- 1Hwa Chong Institution, Singapore, Singapore
- 2National Institute of Education, Nanyang Technological University, Singapore, Singapore
Few studies in the education literature have focused on integrated STEM in a comprehensive manner and, even among these, there does not seem to be a significant emphasis on all the four disciplines. In addition, there are hardly any studies that focus on transitioning across prototype variants to demonstrate students’ understanding of what they must grapple with, including STEM concepts while coming up with a product. This qualitative study explored how a group of high school students harnessed the constituent disciplines of STEM to fabricate an interactive exhibit on laminar flow in an iterative manner. It was found that in the process of prototyping a working model of the exhibit, the students were able to demonstrate a working understanding of 37 relevant concepts in the four STEM disciplines, with science (14) and engineering (10) predominating, followed by technology (8) and mathematics (5). The students were able to incorporate four levels of interactivity in the exhibit, and this allowed visitors to explore the factors affecting laminar flow in the exhibit during a public exhibition in the school. Examination of the STEM dispositions of the students indicated a positive influence as a result of the activity. To the best of our knowledge, this study has incorporated the highest number of STEM concepts. We argue for the need for more studies that integrate all the four constituent disciplines of STEM in promoting integrated STEM education.
Introduction
STEM education has become an important area of engagement for educational stakeholders. The importance of STEM is underpinned by the fact that it is a key driver of contemporary economic development. It is thus important for schools to prepare students to be active participants in the new economy. STEM promotes 21st century skills such as especially complex problem-solving and teamwork (for example, Struyf et al., 2017; Salonen et al., 2017). A learning environment that is student-centric and collaborative can facilitate the promotion of such skills (Gasiewski et al., 2012; Brush and Saye, 2000).
While science, technology, engineering and mathematics constitute STEM, examination of the school curricula available on the web in a number of countries−for example, Singapore, India, Malaysia and the UK, shows that engineering and technology are not offered as subjects. In the USA, several high schools offer an introductory course on engineering. In the case of Singapore, where this study was located, to a certain extent, technology is covered in many schools in the subject of Design and Technology as well as being embedded in lesson delivery utilizing PowerPoint presentations and use of Internet resources. With the COVID-19 outbreak, several schools have also pivoted to online learning and use of zoom technology for teaching when physical attendance in schools was not possible at certain times. The science and mathematics curricula in Singapore are internationally well regarded. A recent book focused on this aspect (Tan et al., 2021). ICT infrastructure and laboratory facilities are well-resourced in Singapore schools. To the best of our knowledge, STEM as a subject is not offered in the curricula of any country−presumably, this would have allowed the subject to be taught in an integrated manner for students to experience how the constituent disciplines of STEM are intertwined.
Owing to the foregoing issues, STEM as an integrated discipline is mainly promoted via school-based project work or informal science education initiatives. Since these are not usually compulsory, exposure of students to STEM in an integrated manner is very much on an ad-hoc basis. In the context of the foregoing, there is a need for students to be exposed to integrated STEM activities. These would be more on hands-on project work rather than those centered on formal instruction. Such programs should preferably be conducted over a period of time rather than be completed over a single session.
Keeping in mind the foregoing, the present study sought to explore how a group of students fabricated an interactive exhibit that incorporated the four constituent disciplines of STEM. The medium of exhibit was chosen as this allows students to come up with variants of a prototype, with the final version being available for interaction by visitors and students.
Literature review on integrated STEM education
The abbreviation STEM, when it was first introduced in the 1990s, referred to the various disciplines within it. That means STEM can refer to any one of science, technology, engineering or mathematics. The focus on STEM integration was a later development that sought to harmonize and tap on the synergies within the respective disciplines. One definition of integrated STEM is “the seamless amalgamation of content and concepts from multiple STEM disciplines” (Nadelson and Seifert, 2017, p. 221). The authors also indicate that the “integration takes place in ways such that knowledge and processes of the specific STEM disciplines are considered simultaneously, without regard to the discipline, but rather in the context of a problem, project or task” (Nadelson and Seifert, 2017, p. 221). Another definition is by Moore et al. (2015), who define integrated STEM as the “teaching and learning of the content and practices of disciplinary knowledge which include science and/or mathematics through the integration of the practices of engineering and engineering design of relevant technologies”. Further the same authors emphasize that “while any discipline can also have learning goals in integrated STEM environments, mathematics, science, and engineering will be the primary goals”.
It has not been unequivocally articulated in the literature that all four disciplines need to be integrated when working on integrated STEM. Quite a number of studies on integrated STEM education have two or more disciplines woven together, a point noted in the excellent review by Thibaut et al. (2018), and references therein. This was earlier noted in the thought-provoking commentary by English (2016), who mentioned that “studies that address multiple disciplines appear scant, with mixed findings and inadequate directions for STEM advancement”. She also noted that “mathematics learning benefits less than the other disciplines in programs claiming to focus on STEM integration” and that “learning outcomes for engineering in K-12 integrated STEM programs appear under-researched”. Overall, English (2016) argues for more focus on the disciplines of mathematics and engineering within integrated STEM. Inadequate discipline representations in STEM studies that focus on learning have been a source of concern−for example, English, 2016; Hoachlander, 2014.
To promote integrated STEM education, the new National Science, Technology and Engineering standards (NGSS, 2013) in the USA have emphasized on the need for integrated learning while ensuring that the separate STEM disciplines are still given importance. Pursuant to this, curricula have been developed across grade levels to mainstream technology and engineering in US schools. For example, the study by Phelps et al. (2018) noted that high school students in USA who attended engineering and engineering technology courses are 1.6 times more likely to enroll in STEM programs in tertiary institutions than those who did not enroll for these courses. In a review of the presence and extent of engineering in K-12 STEM Standards in the US, Carr et al. (2012) noted that “While engineering standards do exist, uniform or systematically introduced engineering standards are less prevalent”.
It has been recognized that implementing integrated STEM education in schools, where there is a clear demarcation between the disciplines, especially science and mathematics, is fraught with challenges with respect to curriculum and lesson delivery (Nadelson and Seifert, 2017). Resources such as DIY tools, materials (for example, wood, plastic, Styrofoam, cardboard and glue) and measuring equipment are essential (Stohlmann et al., 2012). In addition, teachers who have been trained in traditional disciplines such as Physics, Chemistry, Biology or Mathematics may not have the skill sets necessary to conduct such courses in the absence of professional development programs. This is especially true when it comes to integrating engineering and technology with science and mathematics. That means school-wide implementation, in the absence of directives from the education authorities, can only be on an ad hoc basis.
There has been little consensus in the literature on how integrated STEM education can be promoted with respect to teaching and learning. As noted in the review by Thibaut et al. (2018), though there are examples of how this could be conducted, researchers have not explained the instructional frameworks for their design (for example, Barrett et al., 2014; Gentile et al., 2012) or the theoretical backgrounds for the approaches espoused (for example, Moore et al., 2014; Sanders, 2009).
When examining the instructional strategies used in studies on integrated STEM education in the foregoing references and those in the reviews, it is noted that most focused on design-based learning, inquiry-based learning, problem-based learning, or project-based learning. Notwithstanding the nomenclature used, since there is a blurring of boundaries among these terms, it is clear that hands-on work of a project nature in a group setting is involved. Also, most studies focused on the secondary level. This is not surprising since a certain level of prior knowledge is needed before students can embark on such programs. At the secondary level, students would have good foundational knowledge in the sciences and mathematics, and these would come in useful as they work on integrated STEM activities.
We feel that integration across all the four disciplines of STEM would be desirable to facilitate better learning. This would allow students to harness the synergies inherent in each discipline and bring these to bear on a task. Real world scenarios occur in an integrated manner, a point also noted by Cheng and So (2020) as well as Moore et al. (2015). Indeed, Kelley and Knowles (2016) argue that “all four domains of integrated STEM must occur during every STEM learning experience”. There is also a perception among teachers that integration of STEM requires all four disciplines even though they are not able to articulate how this can be enacted in practice (Breiner et al., 2012). Ideally, STEM integration needs to also focus on the interdisciplinary processes (English, 2016) during the navigation from one discipline to another.
Project-based learning in the four STEM disciplines has a long history, with its pros and cons well recognized - for example, Mihic and Zavrski (2017) and Kokotsaki et al. (2016). The key attributes of project-based learning include knowledge construction in an active setting, situated nature of the learning experience, group work by students, and leverage on cognitive tools such as graphs and computer software (Krajcik and Blumenfeld, 2006). The task that students engaged in our study mirrors these aspects, especially the first three.
Our focus in this study is on project work on integrated STEM. We review relevant references in this area. Integrated STEM has been promoted through project-based learning−for example, Han et al. (2015) and Tseng et al. (2013) as well as through programs spread across a few years−for example, Han et al. (2023). The edited book by Capraro et al. (2013) focuses solely on this. The approach has been found to be useful to promote content across disciplines (Sahin, 2019), foster critical thinking among primary students (Gandi et al., 2019), promote engagement (Hall and Miro, 2016), improve attitudes towards STEM (Tseng et al., 2013), and develop skills needed for the 21st century (Baran et al., 2021). Further examples of projects on integrated STEM include the following: designing and building an autonomous car, museum security system, and rehabilitation device (Struyf et al., 2019), with the samples being grade 9 students; community builder, nanotechnology, Mars rover, and energy (Gardner and Tillotson, 2020), with middle school students being the samples; electrolysis (Huri and Karpudewan, 2019), with secondary students being the samples; and fabricating technical toys (Quang et al., 2015), with 8th grade students as samples. While these studies have been shown to promote interest in integrated STEM education among students, these have not harnessed adequately the unique affordances of the four disciplines within STEM. We also note the paucity of studies that focus on providing high ability students with hands-on experiences involving integrated STEM projects.
In integrated STEM, it is very important that especially the disciplines of technology and engineering are clearly included so that students know what they have to grapple with. In general, technology can be defined as “anything that is human-made and makes life easier” (Stohlmann et al., 2012). As for engineering, it involves a few processes such as design, build and iterate (Strimel and Grubbs, 2016), among others.
Integrated STEM programs have been reported to promote positive dispositions towards STEM among middle school students (Christensen et al., 2015; Christensen and Knezek, 2017; Knezek and Christensen, 2020). Simply put, disposition refers to inclination towards something, and it is known to be a complex construct. There is thus a need for more of such programs to be conducted in schools.
Examination of the literature shows the following gaps that are worth bridging:
1. While most studies on integrated STEM education have focused on a limited number of disciplines, few studies have focused on the full complement of disciplines constituting STEM.
2. Most studies on integrated STEM have focused on a limited number of concepts within a number of these disciplines. A focus on studies that entail multiple concepts within each STEM discipline can better illustrate how students navigate boundary crossings within these disciplines.
3. There are hardly any studies that focus on how transitioning across prototype variants in integrated STEM projects demonstrate students’ thinking of what they have to grapple with in the course of coming up with a product.
4. There are hardly any studies that have focused on high ability students.
5. In relation to hands-on activities that promote integrated STEM, only a limited number of studies have been reported in the mainstream literature.
6. Fabrication of an interactive exhibit does not seem to have been explored in integrated STEM projects.
The current study was thus initiated with the above gaps in mind. The specific research questions that we wished to explore were as follows:
1. What do the different phases of prototyping indicate about the students’ progress in using STEM to fabricate an exhibit?
2. What concepts in science, technology, engineering and mathematics do students leverage in the process of building the exhibit?
3. What effect does the experience have on the students’ dispositions towards STEM?
Theoretical frameworks
It is unlikely that a single theoretical tradition can provide sufficient ballast to undergird the conceptual underpinnings of the work reported here. Though a few frameworks for integrated STEM education have been proposed−for example, Johnson et al. (2021) Hallström and Ankiewicz (2023) and Ortiz-Revilla et al. (2020), Bryan et al. (2015) and Kelley and Knowles (2016), we prefer to use three frameworks commonly used in the science education literature, all of which are also found in the foregoing references to varying extents.
The first framework is on situated cognition (Brown et al., 1989; Lave and Wenger, 1991). The theory posits that the learner’s cognition and the situation (or contexts) in which the learning takes place are not separate. With the proper choice of activity, it is possible for students to develop the requisite knowledge and skills necessary during the learning process (Putnam and Borko, 2000). More importantly, the learning is authentic since it relates to the task at hand and not something that needs to be imbibed as part of passive pedagogy.
The second framework refers to socio-constructivism. Vygotsky’s theory of socio-cultural learning (Tzuriel and Tzuriel, 2021) emphasizes that learning is a social activity, and that individuals learn better in groups since there is scope for negotiation of understanding while discussing ideas. Thus, collectively, more learning takes place. Key to realizing this framework is judicious choice of students in a group. What is clear is that students need to be given opportunities that promote teamwork (Guzey et al., 2016), communication skills (Bryan et al., 2015) and positive interdependence (Asghar et al., 2012).
The third framework is on engineering design. This can provide the fulcrum to integrate, especially science and mathematics (National Research Council [NRC], 2012). When students engage in hands-on projects that involve engineering design challenges, they can learn more about engineering practices, design challenges and integrating cross-disciplinary ideas (Guzey et al., 2016; Hernandez et al., 2014). Engaging in engineering practices also helps students to bridge the chasm between theoretical knowledge in STEM and the challenges of their applications in real world settings (Riskowski et al., 2009). Moreover, engineering design provides scope to identify the points of convergence within the STEM disciplines and build on these (Frykholm and Glasson, 2005; Kelley and Knowles, 2016).
We now elaborate on how our study draws on the foregoing frameworks. The first framework on situated cognition is inherent in the nature of the project−for example, as students fabricate the interactive exhibit, their learning experiences are very much inherent in the prototypes they iteratively develop; that is, these are linked to the task they are focused on and learn from. The second framework on social constructivism is related to the knowledge building that occurs in the group work setting that the students work in. The third framework on engineering design is harnessed as students work on designing and fabricating the exhibit iteratively.
Materials and methods
Research design
A qualitative case study approach (Creswell, 2007) was adopted for data collection, with a group of three students constituting the samples for the project-based learning experience. This allows for multiple methods of data collection - questionnaire, examination of entries in logbooks, inspection of prototypes at various stages of iterations, and reflections by students. Also, there are insights to be obtained from the teacher facilitator supervising the project. The approach is also well suited to answering the research questions, which we elaborate in the section on data analysis.
Context and participants
The high school where the study was conducted has a policy where all Grade 8 to 10 students are required to work on a project for 6 months under the mentorship of a teacher, comprising about 2 h per week during term time (5 months) and about 8 h per week during the month-long school vacation. The project work aimed to provide students with well-rounded experiences−for example, doing research, collaborative work, engagement with real world contexts and learning beyond the classroom.
A group of Grade 9 male students (N = 3) (with pseudonyms Royston, Henry and Zach) constituted the samples for this study. Based on their results from their Grade 6 primary school national leaving level examinations, as well as their Grade 8 school-based examinations, the students can be regarded as being of high academic ability. Grades 7–10 refer to secondary level while grades 11–12 are junior college level.
The first author was the teacher-mentor of the students, and he supervised the project carried out by the students.
Project
The students in this study were set a challenge by their teacher-mentor−to come up with an interactive exhibit that could integrate science, technology, engineering and mathematics. Interactivity here means that it allows for hands-on participation by visitors−that is, they can fiddle with it in a few ways to learn more about the science behind the exhibit. Importantly, the exhibit needs to be reasonably robust and not vulnerable to damage. These were the basic criteria for the exhibit. As the problem was sufficiently open-ended, they had the flexibility to focus on any suitable topic. The students were allowed to make use of the facilities and items in the laboratory. A small budget (about US$50) was also made available for purchase of any other items.
Group members took the initiative to visit the local science center to learn more about the various science exhibits and observed how visitors interacted with these exhibits. Though group discussions and reflections, they learnt how some exhibits appeared fun to play with, but visitors end up learning little science, as well as the reasons behind why visitors ignore or spend little time at some exhibits (which translates into little learning). At the same time, they also realized why other exhibits are fun to play with as well as why visitors were more engaged in the learning experience. The visit gave them some ideas on how to make their choice of exhibit more interactive.
The students decided to work on an exhibit that could demonstrate laminar flow (which was not available at the science center) and managed to obtain the agreement of their teacher-mentor after discussion. Laminar flow is a phenomenon where fluid (air in this case) travels smoothly in a regular path. They individually researched on the concepts behind the operation of a wind tunnel (via the Internet) and met up frequently (at least once a week) to share and discuss the concepts they had learnt. As a group, they brainstormed for ideas to showcase in their exhibit as well as how to turn these ideas into reality. They were also open to suggestions from one another.
The students also attended a workshop at the science center and obtained advice on prototyping. They received affirmation from the science center staff that the idea of constructing an exhibit on laminar flow was a good one, as the science center had previously planned to build a larger exhibit showcasing the same concept but was ultimately scrapped due to logistical challenges.
The students developed their prototypes in the school science laboratory. The prototypes were piloted on the classmates of the group members for feedback. For example, an improvement suggested was to add lights in the chamber incorporating the wing to enable the air flow to be more visible. This later led to the design and inclusion of a simple circuit with blue LED lights.
By and large, the students carried out the project on their own. They required minimal guidance and scaffolding from their teacher-mentor. There were two reasons for this: (a) the students were of high academic ability; and (b) as they were working in a group, with members having diverse capabilities, they were able to reasonably overcome challenges encountered in the project. The science laboratory technician taught them how to operate the 3D printer in the laboratory as well as helped to access materials available in the laboratory for their project.
Instruments
The group was requested to open a logbook to record their experiences−this included the labeled sketches as well as photographs of iterated versions of the prototypes; summaries of points raised during brainstorming sessions and other discussions; as well as specific science, technology, engineering and mathematics concepts that they perceived were involved in the fabrication of the exhibit. The students also created an A1-sized poster that helped to explain the concept and workings behind the exhibit.
To ascertain their dispositions towards STEM as a result of embarking on this project, a simple questionnaire was developed. While we consulted the literature in the development of the instrument, we realized that owing to the nature of the study, we have to essentially come up with an instrument that is more suited for this study. A preliminary version of the questionnaire was developed by the first author and reviewed by the second author. Minor refinements were made, with both authors in agreement on the final version of the questionnaire. That is, content validity and face validity constituted the validation process. The instrument had the following questions:
1. Before embarking on the project, what were your views and attitudes toward STEM?
2. What did you think contributed to these views and attitudes?
3. What are the main reasons you chose to take up a STEM-related project?
4. After completing the project, what were your views on, and attitudes toward STEM as compared to before embarking on the project?
5. Please provide up to 3 examples of specific incidences during the progress of the project and elaborate on how these incidences affected your views and attitudes towards STEM.
It was not possible to administer the instrument in a pretest/posttest configuration owing to the nature of the questions. Hence, it was administered after the students have completed the project.
Students were each also requested to submit one-page reflections of their experiences after going through this project. The mentor made simple notes of the students’ engagement levels while supervising the project.
Data analysis
For Research Question 1, the various prototypes that the students designed and fabricated were analyzed via the different components utilized by the students in fabricating the exhibit, with improvements across the prototypes noted and cross-compared. This was correlated with the labeled sketches and notes that the students had recorded in their logbook, and this helped to see how STEM concepts were used in the process of fabricating the exhibit.
For Research Question 2, the students’ records in their logbook and their replies to the questionnaire were studied for examples where concepts in science, technology, engineering and mathematics could be identified. While the students were fairly clear about the science and mathematics concepts they acquired whilst undertaking the project, the concepts related to technology and engineering did not seem to be so apparent to the students. As such, the technology and engineering concepts used were mainly inferred by the authors.
For Research Question 3, the responses to the questionnaire and the individual reflections submitted by the students were examined for common strands of thought emerging with respect to the students’ dispositions towards STEM. Through engagement and re-engagement with the data, these strands were allowed to emerge naturally rather than imposed by us. The first author carried out the coding of the responses, with the second author checking these for consistency. The authors discussed amongst themselves on ambiguous strands until in-principle agreement was achieved. These strands were grouped together and the associations amongst these examined. The categories were labeled accordingly.
Ethics approval
Ethics consent was provided by the Institutional Review Board of the second author’s university. Informed consent to participate in the research study was provided by the parents of the students.
Results
We present the findings according to the order of the research questions.
Research question 1: What do the different phases of prototyping indicate about the students’ progress in using STEM to fabricate the exhibit?
To answer this RQ, we examined the students’ logbook and the various prototypes they developed. The figures are from the logbook while the tables were compiled by the authors after examining their logbook.
In fabricating the exhibit, a process of iteration and experimentation was very much evident by the students. When analyzing the various prototypes created by the students, we observed that there was also a progression in the number of concepts used within the STEM disciplines. Through fabricating their prototypes, examining them for failures and incorporating improvements into the next design, the students learnt to incorporate STEM concepts in an integrated manner in their redesign process (Moore et al., 2014).
In prototyping the first version from a simple design sketch, the students were able to get a feel for what is needed to get the exhibit satisfactorily working. They recognized that for safety reasons, flow visualization is not desirable by using smoke from a cigarette or incense stick or by using sprinkled talcum powder. This led them to the use of a humidifier, which dispenses water vapor as a visible mist. Moving the water vapor emerging from the humidifier in an aligned manner posed difficulties but was resolved by directing the water vapor through a bundle of plastic straws. To aid in laminar flow, a fan was positioned at the front end, away from the humidifier at the other end. When the fan was switched on, the suction produced helped to draw the mist in a streamlined manner. A number of STEM concepts are already at work−for example, the design-n-build processes in coming up with the prototype is related to engineering; producing the water mist using a humidifier, directing it through a bundled configuration of straws and drawing the mist to the other end with the use of a fan are related to technology. The suction produced during the operation of the fan is related to concepts in pressure, that is, science. The material for use in the straw (plastic) is also related to science. Only basic mathematics ideas were apparent in the first version−that related to measurement of straw lengths. The first version was satisfactory but not fully functional. It is elaborated further in Figures 1, 2 as well as in Table 1, which is based on an examination of the prototype and logbook of the students. There were no elements of interactivity in the first prototype as the students focused more on getting the basics in place.
In transitioning to the second version, a number of improvements were apparent. These are summarized in Figures 3, 4 as well as in Table 2. A working version was obtained but not yet optimal. Again, there were no elements of interactivity in the second version of the prototype as the students wanted to first show laminar flow. Table 2 is also based on an examination of the prototype and logbook of the students.
The final version is shown in Figure 5. The improvements made are indicated in Table 3, which is also based on an examination of the prototype and logbook of the students. There were further enhancements in the demonstration of laminar flow, and the students incorporated a number of elements of interactivity for visitors to play with and, in the process, learn more about laminar flow.
The interactive elements in the final prototype are reminiscent of the participatory nature of many exhibits found in science centers. The following are the ways in which visitors to the exhibition on student projects in the school can interact with the exhibit:
1. Vary the angle of the 3-D printed wing (using the affixed protractor) to explore the relationship between air flow and angle of wing.
2. Vary the speed (2, 5 and 7 ms–1) of the box fan to explore how air flows to the other side.
3. Interchange the movable 3-parts section that incorporates no straws, straws of 0.5 cm diameter, and straws of 1.0 cm diameter to explore how air flow occurs as a function of straw diameter.
4. Switch on the blue LED light to compare the visibility of airflow with that when ambient light is present.
Varying the above parameters helps visitors explore some of the factors affecting laminar flow.
The students created a poster which was used to explain the working of the exhibit (Figure 6). The final prototype was entered into a competition organized by the local science center, and it won a prize.
Overall, the transitioning across the different phases of prototyping indicates a reasonable sophistication in the thought processes of the students and an increase in the number of STEM concepts that were leveraged. These would be elaborated in further detail in the next section.
Research question 2: What concepts in science, technology, engineering and mathematics did students leverage in the process of building the exhibit?
To answer this RQ, we examined the students’ logbook and their qualitative responses to the questionnaire.
Through the engineering design challenge, the students learnt new science and mathematics concepts beyond those encountered in their science syllabus. They engaged in problem solving processes similar to those undertaken by engineers and leveraged technologies for their project. The activities undertaken by the students mirror the STEM Integration framework closely (Moore et al., 2014).
Examination of the students’ logbooks showed that students grappled with several STEM concepts in the course of working on the exhibit. To further understand the variety of the STEM concepts encountered by the students during the course of the project, we have identified the concepts, and a summary is shown in Table 4. There are instances where they have shown acquisition of new concepts while other STEM concepts they have used were inferred by the authors from their logbook. While all the constituent elements of STEM (37) are present, the concepts involving science predominate (14), followed by engineering (10), while technology (8) and mathematics (5) had fewer components. It needs to be noted that in a number of cases in Table 4, the relevant constituents of STEM can be argued to occur in unison rather than individually. For mathematics, some of the concepts relate more to measurement and shape. What we have presented are the predominant disciplines for the respective key concepts. We elaborate on a few key aspects in this section.
While the findings above point to an increase in awareness of STEM by the students as a result of working on the project, we wanted to elucidate more information on the STEM-related actions that the students were involved in as well as how the students perceived that the construction of their exhibit was related to each of the components of STEM. We hence looked at the students’ logbooks, and extracted entries that we felt were examples pertinent to STEM concepts or activities. Moreover, we asked each of the students to provide examples of the STEM concepts learnt or activities carried out during the project and relate how they thought that the use of the concept or the nature of the activity contributed to the development of their product. From the logbook entries and student responses, we searched for commonalities in concepts and activities described, as well as highlight the variations that were raised. We noted that these could be described under two categories, the first being understanding of the STEM concepts and principles while undertaking the project and, second, application of these concepts and principles in overcoming design and construction challenges.
Developing conceptual comprehension
Upon examining the entries in the logbook, we noticed an entry that highlighted the inspiration for the students’ choice for the laminar flow exhibit, as well as related scientific concepts:
We had been wondering why Formula 1 cars had such unique and weird shapes. One thing led to another and we did some reading up on the fields of aerodynamics and fluid dynamics. Turns out that wind tunnels are used regularly in these fields. Why not build a simple, low-cost wind tunnel that can be used as an exhibit? After some further research into aerodynamics, we decided to use the wind tunnel to show how the angle of attack of a wing changes the flow of air around it. (Logbook, p19).
The students quickly recognized that the concept of laminar flow was the underlying principle behind fluid dynamics, as shown below in their logbook entry (Logbook, p19) along with a diagram to show this (Figure 7).
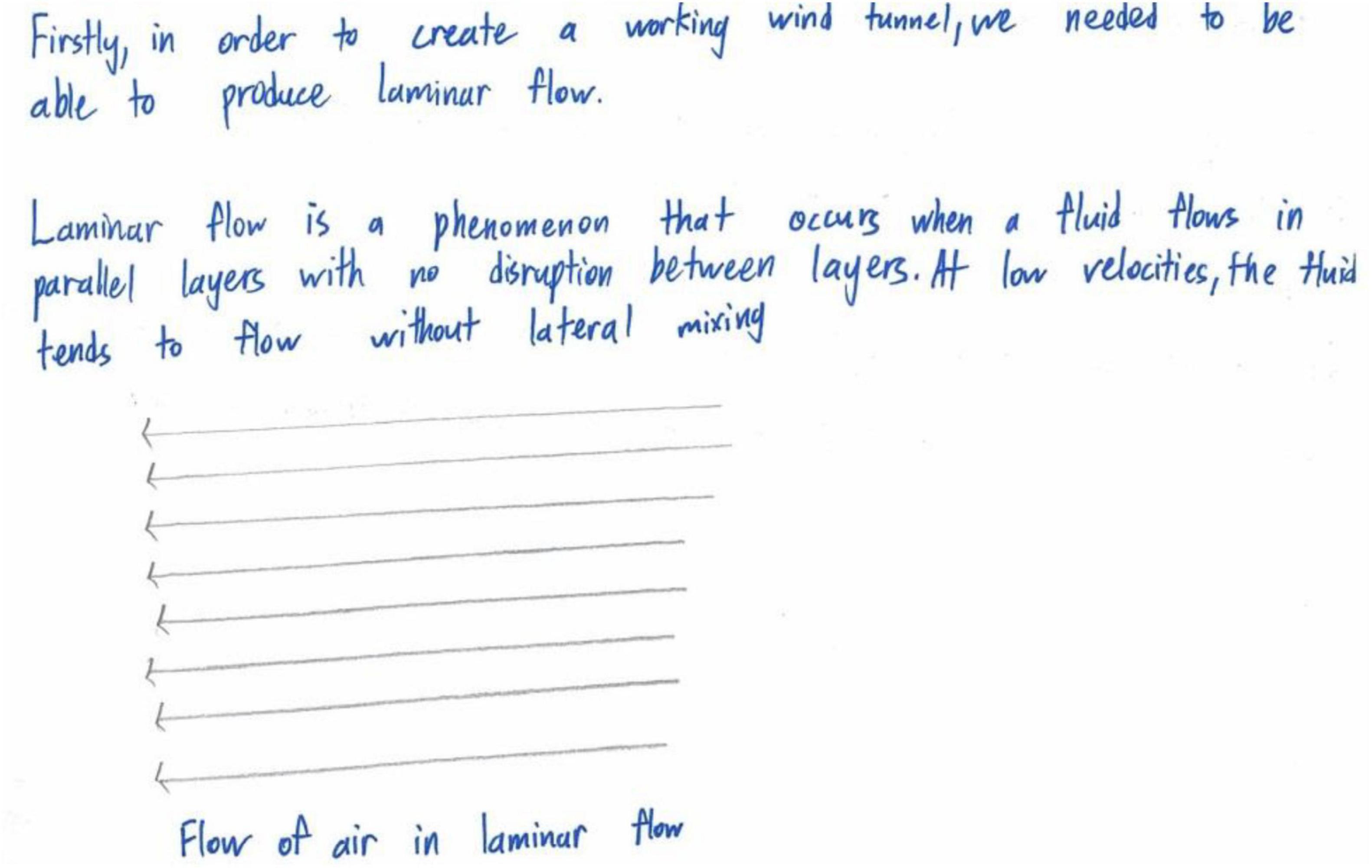
Figure 7. Excerpt from students’ logbook showing description of, and diagram representing laminar flow.
For the topic of aerodynamics, Bernoulli’s principle was ascertained by the students to be the concept that they had to comprehend. We found two pieces of evidence in the logbook demonstrating the students’ interpretation as well as application of Bernoulli’s principle in their attempts to obtain laminar flow through increasing the velocity of air. The first entry was logged during the initial brainstorming phase of the project, while the second entry occurred at the construction stage of the prototype. Both entries were accompanied by diagrams showing an increase in air velocity when air moves into a region of decreased surface area (Logbook, p19b, Figure 8).
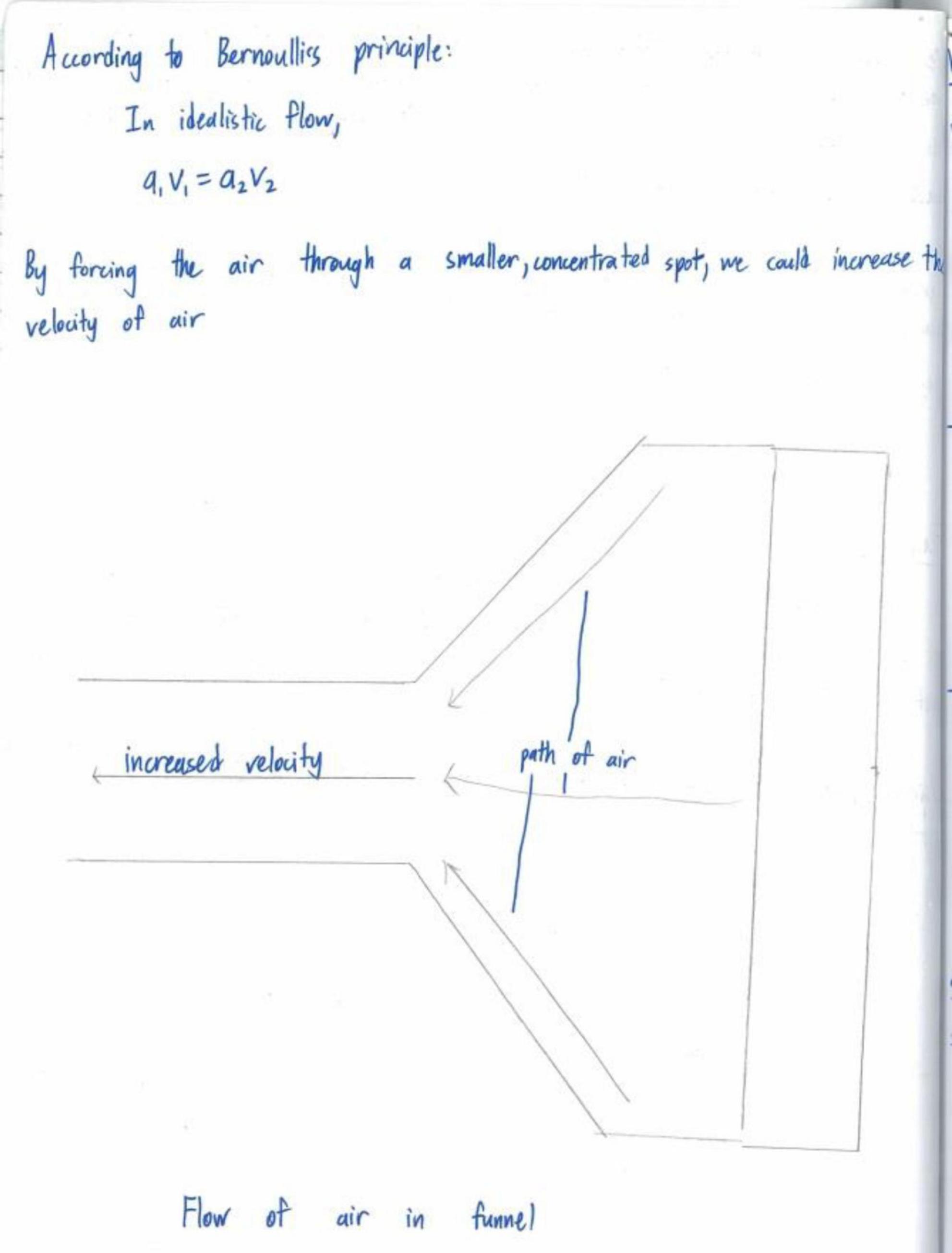
Figure 8. Excerpts from students’ logbook showing descriptions of, and diagrams representing Bernoulli’s principle.
Further, they noted the following:
Using Bernoulli’s flow principle, whereby a1v1 = a2v2, the funnel will increase wind speed as the wind is forced into a smaller container (a), resulting in a higher velocity (v). (Logbook, p26).
In their reflections and responses to the open-ended questionnaire, all three students emphasized the importance of understanding the scientific concepts behind laminar flow and Bernoulli’s principle for their project. The origins of these opinions stemmed from the fact that these were among the scientific principles that the exhibit would be showcasing, and these therefore come as no surprise that the group dedicated much time and effort to ensure that they comprehended the concepts well, prior to presenting them in the form of an exhibit. Sample entries follow.
Throughout the year, I had to get myself familiar with the concepts of aerodynamics very well, to understand explanations that I myself will have to make to judges and students alike. This included many different mechanics and while I was given the liberty of leaving out anything related to calculation, I had to understand the various factors which affect flight. I learnt of the paramount importance of the angle of attack, the very complicated and widely mistaken concept of mechanics of flight and such. I’ve learnt most of this through the NASA website. (Zach, questionnaire).
I had to read up on laminar flow, which is the parallel flowing of fluids without any lateral mixing and the flow of air around a car or a plane as the whole idea of the project revolves around this concept. I learnt that manufacturers make them so that the air around is laminar, so there is less air resistance. (Henry, questionnaire).
I learnt about the different types of air flow (laminar, transient, and turbulent) as well as the different types of laminar flow (creeping, etc.). This was used in the project as different variables in order to let the viewer observe how different conditions will induce different types of laminar flow. I also learnt about Bernoulli’s principle, which states that when, the speed of a moving fluid increase, the pressure decreases. This was one of the main concepts we wanted to show the viewers. Bernoulli’s principle was the basic concept behind what made planes fly. The curvature of the bottom of the wing caused the air moving pass it to slow down, creating higher pressure. The top of the wing had air at normal speed, so it was at a lower pressure. As the higher pressure at the bottom pushes up against the lower pressure at the top, the wing entire plane is able to go up. We displayed this in showing how the individual streams of air curved along the surface of the wing. (Royston, questionnaire).
For each of the two scientific concepts mentioned above - laminar flow and Bernoulli’s principle−an intimately linked mathematical equation was identified and further utilized by the group to obtain information from which they could construct their exhibit. The students acknowledged Reynolds number as “an important parameter that indicates whether a flow is laminar (smooth) or turbulent (choppy & rough)” (Logbook, p19), and provided two versions of the equation for Reynolds number in their logbook (Figure 9). They envisioned that “We will use the Reynolds number to determine the ideal speed of air in the exhibit as well as ideal length” (Logbook, p19a), and calculated the Reynolds number to confirm the establishment of laminar flow for their prototype (Logbook, p29c). The implications of the equation related to Bernoulli’s principle, which describes the inverse relationship between area and velocity for a fluid of constant density, were also apparent when the students elucidated that “by forcing the air though a smaller, concentrated spot, we could increase the velocity of air” (Logbook, p19b); in another logbook entry, the theoretical value for the air velocity for their prototype was calculated using the formula (Figures 9, 10).
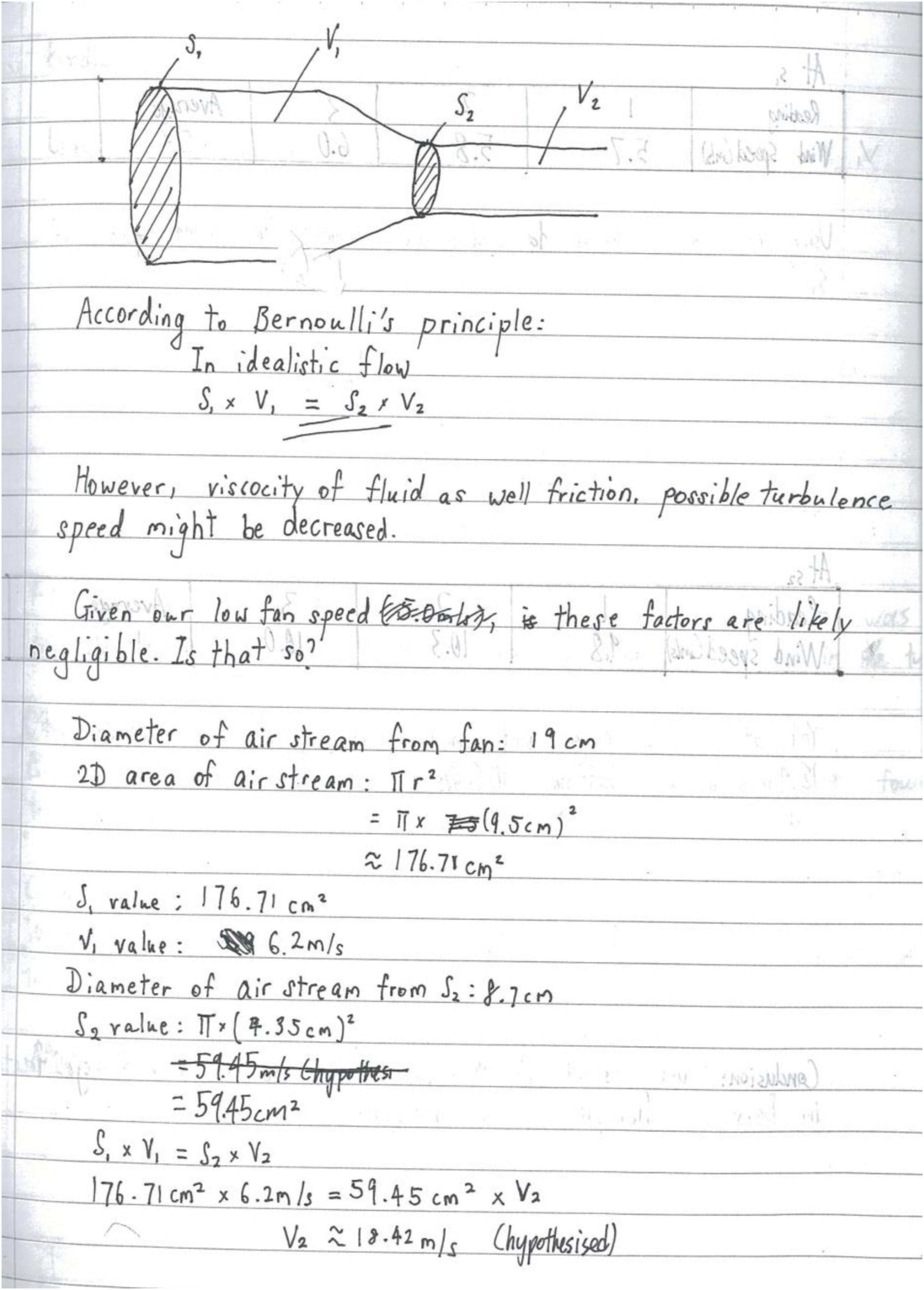
Figure 10. Excerpt from students’ logbook showing calculations using the equation related to Bernoulli’s principle.
The responses from the students were aligned with regards to the importance of the predominant mathematical concept which they felt was closely related to their understanding of the concept of laminar flow. Reynolds number was explicitly specified by Royston to be “an important formula and parameter that governs whether the flow of wind in the tunnel is turbulent or laminar” (Royston, questionnaire). Zach also stressed that the formula “was very crucial in order to calculate our parameters necessary” (Zach, questionnaire). Analysis of the responses revealed that the students’ comprehension of the application of Reynolds number to their project was also consistent:
The Reynolds number was used to ensure that what we were observing in the tunnel was indeed laminar flow. We also used it to determine the properties of the tunnel such as its length as well as the speed of the fan. (Royston, questionnaire).
……we had to use this formula to calculate our pipe length for the wind speed of the fan. (Henry, questionnaire).
We also used it to calculate a desirable laminator length for our straws… (Zach, questionnaire).
Although we could not find further evidence to establish if the students obtained a deep understanding of the relationship between the variables in the mathematical formulae, there were strong indications that at the very least, they were able to appreciate the importance of the formulae and employ these correctly to calculate the parameters relevant to their exhibit.
Meeting the practicalities of design and construction
There were three important issues that the team had to address during the design and construction of their exhibit. The first challenge that the team set out to tackle was producing and optimizing the laminar flow for their wind tunnel. Based on their preliminary research, they surmised that “In order to produce laminar flow, many commercial wind tunnels use honeycomb structures to separate the air into separate streams of air”, but through brainstorming, quickly deduced that “This would not be necessary, as we had found an alternative – straws” (Logbook, p19a). They went on to elaborate:
We could use normal drinking straws to sufficiently separate the air. This was one of our hypothesis. We are proud to say that we have actually thought of this idea, using a normal, everyday object to eliminate the need for making a honeycomb structure. Bundled straws are similar in structure to the traditional honeycomb structure save for the small gaps between straws, which can be easily plugged with glue or blu-tack. (Logbook, p19a).
A subsequent entry in the logbook revealed how the students bundled the straws into one end of an acrylic container (Figure 11). Another entry logged later in the course of the project indicated that the students also experimented with straws of different lengths, in order to determine the optimal straw length for clear and visible laminar flow (Logbook, p30-31a); eventually, this idea is incorporated into the final exhibit as one of the variables that could be changed by visitors interacting with the exhibit (Content of accompanying poster).
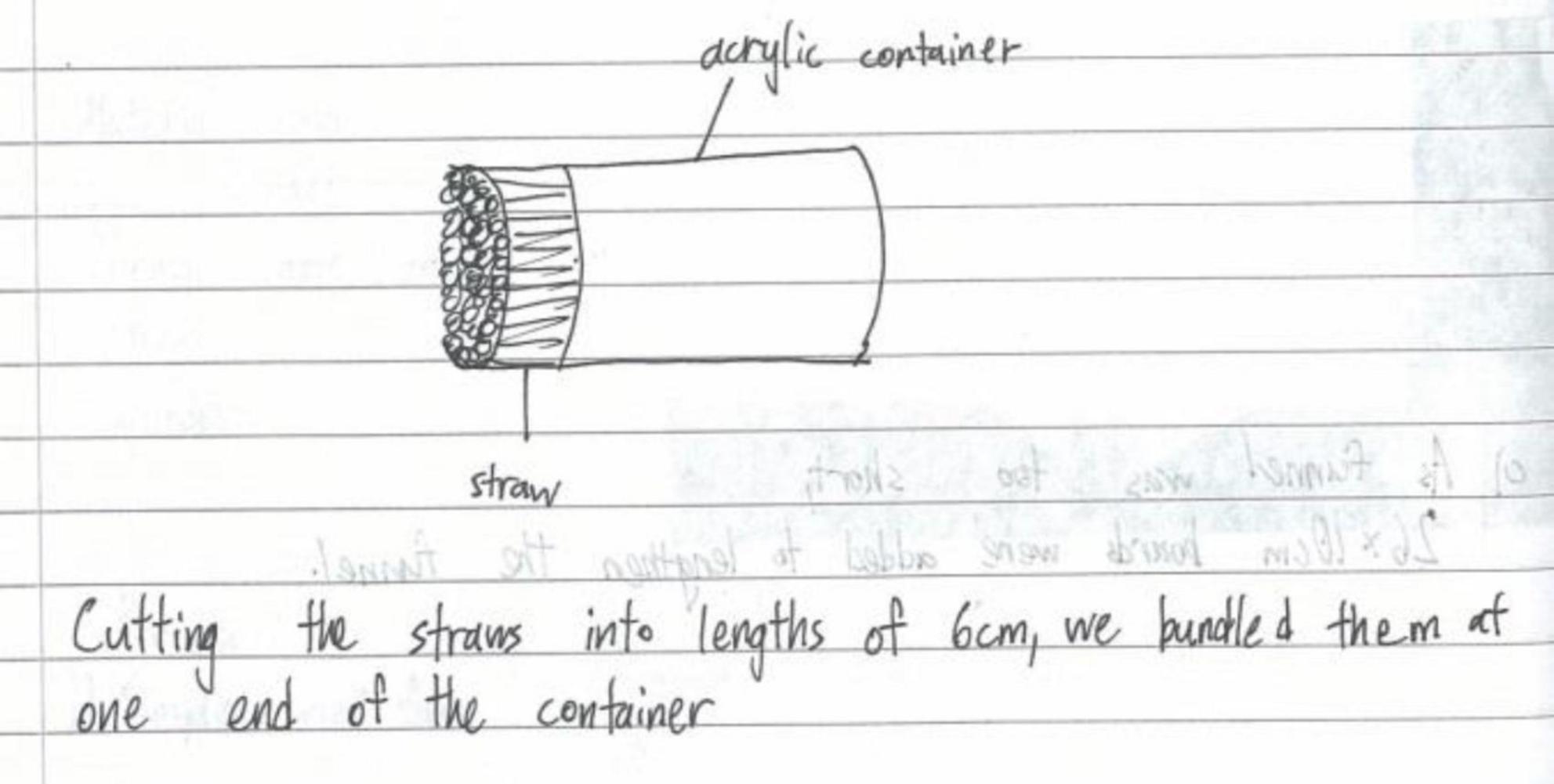
Figure 11. Excerpt from students’ logbook showing their idea of bundling of straws into one end of an acrylic container, as an alternative to honeycomb structures.
In addition to testing out different lengths of straws, the team also investigated the effect of different wind speeds on the quality of laminar flow, conjecturing that “our hypothesis was that the higher the wind speed, the more visible and strong the laminar flow is” (Logbook, p31b). Changing the wind speeds by adjusting the knob on the electric fan, they concluded that there was a correlation between the wind speed and the visibility of the laminar flow produced. This effect was also integrated into the final exhibit as another variable that could be changed by the people interacting with the exhibit (Content of accompanying poster).
Another change implemented by the team to improve the laminar flow was the relative positions of the fan and the humidifier which produced the water vapor making laminar flow visible. As documented in their logbook (Figures 12, 13), when the students tested out their prototype where the water vapor was sucked into the fan prior to passing through the bundle of straws, they assessed that “No laminar flow observed in acrylic tube. Upon further observation, water vapor was found to have been dissipated in the fan, due to the high wind speed” (Logbook, p29b). They proceeded to change the position of the fan relative to the humidifier, by placing the fan and the humidifier on two separate sides of the bundle of straws and evaluated that “Visible and clear laminar flow observed” and “suction based set-up works better” (Logbook, p29c).
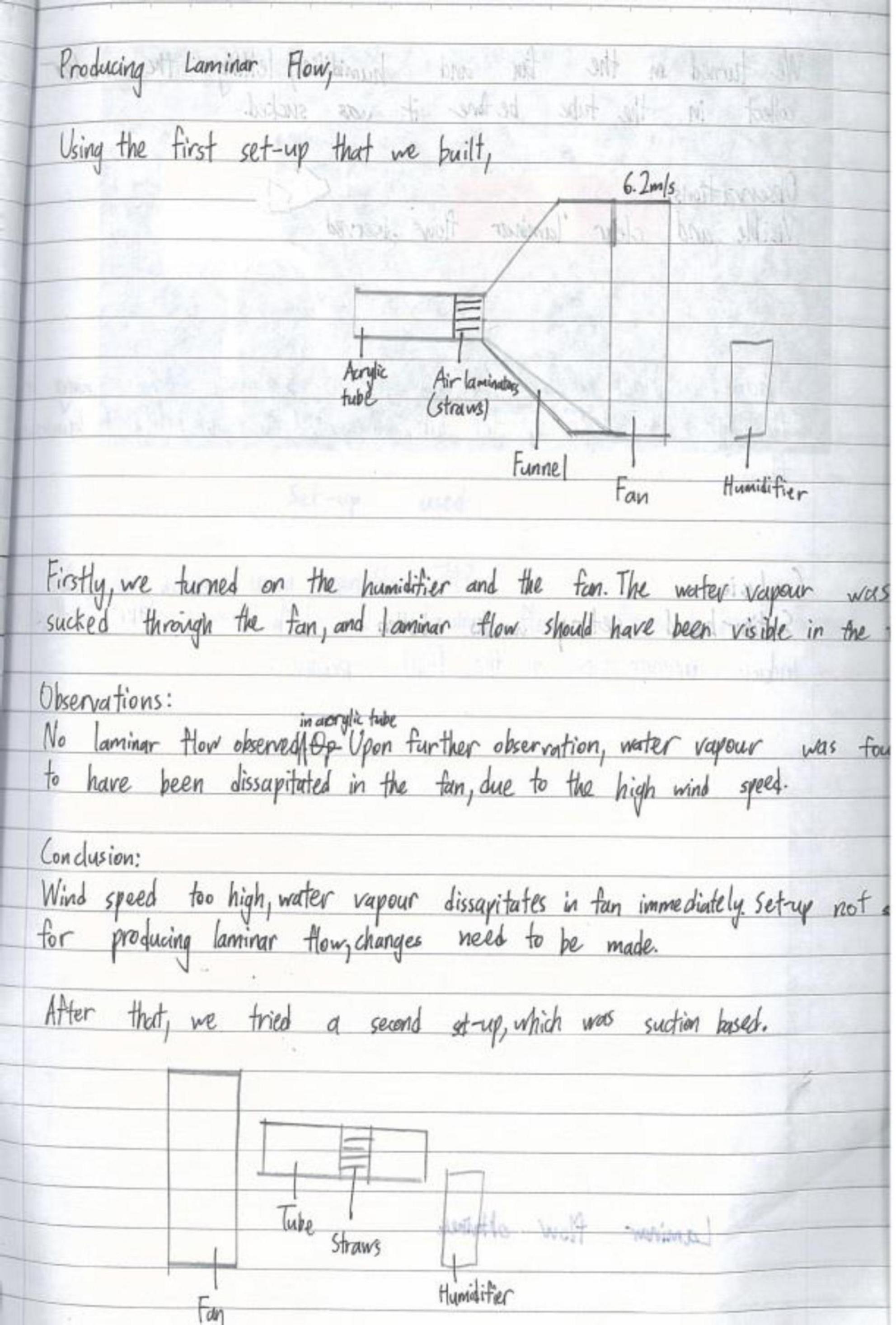
Figure 12. Excerpt from students’ logbook showing trials in which the positions of the fan and humidifier are changed.
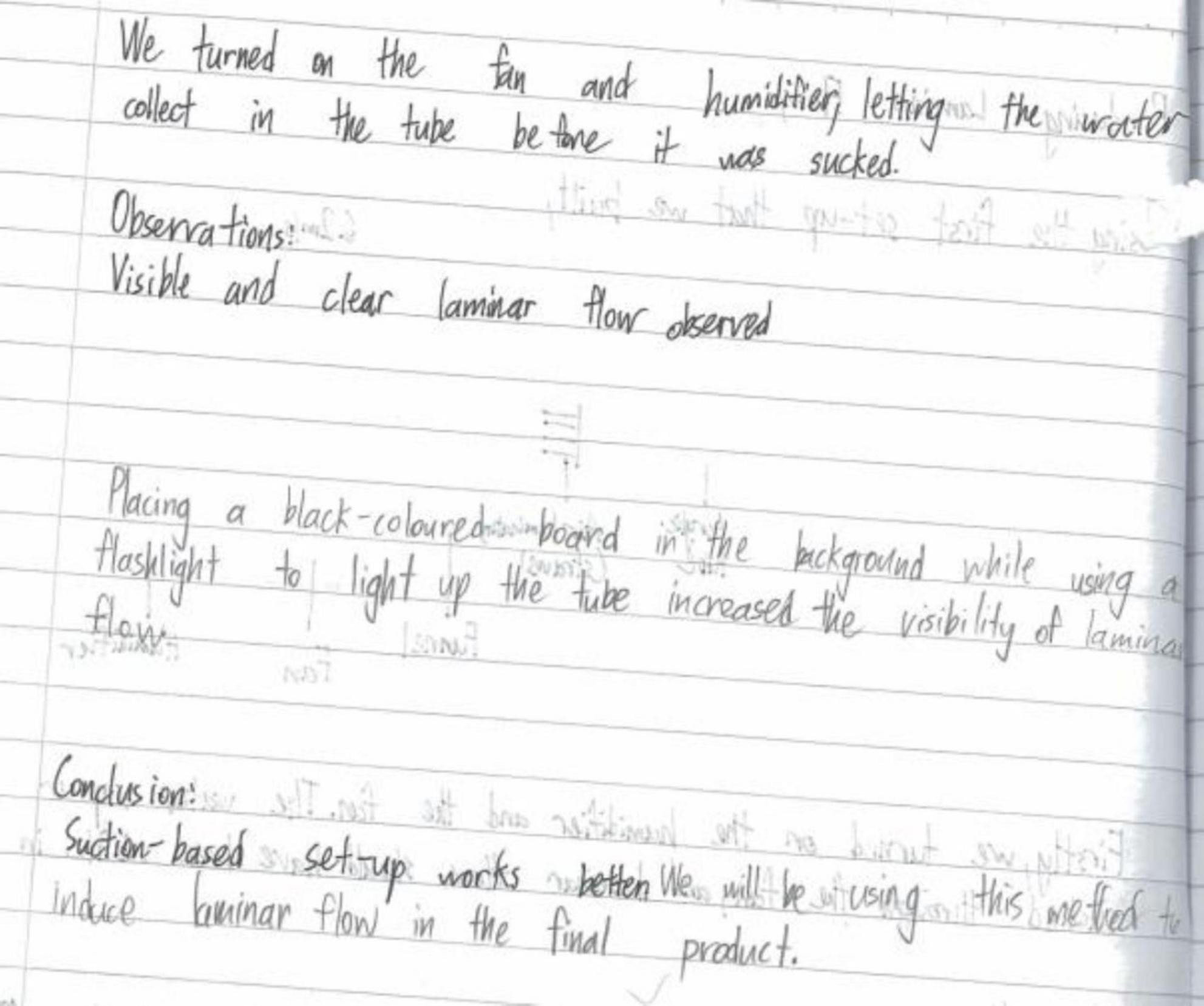
Figure 13. Excerpt from students’ logbook showing trials in which the positions of the fan and humidifier are changed (cont’d).
Another challenge faced by the students was to increase the visibility of the air flow. In one of the entries from the logbook, we found evidence that the students were able to grasp that this was a major issue, and also generated some possible solutions:
An important aspect of the wind tunnel would be to make certain parts of the air visible to human eye, so that the flow of wind can be observed easily by the naked eye. Not doing so would render the exhibit useless. The whole point of the wind tunnel is to showcase and display the flow of air. Some potential ways to make the flow of wind visible is to use smoke-particle sources such as a smoke generator to mix smoke with the air and make it visible. The whole idea is to have visible particles mix with air entering the tunnel, so flow will be visible. Some potential sources: Incense; Smoke Generator; Powder. (Logbook, p19b).
Through discussions with their mentor as well as further investigations and evaluating the experimental results, the students were able to narrow down to a suitable source to enable the air flow of their exhibit to be more conspicuous. The process of eliminating unsuitable sources is documented in their logbook:
After consulting our mentor, we learnt that it was not safe to use smoke sources such as incense or cigarettes as it posed a threat to viewers lest they inhale it. Dry ice was not viable as it was costly. Hence we decided to experiment with baby powder for flow visualization.
Observations: Powder was sucked into the fan. Minimally-visible laminar flow observed in container, powder left as residue in straws. Out of 100% of powder, 50% (rough estimate) were collected in the straws rather blown with the wind to aid flow visualization.
Conclusion: Powder is too heavy a particle and a lot gets collected in the straws. While some laminar flow is visible, it is not an ideal particle for flow visualization. In addition, another problem of powder is the need to repeatedly clear it from the straws. If powder continues to build up, it will clog up the straws and render the setup useless.
Hence we used an alternative.
An ultrasonic humidifier was used in place of the powder. Water vapor was produced, which was then sucked into the fan.
Observations: Visible laminar flow observed. However, some water vapor is observed to have dissipated in the fan.
Conclusions: Result was far better than previous experiment with powder. Ideal for use due to continuous, never-ending flow of water vapor. Can be refilled easily. Obvious issues such as condensation and dissipation in the fan still remain. (Logbook, p29 and 29a).
In addition to using a humidifier to aid the visualization of the air flow, the students also included other improvisations, commenting that “Placing a black-colored board in the background while using a flashlight to light up the tube increased the visibility of laminar flow” (Logbook, p29c). During a later part of their project, they expanded on this idea by making further modifications on their prototype.
As the current streams of air were white in color due to water vapor being found in it, we decided to create a black background of corrugated plastic to contrast with the air so that it is more visible to the viewers. A rubber tubing was attached to the humidifier so that we could direct the position of water vapor to where we wanted it to be. (Logbook, p49).
However, these modifications were not without problems, as the students evaluated that “Despite the black background, the tube reflected some light from the ceiling lights in the lab” and “Also, when we tried to use a torch to illuminate the tunnel from the bottom, there was reflection of light. In many positions, it was hard to see what was going on in the tube due to these two factors” (Logbook, p49a). After some brainstorming, the students managed to innovate further and resolve the complications, mentioning in their logbook entry that “we swapped the plastic tube for a black box made of corrugated plastic, a sturdier material” and “to eliminate the problem of refraction and reflection of light, we decided to put blue LED lights inside the box to contrast with the red wing and white water vapor, so that both will be highly visible” (Logbook, p49b). The students designed a simple circuit to install blue LED lights for their exhibit, electing to use a parallel circuit to optimize the brightness, as well as learning soldering skills in the process (Figure 14).
The third aspect of the challenges facing the team was also acknowledged and addressed early in the project. Although they proposed using straws as an alternative to the traditional honeycomb structures to produce laminar flow, the students were also aware that “as using the straws slow down the flow of air, we need to increase the velocity of air before it entered the straws to ensure that it is not slowed down to the point that air flow is non-existent”, and proceeded to recommend a plausible solution: “Using Bernoulli’s principle, a funnel could be used to increase the velocity of air” (Logbook, p19a).
In line with the multi-faceted challenges and corresponding solutions discussed above, we noticed a range of interpretations in the discussion of engineering-related concepts or activities by each of the three team members. Royston expressed that “The building of the exhibit was chock-full of engineering principles” (Royston, questionnaire), proceeding to discuss the use of the choice of materials in the assembly for each section of the wind tunnel. Interestingly, he also interpreted the different ways to ensure visibility of the airflow in the wind tunnel as a form of technological impact on the project, rather than an engineering-related one.
For example, I had to decide on materials, and used corrugated plastic as it was light and waterproof. I used a funnel to increase the wind speed. For the wing, we used a high-camber model as it maximized the visual effect of the flow. (Royston, questionnaire).
I learnt about the different possible sorts of flow visualization, for example through smoke or fine powder. Both were determined to be hazards, so I substituted them for something with a similar effect that would make the streams of air visible. I used a humidifier to produce water vapor, which would then be sucked into the tunnel and show the streams of air. (Royston, questionnaire).
In contrast, Henry brought up the relationship between the positioning of the fan, the fan speed and the observed laminar flow:
We made a setup to demonstrate the visual effects of laminar flow on a wing of a plane, taking into account that a higher fan speed actually creates a more stable and clear stream of laminar flow and a blowing the wind would create a higher wind speed but less consistent laminar flow while sucking the wind would result in a slower wind speed but more consistent and clear laminar flow. (Henry, questionnaire).
While Zach’s claim that “Our project in its entirety is a product of engineering itself” (Zach, questionnaire) is reminiscent of Royston’s expression above, he also felt that “One of our greatest achievements in Engineering was to make the LED lights which serves to illuminate the exhibit work. We had to design our own circuits and find intrusions in which the LED lights can work to the best of its abilities” (Zach, questionnaire).
When we examined the logbook as well as replies from the students with regards to the correlation between technology and the construction of their exhibit, two prevalent strands emerged. The first idea that was articulated involved the use of a virtual simulator found on the National Aeronautics and Space Administration (NASA) website. In their logbook, it was written that “using a NASA simulator, we found the ideal specifications of our wing”; this wa followed by a screenshot of the webpage showing the parameters (Logbook, p40). Henry reported the use of the simulator as “an example of us using technology as we can freely adjust certain aspects of our aerodynamic wing without having to resort to trial and error in real life” (Henry, questionnaire). Similar views were adopted by Zach, who commented that “…in order to find the optimal conditions of a wing for flight, I went and used a very complex flight stimulator on the NASA website and find plane wings with maximum lift, but which did not exceed our experimental parameters” (Zach, questionnaire).
The utilization of additive manufacturing was another means in which the students managed to incorporate technology into the construction of their exhibit. The school had recently purchased a 3D printer which used polylactic acid (PLA) as the printing filament; this printer was placed in one of the science laboratories and made available for student use. The team realized that employing 3D printing to obtain the wing desired for their exhibit, instead of following their original intention of cutting it out from Styrofoam blocks, would result in a huge savings of time as well as improve accuracy, and noted this in their logbook.
Originally, we thought of using styrofoam to make the aerofoil. We had planned to carve the aerofoil from a styrofoam block. This would be an extremely complicated and hard process as the cutting needs to be very precise. Hence, for convenience sake, we 3D printed it. (Logbook, p41).
The process entailed learning about related 3D printing software for designing the wing; hence the students set upon themselves to gain proficiency in this skill as well.
In order to be able to effectively create an airplane wing that is aerodynamic in nature, I had to familiarize myself with 3D-software to be able to utilize the 3D-printer. (Zach, questionnaire).
I learnt about the various aspects of 3D printing, which was used to create the model wing used in the wind tunnel. I had to learn about 3D modeling, transferring.stl files, and the subsequent 3D printing of the wing. (Royston, questionnaire).
The students recorded in their logbook that “we started working on the aerofoil today to place in our exhibit. Using the 3d-modeling software, 3dsmax, we imported it into the schools’ 3d printer and printed it” (Logbook, p41). Screenshots of the wing being modeled using the 3D software, as well as the final 3D printed wing, were also documented in the logbook (Logbook, p40). Both Royston and Zach unequivocally found the use of the 3D printer to have played a major role in the construction the aerofoil for their exhibit, with Zach citing “We managed to incorporate Technology into our project through our extensive use of the 3D-Printer” (Zach, questionnaire). Thus, for the design and construction of the wing for their exhibit, the students accessed and used computer programs as well as tapped into an emerging technology, both of which they reasoned to be contributions by technology to enable their product to be enhanced.
Although not mentioned by the students in their responses to the questionnaire, we also noted the use of a datalogger in two of the entries in the logbook (Logbook, p29b and p31b); the datalogger was used to measure the velocity of air produced in the prototype of the wind tunnel.
Overall, we observed a consensus amongst the students with regards to their perceived concepts or activities related to science, technology and mathematics. They familiarized themselves with the scientific principles behind aerodynamics and laminar flow, and relied on related mathematical concepts of Reynolds number as well as Bernoulli’s equation to determine the numerical values of several important parameters for the construction of their wind tunnel. The significance of these scientific and mathematical concepts in relation to their exhibit was also evidenced by the entries in the students’ logbook, which contained multiple accounts of these concepts supported by relevant diagrams to underscore the students’ understanding. Using a virtual simulator available on the web as well as acquiring 3D modeling skills empowered them to design and 3D-print a crucial section of their product. While the students were generally in agreement that their project incorporated several elements linked to engineering, they provided diverse examples of what they believed to be these elements, which encompassed integration of suitable materials, judicious placement of various components, and circuit design for lighting into the exhibit. They also recognized that the design-n-build phases of their project was very much engineering-based. All of these instances were illustrated in the logbook and may be viewed as encompassing a representation of the multiple practical challenges that the students had to overcome in the course of constructing the wind tunnel exhibit.
Overall, it can be seen that students harnessed multiple concepts in the four disciplines within STEM in the process of fabricating the exhibit.
RQ3: What effect does the experience have on students’ dispositions towards STEM?
With the evidence obtained from the reflections submitted by the students as well as their responses to the questionnaire, we were able to identify the pre- and post-project dispositions that the students had towards STEM, as well as the contributing factors to these dispositions. They can be divided into a few categories, which we present below.
Apprehensive approach into unfamiliar territory
All three students had no prior experience and were initially hesitant to take on a STEM-related project. Two of the students, Zach and Henry, professed a limited awareness about STEM prior to working on the project, attributing it to a lack of exposure and motivation to find out more.
Before embarking on the project, I did not really think much about the STEM category…I had very little information on this category…(Henry, questionnaire).
Before the project, I had not a clue or cue about what STEM was. I literally had no idea that it stood for Science, Technology, Engineering and Maths…I did not have prior experience with STEM, and was not prompted to learn about it. (Zach, questionnaire).
The above comments are not surprising since a clear and consistent definition of STEM does not seem to be available in the literature. While Zach and Henry were forthcoming about their inadequate knowledge, Royston, who was the leader of the team, admitted that he alone possessed some surface knowledge of what STEM is. He was under the impression that “STEM was a category full of complicated projects” (Royston, questionnaire), which heightened his level of insecurity; the anxiety and fear that Royston has come to associate with undertaking a STEM-related project is accentuated in his comments below:
I was afraid that the entire project would be out of my depth and that I would be unable to handle it. I was under the impression that expert prior knowledge was needed and only top students could handle it. I viewed STEM in the sense that one had to go through years of schooling, get a degree, before being able to enter the field as a job. (Royston, questionnaire).
Royston attributed the misconceptions that he held about STEM to the socializing messages that he perceived from the media. Moreover, the unintentional message conveyed by his father also played a role in shaping his perception.
I had read many articles that talked about STEM competitions, and many winning entries had complicated names which were hard to understand. Also, my father has a degree in mechanical engineering. He has many thick books related to the subject on our bookshelves. This intimidated me further as it seemed that I would have to learn a lot before taking up this project. (Royston, questionnaire).
It is of interest to note that despite attending science and mathematics classes as part of their school curricula, the students had the impression of STEM as a foreign concept. The very fact that both Royston and Henry described STEM as a “category” underscores the fact that for the students, STEM is perceived to be another externally imposed term by the education system and media. There is an apparent dissociation to the subjects that the students studied in school, with little or no connection to daily life, as well as no applied purpose for STEM. In addition, parents and the media wield substantial influence on student interest in STEM, either positively or negatively; the important mediating role played by these two entities to motivate STEM interest in students cannot be understated.
Despite their deficient knowledge or misgivings about STEM, the students did not dismiss outright the opportunity to engage in a STEM-related project and were willing to take on the challenge of fabricating an exhibit. When looking at the narratives of the students, we found that they unequivocally expressed their desire to acquire new learning through the process.
…I decided to give it a try and see how things go. (Henry, questionnaire).
I was attracted by the prospect of creating a Science Exhibit…, and hence participated in the project, hoping to gain more experience in the field of STEM. (Zach, questionnaire).
I wanted to learn more engineering and technology as a whole. I also wanted to build something. Despite it seeming tough, I decided to just give it a try, as it was something new that I had never done before too. (Royston, questionnaire).
The students were attracted by the novelty of STEM and, for Zach, the positive experience relayed to him by senior students who had completed a STEM-related project also helped in reassuring him. This suggests that the attitudes and achievements of peers can be highly influential on students’ motivations for learning, as the self-efficacy of the students and their willingness to take up challenges are enhanced in the presence of a strong support system from their peers.
Building confidence through problem solving and engagement
The team encountered a setback early in their project when they presented their initial idea of an exhibit illustrating acoustic levitation to a panel of science teachers. The teachers felt that such an exhibit, if constructed, would give rise to several safety concerns for the users, and advised the students to revisit the purpose of constructing the exhibit. The students were disappointed but remained undaunted, returning to the drawing board where they brainstormed for ideas and researched on other possible science concepts to showcase, finally settling on the building of a an exhibit on laminar flow, which the panel of teachers supported. As the students busied themselves with the construction of the exhibit, many opportunities arose for them to probe deeply into concepts, search for meaningful connections, grapple with complexity, and engage in risk taking. Zach related that “STEM has placed myself in many a situation whereby I am compelled to make a judgment and make impactful decisions for my project” (Zach, questionnaire). One such area of decision making was on the choice of materials to construct their exhibit, as recounted by Henry:
…(we) were faced with many limitations such as safety measures and item cost management. Nonetheless, we searched for the safest and cheapest possible parts to start building our exhibit. (Henry, reflection).
We had to discuss on the pros and cons of different materials such as our fan and how we were going to show the laminar flow. (Henry, questionnaire).
An active, innovative learning environment was created where the students were not merely imitating a series of physical operations akin to science laboratory sessions in school. Instead, they had to collaboratively work towards the resolution of complex and challenging problems similar to those found in the real world. Furthermore, the students highlighted their engagement in the problem-solving process, with both Royston and Zach recalling the many situations where they had to rethink their opinions and assumptions as part of their “trial and error” journey of discovering different methods to solve the problems by themselves.
Throughout the project, I was constantly adjusting, changing, adding or even removing certain aspects of the project as it progressed. The end product I got was vastly different from my original blueprint. Originally, I had envisioned the tunnel to have 2 sets of straws, a fan at the back, and incense as a flow visualization source. However, I used only 1 set of straws as I realized that 2 sets would slow down the flow a lot. I ended up with a fan at the front as I realized that suction could produce a better flow. Incense was not used as it was determined to be a health hazard to viewers, so a humidifier was used instead. (Royston, questionnaire).
One very important event we had…was actually the placement of the fan…On one hand, we had to ensure that Reynolds number was within laminar range as we had to produce laminar flow in order for visible flow to be produced. On the other hand, it was crucial we had to generate lift. It was only through that that we can effectively show how the air interacts with the airplane wing. If we placed the fan in front of the set up, we would generate substantially more lift (183N) than otherwise, due to increased wind speed. However, we could kiss our laminar flow goodbye, as it is impossible to achieve with the wing rotors. Now, if we placed the fan behind the set-up, we could produce much clearer laminar flow, it was quite nice. However, our lift generated was thus very, very low. We tried out such a configuration, and the air simply breezed past the airplane wing, what we found out later to be called a creeping flow. By adjusting the pipe length at different intervals, we actually could increase the wind speed very greatly. This helped tremendously as it meant our fan can be placed at the back. However, this just created another problem, as the Reynolds number we needed was exceeded, because of the increased speed. We tried out the exhibit as such and we produced unstable turbulent flow. Far from what we wanted. We tried out some other methods, researching on equations, and at a point in time we wanted to use pressure exerted by air on pipe to induce laminar flow. It was only after a while did we trod upon this straw inspiration… Thus, we decided to change an approach and instead made use of trial-and-error to find our desired pipe length, since our pipe diameter is already fixed. After that, we arranged the pipe in a hexagonal shape, to ensure maximum space efficiency. This was actually very useful, because it meant we could exceed the required Reynolds number, 2100, by quite a lot, because the straws acts as a laminator and forces the air into laminar streams. However, there was a limitation to this. When we achieved Reynolds number of 3800, which is speed 3 of the fan, we returned to highly turbulent flow, which surprised us because at 3000, it was very smooth laminar flow. (Zach, reflection).
When challenged to consider alternative perspectives, think deeply about concepts and ideas, and synthesize information, the students demonstrated a flexibility to design and formulate their own creative solutions in dealing with novel situations. By doing so, they began to grow in confidence to acquire and apply new knowledge in their quest to circumvent the challenges that they encountered, and furthermore developed the capability to appraise the viability of their proposed solutions.
Despite encountering difficulties in the course of working on the project, we found a high level of engagement by the students throughout the entire process. When the students realized that STEM is connected to the surrounding world, they began to appreciate the relevance of the activity. The task becomes motivating and fascinating, as the students began to cultivate a personal interest in it. This is illustrated by an excerpt from Royston’s reflection:
To be very honest, it was the first time I had felt so motivated and dedicated to my…project. I always had to look forward to doing the project as I genuinely loved it. I was entirely focused on the project and worked tirelessly on it…. I was able to put more time and effort into an endeavor I found worthwhile. (Royston, reflection).
This intense involvement and active cognitive engagement in non-superficial and stimulating learning is also echoed by Zach, who wrote in his reflection that “I spent days exploring the NASA website, soaking up everything I possibly could, learning about the mechanics of Bernoulli’s ideal gas principle, about the Reynolds Number” (Zach, reflection).
From the above statements, we can surmise that this experience enabled the students to ignite and develop their passion as well as challenged their capabilities and talents, leading them on a pursuit of authentic, meaningful learning.
Developing understanding by reflecting on experience and achievement
As the project progressed, the students learnt to appreciate the rigor associated with undertaking a STEM-related project. Royston grew to comprehend that “the project entailed a heavy workload” and “does not permit the cutting of corners” (Royston, reflection) while Henry realized that “STEM is far more challenging that I think” (Henry, questionnaire). Likewise, Zach admitted that “we had never put in so much effort and time into a…project before” (Zach, questionnaire); he also points out “the sheer amount of effort and investment we put in to complete the project” (Zach, reflection) and “while doing STEM, effort and sacrifice is part and parcel of the package” (Zach, questionnaire). Coupled with the level of engagement shown by the students that was previously discussed, these comments reflect the capacity of the students to set high expectations for themselves, to persist through difficulty and cultivate resilience in the course of meeting their own set targets.
There is an increase in understanding by the students in that not only considerable content knowledge outside of the school syllabus is required but the ability to transfer the newly acquired information quickly to solve a problem is also vital. This is illustrated by the responses given by two of the students:
I did not expect that such a seemingly simple task would actually be very complex, requiring various revisions on the prototype and extensive research. (Royston, reflection).
STEM actually actively requires both intensive research into our topic, and profound understanding of how to make that thing WORK. (Zach, reflection).
Working on this project thus motivated the students to be independent and explore the related topics further as well as fuels their desire to internalize new content and reengage the content over time. Since understanding and transferability of knowledge, rather than purely factual recall, is deemed by the students as important factors which will lead to the success of the project, deep learning was thus encouraged and facilitated.
It was noted earlier that prior to the project, both Zach and Henry confessed to having no knowledge of STEM, while Royston acknowledged that he had some idea but felt overawed even thinking about STEM. After the project, there is evidence that the students displayed a higher level of awareness about STEM. Henry has grown to enjoy interacting with the topic, declaring that “STEM challenges us to think critically and allows us to think as a group to solve problems” and “STEM…is actually quite interesting and exposes students to many events that they may not have been through before” (Henry, questionnaire). In learning to overcome his initial fears and misconceptions about STEM, Royston recognized that “STEM is…a constant journey and process of learning” and “…a STEM project…does not need to be very complicated” (Royston, questionnaire).
In essence, engineering was finding a solution to better overcome a problem. Technology was making it better and easier. Math and Science are the 2 basic foundations to build upon. You do not need to learn and memorise a lot, but rather, gather and absorb information along the way…. This made me realize that STEM is a constant work-in-progress, whereby throughout the project, new things are learnt, and many revisions must be made in order to get a better product. (Royston, questionnaire).
In STEM, I have to take into question the concept of an idea’s practicability. …we would question ourselves constantly whether our decisions are desirable and ideal (Zach, questionnaire).
The students were able to socially construct their understanding of STEM, identify emergent connections and relationships between STEM topics, as well as become aware of the associations between STEM knowledge and their applications in real life. The latter comment by Zach is an indication that the students perceived the relevance of STEM according to how they interpreted its applicability and, in the process, developed a more profound understanding and appreciation of the interdisciplinary STEM concepts embedded in the task.
After the completion of the project, the students articulated their joy and excitement of knowing that they had reached a milestone, with Royston and Henry affirming that they were “very happy with what I/we have achieved” (Royston and Henry, reflections). Royston and Zach also proclaimed exuberantly that “in the end, was it worth it? Definitely.” (Royston, reflection) and “it was 100% worth it” (Zach, questionnaire) respectively. The sense of accomplishment shared by the students after their struggles and successes is exemplified by Zach, who declared that “we felt proud that when our project was subject to questions or criticisms alike, we were able to answer confidently and secure our project” (Zach, questionnaire).
In addition to doing well for their school-based assessment as well as winning an award in the national competition, it was heartening to note that the students were less focused on tangible outcomes but more on the developmental journey. All three boys gave assurance that they would treasure the learning experience, as exemplified by the comments given below:
All in all, it was a very fruitful experience, that I will cherish a lot…. It is valuable knowledge that I know I will value. (Zach reflection).
For a student who had no clue about science exhibits, it was an interesting and enriching experience. Looking back, I feel that the experiences that I had and the things that I learnt while embarking on this project was irreplaceable…(Henry, reflection).
Henry came to the realization that “hard work betrays no one” (Henry, questionnaire) while Zach responded that “I feel that I progressed in terms of my independence and problem-solving skills significantly” (Zach, questionnaire). Hence, for the entire team we could observe an emergent confidence and commitment to personal growth, learning and development.
In summary, we found that initially the students had little knowledge or an incorrect perception of STEM but were enthusiastic in taking on the challenging approach to learning through the construction of the exhibit. The students emphasized their engagement in the collaborative problem-solving process and in their excitement of brainstorming for solutions to solve the challenges that arose. In the course of embarking on their enterprise, the students strengthened both their understanding of STEM concepts related to their exhibit as well as increased their appreciation of STEM. A few of the STEM concepts, which are characteristically abstract and taught independently as separate subjects in the school curricula, became easier to make sense of and visualize when the students perceived a link to the surrounding world. Contrary to their initial perception, they found STEM to be vibrant, exciting and rewarding after the project.
Discussion
The study set out to explore how a group of 9th grade students went about harnessing concepts within the separate disciplines of STEM to fabricate an interactive exhibit on laminar flow. It builds on previous studies on integrated STEM education. Examination of a number of studies in the literature indicate that those that deal with integrated STEM focus on only a few of the disciplines rather than all−for example, Thibaut et al. (2018) and English (2016). There are a few reasons for this. Firstly, it could be due to the nature of the projects explored since not all projects may readily lend themselves to harnessing the four disciplines within STEM. Secondly, school students have generally been taught the subjects of science and mathematics separately rather than in an integrated manner, and this could have caused them to focus more on these disciplines. Thirdly, as engineering and technology are not yet mainstream subjects in many schools, students’ understanding of these subjects are limited and this could have come in the way of working on fully integrated STEM projects. The findings from our study indicate that a context which provides not only for the merging of the four disciplines but also makes intimate connections among them is very important for promoting integrated STEM education in a meaningful manner. However, there was no equitable representations within STEM - science and engineering predominated, with mathematics and technology having lower but acceptable weightings. This is more due to the nature of the project embarked on. In fact, we feel that hybridizing all the four disciplines in an equal manner in the fabrication of the exhibit can be construed as forcing the amalgamation exercise, and this may not reflect the reality of the foundational STEM principles needed for the fabrication of the exhibit. In the present study, the concepts within the respective STEM disciplines that need to be harnessed for the laminar flow exhibit were allowed to emerge naturally using a ground-up approach, and this may well be an indication of the level of integration needed.
To answer the first RQ, the findings show that the different phases of prototyping demonstrate the students’ progress in using STEM to fabricate an exhibit. When interactive STEM activities lead to the production of an artifact or prototype, examination of the literature suggests that there is scant detail, if any, of how students navigated from one phase of prototyping to another. This can provide some indication on the students’ progress in using STEM to fabricate the product. In this study, we made a conscious effort to document what went into the various phases, not only from a STEM viewpoint but also from a process standpoint. Together, these have provided us with useful insights on students’ thinking in the process of prototyping. It has been emphasized that the role of engineering in integrated STEM projects needs enhancement (English, 2016; Moore et al., 2021). It is apparent that the students have gained familiarity with the epistemic practices of engineering (Cunningham and Kelly, 2017) through the process of designing and fabricating a science exhibit. Engineering design and thinking, though unique to its discipline, are also known to provide linkages across the STEM continuum (Bryan et al., 2015; Lucas et al., 2014) provided, of course, the STEM projects are suitably conceptualized. Our study further highlights that engineering design-based STEM integration, where an engineering design catalyzes the learning of all four STEM disciplines concurrently, holds great potential as a pedagogical construct to enable students to develop content mastery and establish core ideas across STEM (Siverling et al., 2019). While other studies have utilized evidence-based reasoning by analyzing student discussions to illuminate how students apply science and mathematics content they had previously learnt to a given engineering design challenge (for example, Mathis et al., 2018), our work has shown that new insights can be gleaned by examining the artifacts and student reflections, in a scenario where the students not just discussed the solution but proceeded to implement their ideas over a period of time. In particular, the iterative process where the students improved their prototypes across stages provided opportunities for them to engage in sense-making (Cunningham and Kelly, 2017; Dalvi and Wendell, 2024). When they encountered situations where their prototypes performed less than optimally, they were prompted to reflect and evaluate critically, as well as seek out and apply new knowledge in carrying out the revisions. Hence, the students’ sense-making abilities are not only supported and developed but also strengthened because of the iterative nature of prototyping. Moreover, we feel that engineering design considerations and STEM content are not mutually exclusive - the latter very much drives the former. The students’ engagement in the engineering design process (Cunningham and Kelly, 2017; Dalvi and Wendell, 2024; Siverling et al., 2019) is evident as we inspected their drawings and prototypes.
To answer the second RQ, the findings indicate that in the process of coming up with a workable prototype of a laminar flow model, students were able to integrate the separate disciplines of STEM in an acceptable manner. The number of predominant STEM concepts that they had to grapple with across the four disciplines was 37. Most of these concepts were not encountered by the students in their school learning but their prior knowledge in science and mathematics was adequate for them to pick up other concepts along the way in these disciplines together with strands of ideas from engineering and technology. As mentioned earlier, more than one STEM discipline can be argued to be present in a number of the concepts in Table 4; also, in the discipline of mathematics, shapes and measurements are involved. What we have presented are the predominant discipline/s for each of the key concepts. Overall, they came up with a working prototype that won an award in a national competition organized by the local science center. A number of researchers emphasize on the need to give equal attention to at least two STEM disciplines when it comes to integrated STEM education – for example, Shahali et al., 2016 and Stump et al., 2016). It is not clear what exactly is meant by ‘equal attention’. Does it mean that the concepts in the two respective disciplines need to be equal in number? If this is the case, then it is going to be difficult when applying this criterion for the project done in this study. It would not be apparent a priori to students on the number of STEM concepts that would be inherent when working on building a prototype since the concepts need to emerge naturally from students’ understanding and not something that can be imposed at the start of the project. Even for the current study, we feel that if another team of students of similar academic ability were to undertake this project, they may well come up with an output where the number of concepts harnessed in the four disciplines can be quite different from what has been found in this study. That is, the choice of project assigned to the students and the competency levels of the students can admit of multiple approaches in designing and building a prototype as well as harnessing multiple concepts across the STEM continuum. While the present study explored the amalgamation of the four disciplines within STEM, what is of interest is that the students were able to see the linkages across disciplines during the processes of navigating boundary crossings (English, 2016) when fabricating the exhibit. This was clear from their logbooks. They recognized that with their prior knowledge of just science and mathematics at the start of the project, there were bound to be challenges in coming up with a working prototype. Thus, they realized the need to harness the synergies provided by other disciplines such as technology and engineering and bringing these to fusion with science and mathematics. The prototypes they developed in the process of harnessing concepts within STEM are testimony to this. In this context, our findings are in line with the work of Mathis et al. (2018), who noted that domain-specific knowledge learned in school settings feature in the design processes for engineering problems as well as those of Siverling et al. (2019), who noted that students would still need to draw on new knowledge as well. All these still operate within the ambit of sense-making by the students. In other words, at the initial phase, it is natural for students to draw on their curriculum-specific domain knowledge, especially in science and mathematics, as a springboard to build their understanding further. Along the way, new knowledge in STEM is acquired. That is, it is more in the initial phase that they get to apply previously learnt knowledge. However, this would not be adequate to bring the project to fruition as they need to leverage new knowledge in the respective STEM disciplines and apply these to fabricate the exhibit satisfactorily. These observations are well in line with the knowledge-building perspective (Scardamalia and Bereiter, 2003; Donna, 2012). The learning across disciplines also comes across clearly when we examined their logbook.
To answer the third RQ, the students’ dispositions towards STEM have changed positively over one semester as a result of the intervention. While at the start of the activity, they held somewhat naïve views about STEM, it was rather positive after the completion of the activity. We attribute this to the interest and learning experiences stimulated by the design-n-build activity leveraging integrated STEM. The activity afforded wholesome experiences in straddling the four disciplines within STEM as well as the satisfaction of having developed a functional prototype on laminar flow, something that might have been perceived to be beyond the capabilities of grade 9 students in the beginning. It might be necessary to sustain engagement with more integrated STEM activities along the way to ensure that enthusiasm is not dampened when they complete their leaving level examinations. If we take their pre-existing levels of STEM disposition to be grade-equivalent across their cohort, then clearly such an intervention affords possibilities for reaching out to other students with a view towards enhancing their STEM dispositions. We have to note that STEM disposition is a multi-faceted construct (Hebebci and Ertuğrul, 2022), and what we have found in this group of students would not represent the full spectrum of attributes such as can be uncovered by administering an instrument like the STEM Semantics Survey (Christensen et al., 2015).
The effectiveness of the approach used in this study can also be traced to the student-centric learning environment (Al-Ansi and Al-Ansi, 2023) in which the study was done. The setting is well suited for students to take control of their learning, and this is consistent with constructivist notions of learning (Baeten et al., 2013). The role of prior knowledge in such endeavors is well recognized as it is the substratum on which new knowledge must be constructed. The collaborative environment in which the project was done is also reminiscent of the socio-constructivist paradigm, which posits that knowledge construction is better facilitated via group discourse where team members get to discuss ideas and negotiate for a common understanding in addressing the challenges spawned by the project. All these were helped by the choice of samples in this study.
The role of the mentor in supervising this project has also provided useful pointers. As mentioned earlier, the students involved in the study were considered to be of high academic ability. They have good foundation in science and mathematics and, by their very nature, possess the requisite skills necessary to pick up new concepts in not only science and mathematics but also the remaining two disciplines with minimum guidance. Though Pearson (2017) has suggested that good support needs to be provided for students to acquire new knowledge and competencies across disciplines, since they may not have the skills necessary to embark on these, we found that in the present study, the nominal level of support provided by the mentor was adequate for the accomplishment of the task objectives by the students. For example, when students encountered difficulties, the mentor provided suggestions to overcome these rather than spoon-feed them with the necessary information. The latter may not be conducive for the development of inquiry thinking, and an approach where students get back to the drawing board and brainstorm for ideas with other team members can provide richer experiences. However, we acknowledge that for students of academic ability lower than that of the samples in this study, the level of mentor support would need to be more than what has been found to be adequate in this study. In a project of this nature, it is essential for the students to take ownership of their learning as much as possible. However, the role of the mentor is still important in guiding the students on the appropriate pathway, acting as a sounding board for ideas, and providing advice on directions when they encounter problems. The need for the mentor to set milestones (Walkington et al., 2020) for the completion of the project cannot be underestimated for otherwise delays and ineffective completion can impoverish the students’ learning experience. Facilitating the learning experience of the students rather than micromanaging them is thus crucial (Walkington et al., 2011). In this context, the following points are noteworthy:
a) The mentor instilled in students a reflective process to evaluate their ideas. For example, when students proposed the use of cigarette or incense smoke to help visualize airflow, the mentor asked students to think about the possible impacts on people operating the exhibit, leading students to realize the potential risks (fire hazard, smoke inhalation, etc.) and subsequently changing to using talcum powder or water vapor instead.
b) The mentor encouraged students to test out their ideas using a rigorous scientific process through data collection and analysis. For example, the group tested out the effects of different straw lengths on the airflow to determine which length was optimal.
Field notes taken by the mentor while supervising the students generally indicated good engagement levels. Support for this assertion can be seen from the following: students were generally focused on the task; there was good interactions among team members during brainstorming sessions as well as design-n-build sessions; enhanced student motivation was discernible when they saw iterated versions of the prototype functioning better; maturing of their science communication practices during group discussions was noteworthy; and satisfaction seen when the students recognized that the level of integrated STEM knowledge needed for a project of this nature, which is generally suitable for students at a higher level, came within their reach. Though the engagement levels cannot be said to be consistent across the duration of the project, these were, overall, considered satisfactory according to observations made by the mentor. This observation is consistent with other studies in the literature – for example, Gasiewski et al. (2012); Hampden-Thompson and Bennett (2013); and Struyf et al. (2019)), which reported on enhanced student engagement levels in STEM learning environments that were student-centric.
The theoretical frameworks used in this study – situated cognition, where the students focused on the task at hand, which is to design and build an interactive exhibit on laminar flow; the socio-constructivist tradition, where students worked as a group to negotiate understanding in the process of building the exhibit; and engineering design, where students worked on designing, building and iterating an interactive exhibit, have been effective in realizing the goals of this study.
In summary, the contributions of this study to the literature are as follows:
1. The project done by the students in this study incorporated the most number of STEM concepts when compared to other studies in the literature.
2. All four disciplines of STEM are interwoven in the project in good measure.
3. It used the medium of interactive exhibit to harness the affordances of integrated STEM education.
Limitations
The study is underpinned by a few limitations. Firstly, it reflects the work of a group of students of high academic ability. Whether students of lower academic ability could work on such a project remains to be seen. Secondly, there are a number of ways in which the exhibit could be fabricated but the approach involving the use of 3D printing for part of the activity may not be commonly available in some schools, though the cost of such equipment has come down drastically in recent years. Thirdly, in view of the nature of the study, there was no formal assessment of students’ knowledge in the four STEM disciplines but only an interpolation from their logbooks, questionnaire, and interactions with mentor. It was felt that a formal assessment of the students’ knowledge in the four disciplines in relation to content encountered in the fabrication of the exhibit could have stressed up the students; however, we have reasons to believe that if a formal test on the key concepts encountered in the four disciplines were set, they would have fared satisfactorily. Fourthly, the final prototype that was fabricated was adjudged to be more than acceptable by the authors though it may lack the aesthetics and other interactivities expected of a typical exhibit at a science center. However, the model constructed by the students would be adequate for the science center, if necessary, to improve on and scale up using a team of designers, exhibit fabricators and scientific staff.
For student engagement levels, only mentor observations were reported. Though these arose from field notes, observer bias cannot be unequivocally ruled out. As the mentor was supervising the project over a period of time and had regular meetings with the students, observer bias was expected to be minimal. Also, observation of engagement levels was made at the group level rather than at the individual level.
Implications
The findings from this study have a few implications. Integrated STEM education is a worthwhile goal to strive for in the school setting in the form of project work, especially at the high school (Grades 7–12) level. Students need some exposure to work on projects that draw on all the separate disciplines within STEM as it is integral to the modern economy. The choice of topic to focus on is very crucial if the objective is to harness a number of concepts within the constituent disciplines of STEM. In this study, the concept of laminar flow proved suitable for exploring the nuances within the STEM disciplines.
When students work in a team with multiple competencies, it is possible to complete the project within a given period of time and acquire rich experiences. More importantly, students get opportunities to tap on their prior knowledge in science and mathematics to not only apply this knowledge but also learn new knowledge in these disciplines. Engineering and technology may be new subjects, but it is possible to pick up basic knowledge in these disciplines in the context of the nature of the project. A reason for the successful completion of the project in this study is that it was done as part of a team, with each member skilled in specific competencies, and there were ample opportunities to negotiate understandings in a socio-constructivist setting. This point has to be kept in mind when grouping students.
The participants in this study were a group of three Grade 9 male students of high academic ability. It would be of interest to investigate if the idea of fabricating a science exhibit would appeal to female students or a group consisting of students of both genders, as well as students across a range of academic abilities. Further studies can help better understand how the nature of such activities could serve to motivate a variety of student populations and increase their interest and knowledge in STEM.
Conclusion
The study set out to challenge a team of students to come up with an interactive exhibit on laminar flow by harnessing as many of the concepts inherent in all the four disciplines within STEM. The students responded to the challenge satisfactorily and surpassed the expectations of the authors, especially given that their age is about 15 years (Grade 9). Most of the predominant STEM concepts identified by the students to get the exhibit working are, not surprisingly, in the realm of science. Engineering, technology and mathematics (in that order) also had adequate representations. Their STEM dispositions also improved as a result of working on this project.
The contributions of this study to the STEM education literature can be summarized as follows:
1. It focused on a hands-on project which required the students to fabricate an interactive exhibit. We note the limited number of studies in the literature that have sought to foster such hands-on experiences in integrated STEM education.
2. The project worked on by the students demonstrated a good number of concepts in all the four constituent disciplines in STEM. We note that most projects involving integrated STEM focus do not focus on the full complement of the disciplines within STEM.
3. The project has been able to foster STEM dispositions among the students to a reasonable extent.
4. It focused on a sample of high ability students. We note that there hardly any studies that have focused on providing hands-on learning experiences on integrated STEM to high ability students.
Data availability statement
The original contributions presented in the study are included in the article, and further inquiries can be directed to the corresponding author.
Ethics statement
The studies involving humans were approved by the Institutional Research Board, Nanyang Technological University. The studies were conducted in accordance with the local legislation and institutional requirements. Written informed consent for participation in this study was provided by the participants’ legal guardians/next of kin. Written informed consent was obtained from the individual(s) for the publication of any potentially identifiable images or data included in this article.
Author contributions
YLKT: Writing – original draft, Writing – review and editing, Conceptualization, Data curation, Investigation, Methodology, Project administration. RS: Writing – original draft, Conceptualization, Data curation, Investigation, Methodology.
Funding
The author(s) declare that no financial support was received for the research, authorship, and/or publication of this article.
Acknowledgments
We gratefully acknowledge the constructive feedback by the two referees. These have helped in the revision of our manuscript.
Conflict of interest
The authors declare that the research was conducted in the absence of any commercial or financial relationships that could be construed as a potential conflict of interest.
The author(s) declared that they were an editorial board member of Frontiers, at the time of submission. This had no impact on the peer review process and the final decision.
Publisher’s note
All claims expressed in this article are solely those of the authors and do not necessarily represent those of their affiliated organizations, or those of the publisher, the editors and the reviewers. Any product that may be evaluated in this article, or claim that may be made by its manufacturer, is not guaranteed or endorsed by the publisher.
References
Al-Ansi, A. M., and Al-Ansi, A. (2023). Enhancing student-centered learning through introducing module for STEM development and assessment. Int. J. STEM Educ. Sustain. 3, 22–27. doi: 10.53889/ijses.v3i1.114
Asghar, A., Ellington, R., Rice, E., Johnson, F., and Prime, G. M. (2012). Supporting STEM education in secondary science contexts. Interdiscip. J. Problem Based Learn. 6, 85–125. doi: 10.7771/1541-5015.1349
Baeten, M., Dochy, F., and Struyven, K. (2013). The effects of different learning environments on students’ motivation for learning and their achievement. Br. J. Educ. Psychol. 83, 484–501. doi: 10.1111/j.2044-8279.2012.02076.x
Baran, M., Baran, M., Karakoyun, F., and Maskan, A. (2021). The influence of project-based STEM (PjbL-STEM) applications on the development of 21st century skills. J. Turk. Sci. Educ. 18, 798–815. doi: 10.36681/tused.2021.104
Barrett, B. S., Moran, A. L., and Woods, J. E. (2014). Meteorology meets engineering: An interdisciplinary STEM module for middle and early secondary school students. Int. J. STEM Educ. 1, 1–6. doi: 10.1186/2196-7822-1-6
Breiner, J. M., Harkness, S. S., Johnson, C. C., and Koehler, C. M. (2012). What is STEM? A discussion about conceptions of STEM in education and partnerships. School Sci. Math. 112, 3–11. doi: 10.1111/j.1949-8594.2011.00109.x
Brown, J. S., Collins, A., and Duguid, P. (1989). Situated cognition and the culture of learning. Educ. Res. 18, 32–42. doi: 10.3102/0013189X018001032
Brush, T., and Saye, J. (2000). Implementation and evaluation of a student-centered learning unit: A case study. Educ. Technol. Res. Dev. 48, 79–100. doi: 10.1007/BF02319859
Bryan, L. A., Moore, T. J., Johnson, C. C., and Roehrig, G. H. (2015). “Integrated STEM education,” in STEM roadmap: A framework for integration, eds C. C. Johnson, E. E. Peters-Burton, and T. J. Moore (London: Taylor & Francis), 23–37. doi: 10.4324/9781315753157-3
Capraro, R. M., Capraro, M. M., and Morgan, J. R. (eds) (2013). STEM project-based learning: An integrated science, technology, engineering, and mathematics (STEM) approach. Berlin: Springer Science & Business Media. doi: 10.1007/978-94-6209-143-6
Carr, R. L., Bennett, I. L. D., and Strobel, J. (2012). Engineering in the K-12 STEM standards of the 50 US states: An analysis of presence and extent. J. Eng. Educ. 101, 539–564. doi: 10.1002/j.2168-9830.2012.tb00061.x
Cheng, Y. C., and So, W. W. M. (2020). Managing STEM learning: A typology and four models of integration. Int. J. Educ. Manage. 34, 1063–1078. doi: 10.1108/IJEM-01-2020-0035
Christensen, R., and Knezek, G. (2017). Relationship of middle school student STEM interest to career intent. J. Educ. Sci. Environ. Health 3, 1–13. doi: 10.21891/jeseh.275649
Christensen, R., Knezek, G., and Tyler-Wood, T. (2015). Alignment of hands-on STEM engagement activities with positive STEM dispositions in secondary school students. J. Sci. Educ. Technol. 24, 898–909. doi: 10.1007/s10956-015-9572-6
Creswell, J. W. (2007). Qualitative inquiry and research design: Choosing among five approaches, 2nd Edn. Thousand Oaks, CA: Sage Publications.
Cunningham, C. M., and Kelly, G. K. (2017). Epistemic practices of engineering for education. Sci. Educ. 101, 486–505. doi: 10.1002/sce.21271
Dalvi, T., and Wendell, K. (2024). Epistemic conflict and sensemaking in elementary students’ navigation of an engineering design task. Sci. Educ. 108, 1051–1071. doi: 10.1002/sce.21865
Donna, J. D. (2012). A model for professional development to promote engineering design as an integrative pedagogy within STEM education. J. Pre Coll. Eng. Educ. Res. 2:2. doi: 10.5703/1288284314866
English, L. D. (2016). STEM education K-12: Perspectives on integration. IJ STEM Ed. 3:3. doi: 10.1186/s40594-016-0036-1
Frykholm, J., and Glasson, G. (2005). Connecting science and mathematics instruction: Pedagogical context knowledge for teachers. Sch. Sci. Maths. 105, 127–141. doi: 10.1111/j.1949-8594.2005.tb18047.x
Gandi, A. S. K., Haryani, S., and Setiawan, D. (2019). The effect of project-based learning integrated STEM toward critical thinking skill. J. Prim. Educ. 8, 18–23.
Gardner, M. A., and Tillotson, J. W. (2020). Explorations of an integrated STEM middle school classroom: Understanding spatial and temporal possibilities for collective teaching. Int. J. Sci. Educ. 42, 1895–1914. doi: 10.1080/09500693.2020.1794078
Gasiewski, J. A., Eagan, M. K., Garcia, G. A., Hurtado, S., and Chang, M. J. (2012). From gatekeeping to engagement: A multicontextual, mixed method study of student academic engagement in introductory STEM courses. Res. High. Educ. 53, 229–261. doi: 10.1007/s11162-011-9247-y
Gentile, L., Caudill, L., Fetea, M., Hill, A., Hoke, K., Lawson, B., et al. (2012). Challenging disciplinary boundaries in the first year: A new introductory integrated science course for STEM majors. J. Coll. Sci. Teach. 41, 44–50.
Guzey, S. S., Moore, T. J., Harwell, M., and Moreno, M. (2016). STEM integration in middle school life science: Student learning and attitudes. J. Sci. Educ. Technol. 25, 550–560. doi: 10.1007/s10956-016-9612-x
Hall, A., and Miro, D. (2016). A study of student engagement in project-based learning across multiple approaches to STEM education programs. Sch. Sci. Maths. 116, 310–319. doi: 10.1111/ssm.12182
Hallström, J., and Ankiewicz, P. (2023). Design as the basis for integrated STEM education: A philosophical framework. Front. Educ. 8:1078313. doi: 10.3389/feduc.2023.1078313
Hampden-Thompson, G., and Bennett, J. (2013). Science teaching and learning activities and students’ engagement in science. Int. J. Sci. Educ. 35, 1325–1343. doi: 10.1080/09500693.2011.608093
Han, J., Kelley, T., and Knowles, J. G. (2023). Building a sustainable model of integrated stem education: Investigating secondary school STEM classes after an integrated STEM project. Int. J. Technol. Design Educ. 33, 1499–1523. doi: 10.1007/s10798-022-09777-8
Han, S., Capraro, R., and Capraro, M. M. (2015). How science, technology, engineering, and mathematics (STEM) project-based learning (PBL) affects high, middle, and low achievers differently: The impact of student factors on achievement. Int. J. Sci. Maths. Educ. 13, 1089–1113. doi: 10.1007/s10763-014-9526-0
Hebebci, M. T., and Ertuğrul, U. S. T. A. (2022). The effects of integrated STEM education practices on problem solving skills, scientific creativity, and critical thinking dispositions. Particip. Educ. Res. 9, 358–379. doi: 10.17275/per.22.143.9.6
Hernandez, P. R., Bodin, R., Elliott, J. W., Ibrahim, B., Rambo-Hernandez, K. E., Chen, T. W., et al. (2014). Connecting the STEM dots: Measuring the effect of an integrated engineering design intervention. Int. J. Technol. Design Educ. 24, 107–120. doi: 10.1007/s10798-013-9241-0
Huri, N. H. D., and Karpudewan, M. (2019). Evaluating the effectiveness of Integrated STEM-lab activities in improving secondary school students’ understanding of electrolysis. Chem. Educ. Res. Pract. 20, 495–508. doi: 10.1039/C9RP00021F
Johnson, C. C., Moore, T. J., and Peters-Burton, E. E. (2021). STEM road map 2.0. London: Routledge.
Kelley, T. R., and Knowles, J. G. (2016). A conceptual framework for integrated STEM education. Int. J. STEM Educ. 3, 1–11. doi: 10.1186/s40594-016-0046-z
Knezek, G., and Christensen, R. (2020). Project-based learning for middle school students monitoring standby power: Replication of impact on stem knowledge and dispositions. Educ. Technol. Res. Dev. 68, 137–162. doi: 10.1007/s11423-019-09674-3
Kokotsaki, D., Menzies, V., and Wiggins, A. (2016). Project-based learning: A review of the literature. Improving Sch. 19, 267–277. doi: 10.1177/136548021665973
Krajcik, J. S., and Blumenfeld, P. C. (2006). “Project-based learning,” in The Cambridge Handbook of the Learning Sciences, ed. R. Keith Sawyer (Cambridge: Cambridge University Press), 317–334. doi: 10.1017/CBO9780511816833.020
Lave, J., and Wenger, E. (1991). Situated learning. Legitimate peripheral participation. Cambridge: Cambridge University Press. doi: 10.1017/CBO9780511815355
Lucas, B., Claxton, G., and Hanson, J. (2014). Thinking like an engineer: Implications for the education system. London: Royal Academy of Engineers.
Mathis, C. A., Siverling, E. A., Moore, T. J., Douglas, K. A., and Guzey, S. S. (2018). Supporting engineering design ideas with science and mathematics: A case study of middle school life science students. Int. J. Educ. Maths. Sci. Technol. 6, 424–442. doi: 10.18404/ijemst.440343
Mihic, M., and Zavrski, I. (2017). Professors’ and students’ perception of the advantages and disadvantages of project based learning. Int. J. Eng. Educ. 33, 1737–1750.
Moore, T. J., Bryan, L. A., Johnson, C. C., and Roehrig, G. H. (2021). “Integrated STEM education,” in STEM road map 2.0: A framework for integrated STEM education in the innovation age, eds C. C. Johnson, E. E. Peters-Burton, and T. J. Moore (Milton Park: Routledge), 25–42. doi: 10.4324/9781003034902-4
Moore, T. J., Guzey, S. S., and Brown, A. (2014). Greenhouse design to increase habitable land: An engineering unit. Sci. Scope 37, 51–57. doi: 10.2505/4/ss14_037_07_51
Moore, T. J., Johnson, C. C., Peters-Burton, E. E., and Guzey, S. S. (2015). “The need for a STEM road map,” in STEM road map: A framework for integrated STEM education, eds C. C. Johnson, E. E. Peters-Burton, and T. J. Moore (New York, NY: Routledge). doi: 10.4324/9781315753157
Nadelson, L. S., and Seifert, A. L. (2017). Integrated STEM defined: Context, challenges, and the future. J. Educ. Res. 110, 221–223. doi: 10.1080/00220671.2017.1289775
National Research Council [NRC] (2012). A framework for K12 science education: Practices, cross cutting concepts, and core ideas. Washington, DC: National Academies Press.
NGSS (2013). Available online at: https://www.nextgenscience.org/ (accessed January 20, 2024).
Ortiz-Revilla, J., Adúriz-Bravo, A., and Greca, I. M. (2020). A framework for epistemological discussion on integrated STEM education. Sci. Educ. 29, 857–880. doi: 10.1007/s11191-020-00131-9
Pearson, G. (2017). National academies piece on integrated STEM. J. Educ. Res. 110, 224–226. doi: 10.1080/00220671.2017.1289781
Phelps, L. A., Camburn, E. M., and Min, S. (2018). Choosing STEM college majors: Exploring the role of pre-college engineering courses. J. Pre Coll. Eng. Educ. Res. 8:1. doi: 10.7771/2157-9288.1146
Putnam, R. T., and Borko, H. (2000). What do new views of knowledge and thinking have to say about research on teacher learning? Educ. Res. 29, 4–15. doi: 10.3102/0013189X029001004
Quang, L. X., Hoang, L. H., Chuan, V. D., Nam, N. H., Anh, N. T. T., and Nhung, V. T. H. (2015). Integrated science, technology, engineering and mathematics (STEM) education through active experience of designing technical toys in Vietnamese schools. arXiv [Preprint]. arXiv:1509.03807. doi: 10.9734/BJESBS/2015/19429
Riskowski, J. L., Todd, C. D., Wee, B., Dark, M., and Harbor, J. (2009). Exploring the effectiveness of an interdisciplinary water resources engineering module in an eighth grade science course. Int. J. Eng. Educ. 25, 181–195.
Sahin, A. (2019). The role of interdisciplinary project-based learning in integrated STEM education: STEM Education 2.0. Leiden: Brill, 93–103. doi: 10.1163/9789004405400_006
Salonen, A., Hartikainen-Ahia, A., Hense, J., Scheersoi, A., and Keinonen, T. (2017). Secondary school students’ perceptions of working life skills in science-related careers. Int. J. Sci. Educ. 39, 1339–1352. doi: 10.1080/09500693.2017.1330575
Scardamalia, M., and Bereiter, C. (2003). Knowledge building: Encyclopedia of education. New York, NY: Macmillan, 1370–1373.
Shahali, E. H. M., Halim, L., Rasul, M. S., Osman, K., and Zulkifeli, M. A. (2016). STEM learning through engineering design: Impact on middle secondary students’ interest towards STEM. EURASIA J. Math. Sci. Technol. Educ. 13, 1189–1211. doi: 10.12973/eurasia.2017.00667a
Siverling, E. A., Suazo-Flores, E., Mathis, C. A., and Moore, T. J. (2019). Students’ use of STEM content in design justifications during engineering design-based STEM integration. Sch. Sci. Maths. 119, 457–474. doi: 10.1111/ssm.12373
Stohlmann, M., Moore, T. J., and Roehrig, G. H. (2012). Considerations for teaching integrated STEM education. J. Pre Coll. Eng. Educ. Res. 2:4. doi: 10.5703/1288284314653
Strimel, G., and Grubbs, M. E. (2016). Positioning technology and engineering education as a key force in STEM education. J. Technol. Educ. 27, 21–36. doi: 10.21061/jte.v27i2.a.2
Struyf, A., Boeve-de Pauw, J., and Van Petegem, P. (2017). ‘Hard science’: A career option for socially and societally interested students? Grade 12 students’ vocational interest gap explored. Int. J. Sci. Educ. 39, 2304–2320. doi: 10.1080/09500693.2017.1376259
Struyf, A., De Loof, H., Boeve-de Pauw, J., and Van Petegem, P. (2019). Students’ engagement in different STEM learning environments: Integrated STEM education as promising practice? Int. J. Sci. Educ. 41, 1387–1407. doi: 10.1080/09500693.2019.1607983
Stump, S. L., Bryan, J. A., and McConnell, T. J. (2016). Making STEM connections. Maths. Teach. 109, 576–583. doi: 10.5951/mathteacher.109.8.0576
Tan, O. S., Low, E. L., Tay, E. G., and Yan, Y. K. (2021). Singapore math and science education innovation. Singapore: Springer. doi: 10.1007/978-981-16-1357-9
Thibaut, L., Ceuppens, S., De Loof, H., De Meester, J., Goovaerts, L., Struyf, A., et al. (2018). Integrated STEM education: A systematic review of instructional practices in secondary education. Eur. J. STEM Educ. 3:2.
Tseng, K. H., Chang, C. C., Lou, S. J., and Chen, W. P. (2013). Attitudes towards science, technology, engineering and mathematics (STEM) in a project-based learning (PjBL) environment. Int. J. Technol. Design Educ. 23, 87–102. doi: 10.1007/s10798-011-9160-x
Tzuriel, D., and Tzuriel, D. (2021). “The socio-cultural theory of vygotsky,” in The socio-cultural theory of vygotsky, ed. D. Tzuriel (Cham: Springer), 53–66. doi: 10.1007/978-3-030-75692-5_3
Walkington, C., Nathan, M., Wolfgram, M., Alibali, M., and Srisurichan, R. (2011). “Bridges and barriers to constructing conceptual cohesion across modalities and temporalities: Challenges of STEM integration in the precollege engineering classroom,” in Engineering in Pre-college settings: Synthesizing research, policy, and practices, eds S. Purzer, J. Strobel, and M. E. Cardella (West Lafayette, IN: Purdue University Press), 183–210. doi: 10.2307/j.ctt6wq7bh.13
Keywords: integrated STEM education, prototype fabrication, exhibit, STEM dispositions, laminar flow
Citation: Tan YLK and Subramaniam R (2024) Promoting integrated STEM education among students via fabrication of interactive exhibit. Front. Educ. 9:1423158. doi: 10.3389/feduc.2024.1423158
Received: 25 April 2024; Accepted: 03 October 2024;
Published: 18 November 2024.
Edited by:
Ahmet Erol, Pamukkale University, TürkiyeReviewed by:
Tej Dalvi, University of Massachusetts Boston, United StatesWahyu Widada, University of Bengkulu, Indonesia
Copyright © 2024 Tan and Subramaniam. This is an open-access article distributed under the terms of the Creative Commons Attribution License (CC BY). The use, distribution or reproduction in other forums is permitted, provided the original author(s) and the copyright owner(s) are credited and that the original publication in this journal is cited, in accordance with accepted academic practice. No use, distribution or reproduction is permitted which does not comply with these terms.
*Correspondence: R. Subramaniam, cnN1YnJhbWFuaWFtMzU4QGdtYWlsLmNvbQ==