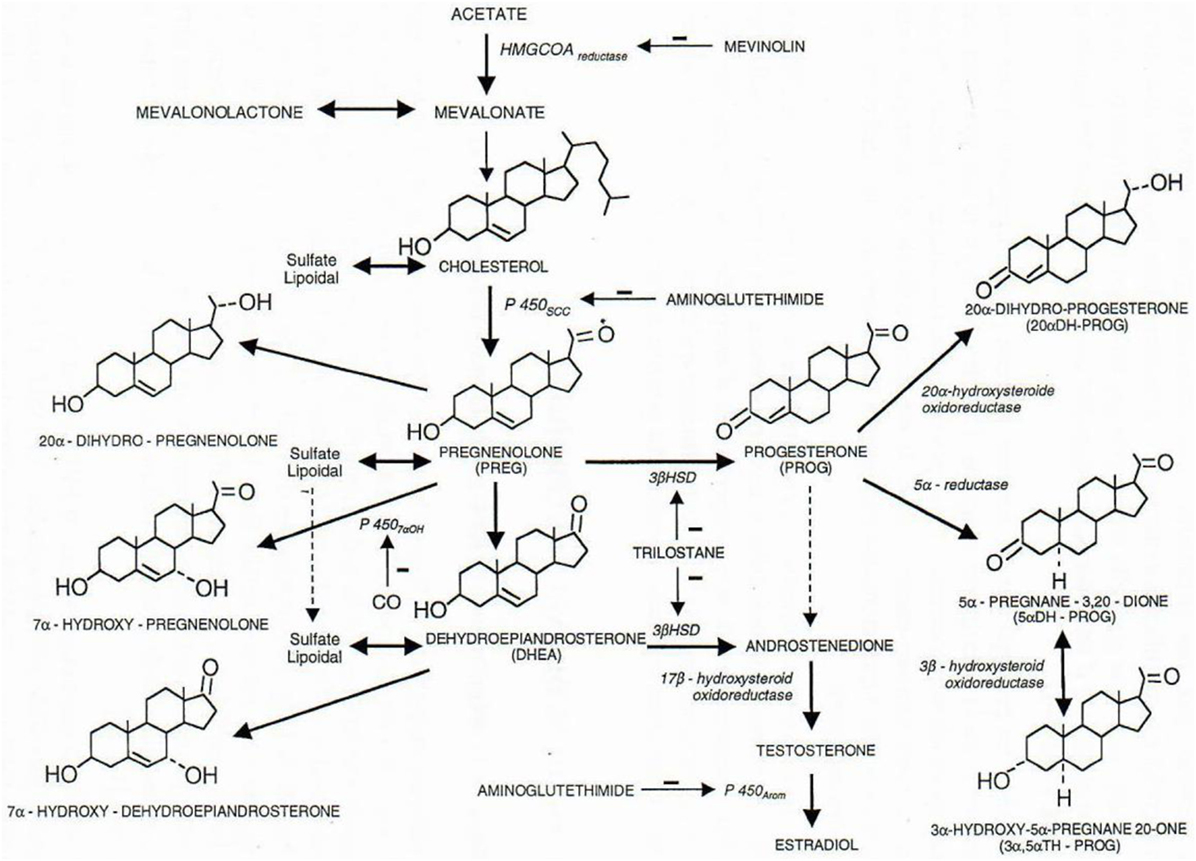
Figure 1. Some metabolic pathways of physiological steroids. All steroids are cholesterol derivatives. The P450scc cytochrome (scc for side chain cleavage) cut the 6 carbon chain of cholesterol, and pregnenolone thus synthetized is precursor of all steroid hormones (3β-HSD, 3β-hydroxysteroid dehydrogenase; 3α-HSOR, 3α-hydroxysteroid oxidoreductase; 17β-HSOR, 17β-hydroxysteroid oxidoreductase). Negative signs (−) indicate the lack of the enzymatic function corresponding to the indicated product. Figure from Baulieu (5).
First, among “neurosteroids” (1), PREG (6) is rather important quantitatively, and MAP4343, its synthetic derivative 3β-methoxy-Δ5-pregnene-20 one [Figure 2; (7)], is remarkably active to treat depressive states and addiction to ethanol (in preparation with G. Koob and O. George); it is currently studied in human beings.
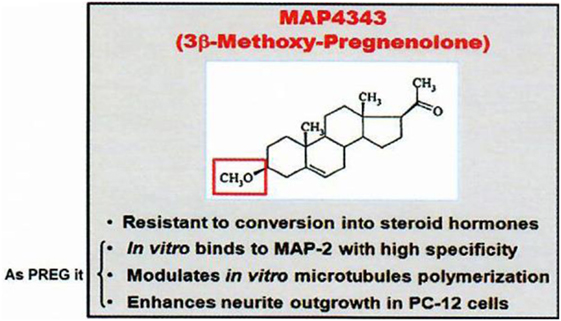
Figure 2. MAP4343, a pregnenolone derivative. Data from Baulieu (8).
Second, “FKBP52” is a protein complexed with hetero-oligomeric steroid receptors that we found to have an unforeseen interaction (4) with the Tau protein centrally involved in Alzheimer’s disease and other dementias. The profound decrease of FKBP52 in several tauopathies (9) suggests its possible therapeutic importance.
Neurosteroids: MAP4343, A Therapeutically Active Derivative of PREG
The synthesis of cholesterol in the nervous system has been described by Bloch (10). Its metabolic steroids synthetized in the central and the peripheral nervous systems (11, 12) are called neurosteroids (1, 13); their syntheses in vertebrate brains (14) occurs in neurons and glial cells, sometimes from some imported steroidal precursors. Neurosteroids include several 3β-hydroxy-Δ5 compounds, such as PREG (6, 15) and dehydroepiandrosterone (DHEA) (16–19), and their sulfate esters (20, 21). Moreover, there are Δ4-3oxo steroid hormones (progesterone is quite abundant) (22), and some of their reduced metabolites, such as one of the tetrahydroderivatives of progesterone (3αhydroxy-5αpregnan-20one, also called allopregnanolone) (23, 24). Several neurosteroids can act as modulators of neurotransmitter receptors, in particular those of GABAA (25), NMDA, and sigma-1. Frequently, 3β-hydroxy-Δ5 steroids are metabolized to Δ4-3oxo steroids in brain by 3βHSD (3β-hydroxysteroid dehydrogenase). Δ4-3oxo neurosteroids act via classical nuclear steroid receptors (26). Recent studies with progesterone indicate the possibility of distinct hormonal derivatives (27). 3β-hydroxy-Δ5 compounds themselves can also bind to the microtubule-associated protein MAP2 (28). With different locations through the brain, neurosteroids may have several concentrations and/or display diverse activities with various roles on environment and behavior (5).
The oxidative transformation of PREG to progesterone and the metabolism of progesterone to other active neurosteroids (including androgens and corticosteroids) have led us to avoid the metabolism in the brain of 3β-hydroxy-Δ5 neurosteroids to oxydated 3oxo compounds; for this reason, we synthetized and used 3β-methoxy-Δ5 steroids which are not metabolizable to Δ4-3oxo steroids of unnecessary or even pathogenically activity: for instance, an appropriate synthetic derivative of PREG is the methoxylated compound MAP4343 [Figure 2; (7)]. Interestingly, both PREG and its 3β-methoxy derivative can bind to protein MAP2 and they display the same activity on the microtubular system through their association to this protein (29). The interaction of MAP2 with PREG (natural neurosteroid) or MAP4343 (synthetic derivative) modifies the function of microtubules in target cells, stimulates their assembly, and is then responsible of positive improvement of behavior: not metabolizable to Δ4-3oxo steroids contrarily to PREG (which therefore cannot be safely administered as such). MAP4343 is very active and therefore medically convenient. Experimentally, MAP4343 increases anxiolytic and anti-depressive activities (30) in rats submitted to a specific psychosocial condition (31), in stressed tree shrews (32), and in Kyoto rats resistant to currently available antidepressants (to be submitted by Villey et al. in 2018). MAP4343 is therapeutically rapid and remarkably safe.
There are other results making 3βhydroxy-Δ5 steroids interesting to study. Their deficit in hippocampus of aged rodents may be responsible for some cognitive alteration (33); however, a similar effect has not yet been demonstrated in human beings. It is also worthwhile to analyze the possible effect of cerebral DHEA and its sulfate (34), unevenly distributed in the human brain.
Progesterone is a steroid difficult to study in the central nervous system (CNS) both qualitatively and metabolically. Progesterone is particularly involved in protection/repair after traumatisms of the CNS (35–37) and it plays a fundamental role in (re)myelination (Figures 3 and 4). After cryolesion of peripheral nerves, we have analyzed the function of progesterone synthetized by Schwann cells (38, 39).
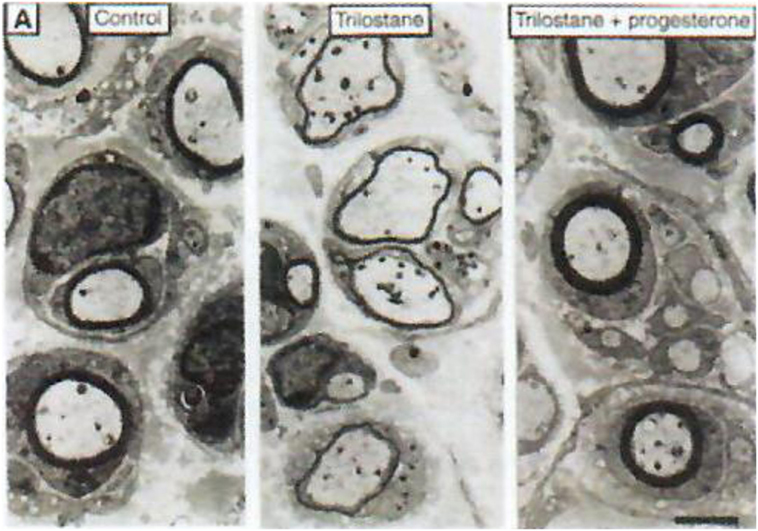
Figure 3. Role of neurosteroids in the formation of myelin sheaths. The thickness of myelin sheaths (number of lamellae) was quantified by electron microscopy of cross section of sciatic nerves from male mice 15 days after cryolesion. Effect of trilostane in the absence (center panel) or presence (right panel) of progesterone on the thickness of myelin sheaths, relative to that in control nerves (left panel). Scale bar, 2 µm. Data from Koenig et al. (38).
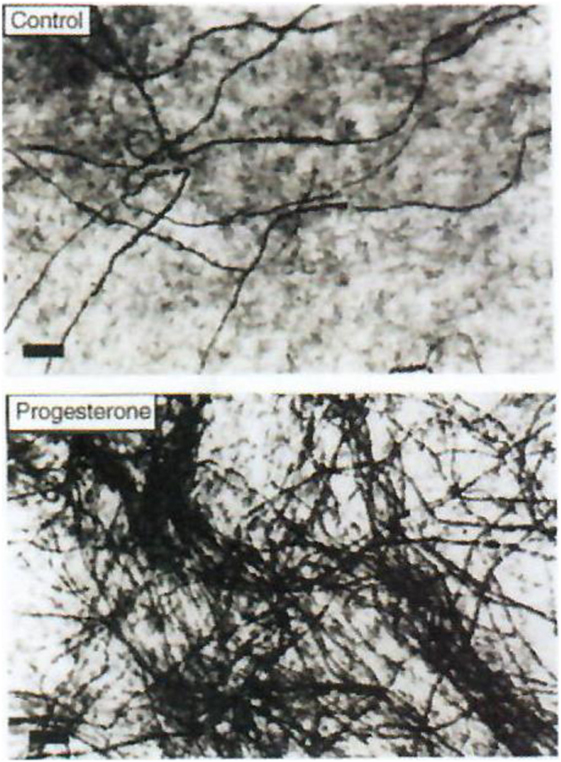
Figure 4. Effect of progesterone on myelin formation in DRG cultures. Cells were cultured for 2 weeks in myelination-promoting medium in the absence (top) or presence (bottom) of 20 nM progesterone. Myelinated fibers were stained with Sudan black and the number of myelin segments was determined. Only myelinated fibers are visible on these photographs. Scale bars, 40 µm. Data from Koenig et al. (38).
A particular transcription factor “Krox-20,” expressed in Schwann cells and stimulated by progesterone, plays an important role in myelination of regenerating sciatic nerve and sensory neurons (40, 41). The blockade of progesterone stimulation by RU486 suggests a function as for a classical progesterone receptor. There is also another membrane-associated progesterone-binding protein initially called 25Dx and currently known as PGRMC [progesterone receptor membrane compound; (42)].
Moreover, besides genomic mechanism, membrane actions of progesterone in the CNS have been described (27). Several membrane progesterone receptor(s) have been discovered (27, 43–47). It is also interesting to note that the reduced tetrahydro-metabolite of progesterone (allopregnanolone), at nanomolar concentration, can modify GABA evoked current (45). Several other neurosteroids modify functionally the activity of neuromediator receptors.
It is clear that the pharmacological function of some neurosteroids such as progesterone is diversified and this may be the reason for which it is not easy to rationalize the medical usage of post-menopausal hormone replacement (48). In addition, there are a number of synthetic progesterone analogs, multiplying the variety of compounds displaying some selective function(s) of progestins (49).
There are also a number of different neuroestrogens which, as estradiol (50), include a phenolic nucleus equivalent to the Δ4-3oxo structure of other steroid hormones and have distinct metabolic properties (51).
Δ4-3oxo neuroandrogens, the best known and active being testosterone, are partly derived from neurosteroidal 3β-hydroxy-Δ5 compounds such as DHEA, but androgenic neurosteroids synthetized in the brain have not yet been properly quantified. The androgen receptor is decisive in the spontaneous regeneration of myelin (52).
It is not yet known if, in the brain, there are neurosteroids with glucocorticosteroid properties active by themselves, or if there are, in the brain, only corticosteroids transferred from the body and participating to the quantitative regulation of their own production.
In summary, not only PREG, DHEA, as well as their sulfates, may by themselves be active in the brain, they also are potential precursors in the CNS of Δ4-3oxo steroids which are biologically active via binding to nuclear receptors. There are also, making it even more varied, well-known hormonal steroids certainly imported at least in part into the brain from glands of the body which have a function of physiologically quantitative importance on the development and the activities of neurons and myelination. They are not really “neurosteroids,” even if they are intimately linked to the function of the CNS.
Other Pharmacological Compounds With Activities Related to Neurosteroids
An Enantiomeric Form of PREG Sulfate (53–55)
This synthetic compound (56) is curiously much promnesic than PREG sulfate itself, and this effect opens a new field of research to compare activity between natural and enantiomeric structures of hormonal steroids [parenthetically, the natural PREG and PREG sulfate both are themselves active on memory; (21)].
Lithium
It has neuroprotective activity, particularly for remyelination of peripheral nerves (57). The results open perspectives in treatments by an inhibitor of glycogen synthase kinase 3β such as lithium.
Etifoxine (58, 59)
This neurostimulant of estrogens and progestins is active on experimental autoimmune encephalomyelitis, a model of multiple sclerosis (60, 61). However, attempts to decrease post-partum relapses in sick women (62) have not shown a significant success.
RU486 (Mifepristone, an Efficient Anti-Progesterone) (63)
The compound, which is also antiglucocorticosteroid, can be orally administered and has demonstrated anti-neurosteroidal effects on inappropriate neuroprogesterone and neuroglucocorticosteroid pathologies. It also permits protective effects against traumatic neuronal alterations [for example, protection of cerebellar Purkinje cells (64, 65)] and has shown therapeutically activity on some psychotic depressions and meningiomas (unpublished).
Alzheimer’s Disease: FKBP52, A Constituent of Hetero-Oligomeric Steroid Hormone Receptors, Interacts with TAU Structure and Function
In human beings, the most frequent and severe human senile dementia, the Alzheimer’s disease, currently is not biochemically explained nor treated in order to recover. However, there are several recent progresses for the diagnostic, biomarkers and imagery of the sick brain (66). Since the publication of Alois Alzheimer in 1907, two proteins remain of greatest interest (67). Up to now, the most studied has been “amyloid-β” (Aβ), essentially observed between neurons but its study has still not been successful in any therapeutic approach. The other protein, the structure and the cloning of which have been only precisely analyzed since the 1980 period, is “Tau” (68–70). Besides association to microtubules, the Tau protein is involved in several other cellular functions, including gene regulation (71). In human patients, Tau is present within neuronal cells, and the six isoforms, hyperphosphorylated (72) according to a well-defined pattern (73), are also partly truncated by caspase activity (74). Isomerization and oligomerization of Tau due to FKBP52 are independent processes (75). These modifications are largely responsible for fibrillation and aggregation, and they contribute to establish intracellular neurodegeneration in transgenic animals. It is accepted that modified Tau can be responsible for altered function of nerves, even if details of the mechanism of pathological function of abnormal Tau are not well explained.
Distinct from Alzheimer’s disease, there are dementias which are exclusively tauopathy (69), supporting the belief that Tau abnormalities are directly responsible of dementia activity of the brain in Alzheimer’s disease (72); the tauopathic diseases without Aβ abnormality could be called “pure tauopathies”: some are FTDP17: frontotemporal dementias and parkinsonism linked to chromosome 17; progressive supra-nuclear palsy; Pick diseases, etc.
Consequently, Tau, mostly in aggregated forms, is clearly involved principally in terms of neurodegeneration, and pathological Tau may be most appropriate as potential target for treating Alzheimer (76). The pure tauopathies, human and experimental in animals, are very severe diseases and matters of a large number of studies: in particular, the diseases due to P301L mutation of Tau have been very much studied as convenient models. In genetically P301L mutated zebrafishes, the Tau is much oligomerized and abnormally phosphorylated (77).
In studying steroid receptors in human beings, we had analyzed some of their hetero-oligomeric variants (78, 79). They differ according to diseases, but often include the protein FKBP52. In Alzheimer’s disease, this protein colocalizes with the autophagic-endolysosomal system (Figure 5). The FKBP structure includes an N-terminal domain (FK1) which harbors a sequence with enzymatic PPIase activity (peptidyl-cis/trans-prolyl-isomerase), thus classifying FKBP52 among the immunophilins which may bind many small molecular ligands (80, 81). The second domain of FKBP52, FK2, is architecturally similar to the FK1 but does not have a PPIase activity. The rest of the FKBP52 protein includes three TetratricoPeptid Repeats’ (TPR) sequences and binds to hsp90, which itself interacts with an hormonal steroid molecule, completing the hetero-oligomeric composition of steroid receptors. After TPR, there is a C terminal sector-binding calmodulin. It is therefore via FKBP52 that the hetero-oligomeric steroid hormones could interact functionally with a neuro-protein such as Tau.
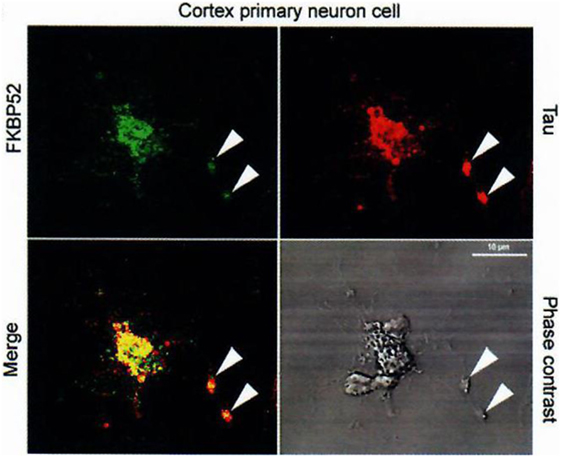
Figure 5. Colocalization of FKBP52 and Tau in primary cortical neurons and PC12 cells. Immunofluorescence staining of primary cortical neurons and PC12 cells treated with 50 nM NGF for 5 days. Double staining for Tau and FKBP52 was performed after cytosol extraction to reveal cytoskeletal association. Arrows indicate preferential colocalization of both proteins in the distal part of the nerve cell axon and at the extremity of PC12 cell neurites. Confocal images of primary cortical neurons. Analysis of 0.5-µm slices confirms the preferential colocalization in the distal part of the axon (arrowheads). Data from Chambraud et al. (4).
Indeed, we had, additionally and very importantly, discovered an interaction of FKBP52 with Tau [(4); Figures 5 and 6], completing the description of steroid–FKBP52–Tau sequence(s) never described till now, and that have not been studied in functional terms.
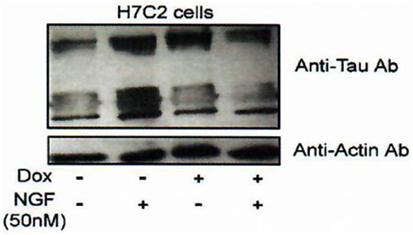
Figure 6. Effect of FKBP52 overexpression on Tau protein accumulation. H7C2 cells: stably transformed PC12 cells by an FKBP52-inducible expression system based on a tetracycline responsive element (82). NGF: 5 days—Dox: 7 days. H7C2 and PC12 cells were treated or not with NGF for 5 days in the presence or absence of DOX for 1 week; 50 µg of extracts was subjected to SDS-PAGE and immunoblotted with anti-Tau (antibody clone DC25). Actin was used as the loading control. Data from Chambraud et al. (4).
Experiments with zebrafishes under the direction of Dr. Marcel Tawk (77) utilizing transgenic animals expressing the human Tau P301L, severely suffering of abnormal escape behavior and with some change of Tau phosphorylation, indicated, in vivo and in vitro studies, the functional abnormalities due to the pathogenic mutation of Tau.
This is the basis of a strategy leading to look for a treatment of tauopathies, including Alzheimer’s disease and other dementias. FKBP52 may serve for inducing qualitatively and anti-pathological Tau activity and/or directly decreases Tau: considering that Tau is centrally involved in the pathophysiology of Alzheimer, we look for modifying the function of FKBP52 to obtain an actively anti-pathological Tau effect. There is a very profound decrease and/or a reduced function of FKBP52 that we have described in pathological circumstances: in Alzheimer’s disease, FTDP17 (9), and other tauopathies. Our hypothesis is based on the concept that the function of Tau is decisively pathological in dementias, and we hypothesize a FKBP 52 induced change quantitatively and/or qualitatively, which could be profitable to the patients (Figure 7) treated as early as possible after the beginning of the disease. In summary, appropriate modification of FKBP52 induced by some ligand(s) may help to normalize Tau activity and consequently be able to improve the cerebral function.
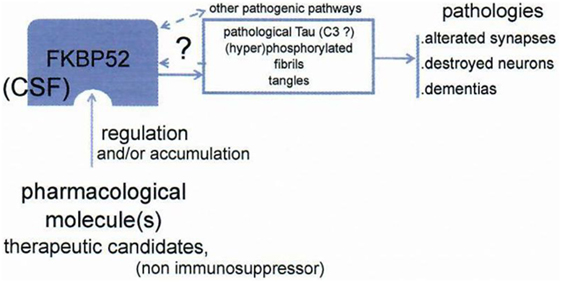
Figure 7. Schema of the working hypothesis and strategy for prevention/treatment of dementias via the novel target FKBP52. FKBP52 is measurable in CSF. Data from Baulieu (8).
It is not excluded that several brain steroids may modify the function of parameters implied in this novel approach for treating cerebral abnormality. Other approaches may help to understand alterations of the Tau protein: for instance, studies of synaptic structure (83, 84) and activity (in preparation), and experiments of deep cerebral stimulation can be involved in methods including autophagic-lysosomal protection (85).
Our therapeutic approach centered on effect of FKBP52 on Tau (dys)function may be still far to be operationally favorable to treat dementias (Figure 7). Working on Tau with new modified/liganded FKBP52 function and steroid activities, we will obtain novel results. Will they be valuable?
Summary
In this “Perspective,” we have treated two distinct examples concerning steroid associated molecules involved in activities of the CNS. They exemplify typical compounds of (1) neurosteroid derivatives and (2) components of hetero-oligomeric steroid receptors. We are happy that, in a number of other laboratories, many publications (not reported in this “Perspective”), have followed our two original studies. This paper is not a review, but we hope to draw attention to the versatile involvement of the steroidal structure and thus in important physiopathological derivatives.
Author Contributions
The author confirms being the sole contributor of this work and approved it for publication.
Conflict of Interest Statement
The author declares that the research was conducted in the absence of any commercial or financial relationships that could be construed as a potential conflict of interest.
Acknowledgments
I thank Christine Broussot-Charbonnier for her remarkable administrative assistance.
Funding
No funding for this “Perspective.” Support for the work in each cited paper to which I collaborated is detailed in the corresponding reference.
References
1. Baulieu EE. Neurosteroids: a new function in the brain. Biol Cell (1991) 71:3–10. doi:10.1016/0248-4900(91)90045-O
2. Bianchi M, Baulieu EE. 3β-Methoxy-pregnenolone (MAP4343) as an innovative therapeutic approach for depressive disorders. Proc Natl Acad Sci U S A (2012) 109:1713–8. doi:10.1073/pnas.1121485109
3. Lebeau MC, Massol N, Herrick J, Faber LE, Renoir JM, Radanyi C, et al. P59, an hsp 90-binding protein. Cloning and sequencing of its cDNA and preparation of a peptide-directed polyclonal antibody. J Biol Chem (1992) 267:4281–4.
4. Chambraud B, Sardin E, Giustiniani J, Dounane O, Schumacher M, Goedert M, et al. A role for FKBP52 in Tau protein function. Proc Natl Acad Sci U S A (2010) 107:2658–63. doi:10.1073/pnas.0914957107
5. Baulieu EE. [Neurosteroids, their role in brain physiology: neurotrophicity, memory, aging…]. Bull Acad Natl Med (2001) 185:349–69; discussion 370–2.
6. Corpéchot C, Synguelakis M, Talha S, Axelson M, Sjövall J, Vihko R, et al. Pregnenolone and its sulfate ester in the rat brain. Brain Res (1983) 270:119–25. doi:10.1016/0006-8993(83)90797-7
7. Fontaine-Lenoir V, Chambraud B, Fellous A, David S, Duchossoy Y, Baulieu EE, et al. Microtubule-associated protein 2 (MAP2) is a neurosteroid receptor. Proc Natl Acad Sci U S A (2006) 103:4711–6. doi:10.1073/pnas.0600113103
8. Baulieu EE. From steroid hormones to depressive states and senile dementias: new mechanistic, therapeutical and predictive approaches. C R Biol (2015) 338:613–6. doi:10.1016/j.crvi.2015.06.003
9. Giustiniani J, Sineus M, Sardin E, Dounane O, Panchal M, Sazdovitch V, et al. Decrease of the immunophilin FKBP52 accumulation in human brains of Alzheimer’s disease and FTDP-17. J Alzheimers Dis (2012) 29:471–83. doi:10.3233/JAD-2011-111895
10. Bloch K. The biological synthesis of cholesterol. Science (1965) 150:19–28. doi:10.1126/science.150.3692.19
11. Le Goascogne C, Robel P, Gouézou M, Sananès N, Baulieu EE, Waterman M. Neurosteroids: cytochrome P-450scc in rat brain. Science (1987) 237:1212–5. doi:10.1126/science.3306919
12. Ibanez C, Guennoun R, Liere P, Eychenne B, Pianos A, El-Etr M, et al. Developmental expression of genes involved in neurosteroidogenesis: 3β-hydroxysteroid dehydrogenase/Δ5-Δ4 isomerase in the rat brain. Endocrinology (2003) 144:2902–11. doi:10.1210/en.2002-0073
13. Baulieu EE, Robel P, Schumacher M. Neurosteroids: beginning of the story. Int Rev Neurobiol (2001) 46:1–32. doi:10.1016/S0074-7742(01)46057-0
14. Tsutsui K, Ukena K, Takase M, Kohchi C, Lea RW. Neurosteroid biosynthesis in vertebrate brains. Comp Biochem Physiol C Pharmacol Toxicol Endocrinol (1999) 124:121–9. doi:10.1016/S0742-8413(99)00065-1
15. Hu ZY, Bourreau E, Jung-Testas I, Robel P, Baulieu EE. Neurosteroids: oligodendrocyte mitochondria convert cholesterol to pregnenolone. Proc Natl Acad Sci U S A (1987) 84:8215–9. doi:10.1073/pnas.84.23.8215
16. Corpéchot C, Robel P, Lachapelle F, Baumann N, Axelson M, Sjövall J, et al. Free and sulfo-conjugated dehydroepiandrosterone in the brain of mice with myelin biosynthesis disorders. C R Seances Acad Sci III (1981) 292:231–4.
17. Corpéchot C, Robel P, Axelson M, Sjövall J, Baulieu EE. Characterization and measurement of dehydroepiandrosterone sulfate in rat brain. Proc Natl Acad Sci U S A (1981) 78:4704–7. doi:10.1073/pnas.78.8.4704
18. Majewska MD, Demirgören S, Spivak CE, London ED. The neurosteroid dehydroepiandrosterone sulfate is an allosteric antagonist of the GABAA receptor. Brain Res (1990) 526:143–6. doi:10.1016/0006-8993(90)90261-9
19. Zwain IH, Yen SS. Dehydroepiandrosterone: biosynthesis and metabolism in the brain. Endocrinology (1999) 140:880–7. doi:10.1210/endo.140.2.6528
20. Schumacher M, Liere P, Akwa Y, Rajkowski K, Griffiths W, Bodin K, et al. Pregnenolone sulfate in the brain: a controversial neurosteroid. Neurochem Int (2008) 52:522–40. doi:10.1016/j.neuint.2007.08.022
21. Ladurelle N, Eychenne B, Denton D, Blair-West J, Schumacher M, Robel P, et al. Prolonged intracerebroventricular infusion of neurosteroids affects cognitive performances in the mouse. Brain Res (2000) 858:371–9. doi:10.1016/S0006-8993(00)01953-3
22. Guennoun R, Fiddes RJ, Gouézou M, Lombès M, Baulieu EE. A key enzyme in the biosynthesis of neurosteroids, 3 beta-hydroxysteroid dehydrogenase/delta 5-delta 4-isomerase (3 beta-HSD), is expressed in rat brain. Brain Res Mol Brain Res (1995) 30:287–300. doi:10.1016/0169-328X(95)00016-L
23. El-Etr M, Akwa Y, Fiddes RJ, Robel P, Baulieu EE. A progesterone metabolite stimulates the release of gonadotropin-releasing hormone from GT1-1 hypothalamic neurons via the gamma-aminobutyric acid type A receptor. Proc Natl Acad Sci U S A (1995) 92:3769–73. doi:10.1073/pnas.92.9.3769
24. Guennoun R, Labombarda F, Gonzalez-Deniselle MC, Liere P, De Nicola AF, Schumacher M. Progesterone and allopregnanolone in the central nervous system: response to injury and implication for neuroprotection. J Steroid Biochem Mol Biol (2015) 146:48–61. doi:10.1016/j.jsbmb.2014.09.001
25. Paul SM, Purdy RH. Neuroactive steroids. FASEB J (1992) 6:2311–22. doi:10.1096/fasebj.6.6.1347506
26. Evans RM. The steroid and thyroid hormone receptor superfamily. Science (1988) 240:889–95. doi:10.1126/science.3283939
27. Schumacher M, Coirini H, Robert F, Guennoun R, El-Etr M. Genomic and membrane actions of progesterone: implications for reproductive physiology and behavior. Behav Brain Res (1999) 105:37–52. doi:10.1016/S0166-4328(99)00081-9
28. Murakami K, Fellous A, Baulieu EE, Robel P. Pregnenolone binds to microtubule-associated protein 2 and stimulates microtubule assembly. Proc Natl Acad Sci U S A (2000) 97:3579–84. doi:10.1073/pnas.97.7.3579
29. Baulieu EE, Robel P, Fellous A, Duchossoy Y, Fontaine-Lenoir V, David S. Mapreg: toward a novel approach of neuroprotection and treatment of Alzheimer’s disease. J Mol Neurosci (2004) 24:63–5. doi:10.1385/JMN:24:1:063
30. Akwa Y, Ladurelle N, Teixeira-Silva N, Sananès N, Weill-Engerer S, Baulieu EE. Neurostéroïdes: rôle dans l’anxiété et la mémoire. In: Ferreri M, editor. Anxiété, anxiolytiques et troubles cognitifs. Paris: Elsevier Masson (2004). p. 59–70.
31. Bianchi M, Fone KF, Azmi N, Heidbreder CA, Hagan JJ, Marsden CA. Isolation rearing induces recognition memory deficits accompanied by cytoskeletal alterations in rat hippocampus. Eur J Neurosci (2006) 24:2894–902. doi:10.1111/j.1460-9568.2006.05170.x
32. Parésys L, Hoffmann K, Froger N, Bianchi M, Villey I, Baulieu EE, et al. Effects of the synthetic neurosteroid: 3β-methoxypregnenolone (MAP4343) on behavioral and physiological alterations provoked by chronic psychosocial stress in tree shrews. Int J Neuropsychopharmacol (2016) 19:1–12. doi:10.1093/ijnp/pyv119
33. Vallée M, Mayo W, Darnaudéry M, Corpéchot C, Young J, Koehl M, et al. Neurosteroids: deficient cognitive performance in aged rats depends on low pregnenolone sulfate levels in the hippocampus. Proc Natl Acad Sci U S A (1997) 94:14865–70. doi:10.1073/pnas.94.26.14865
34. Weill-Engerer S, David JP, Sazdovitch V, Liere P, Schumacher M, Delacourte A, et al. In vitro metabolism of dehydroepiandrosterone (DHEA) to 7α-hydroxy-DHEA and Δ5-androstene-3β, 17β-diol in specific regions of the aging brain from Alzheimer’s and non-demented patients. Brain Res (2003) 969:117–25. doi:10.1016/S0006-8993(03)02288-1
35. Gonzalez-Deniselle MC, Lopez Costa JJ, Gonzalez SL, Labombarda F, Garay L, Guennoun R, et al. Basis of progesterone protection in spinal cord neurodegeneration. J Steroid Biochem Mol Biol (2002) 83:199–209. doi:10.1016/S0960-0760(02)00262-5
36. Labombarda F, Gonzalez SL, Lima A, Roig P, Guennoun R, Schumacher M, et al. Effects of progesterone on oligodendrocyte progenitors, oligodendrocyte transcription factors, and myelin proteins following spinal cord injury. Glia (2009) 57:884–97. doi:10.1002/glia.20814
37. De Nicola AF, Labombarda F, Gonzalez-Deniselle MC, Gonzalez SL, Garay L, Meyer M, et al. Progesterone neuroprotection in traumatic CNS injury and motoneuron degeneration. Front Neuroendocrinol (2009) 30:173–87. doi:10.1016/j.yfrne.2009.03.001
38. Koenig HL, Schumacher M, Ferzaz B, Do Thi AN, Ressouches A, Guennoun R, et al. Progesterone synthesis and myelin formation by Schwann cells. Science (1995) 268:1500–3. doi:10.1126/science.7770777
39. Robert F, Guennoun R, Désarnaud F, Do-Thi A, Benmessahel Y, Baulieu EE, et al. Synthesis of progesterone in Schwann cells: regulation by sensory neurons. Eur J Neurosci (2001) 13:916–24. doi:10.1046/j.0953-816x.2001.01463.x
40. Jung-Testas I, Schumacher M, Robel P, Baulieu EE. Demonstration of progesterone receptors in rat Schwann cells. J Steroid Biochem Mol Biol (1996) 58:77–82. doi:10.1016/0960-0760(96)00009-X
41. Guennoun R, Benmessahel Y, Delespierre B, Gouézou M, Rajkowski KM, Baulieu EE, et al. Progesterone stimulates Krox-20 gene expression in Schwann cells. Brain Res Mol Brain Res (2001) 90:75–82. doi:10.1016/S0169-328X(01)00094-8
42. Guennoun R, Meffre D, Labombarda F, Gonzalez SL, Gonzalez-Deniselle MC, Stein DG, et al. The membrane-associated progesterone-binding protein 25-Dx: expression, cellular localization and up-regulation after brain and spinal cord injuries. Brain Res Rev (2008) 57:493–505. doi:10.1016/j.brainresrev.2007.05.009
43. Zhu Y, Bond J, Thomas P. Identification, classification, and partial characterization of genes in humans and other vertebrates homologous to a fish membrane progestin receptor. Proc Natl Acad Sci U S A (2003) 100:2237–42. doi:10.1073/pnas.0436133100
44. Thomas P. Characteristics of membrane progestin receptor alpha (mPRalpha) and progesterone membrane receptor component 1 (PGMRC1) and their roles in mediating rapid progestin actions. Front Neuroendocrinol (2008) 29:292–312. doi:10.1016/j.yfrne.2008.01.001
45. Schumacher M, Mattern C, Ghoumari A, Oudinet JP, Liere P, Labombarda F, et al. Revisiting the roles of progesterone and allopregnanolone in the nervous system: resurgence of the progesterone receptors. Prog Neurobiol (2014) 113:6–39. doi:10.1016/j.pneurobio.2013.09.004
46. Schumacher M, Guennoun R, Mercier G, Désarnaud F, Lacor P, Bénavides J, et al. Progesterone synthesis and myelin formation in peripheral nerves. Brain Res Brain Res Rev (2001) 37:343–59. doi:10.1016/S0165-0173(01)00139-4
47. Schumacher M, Ghoumari A, Guennoun R, Labombarda F, Gonzalez SL, Gonzalez-Deniselle MC, et al. Progestins and antiprogestins: mechanisms of action, neuroprotection and myelination. In: Kordon C, Gaillard RC, editors. Hormones and the Brain. Berlin, Heidelberg: Springer-Verlag (2005). p. 111–54.
48. Schumacher M, Guennoun R, Ghoumari A, Massaad C, Robert F, El-Etr M, et al. Novel perspectives for progesterone in hormone replacement therapy, with special reference to the nervous system. Endocr Rev (2007) 28:387–439. doi:10.1210/er.2006-0050
49. Schumacher M, Akwa Y, Guennoun R, Robert F, Labombarda F, Desarnaud F, et al. Steroid synthesis and metabolism in the nervous system: trophic and protective effects. J Neurocytol (2000) 29:307–26. doi:10.1023/A:1007152904926
50. Naftolin F, Ryan KJ, Davies IJ, Reddy VV, Flores F, Petro Z, et al. The formation of estrogens by central neuroendocrine tissues. Recent Prog Horm Res (1975) 31:295–319.
51. Toran-Allerand CD. Minireview: a plethora of estrogen receptors in the brain: where will it end? Endocrinology (2004) 145:1069–74. doi:10.1210/en.2003-1462
52. Bielecki B, Mattern C, Ghoumari AM, Javaid S, Smietanka K, Ghanem CA, et al. Unexpected central role of the androgen receptor in the spontaneous regeneration of myelin. Proc Natl Acad Sci U S A (2016) 113:14829–34. doi:10.1073/pnas.1614826113
53. Akwa Y, Ladurelle N, Covey DF, Baulieu EE. The synthetic enantiomer of pregnenolone sulfate is very active on memory in rats and mice, even more so than its physiological neurosteroid counterpart: distinct mechanisms? Proc Natl Acad Sci U S A (2001) 98:14033–7. doi:10.1073/pnas.241503698
54. Petit GH, Tobin C, Krishnan K, Moricard Y, Covey DF, Rondi-Reig L, et al. Pregnenolone sulfate and its enantiomer: differential modulation of memory in a spatial discrimination task using forebrain NMDA receptor deficient mice. Eur Neuropsychopharmacol (2011) 21:211–5. doi:10.1016/j.euroneuro.2010.09.010
55. El Bitar F, Meunier J, Villard V, Alméras M, Krishnan K, Covey DF, et al. Neuroprotection by the synthetic neurosteroid enantiomers ent-PREGS and ent-DHEAS against Aβ25-35 peptide-induced toxicity in vitro and in vivo in mice. Psychopharmacology (2014) 231:3293–312. doi:10.1007/s00213-014-3435-3
56. Covey DF. ent-Steroids: novel tools for studies of signaling pathways. Steroids (2009) 74:577–85. doi:10.1016/j.steroids.2008.11.019
57. Makoukji J, Belle M, Meffre D, Stassart R, Grenier J, Shackleford GG, et al. Lithium enhances remyelination of peripheral nerves. Proc Natl Acad Sci U S A (2012) 109:3973–8. doi:10.1073/pnas.1121367109
58. Verleye M, Akwa Y, Liere P, Ladurelle N, Pianos A, Eychenne B, et al. The anxiolytic etifoxine activates the peripheral benzodiazepine receptor and increases the neurosteroid levels in rat brain. Pharmacol Biochem Behav (2005) 82:712–20. doi:10.1016/j.pbb.2005.11.013
59. Girard C, Liu S, Cadepond F, Adams D, Lacroix C, Verleye M, et al. Etifoxine improves peripheral nerve regeneration and functional recovery. Proc Natl Acad Sci U S A (2008) 105:20505–10. doi:10.1073/pnas.0811201106
60. El-Etr M, Vukusic S, Gignoux L, Durand-Dubief F, Achiti I, Baulieu EE, et al. Steroid hormones in multiple sclerosis. J Neurol Sci (2005) 233:49–54. doi:10.1016/j.jns.2005.03.004
61. El-Etr M, Ghoumari A, Sitruk-Ware R, Schumacher M. Hormonal influences in multiple sclerosis: new therapeutic benefits for steroids. Maturitas (2011) 68:47–51. doi:10.1016/j.maturitas.2010.09.014
62. Vukusic S, El-Etr M, Cornu C, Cucherat M, Gignoux L, Durand-Dubieg F, et al. POPART’MUS: un essai de phase III de prévention des poussées du post-partum dans la sclérose en plaques par les hormones sexuelles. Rev Neurol (2005) 161(1):1S38. doi:10.1016/S0035-3787(05)85017-9
63. Herrmann W, Wyss R, Riondel A, Philibert D, Teutsch G, Sakiz E, et al. The effects of an antiprogesterone steroid in women: interruption of the menstrual cycle and of early pregnancy. C R Seances Acad Sci III (1982) 294:933–8.
64. De Nicola AF, Gonzalez SL, Labombarda F, Gonzalez-Deniselle MC, Garay L, Guennoun R, et al. Progesterone treatment of spinal cord injury: effects on receptors, neurotrophins, and myelination. J Mol Neurosci (2006) 28:3–15. doi:10.1385/JMN:28:1:3
65. Rakotomamonjy J, Levenes C, Baulieu EE, Schumacher M, Ghoumari AM. Novel protective effect of mifepristone on detrimental GABAA receptor activity to immature Purkinje neurons. FASEB J (2011) 25:3999–4010. doi:10.1096/fj.11-183384
66. Hane FT, Robinson M, Lee BY, Bai O, Leonenko Z, Albert MS. Recent progress in Alzheimer’s disease research, part 3: diagnosis and treatment. J Alzheimers Dis (2017) 57:645–65. doi:10.3233/JAD-160882
67. Ittner LM, Götz J. Amyloid-β and tau – a toxic pas de deux in Alzheimer’s disease. Nat Rev Neurosci (2011) 12:65–72. doi:10.1038/nrn2967
68. Goedert M, Wischik CM, Crowther RA, Walker JE, Klug A. Cloning and sequencing of the cDNA encoding a core protein of the paired helical filament of Alzheimer disease: identification as the microtubule-associated protein tau. Proc Natl Acad Sci U S A (1988) 85:4051–5. doi:10.1073/pnas.85.11.4051
69. Goedert M, Spillantini MG, Jakes R, Rutherford D, Crowther RA. Multiple isoforms of human microtubule-associated protein Tau: sequences and localization in neurofibrillary tangles of Alzheimer’s disease. Neuron (1989) 3:519–26. doi:10.1016/0896-6273(89)90210-9
70. Spillantini MG, Goedert M. Tau pathology and neurodegeneration. Lancet Neurol (2013) 12:609–22. doi:10.1016/S1474-4422(13)70090-5
71. Lebouvier T, Pasquier F, Buée L. Update on tauopathies. Curr Opin Neurol (2017) 30:589–98. doi:10.1097/WCO.0000000000000502
72. Buée L, Bussière T, Buée-Scherrer V, Delacourte A, Hof PR. Tau protein isoforms, phosphorylation and role in neurodegenerative disorders. Brain Res Brain Res Rev (2000) 33:95–130. doi:10.1016/S0165-0173(00)00019-9
73. Despres C, Byrne C, Qi H, Cantrelle FX, Huvent I, Chambraud B, et al. Identification of the Tau phosphorylation pattern that drives its aggregation. Proc Natl Acad Sci U S A (2017) 114:9080–5. doi:10.1073/pnas.1708448114
74. Rissman RA, Poon WW, Blurton-Jones M, Oddo S, Torp R, Vitek MP, et al. Caspase-cleavage of Tau is an early event in Alzheimer disease tangle pathology. J Clin Invest (2004) 114:121–30. doi:10.1172/JCI200420640
75. Kamah A, Cantrelle FX, Huvent I, Giustiniani J, Guillemeau K, Byrne C, et al. Isomerization and oligomerization of truncated and mutated Tau forms by FKBP52 are independent processes. J Mol Biol (2016) 428:1080–90. doi:10.1016/j.jmb.2016.02.015
76. Li C, Götz J. Tau-based therapies in neurodegeneration: opportunities and challenges. Nat Rev Drug Discov (2017) 16:863–83. doi:10.1038/nrd.2017.155
77. Giustiniani J, Chambraud B, Sardin E, Dounane O, Guillemeau K, Nakatani H, et al. Immunophilin FKBP52 induces Tau-P301L filamentous assembly in vitro and modulates its activity in a model of tauopathy. Proc Natl Acad Sci U S A (2014) 111:4584–9. doi:10.1073/pnas.1402645111
78. Baulieu EE, Binart N, Cadepond F, Catelli MG, Chambraud B, Garnier J, et al. Do receptor-associated nuclear proteins explain earliest steps of steroid hormone function? In: Carlstedt-Duke J, Eriksson H, Gustafsson JA, editors. The Steroid/Thyroid Hormone Receptor Family and Gene Regulation. Proceedings of the 2nd International CBT Symposium; 1988 Nov 4-5. Stockholm, Sweden (1989). p. 301–18.
79. Schiene-Fischer C, Yu C. Receptor accessory folding helper enzymes: the functional role of peptidyl prolyl cis/trans isomerases. FEBS Lett (2001) 495:1–6. doi:10.1016/S0014-5793(01)02326-2
80. Steiner JP, Hamilton GS, Ross DT, Valentine HL, Guo H, Connolly MA, et al. Neurotrophic immunophilin ligands stimulate structural and functional recovery in neurodegenerative animal models. Proc Natl Acad Sci U S A (1997) 94:2019–24. doi:10.1073/pnas.94.5.2019
81. Blackburn EA, Walkinshaw MD. Targeting FKBP isoforms with small-molecule ligands. Curr Opin Pharmacol (2011) 11:365–71. doi:10.1016/j.coph.2011.04.007
82. Gossen M, Bujard H. Tight control of gene expression in mammalian cells by tetracycline-responsive promoters. Proc Natl Acad Sci U S A (1992) 89:5547–51. doi:10.1073/pnas.89.12.5547
83. Doudna JA, Charpentier E. Genome editing. The new frontier of genome engineering with CRISPR-Cas9. Science (2014) 346:1258096. doi:10.1126/science.1258096
84. Incontro S, Asensio CS, Edwards RH, Nicoll RA. Efficient, complete deletion of synaptic proteins using CRISPR. Neuron (2014) 83:1051–7. doi:10.1016/j.neuron.2014.07.043
Keywords: brain steroids, neurosteroids, Alzheimer’s disease, tauopathies, pregnenolone, MAP4343, FKBP52, RU486
Citation: Baulieu E-E (2018) Steroids and Brain, a Rising Bio-Medical Domain: a Perspective. Front. Endocrinol. 9:316. doi: 10.3389/fendo.2018.00316
Received: 26 December 2017; Accepted: 25 May 2018;
Published: 15 June 2018
Edited by:
Takayoshi Ubuka, Monash University Malaysia, MalaysiaReviewed by:
Gianfranco Spalletta, Fondazione Santa Lucia (IRCCS), ItalyVassilios Papadopoulos, University of Southern California, United States
Copyright: © 2018 Baulieu. This is an open-access article distributed under the terms of the Creative Commons Attribution License (CC BY). The use, distribution or reproduction in other forums is permitted, provided the original author(s) and the copyright owner are credited and that the original publication in this journal is cited, in accordance with accepted academic practice. No use, distribution or reproduction is permitted which does not comply with these terms.
*Correspondence: Etienne-Emile Baulieu, etienne.baulieu@inserm.fr