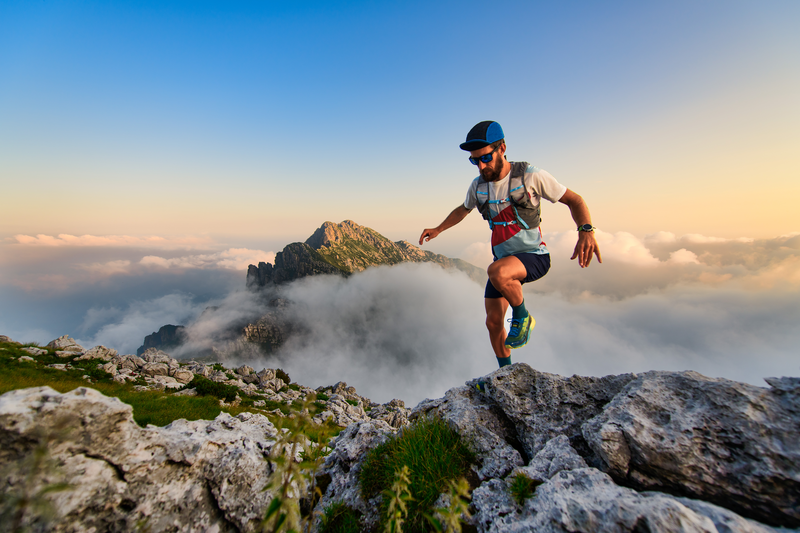
95% of researchers rate our articles as excellent or good
Learn more about the work of our research integrity team to safeguard the quality of each article we publish.
Find out more
ORIGINAL RESEARCH article
Front. Educ. , 23 October 2024
Sec. STEM Education
Volume 9 - 2024 | https://doi.org/10.3389/feduc.2024.1460496
The fast-paced and intricate nature of the current society, coupled with the ongoing changes in educational requirements, calls for the cultivation of higher-order thinking skills. To that end, adopting metacognitive strategies could be instrumental. This paper aimed to evaluate the effects of a 10-week-long metacognition-based biology course on school students’ metacognition and biology comprehension. Employing a mixed-methods quasi-experimental design, the study involved 120 eighth-graders from two public schools. One group was exposed to metacognitive questioning, while the other one continued with regular classroom activities. The results showed that participants who received metacognition-guided instruction achieved higher scores on the biology test and reported improved metacognition as compared to those who followed the standard curriculum. These outcomes were consistent across genders and regardless of whether a student reported a deep or surface learning approach. Qualitative analysis extracted themes of collaboration and understanding among the students. They appreciated the specificity of tasks, which deepened their comprehension, and valued collaborative learning facilitated by metacognitive prompts. These prompts not only enhanced their understanding but also contributed to fostering a positive classroom environment. This research underscores the effectiveness of metacognitive support in enhancing biology education. It advocates for the integration of metacognitive strategies into classroom practices, emphasizing the positive impact they can have on students’ learning experiences and outcomes.
While advancing students’ cognitive capacities is crucial, it alone may not be sufficient to address the needs of our rapidly changing and complex society, including the evolving demands of the educational process (Bettayeb et al., 2024; Chimbunde and Moreeng, 2024; Le and Nguyen, 2024). Moving beyond memorization toward solution-oriented thinking, pattern recognition, and forming conjectures can afford a stronger grasp of the subject (Hidayat and Ying, 2023). Hence, fostering higher-order thinking skills has emerged as a crucial educational objective in the 21st century (Zhan et al., 2023; Zheldibayeva, 2023). One key component of higher-order thinking is metacognition, encompassing the reflective and self-aware dimensions of cognition vital for effective problem-solving (Sutarto et al., 2022). Metacognition, as conceptualized by John Flavell, the pioneer of the term, refers to the cognitive process of introspectively examining one’s own thoughts (Flavell, 1979). In essence, it encompasses comprehension and regulation of one’s own knowledge, cognitive processes, and learning strategies (Ain et al., 2023). Metacognitive skills include planning, monitoring, evaluating, and revising one’s own learning strategies and outcomes (Roorda et al., 2024; Teng and Yue, 2023). Metacognition operates on meta-levels and mental processes, guiding thinking in ways that can forecast the success of practical problem-solving endeavors (Ramlah et al., 2023). Students equipped with well-honed metacognitive abilities can pinpoint unfamiliar concepts and employ suitable learning strategies to grasp these concepts effectively (Biwer et al., 2023). Metacognition has been recognized as an essential skill for effective learning and problem solving (Bowden and Purper, 2024; Brausch-Böger and Förster, 2024; Jaeger and Fiorella, 2024; Shin et al., 2024).
However, metacognition is not a natural or automatic process for many students. It requires explicit instruction, practice, and feedback to develop and improve. Moreover, metacognition is not a generic skill that can be applied to any situation, it is context-specific and depends on the nature and complexity of the task at hand (Stebner et al., 2022). Consequently, metacognition needs to be tailored to the specific goals and imperatives of different learning environments and tasks. One such domain that poses high cognitive and self-regulatory demands on students is school biology. Biology is a complex and dynamic science that involves multiple levels of organization, from molecules to ecosystems, and multiple perspectives, from molecular to evolutionary. Biology education requires not only the acquisition of vast factual knowledge but also the development of critical thinking skills to understand intricate biological concepts (Tibell and Rundgren, 2010). The diverse range of topics, spanning from cellular processes to ecological systems, often overwhelms students, making it essential to employ effective learning strategies. Metacognitive skills training seems like a promising avenue to help students navigate this complexity by providing them with tools to monitor their understanding, regulate their learning processes, and adapt their strategies to different biological contexts. Biology, as a scientific discipline, requires students to engage in problem-solving tasks that involve analyzing experimental data, interpreting complex phenomena, and constructing coherent explanations (Lucas et al., 2022). Metacognitive skills, such as evaluating the relevance of information and assessing the validity of scientific arguments, are pivotal in such activities. Therefore, there is a need for effective instructional strategies that can support students’ metacognition when learning biology. Here we suppose that metacognitive guidance can enhance eighth grade students’ metacognition and biology comprehension, and the effectiveness of a metacognition-focused intervention can be influenced by individual differences in students’ learning approaches. By implementing metacognitive practices, students could develop a deeper understanding of biological concepts and refine their problem-solving abilities. The premises for this assumption can be extrapolated from earlier research, although it informs us on discordant findings. For instance, a non-controlled study (Antonio and Prudente, 2021) describes how a metacognitive argument-driven inquiry approach was integrated into university biology lessons over 4 weeks. It is reported that the intervention significantly aggrandized students’ argumentation skills and understanding of biology concepts, such as protein synthesis and antimicrobial resistance. Nonetheless, in a recent study (Zeitlhofer et al., 2023), metacognitive prompts were embodied into school geography learning. The authors claimed that this scaffold did not yield a significant difference in post-interventional knowledge scores between learners in metacognition-guided and non-treated conditions. Teng (2022) revealed that incorporating metacognitive prompts into cooperative learning for 18 weeks did not cause significant changes in English as a foreign language learners’ metacognitive awareness. However, participants who received metacognitive instruction significantly outperformed those in comparison conditions in terms of academic writing skills.
Despite the copious body of research on metacognitive support in educational contexts, there is an extremely meager and incongruous empirical research evidence regarding the effects of metacognitive interventions on school students’ metacognition and biology learning. The present study aims to fill this gap by investigating the effects of a metacognitive intervention on eighth grade students’ biology comprehension and metacognitive capacity. The research questions were formulated as follows:
RQ1. How does metacognitive questioning influence students’ biology comprehension?
RQ2. How does metacognitive questioning influence students’ metacognition?
RQ3. Does learning approach play a role in the effect of metacognitive questioning on students’ metacognition?
RQ4. How do students perceive the impact of metacognitive questioning on their learning experiences and metacognition in the context of the biology course?
Flavell’s Metacognitive Theory posits that metacognition is not a uniform ability but a multifaceted construct comprising metacognitive knowledge and metacognitive regulation (Flavell, 1979). Metacognitive knowledge includes awareness of cognitive processes, strategies, and task characteristics, while metacognitive regulation involves planning, monitoring, and evaluating cognitive activities (Cherrier et al., 2020).
Vygotsky’s Socio-Cultural Theory contends that cognitive development is intricately linked with social interaction and cultural tools (Vygotsky, 1978). Within this framework, metacognitive learning can be viewed as a social process where students learn not only from individual reflection but also from collaborative discussions and guidance from peers and educators. Metacognitive strategies, such as reflective questioning and self-explanation, serve as cultural tools that facilitate the internalization of metacognitive knowledge and regulation within the individual’s cognitive repertoire. Moreover, Vygotsky introduced the concept of the Zone of Proximal Development (ZPD), denoting the range of tasks a learner can perform with assistance but cannot accomplish alone (see Glassman et al., 2023, for a review on ZPD). Metacognitive interventions provide the necessary scaffolding to guide students within their ZPD, enabling them to develop metacognitive skills beyond their current abilities. Through collaborative metacognitive activities, students receive the support needed to enhance their metacognitive regulation and knowledge.
By integrating Flavell’s Metacognitive Theory and Vygotsky’s Socio-Cultural Theory, this study hypothesizes that metacognitive learning strategies, grounded in collaborative and reflective practices, can significantly elevate eighth graders’ metacognition. These theoretical frameworks provide a foundation for understanding metacognition not as a static trait but as a malleable skill that can be nurtured and enhanced through appropriate educational interventions. Through the lens of these theories, this study explores the transformative potential of metacognitive learning in shaping the metacognitive abilities of eighth-grade students.
The learning approach, broadly categorized as either deep or surface learning, represents a fundamental aspect of students’ learning behaviors and cognitive engagement (Saqr et al., 2023). Deep learners tend to engage with the learning material in a meaningful way, focusing on understanding and connecting concepts, whereas surface learners adopt a more superficial approach, concentrating on memorization and reproducing information without a deep understanding (Marton and Saljo, 1976). Cognitive Load Theory (CLT; Sweller, 1988) and Social Cognitive Theory (SCT; Bandura, 1986) offer theoretical perspectives on the hypothesis that learning approach moderates the effects of metacognitive learning on biology comprehension and metacognition.
CLT posits that working memory capacity is limited, and that learning is most effective when working memory resources are not overloaded. In the context of learning approaches, it can be speculated that when integrating metacognitive strategies into a biology curriculum, students adopting a deep learning approach are more likely to effectively manage their cognitive load than surface learners since metacognitive techniques, such as self-monitoring and planning, help deep learners to organize their cognitive resources efficiently. In contrast, surface learners might struggle to apply metacognitive strategies effectively, ending up having increased cognitive load. Thence, the interplay between learning approach and metacognition, in the context of CLT, suggests that deep learners are better positioned to optimize their cognitive resources, accelerating biology comprehension and metacognition.
SCT views people as active agents who both influence and are influenced by their environment. A major component of SCT is so-called observational learning, i.e., the process of learning desirable and undesirable behaviors by observing others, then reproducing the behaviors in order to maximize rewards (see, for example, Silver et al., 2021). Relatedly, it can be conjectured that, as opposed to surface learners, deep learners are more likely to observe and imitate their teachers and peers utilizing metacognitive strategies as they are more motivated to understand the meaning of the matter they are pursuing.
Incorporating CLT and SCT into the hypothesis framework illuminates the moderating role of learning approaches. Deep learning strategies, aligned with meaningful understanding and effective management of cognitive load, may magnify both biology comprehension and metacognitive development. Surface learning approach, on the other hand, may present challenges in applying metacognitive techniques, potentially impeding both outcomes.
The present research adopts a mixed-methods quasi-experimental design. Quasi-experimental designs involve manipulating an independent variable without random assignment. In this study, the metacognitive questioning intervention served as the independent variable. The study incorporates both quantitative and qualitative methods, with the triangulation design allowing the researchers to collect and compare data from both methods concurrently to corroborate the findings.
The study involved four classes of eighth-grade students (n = 120; 13-15-year-olds; 67 females, 53 males) from two Nazarbayev Intellectual Schools located in different Kazakhstani cities. Within each school, one class served as the experimental group, while the other class was the control group. The experimental group (with 26 students in one school and 32 students in another) participated in a metacognitive questioning approach, while the control group (with 29 students in one school and 33 students in another) followed a conventional classroom approach. Participant recruitment took place between September and December 2023. Prior to the study, the researchers received ethical approval from the Institutional Review Board of the Alikhan Bokeikhan University (approval no. ABU-197/04). Digital informed consent was obtained from students and their legal guardians. Throughout the study, confidentiality, participant well-being, and respect for participants’ rights were diligently upheld. To safeguard participants’ privacy, all collected data, including questionnaires and interview recordings, were anonymized.
Two authors of this paper, who hold teaching positions at Nazarbayev Intellectual Schools, directly implemented the metacognitive intervention. Both teachers completed an online training module on how to apply the metacognitive approach to pedagogical practice offered by the Alikhan Bokeikhan University in November 2023. In December 2023, these teachers held three Zoom meetings to reach an agreement on teaching materials, prompts, and tasks.
Both the control and experimental groups adhered to an identical biology curriculum aligned with the guidelines set forth by the Kazakhstani State Agency for Standardization. This curriculum involved the use of the same biology textbook (Korotkova et al., 2019) and encompassed 2 hours of weekly instruction delivered by the same experienced teacher. Specifically, three consecutive lessons were merged into a single session usually covering two topics, with teacher-led collaborative discussions among students, often in pairs or groups. However, students in the experimental group received additional pedagogical support in the form of metacognitive prompts adapted from the IMPROVE method. These prompts were conceived as scaffolding to help learners engage with concepts, enhance problem-solving skills, find connections between problems, and contemplate their cognitive processes (Kramarski and Zoldan, 2008; Mevarech and Kramarski, 1997).
The intervention comprised several key steps upon each biology topic:
1. Teacher’s introduction of the topic to the entire class.
2. Allocating students into small groups.
3. Metacognitive questioning within these groups. Each mini-group was equipped with metacognitive prompt cards tailored to the specific topic so that students were encouraged to ask and answer four types of metacognitive questions (a) comprehension questions, designed to prompt students to grasp and articulate the principal ideas embedded within the presented problems; (b) strategic questions, aimed at fostering problem-solving capability by prompting students to select suitable principles or strategies, substantiate their choices, and elucidate their application to problem resolution; (c) connection questions, which were anticipated to guide students in identifying parallels and distinctions between the current problem and previously encountered problems; (d) reflection questions, compelling students to reflect on their problem-solving approaches and rationales.
For example, the biology textbook presented the following task:
“Today horse’s ancient ancestors were evolving toward an adaptation to rapid locomotion on hard ground and to feeding on tough steppe vegetation. List the new traits the horse acquired as it evolved.”
In the experimental condition, this task was complemented by the following metacognitive questions:
“What is the main idea or concept that this problem is addressing?” (Comprehension question).
“Think about other instances of evolution you have learned about. How does the process of evolution in horses compare to the evolution of another species you have studied?” (Connection question).
“How can you go about creating a list of the new traits the horse acquired during its evolution?” (Strategic question).
“How might understanding the adaptations of ancient horses help us understand the world around us today?” (Reflection question).
The iterative process involved students taking turns to resolve problems while responding to the questions posed on the cards. During these metacognitive questioning activities, learners sought to reach a consensus within their groups by resorting to discussions guided by the metacognitive prompts. Students were permitted to access their gadgets to search for information related to the assignment. Metacognitive guidance was embedded into the biology lessons’ content for 10 weeks, commencing on January 9, 2024, with the initial introduction of students to the concept of metacognition and the benefits of metacognitive learning activities. The intervention concluded on March 12, 2024, with the final metacognition-driven lesson. A total of 19 biological topics spanning four units (respiration and excretion, regulation of body functions, reproduction, and selection and evolution) were explored throughout the metacognitive learning period.
The pre-test and post-test data collection took place 3 days before the start of the intervention and 3 days after the course ended. To address RQ1, researchers collaboratively composed a biology test encompassing the four specified topics nonconsecutively distributed among 38 multiple-choice questions (with a maximum achievable score of 38 points), each tasking students with selecting the correct option from among distractors. The total score was calculated by adding up all the correct responses. The test items remained consistent between the pre-test and post-test, except that the pre-test allowed respondents to choose the option “I do not know” to minimize guessing. An exemplar question from the test is provided below:
“What is the primary mechanism by which glucose enters a cell?
A. Active transport facilitated by carrier proteins.
B. Endocytosis engulfing glucose molecules.
C. Passive diffusion through the cell membrane.
D. Facilitation of glucose uptake by cell surface receptors.”
The validity of the biology test was reviewed by two independent subject matter experts who evaluated each item for its alignment with the curriculum and its ability to measure students’ comprehension of the topics (Cohen’s kappa of 0.83).
To answer RQ2, the Metacognitive Awareness Inventory (MAI; Schraw and Dennison, 1994) as adapted for 6–9 graders (Sperling et al., 2002) was completed by the participants prior to the intervention and upon its completion. Each of the 18 items (e.g., “I think about what I need to learn before I start working”) was scored on a five-point Likert type scale ranging from “Never” to “Always.” Higher scores indicated more effective metacognitive processing. In our research, the even-odd split-half reliability of the MAI was 0.77 pre-test and 0.81 post-test.
Additionally, participants’ gender and type of learning approach the student uses (deep approach or surface approach) were collected in the pre-intervention survey only. Knowing students’ learning approach was required to address RQ3 and was evaluated using the Revised Two-factor Learning Process Questionnaire (R-LPQ-2F; Chow and Chapman, 2018) comprising 22 statements (e.g., “I like constructing theories to fit odd things together”) estimating surface and deep approach dimensions based on a 5-point Likert-scale from 1 (“never or only rarely true of me”) to 5 (“always or almost always true of me”). The score for each factor (11 items each) per an individual was derived by summing the scores of all 11 items. In this study, the even-odd split-half reliability of the R-LPQ-2F was 0.86 pre-test and 0.90 post-test.
The R-LPQ-2F and MAI were translated from English to Russian by an accredited translator. A second translator performed back-translation to validate the accuracy of the translations. To assess the clarity and answerability of the items, the translated tests and inventories were administered to four eighth-grade students who were not part of the participant pool. This pilot testing revealed that the items were clear and did not require any adjustments.
The instruments were filled out in a paper-pencil format in the classroom, with all forms and responses in Russian. To maintain anonymity, questionnaires were distributed and collected in sealed envelopes without any identifying information on the response sheets. The internal consistency of all scales was confirmed with random split-half values above 0.70.
To answer RQ4, in-depth semi-structured interviews were conducted by two researchers who were not participants’ teachers (one interviewer per school) within the school premises. The interview protocol consisted of open-ended questions, such as “Can you describe your experience with metacognitive questioning in the biology class?” A subset of ten students was selected for interviews, with five randomly chosen participants from each experimental group who had consented to be interviewed. These interviews were audio-recorded, coded, and transcribed for subsequent analysis.
Descriptive statistics (means and standard deviations) were utilized to explore the dataset, providing an overview of the data. Following this, inferential methods were applied. Post-intervention group comparisons were made using analysis of covariance (ANCOVA), controlling for potential covariates, namely pre-existing scores, learning approaches, and gender. Levene’s test was insignificant for both response variables. Upon inspecting the quantile-quantile plot for the data, no violation of normality was detected.
As an additional layer of the intervention effectiveness examination, a two-tailed t-test of independent samples was included in the analysis. The threshold for statistical significance was set at p < 0.05 for all criterions. Both inferential statistics were executed using the R programming language.
To explore if the effect of the metacognitive intervention on learners’ post-test MAI scores differed based on their learning approach, moderation analysis was performed using the PROCESS (Perosanz and Hayes, 2021). In this analysis, deep learning approach and surface learning approach were modeled as moderators, whereas gender and pre-test metacognition levels were inputted as covariates. The R2 criterion was used to deduce the extent to which the statistical model accounted for the variance in the outcome variable. Qualitative data obtained from interviews were subjected to thematic analysis. This method involved identifying key themes related to students’ perceptions of the metacognitive questioning intervention.
RQ1. “How does metacognitive questioning influence students’ biology comprehension?”
Descriptive statistics for the data are reported in Table 1. To target RQ1, ANCOVA was conducted, with metacognitive questioning as an independent variable and post-intervention biology test scores as an outcome variable, while controlling for pre-intervention biology test scores, deep learning approach scores, surface learning approach scores, and gender (Table 2).
Resultantly, only the effect of metacognitive questioning was significant (F (1, 114) = 20.67, p < 0.001). The significant between-group difference revealed by t-test (t = 4.60, p < 0.001; Figure 1) supported the ANCOVA results.
RQ2. “How does metacognitive questioning influence students’ metacognition?”
ANCOVA indicated a significant impact of the metacognitive intervention on post-intervention metacognition scores (F (1, 114) = 28.61, p < 0.001; Table 3). Accounting for pre-intervention metacognition scores, deep learning approach scores, surface learning approach scores, and gender, the metacognitive learning was associated with heightened metacognitive skills among students. The pre-test metacognition was significant as well, but again, the baseline between-group difference was too sparse to draw any conclusions. The independent sample t-test found that the difference between post-intervention metacognition in control and experimental groups was significant (t = 2.50, p = 0.013; Figure 2).
RQ3. “Does learning approach play a role in the effect of metacognitive questioning on students’ metacognition?”
The moderation analysis showed insignificant regression coefficients of both learning approaches adopted and interactions between metacognitive questioning and learning approaches on metacognition outcome (Figure 3). The statistical model explained 73% of the variance.
Figure 3. Moderation analysis structural model. Gender and pre-test metacognition are covariates. Values represent unstandardized regression coefficients.
RQ4. “How do students perceive the impact of metacognitive questioning on their learning experiences and metacognition in the context of the biology course?”
Two key themes emerged from the interview responses: collaboration and understanding. Under the theme of understanding, students discussed how the course helped them grasp and articulate the principal ideas embedded within the presented problems. Particularly, respondent H said: “We have a program that already assumes in-depth exploration, but this course was a bit different. I would say that the focus in this course is more on broadening the mind. For example, when we were going through the topic of selection, in the textbook there was an assignment to discuss with classmates the pros and cons of artificial selection. Honestly, I do not know how we would go about it. Meanwhile, in the card from the teacher for our group, it was more specific: to discuss the potential impact of GMOs on small-scale farms and the diversity of plant crops. Well, things are easier when concretized. We started looking for information on GMOs, someone even found excerpts from Panchin’s book. Frankly speaking, I have not really taken on board whether GMOs are more harmful or beneficial, where the myths are and where the truth is, but anyway, it was substantive and informative, and when you see the polyphony of data, you realize that you have to be careful how you perceive them and not immediately adopt the opinions you encountered.” Respondent A, in turn, captioned the focus of the experimental learning on building a bigger picture of biology: “I liked the fact that there was a task to work out how the release of cortisol due to late sleep patterns affects the functioning of the human heart, bringing us back to the circulatory system topic, which we had learned about prior to this course. It feels more exciting when you see those linkages, like you get a more meaningful learning experience.”
The theme of collaboration concerns how the students were able to join forces and learn from each other. The participants reported that working in small groups with the metacognitive prompts was comfortable and helpful. Respondent E recalled: “At first I had a stupor, since the program already included tons of information, so there seemed to be even more coming for some reason, but then I watched how one mini-group performed, I saw that the format was rather laid-back in fact, and it did not provoke me to slack off, but contrary, I wanted to juxtapose facts on my topic like they did, to make a similar chart, and to have a dispute. Ultimately, the growing amount of information was not exhausting.” Respondent J noted another point about working together on this course: “I remember the group was not always happy with the prompts: sometimes we wanted to modify the question or the problem, but we still tried to work around it. Perhaps it affected the results, as 1 day the teacher reminded us that the prompt is just a rough path, not something we have to stick to strictly. After that it became mentally easier, and afterwards it even became a joy to dive into the topics together.” Respondent C mentioned a psychological facet of the experience: “When it comes to homework, everyone is sort of autonomous. But here everyone strives to contribute to the collective effort, and I think it really transforms the classroom climate.” Respondent F also stated: “There were more opportunities to see the way one behaves when working in a team, which was another valuable life experience.”
The objective of this investigation was to explore the impact of a metacognition-based biology course on eighth-grade students’ comprehension of biology concepts and their metacognitive skills. The findings indicate that learners who underwent the metacognitive intervention exhibited a superior understanding of complex biological concepts as well as better metacognitive processing compared to the non-treatment group. This evidence backs the importance of metacognitive interventions in fostering students’ metacognitive development. By encouraging students to think about their thinking processes, metacognitive activities enable them to monitor their understanding, evaluate their problem-solving approaches, adjust their strategies as needed, and eventually attain better learning performance (Halmo et al., 2024; Nilimaa, 2023; Suliani et al., 2024).
The absence of a significant interaction between learning approaches and the effect of metacognitive prompts on self-reported metacognition suggests that the course was helpful across different learning styles. Whether students adopted a deep or surface approach to learning, they could have equitable learning outcomes. Such inclusivity is crucial in diverse classrooms where students may have different slant on the knowledge acquisition.
The qualitative data revealed two key themes: collaboration and understanding. Collaborative learning experiences facilitated by metacognitive questioning were perceived positively by students. Working in small groups and engaging in discussions guided by metacognitive prompts engendered a supportive learning environment where students could learn from one another. Not only did this collaborative manner enhance their understanding of biology concepts but it also contributed to a positive classroom climate. Additionally, students appreciated the depth of understanding achieved through metacognitive guidance. The prompts encouraged them to explore topics in detail, make connections between different concepts, and critically evaluate information, leading to a more profound comprehension of the subject matter.
The research on how teaching students to think about their own thinking affects their learning of biology is scant. The few relevant publications available at the time of this writing show mixed results. Our findings are consonant with the study by Zion and Cohen (2021) in which fifth and sixth graders participated in a nutritional literacy learning unit about the importance of drinking enough water and the dangers of drinking too much sugar-sweetened beverages. In addition, the intervention group received drinking-related metacognitive guidance, instructing them on how to use metacognition to stay hydrated. At the end of the research, significant improvement in drinking-related metacognitive awareness, including goal setting and planning strategies, was observed in the trial group relative to non-metacognition counterparts. Another paper (Recede et al., 2020) examined the effects of metacognitive analogy instruction on secondary students’ reflective thinking skills in biology. Reportedly, there were no significant differences between the metacognitive and non-metacognitive groups in terms of performance on the understanding, reflection, and critical reflection dimensions of reflective thinking. Additionally, Wang (2015) explored the impact of scaffolds, specifically cognitive prompts and metacognitive evaluation, on seventh-grade students’ content knowledge and construction of scientific explanations through inquiry-based biology activities. Ultimately, metacognitive evaluation did not significantly affect biology content post-test scores. On the other hand, the metacognitive support improved all scientific explanation components across five biology units, particularly claims and reasoning. These research findings evince that metacognitive guidance can have inconsistent effects on various facets of biology learning.
Readers should remain cognizant of some limitations of this study when interpreting the findings. One limitation is the duration of the experiment. Although the period of 10 weeks allowed for a comprehensive exploration of the intervention effects, a more extended study duration might enlighten academia about the long-term impact of metacognitive support on students’ learning performance and metacognition level. Additionally, the research context was limited to eighth-grade students in specific educational settings. Generalizing the findings to different grade levels or educational contexts might require further research. Addressing these limitations in future research endeavors can contribute to a more nuanced understanding of metacognitive strategies in educational contexts and their implications for diverse student populations.
Overall, the present study contributes to the body of research by providing further evidence of the potential benefits of incorporating metacognitive strategies into biology education. Drawing on the outcomes of this study, biology teachers are encouraged to revitalize their instructional practices by integrating metacognitive guidance to enhance students’ learning experiences and outcomes in biology. Educators should consider integrating similar metacognitive activities in their teaching practices, incubating collaborative learning atmosphere where students can discuss and analyze the subject matter content together.
To conclude, this study demonstrates the positive impact of a metacognition-based biology course on eighth-grade students’ biology comprehension and metacognitive skills. Involving students in metacognitive questioning activities enhanced their understanding of complex biological concepts and promoted the development of metacognitive processing. The findings highlight the inclusivity of the metacognitive learning course, benefiting students with heterogeneous learning approaches. Collaborative learning experiences facilitated by metacognitive prompts contributed to a positive classroom environment and deepened students’ comprehension of biology topics.
The raw data supporting the conclusions of this article will be made available by the authors, without undue reservation.
The studies involving humans were approved by the Institutional Review Board of the Alikhan Bokeikhan University. The studies were conducted in accordance with the local legislation and institutional requirements. Written informed consent for participation in this study was provided by the participants’ legal guardians/next of kin.
ASa: Conceptualization, Supervision, Writing – original draft. MI: Conceptualization, Methodology, Writing – original draft. GI: Conceptualization, Formal analysis, Writing – original draft. AI: Formal analysis, Investigation, Writing – review & editing. ASo: Data curation, Writing – review & editing. KM: Investigation, Writing – review & editing.
The author(s) declare that no financial support was received for the research, authorship, and/or publication of this article.
The authors declare that the research was conducted in the absence of any commercial or financial relationships that could be construed as a potential conflict of interest.
All claims expressed in this article are solely those of the authors and do not necessarily represent those of their affiliated organizations, or those of the publisher, the editors and the reviewers. Any product that may be evaluated in this article, or claim that may be made by its manufacturer, is not guaranteed or endorsed by the publisher.
Ain, Q., Thurston, A., MacKenzie, A., and Ozkaya, C. (2023). What does previous research tell us about the effects of peer tutoring on metacognition in primary and secondary schools? Int. J. Educ. Res. Open. 4:100248. doi: 10.1016/j.ijedro.2023.100248
Antonio, R. P., and Prudente, M. S. (2021). Metacognitive argument-driven inquiry in teaching antimicrobial resistance: effects on students’ conceptual understanding and argumentation skills. J. Turk. Sci. Educ. 18, 192–217. doi: 10.36681/tused.2021.60
Bandura, A. (1986). Social foundations of thought and action: A social cognitive theory. Englewood Cliffs: Prentice-Hall.
Bettayeb, A. M., Talib, M. A., Altayasinah, A. Z. S., and Dakalbab, F. (2024). Exploring the impact of ChatGPT: conversational AI in education. Front. Educ. 9:1379796. doi: 10.3389/feduc.2024.1379796
Biwer, F., de Bruin, A., and Persky, A. (2023). Study smart–impact of a learning strategy training on students’ study behavior and academic performance. Adv. Health Sci. Educ. 28, 147–167. doi: 10.1007/s10459-022-10149-z
Bowden, A. G., and Purper, C. J. (2024). Students’ perceptions of an online metacognitive journal assignment. J. Educ. Online 21, 1–14. doi: 10.9743/JEO.2024.21.1.15
Brausch-Böger, M. E., and Förster, M. (2024). The effects of an entrepreneurial project on the career-choice readiness, metacognition, and growth mindset of secondary students. Educ. Sci. 14:485. doi: 10.3390/educsci14050485
Cherrier, S., Le Roux, P. Y., Gerard, F. M., Wattelez, G., and Galy, O. (2020). Impact of a neuroscience intervention (NeuroStratE) on the school performance of high school students: academic achievement, self-knowledge and autonomy through a metacognitive approach. Trends Neurosci. Educ. 18:100125. doi: 10.1016/j.tine.2020.100125
Chimbunde, P., and Moreeng, B. B. (2024). The sustainability of curriculum reform and implementation through teacher participation: evidence from social studies teachers. J. Curric. Stud. Res. 6, 83–98. doi: 10.46303/jcsr.2024.6
Chow, C. W., and Chapman, E. (2018). Construct validity of the two-factor revised learning process questionnaire in a Singapore high school. J. Educ. Dev. Psychol. 8, 159–173. doi: 10.5539/jedp.v8n2p159
Flavell, J. H. (1979). Metacognition and cognitive monitoring: a new area of cognitive-developmental inquiry. Am. Psychol. 34, 906–911. doi: 10.1037/0003-066x.34.10.906
Glassman, M., Lin, T. J., and Ha, S. Y. (2023). Concepts, collaboration, and a company of actors: a Vygotskian model for concept development in the 21st century. Oxf. Rev. Educ. 49, 137–152. doi: 10.1080/03054985.2022.2028611
Halmo, S. M., Yamini, K. A., and Stanton, J. D. (2024). Metacognition and self-efficacy in action: how first-year students monitor and use self-coaching to move past metacognitive discomfort during problem solving. CBE Life Sci. Educ. 23:13. doi: 10.1187/cbe.23-08-0158
Hidayat, R., and Ying, S. T. D. (2023). The sub-dimensions of metacognition and their influence on modeling competency. Humanit. Soc. Sci. Commun. 10:763. doi: 10.1057/s41599-023-02290-w
Jaeger, A. J., and Fiorella, L. (2024). Metacognitive effects of instructional visuals: the role of cue use and judgment type. Metacogn. Learn. 19, 249–291. doi: 10.1007/s11409-023-09370-x
Korotkova, I. G., Dzhezherya, T. V., and Zhakenova, Z. Z. (2019). Biology. Grade 8 textbook. Nazarbaev Intellektual’nye shkoly. Available at: https://shorturl.at/guwIT
Kramarski, B., and Zoldan, S. (2008). Using errors as springboards for enhancing mathematical reasoning with three metacognitive approaches. J. Educ. Res. 102, 137–151. doi: 10.3200/joer.102.2.137-151
Le, H. V., and Nguyen, L. Q. (2024). Promoting L2 learners’ critical thinking skills: the role of social constructivism in reading class. Front. Educ. 9:1241973. doi: 10.3389/feduc.2024.1241973
Lucas, L., Helikar, T., and Dauer, J. (2022). Revision as an essential step in modeling to support predicting, observing, and explaining cellular respiration system dynamics. Int. J. Sci. Educ. 44, 2152–2179. doi: 10.1080/09500693.2022.2114815
Marton, F., and Saljo, R. (1976). On qualitative differences in learning: I. Outcome and process. Br. J. Educ. Psychol. 46, 4–11. doi: 10.1111/j.2044-8279.1976.tb02980.x
Mevarech, Z. R., and Kramarski, B. (1997). Improve: a multidimensional method for teaching mathematics in heterogeneous classrooms. Am. Educ. Res. J. 34, 365–394. doi: 10.3102/00028312034002365
Nilimaa, J. (2023). New examination approach for real-world creativity and problem-solving skills in mathematics. Trends High. Educ. 2, 477–495. doi: 10.3390/higheredu2030028
Perosanz, J. J. I., and Hayes, A. F. (2021). Mediation, moderation, and conditional process analysis: concepts, computations, and some common confusions. Span. J. Psychol. 24:e49. doi: 10.1017/sjp.2021.46
Ramlah, A., Abadi, A. P., Aisyah, D. S., Lestari, K. E., and Yudhanegara, M. R. (2023). Digital puzzle worksheet for identifying metacognition level of students: a study of gender differences. European J Ed Res 12, 795–810. doi: 10.12973/eu-jer.12.2.795
Recede, R. A. A., Capati, A. T., Yangco, R. T., and Castro, M. A. (2020). Metacognitive analogy instruction: effects on students’ reflective thinking in learning biology. J. Pendidik. MIPA. 21, 12–21. doi: 10.23960/jpmipa/v21i1.pp12-21
Roorda, G., De Vries, S., and Smale-Jacobse, A. (2024). Using lesson study to help mathematics teachers enhance students’ problem-solving skills with teaching through problem solving. Front. Educ. 9:1331674. doi: 10.3389/feduc.2024.1331674
Saqr, M., Lopez-Pernas, S., Jovanovic, J., and Gasevic, D. (2023). Intense, turbulent, or wallowing in the mire: a longitudinal study of cross-course online tactics, strategies, and trajectories. Internet High. Educ. 57:100902. doi: 10.1016/j.iheduc.2022.100902
Schraw, G., and Dennison, R. S. (1994). Assessing metacognitive awareness. Contemp. Educ. Psychol. 19, 460–475. doi: 10.1006/ceps.1994.1033
Shin, Y., Jung, J., and Lee, H. J. (2024). Exploring the impact of concept-oriented faded WOE and metacognitive scaffolding on learners’ transfer performance and motivation in programming education. Metacogn. Learn. 19, 147–168. doi: 10.1007/s11409-023-09362-x
Silver, D., Singh, S., Precup, D., and Sutton, R. S. (2021). Reward is enough. Artif. Intell. 299:103535. doi: 10.1016/j.artint.2021.103535
Sperling, R. A., Howard, B. C., Miller, L. A., and Murphy, C. (2002). Measures of children's knowledge and regulation of cognition. Contemp. Educ. Psychol. 27, 51–79. doi: 10.1006/ceps.2001.1091
Stebner, F., Schuster, C., Weber, X. L., Greiff, S., Leutner, D., and Wirth, J. (2022). Transfer of metacognitive skills in self-regulated learning: effects on strategy application and content knowledge acquisition. Metacogn. Learn. 17, 715–744. doi: 10.1007/s11409-022-09322-x
Suliani, M., Juniati, D., and Lukito, A. (2024). The influence of student’s mathematical beliefs on metacognitive skills in solving mathematical problem. Int. J. Eval. Res. Educ. 13, 1481–1491. doi: 10.11591/ijere.v13i3.27117
Sutarto, S., Hastuti, I. D., Fuster-Guillen, D., Garay, J. P. P., Hernandez, R. M., and Namaziandost, E. (2022). The effect of problem-based learning on metacognitive ability in the conjecturing process of junior high school students. Educ. Res. Int. 2022, 1–10. doi: 10.1155/2022/2313448
Sweller, J. (1988). Cognitive load during problem solving: effects on learning. Cogn. Sci. 12, 257–285. doi: 10.1016/0364-0213(88)90023-7
Teng, M. F. (2022). Effects of cooperative-metacognitive instruction on EFL learners’ writing and metacognitive awareness. Asia Pac. J. Educ. 42, 179–195. doi: 10.1080/02188791.2020.1835606
Teng, M. F., and Yue, M. (2023). Metacognitive writing strategies, critical thinking skills, and academic writing performance: a structural equation modeling approach. Metacogn. Learn. 18, 237–260. doi: 10.1007/s11409-022-09328-5
Tibell, L., and Rundgren, C. (2010). Educational challenges of molecular life science: characteristics and implications for education and research. CBE Life Sci. Educ. 9, 25–33. doi: 10.1187/cbe.08-09-0055
Vygotsky, L. S. (1978). Mind in society: The development of higher psychological processes. Cambridge: Harvard University Press.
Wang, C. Y. (2015). Scaffolding middle school students’ construction of scientific explanations: comparing a cognitive versus a metacognitive evaluation approach. Int. J. Sci. Educ. 37, 237–271. doi: 10.1080/09500693.2014.979378
Zeitlhofer, I., Hormann, S., Mann, B., Hallinger, K., and Zumbach, J. (2023). Effects of cognitive and metacognitive prompts on learning performance in digital learning environments. Knowledge 3, 277–292. doi: 10.3390/knowledge3020019
Zhan, Y., Yan, Z., Zhan, Y., Wang, X., Zeng, Y., Yang, M., et al. (2023). Effects of online peer assessment on higher-order thinking: a meta-analysis. Br. J. Educ. Technol. 54, 817–835. doi: 10.1111/bjet.13310
Zheldibayeva, R. (2023). The adaptation and validation of the global competence scale among educational psychology students. Int. J. Educ. Pract. 11, 35–46. doi: 10.18488/61.v11i1.3253
Keywords: deep learning approach, metacognition, metacognitive questioning, school biology, surface learning approach
Citation: Sadykova A, Iskakova M, Ismailova G, Ishmukhametova A, Sovetova A and Mukasheva K (2024) The impact of a metacognition-based course on school students’ metacognitive skills and biology comprehension. Front. Educ. 9:1460496. doi: 10.3389/feduc.2024.1460496
Received: 06 July 2024; Accepted: 07 October 2024;
Published: 23 October 2024.
Edited by:
Lindelani Mnguni, University of the Witwatersrand, South AfricaReviewed by:
Sueb Sueb, State University of Malang, IndonesiaCopyright © 2024 Sadykova, Iskakova, Ismailova, Ishmukhametova, Sovetova and Mukasheva. This is an open-access article distributed under the terms of the Creative Commons Attribution License (CC BY). The use, distribution or reproduction in other forums is permitted, provided the original author(s) and the copyright owner(s) are credited and that the original publication in this journal is cited, in accordance with accepted academic practice. No use, distribution or reproduction is permitted which does not comply with these terms.
*Correspondence: Ainur Sadykova, YWlzYWRAZ214LmNvbQ==
Disclaimer: All claims expressed in this article are solely those of the authors and do not necessarily represent those of their affiliated organizations, or those of the publisher, the editors and the reviewers. Any product that may be evaluated in this article or claim that may be made by its manufacturer is not guaranteed or endorsed by the publisher.
Research integrity at Frontiers
Learn more about the work of our research integrity team to safeguard the quality of each article we publish.