- 1Teaching and Learning Center, University of Insubria, Varese, Italy
- 2Department of Biotechnology and Life Sciences, University of Insubria, Varese, Italy
- 3Department of Human Sciences and Sciences of the Innovation for the Territory, University of Insubria, Varese, Italy
Immersive virtual reality (VR) simulations are increasingly being used in diverse educational and training contexts to supplement traditional learning methods. The high versatility of virtual laboratories allows students to take advantage of many benefits, like experiencing dangerous reactions, time-consuming protocols, or expensive equipment without the necessity of a real science laboratory. However, little research is currently available to support the efficacy and efficiency of this new learning tool. In this context, the main objective of this study was to assess the influence of biotechnology training by using immersive VR technologies on the student’s motivational and learning outcomes as compared with learning with conventional methods only. To this aim we tested two diverse strategies, respectively VR simulations were used in place of or in addition to the teacher’s introductory lesson of a hands-on laboratory experience. Aligned questionaries were administered before and after the proposed activities to assess theoretical knowledge, self-efficacy, interest in biotechnology, and engagement of the participants. We found that when the introductory lesson to a biotechnology hands-on laboratory is replaced with an equivalent immersive VR simulation, the student’s learning outcomes are lower with respect to the traditional approach. On the contrary, when VR simulations are integrated as an additional tool to the existing learning methods, higher learning outcomes were observed demonstrating a deeper understanding of the learning contents. Furthermore, our study showed that learning with immersive VR simulations motivates students more than the traditional methods, thus, using this new technology in addition to the existing educational methods in biotechnology could be considered as a win-win strategy to raise the attention of the students while increasing the learning outcomes.
1 Introduction
Educational institutions have found in technology a variety of innovative solutions to solve problems such as the inability to conduct in-person classes or perform hands-on laboratory experiences during the COVID-19 pandemic, boosting the development of new learning approaches. Consequently, virtual learning laboratory simulations are increasingly being used in diverse educational and training contexts to supplement traditional educational methods (Wismer et al., 2021). The high versatility of virtual learning allows students to take advantage of its benefits in many different fields, from chemistry (Jumbri and Ishak, 2022) to engineering (Schnieder et al., 2022), passing through medicine (Carnevale et al., 2022) and biotechnology (Bonde et al., 2014). Previous research has shown that virtual learning simulations provide important educational benefits such as cost-effective access to state-of-the-art training equipment and learning tools, beyond what many teaching institutions would be able to provide (Thisgaard and Makransky, 2017). Furthermore, virtual simulations make it possible for students to engage in realistic scenarios that may not be possible to experience in real life because they may be too dangerous, time-consuming, or expensive (Thisgaard and Makransky, 2017), allowing the students to engage in trials and errors (Eastwood and Sadler, 2013). Importantly, the laboratory experience is not limited to the availability of an equipped laboratory, as virtual learning can be located in a variety of other spaces (Makransky et al., 2016). The requirement for laboratory work shifts from being location-dependent to device-dependent (Soliman et al., 2021), allowing the possibility to practice even at home (Makransky et al., 2019b). Distance learners with their own headsets could benefit from being able to experience the same level of education as students on campus when unable to attend the hands-on laboratory, as in the case of social distancing periods imposed by a pandemic outbreak (Soliman et al., 2021).
While it is true that desktop virtual reality (VR) laboratory simulations provide visual experiences and learning, they do not replicate the process of handling equipment and materials. In this context, immersive VR laboratory simulations, accessed through head-mounted displays, are predicted to further raise the bar of virtual learning allowing for realistic sensations of grabbing, interacting, and moving objects around, just as in a real laboratory (Bodekaer, 2016). As a consequence of the price drop of VR devices, many companies and educational institutions are investing significant resources in developing new VR educational tools, with the expectation that a higher level of immersion will increase student motivation (Makransky et al., 2019a), interest in science (Makransky et al., 2020), and learning (Makransky et al., 2019c). Consequently, the field of virtual learning is rapidly developing, and little research is currently available to support the efficacy and efficiency of these new virtual and highly immersive learning tools, especially in the field of biotechnology. Among the available literature, there is agreement in stating that immersive virtual reality can increase interest in science, self-efficacy, enjoyment, intrinsic motivation, and engagement (Parong and Mayer, 2018; Makransky et al., 2020; Sviridova et al., 2023). On the contrary, the results regarding learning outcomes are contradictory. Some studies found that immersive VR technologies are equivalent in conveying basic knowledge when compared to conventional learning methods (Makransky et al., 2019a; Sviridova et al., 2023), while other authors found that students who joined the VR approach in place of the conventional method performed worse than the control group (Parong and Mayer, 2018; Makransky et al., 2019c). The latter suggested that this outcome was due to the significant presence of non-essential visual stimuli in VR simulations, which distracted the learner from the essential content and increased the overall cognitive load (Makransky et al., 2019c; Chan et al., 2021). Furthermore, we found a lack of data concerning the use of VR simulation in addition to the conventional methods in biotechnology teaching, instead of using it in place of the existing methods.
To test these two diverse strategies to improve students’ learning outcomes with immersive VR technologies, we performed two independent studies, respectively, introducing VR simulations in place of or in addition to the teacher’s introductory lesson to a hands-on laboratory experience. Theoretical knowledge, self-efficacy, interest in biotechnology, and engagement were assessed with dedicated questionaries before and after the proposed activities. Based on the currently available literature, our two-fold hypothesis was that (i) using immersive VR simulations in place of the traditional face-to-face lesson could increase the students’ motivational outcomes like interest in biotechnology, self-efficacy and engagement while not affecting the learning outcomes and (ii) using immersive VR simulations in addition to the traditional lesson could allow obtaining both higher motivational outcomes and higher learning outcomes.
2 Materials and methods
2.1 Participants
In Study 1 the sample consisted of 92 students from 4 different high schools in north-western Italy (Varese, Lombardy region). Biotechnology was the main focus of each school’s scholastic program. Among the participants, 2% of the students were attending their second year, 60% in their third year, 24% in their fourth year, and 14% in their fifth year of high school. The average age was 17.6 years (SD = 0.96), 67% were females and 33% were males. Half of the students had already dealt in class with the arguments addressed during the present study, while only 31% had already done something similar in a hands-on laboratory during their studies. Among the participants who used the VR simulations, 23.1% had already used VR headsets before, 10.3% declared to have only little experience with them, and 66.7% had never used a VR headset before. The study took place as part of the educational guidance program of the University of Insubria (Varese – Italy) for local high school students.
In Study 2 the sample consisted of 94 students from the Organic Chemistry course in the first year of the Biological Sciences B.S. degree at the University of Insubria (Varese – Italy). Among the participants, 64% were females and 36% were males, with an average age of 20.2 years (SD = 0.90). In their previous studies, 34% of the students had already done chemistry experience in a laboratory while the other 66% had no or little experience with a chemistry laboratory. Regarding the specific argument addressed in this study, i.e., caffeine extraction, 57% of students had already dealt with it during previous studies while only 13% had already performed this experience in a hands-on laboratory. Among the participants who used the VR headsets, 25.0% had already used them before, 8.3% declared to have only little experience with them, and 66.7% had never used a VR headset before.
2.2 Procedure and questionnaire administering
In Study 1 the participants performed the laboratory activity in groups of 10–20 students, according to the class size.
(1) All participants received oral information describing the experiments and the aim of the research and were asked to compile the Pre-test using personal smartphones.
(2) The class was randomly split into two groups to follow an introductory lesson with two different approaches (Figure 1-Study1):
i. The traditional group (control) received a 1-h conventional lesson from a university teacher consisting of an oral speech supported by a slideshow. The lessons included a complete overview of the equipment and reagents that will be used in the subsequent hands-on part of the laboratory as well as the theoretical concepts necessary to understand the procedures. At the end of the lesson, students were asked to compile the Mid-test followed by a short break.
ii. The virtual reality group used the VR laboratory simulation in place of the conventional lesson from the university teacher. This group followed a short introduction on how to use VR headsets and performed a 10-min tutorial followed by the two virtual laboratories of plasmid DNA extraction (25 min.) and Polymerase Chain Reaction (20 min.). The VR laboratory simulations provided a comprehensive overview of the equipment and reagents that will be utilized in the following hands-on part of the laboratory, along with the theoretical concepts essential for comprehending the procedures. Further details about the VR laboratory simulations are presented in paragraph 2.3. At the end of the simulation, students were asked to compile the Mid-test followed by a short break.
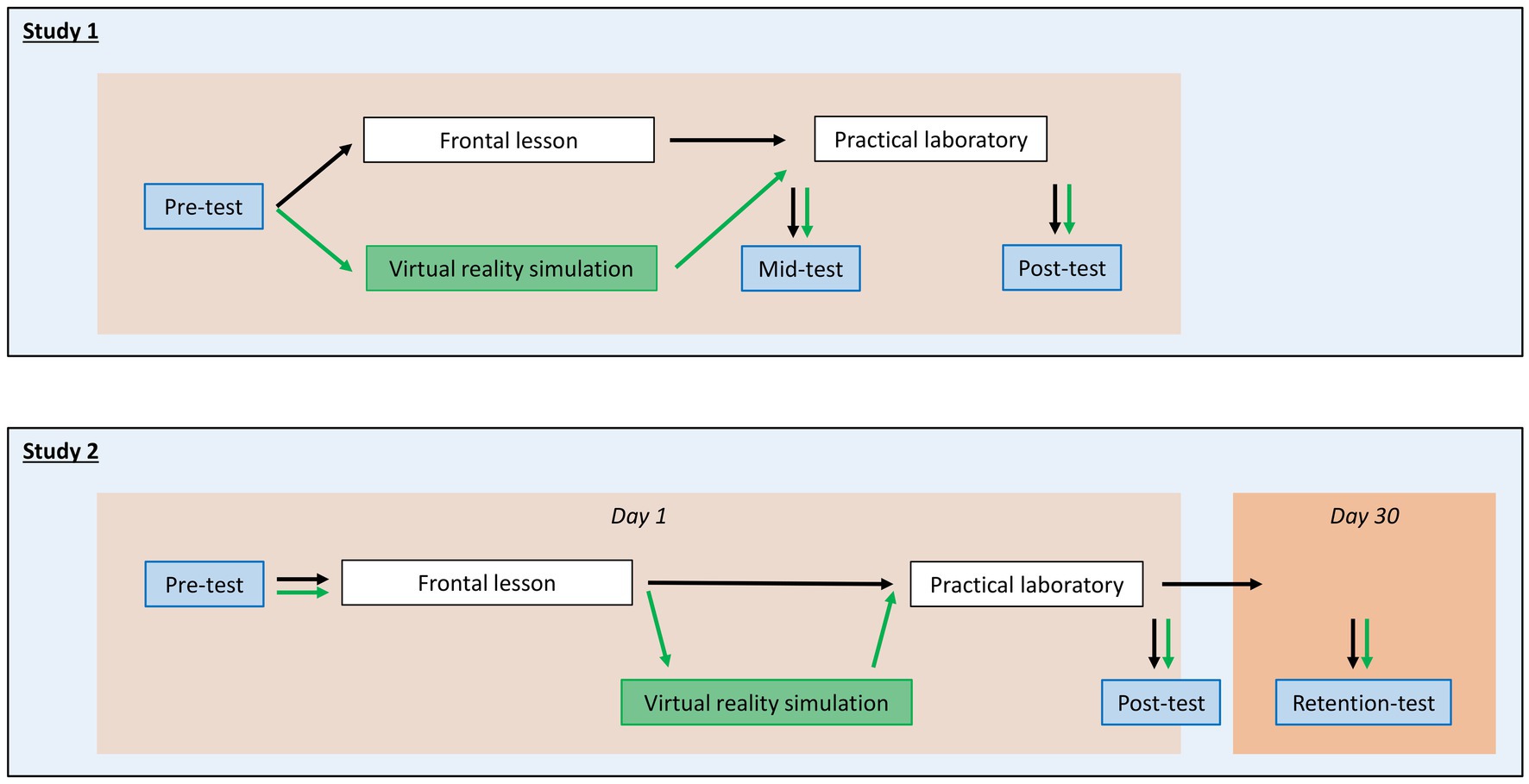
Figure 1. Flow charts illustrating the experimental setup of the 2 studies. In Study 1 VR simulations were used in place of the teacher’s introductory lesson of a hands-on laboratory experience, while in Study 2 VR simulations were used in addition to the teacher’s lesson. Green arrows represent the tasks carried out by the virtual reality group, while black arrows represent the tasks carried out by the traditional group (control).
(3) In the second part of the laboratory, the two groups joined together for the hands-on part (3 h). Supervised by two tutors and the teacher, the participants received step-by-step instructions to perform a plasmid DNA extraction using the ZymoPURE™ Plasmid Miniprep Kit (Zymo Research) and DNA quantification using the NanoDrop™ One spectrophotometer (Thermo Scientific), followed by the setup of a polymerase chain reaction (PCR) and gel electrophoresis. The students learned how to use the molecular biology laboratory equipment (like micropipettes, centrifuge, and thermal cycler) and the specific reagents (like buffers, enzymes, and primers). To understand how each step of the procedure works, the functioning of each specific reagent and instrument was explained while proceeding with the laboratory experience. Immediately after finishing the hands-on laboratory, students were asked to compile the Post-test.
In Study 2 the participants were split into 5 turns attended by approximately 20 participants each.
(1) The conventional introductory lesson was taught once for all participants during a lesson of the Organic Chemistry course, approximately 1 week before the hands-on laboratory. All participants received oral information describing the aim of the research and were asked to compile the Pre-test using personal smartphones. Subsequently, the teacher provided a complete overview of the techniques and instruments that will be used during the hands-on laboratory as well as the theoretical concepts necessary to understand the applied procedures via a 1.5-h conventional lesson consisting of an oral speech supported by a slideshow.
(2) The hands-on laboratory consisted of standard caffeine extraction from coffee with organic solvents and lasted approximately 3 h. The students learned how to use the organic chemistry laboratory equipment (like funnels, vacuum flasks, and the rotavapor) and the specific solvents (like sodium hydroxide, methylene chloride, anhydrous sodium sulfate, and acetone). To understand how each step of the procedure works, the functioning of each specific reagent and instrument was explained while proceeding with the laboratory experience. At this stage, two different approaches were used (Figure 1-Study 2):
i. The participants in the first two turns (traditional group) directly performed the hands-on laboratory, as was usually done the previous years. After finishing the hands-on laboratory, the participants were asked to compile the Post-test.
ii. The participants in the other three turns (virtual reality group) performed a VR laboratory simulation before the hands-on laboratory and in addition to the traditional program. These participants received a short introduction on how to use VR headsets and performed a 10-min tutorial followed by the caffeine extraction VR laboratory (30 min.). The VR laboratory simulation included a complete overview of the equipment and solvents that will be used in the subsequent hands-on part of the laboratory as well as the theoretical concepts necessary to understand the procedures. Further details about the VR laboratory simulations are presented in paragraph 2.3. After a short break, the participants continued with the hands-on laboratory (3 h) as was done by the traditional group (control). After finishing the hands-on laboratory, the participants were asked to compile the Post-test.
(3) During a lesson in the Organic Chemistry course, 30 days after the hands-on experience, a retention test (Ret-test) was provided to the participants.
2.3 VR laboratory simulations
The immersive virtual reality laboratory simulations used in these studies were developed by OpenLab S.R.L. (Peruzzo et al., 2023) and administered through the Oculus Quest 2 headsets (Meta). The virtual laboratory simulations are set in a realistic laboratory (Figure 2A) and utilize hand-tracking technologies to allow realistic interactions with pipettes, solutions, and instruments just as in a real laboratory (Figures 2B,C). A voice guide provides step-by-step instructions and information throughout the simulation flow to guide the student in the laboratory experience and provides the theoretical background necessary to understand each step of the procedures. The students experience in an immersive and realistic virtual environment the use of all the equipment, reagents, and solvents that will be used in the subsequent hands-on part of the laboratory. Specific virtual supplements allow the students to deepen their knowledge of difficult theoretical concepts through full immersion in the reaction solution (Figure 2D). In Study 1, we used the plasmid DNA extraction (25 min) and the Polymerase Chain Reaction (20 min) laboratory simulations, while in Study 2, the caffeine extraction (30 min) simulation was used. Each virtual experience was designed to perfectly match the hands-on experience proposed in the subsequent real laboratory experience. The simulations were supplied in Italian to accommodate the student’s language abilities.
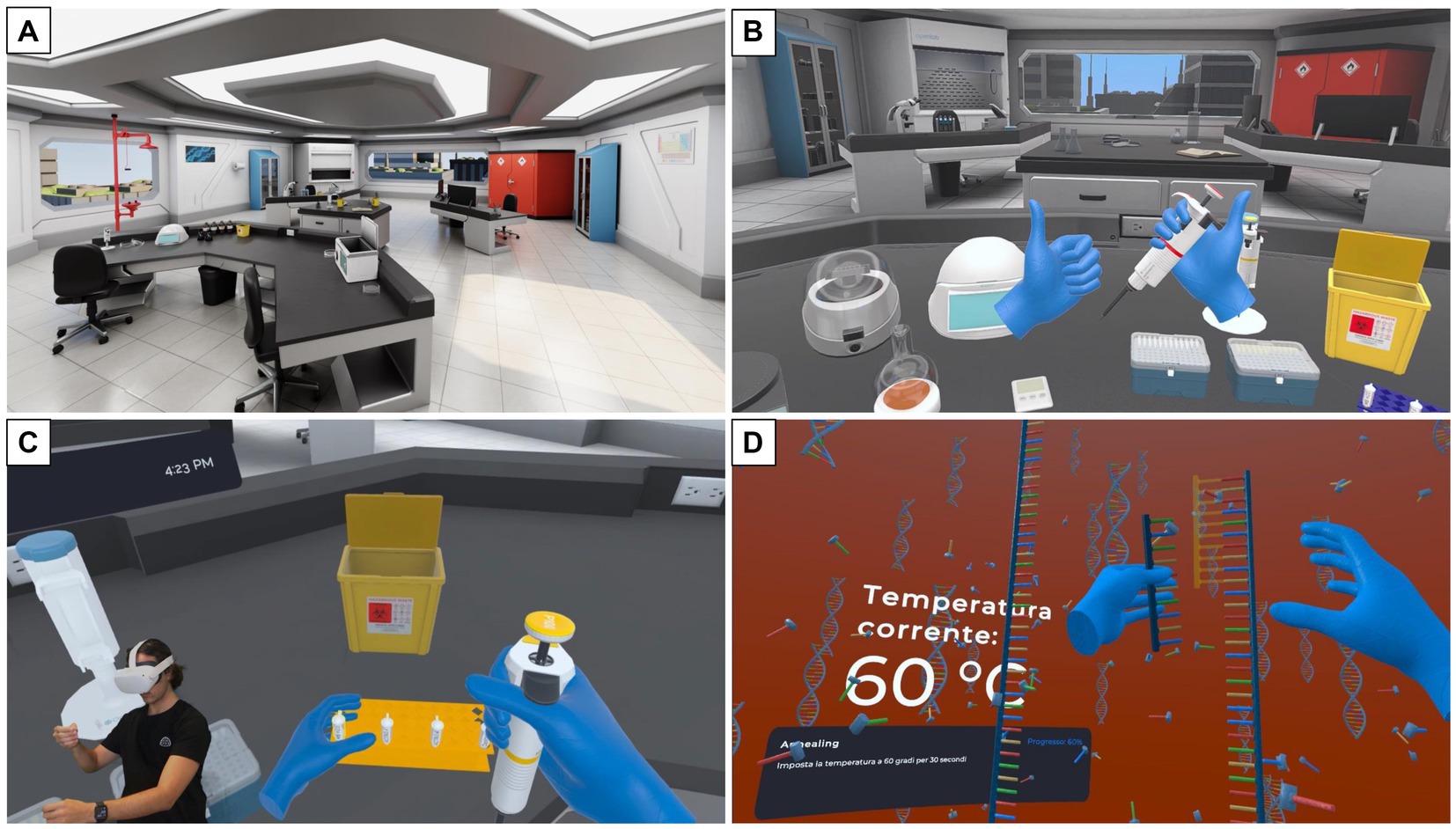
Figure 2. Demonstrative screenshots from the immersive virtual reality laboratory simulations tested in this study. Respectively (A) the lab where the experiences are set, (B) a step of the plasmid DNA extraction experience, (C) an example of the hand-tracking technology from the PCR experience, and (D) a full immersion in the polymerase chain reaction solution.
2.4 Outcomes measure and statistical analysis
The tests of both studies were anonymous and contained a short demographic survey with items concerning age, gender, school grade, experience with the topics covered by the laboratories, and experience with VR headsets. These data have been used to assess if differences in these factors can affect the students’ learning outcomes when studying with VR laboratory simulations. In line with previous research regarding virtual learning simulations (Makransky et al., 2016; Thisgaard and Makransky, 2017), the learning outcome was assessed by measuring the students’ theoretical knowledge via 10 multiple-choice questions aligned with the laboratory contents. The knowledge questions addressed the use of the main reagents and equipment taught in the traditional lesson, experienced in the VR laboratory simulations, and used in the hands-on part of the laboratory, as well as the theoretical concepts necessary to understand the procedures. To prevent overalignment, the questions addressed only the information that was supplied in both the traditional lessons, the VR laboratory simulations, and the hands-on laboratory. A 5-point Likert scale from 1 (very low) to 5 (very high) (Klingenberg et al., 2023) was used to measure interest in biotechnology (3 items) and self-efficacy (5 items). The items were adapted from the Interest/Enjoyment scale from the Intrinsic Motivation Inventory (Center for Self-Determination Theory; Deci et al., 1994) and the Motivated Strategies for Learning Questionnaire Manual (Pintrich et al., 1991). Further questions, adapted from previous research regarding virtual learning simulations (Makransky et al., 2019a), investigated the participants’ perceived learning, engagement, opinions about the VR laboratories, and the laboratory experience in general. The students could access the specific test by scanning a QR code with their personal smartphones. All questions were presented via Google Forms (Google LLC) and administered in Italian to accommodate the student’s language abilities. Further details and the translated questions can be found in Supplementary Tables S1, S2. The statistical analysis was carried out with SPSS Statistics 25.0 (IBM) using the post hoc Dunnett’s test for multiple comparisons of data with unequal variances and the independent samples Student’s t-test when only two means were compared. Statistically significant differences (p < 0.05) between the means were marked with the letters a, b, and c for the traditional group (control), with the letters x, y, and z for the VR group, and with an asterisk for comparisons between the two types of didactic approaches. All presented data are summarized in Supplementary Tables S3–S6.
3 Results
3.1 Study 1: virtual reality laboratory simulation in place of the traditional introductory lesson
The participants in Study 1 were prepared for a biotechnology hands-on laboratory with an introductory lesson performed with two different approaches. The participants who attended the traditional lesson, consisting of an oral speech supported by a slideshow, reported a statistically significant increase in their theoretical knowledge, from 3.0 (Pre-test) up to 6.0 (Mid-test) correct answers out of 10 questions (Figure 3A). With the subsequent hands-on laboratory, no further knowledge increase was observed (Figure 3A). Diversely, the participants who attended the virtual reality (VR) laboratory simulations reported a slight knowledge increase, not statistically significant, from 3.2 (Pre-test) to 3.7 (Mid-test) correct answers (Figure 3A). With the subsequent hands-on laboratory, a further slight knowledge increase was observed (4.75 correct answers), statistically higher with respect to their initial knowledge (Pre-test) but not significant with respect to their intermediate knowledge (Mid-test). When the two didactical approaches were compared, no differences were observed between the two groups at the beginning of the study (Pre-test), while a significantly higher theoretical knowledge level was observed in both Mid- and Post-test in the participants who attended the traditional lesson with respect to the participants who attended the VR simulations (Figure 3A).
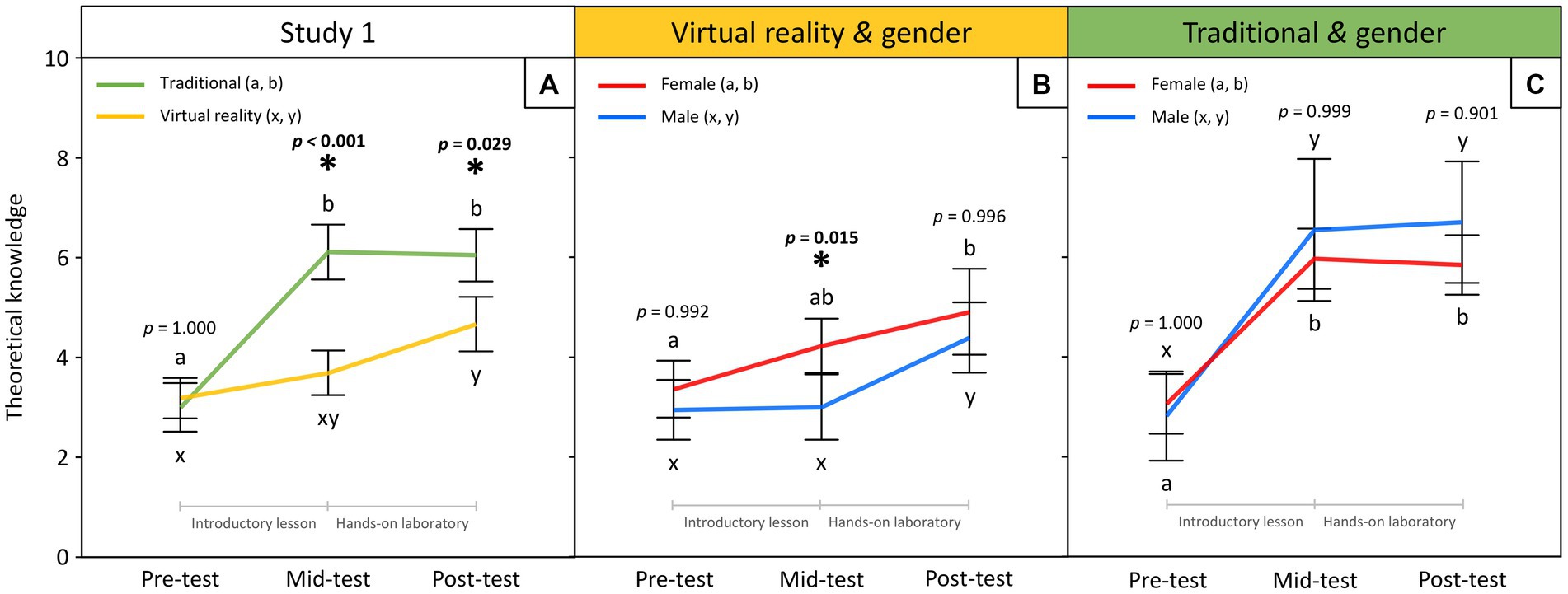
Figure 3. (A) Change in theoretical knowledge with the progress of the laboratory experience for the two groups of participants to Study 1. In panels (B,C), the data of the virtual reality and traditional groups are analyzed singularly to highlight gender differences. Error bars represent the 95% confidence interval, while asterisks indicate statistically significant differences between the two groups.
The statistical analysis showed that in the Mid-test female students have assimilated significantly more information than males from the VR simulations (Figure 3B). On the contrary, no differences between males and females were observed in participants who attended the traditional introductory lesson (Figure 3C). No other differences in the students’ learning outcomes were found when the other demographic factors were analyzed separately (data not shown).
Figure 4 shows the responses to questions aimed at measuring the participants’ interest in biotechnology (Figures 4A–C) and self-efficacy (Figures 4D–F). No statistically significant differences were observed in the participants’ interest in biotechnology, both with the progress of the laboratory experience and between the two participants’ groups, i.e., Traditional and VR (Figures 4A–C). A similar result was also observed for part of the self-efficacy questions (Figures 4D,E). On the contrary, statistically significant differences were found in the student’s perceived ability to explain to a classmate the laboratory procedures, i.e., DNA extraction and Polymerase Chain Reaction (Figure 4F). We observed a significant increase with the progress of the laboratory experience, but no significant differences were observed between the two groups of participants (Figure 4F).
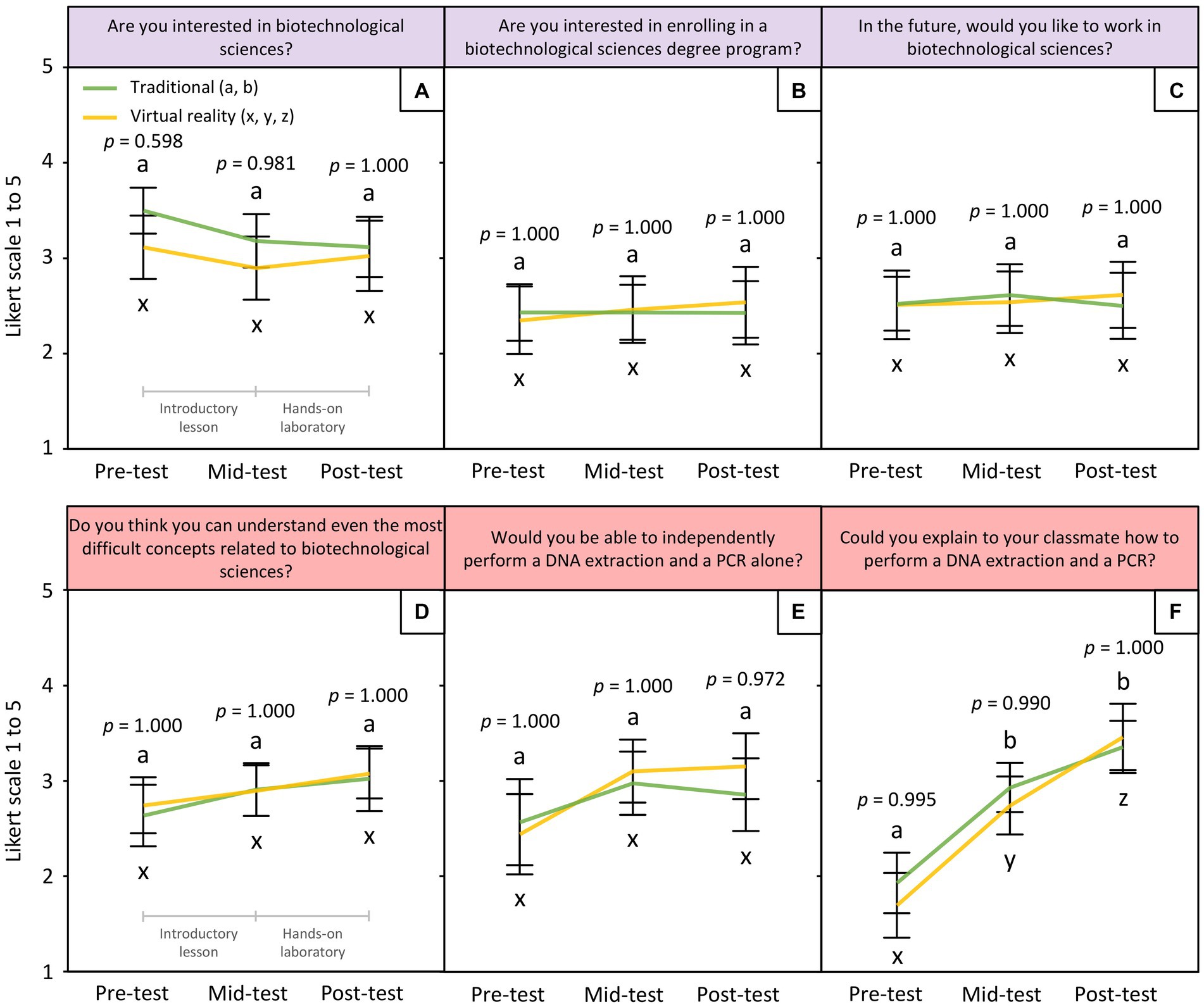
Figure 4. Change in the responses to questions related to (A-C) interest in biotechnology (purple) and (D-F) self-efficacy (red) with the progress of the laboratory experience and for the two groups of participants. Measurements were done through a 5-point Likert scale spanning from 1 (very low) to 5 (very high). Error bars represent the 95% confidence interval.
Further questions were administered both in the Mid- and Post-test to assess the participants’ perception regarding different aspects of the laboratory experience (Figure 5). We observed that the students who joined the traditional lesson felt to have better understood the concepts that were explained to them with respect to the students who attended the VR simulation (Figure 5A). On the contrary, the students who attended the VR simulation reported a higher enjoyment (Figure 5D) and felt that the introductory lesson was less boring (Figure 5E) with respect to the students who attended the traditional lesson. Furthermore, the students who joined the traditional lesson scored significantly higher ratings when asked if the concepts necessary to understand the hands-on experience were clear (Figure 5H). No statistically significant differences were observed regarding the other 5 items tested, respectively the difficulty in following the lesson, the difficulty of the subject itself, the usefulness of the lesson for their school career, their pleasure in learning new biotechnology concepts, and the usefulness of the lesson before doing the hands-on laboratory (Figures 5B,C,F,G,I). Among the participants who used the VR headsets, 89.7% of the students think that immersive VR should be used more often for school learning, while 10.3% of the students responded negatively to this question (Figure 5J).
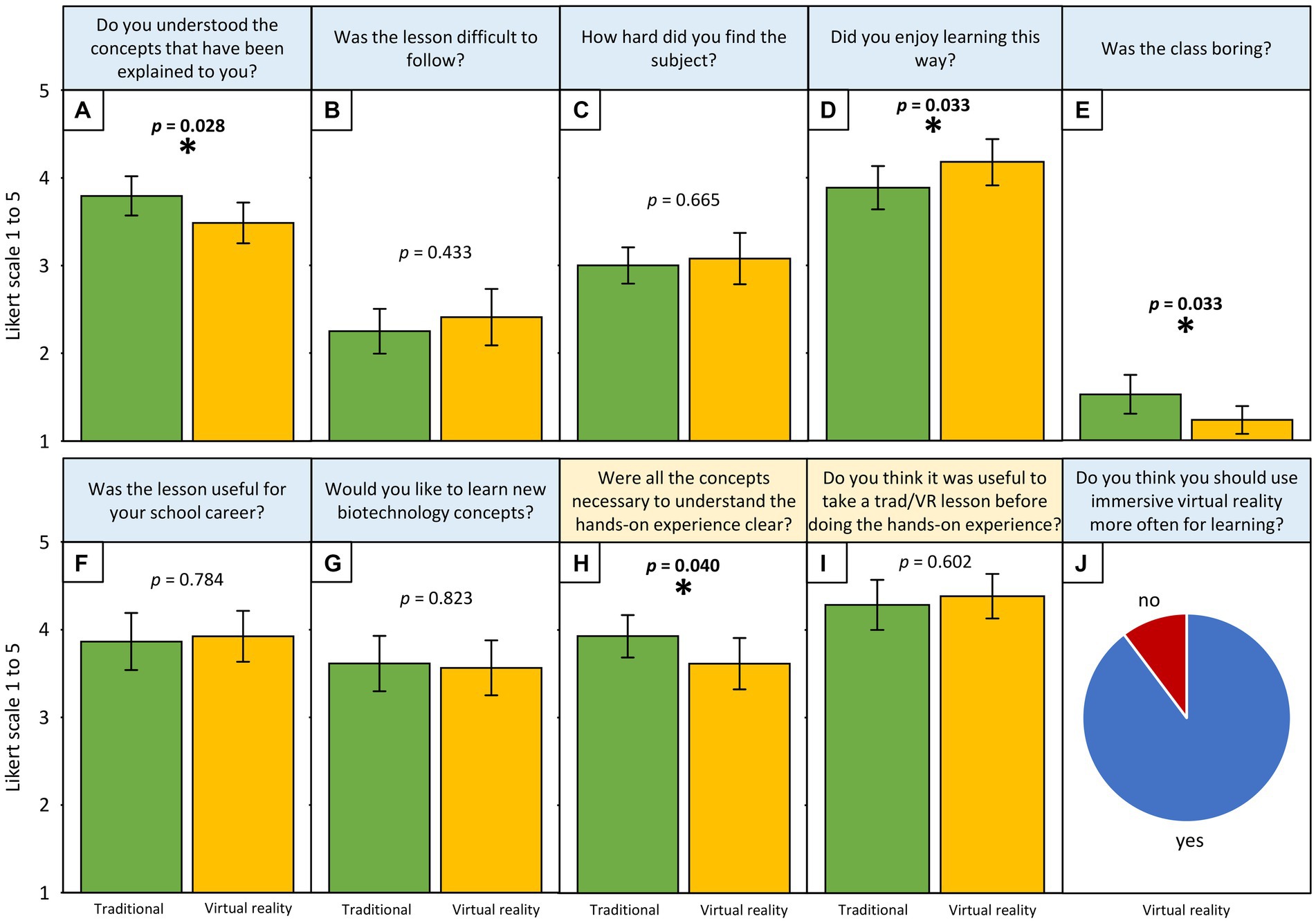
Figure 5. (A-J) Responses of the two groups to questions related to the participants’ perception regarding different aspects of the laboratory experience. Measurements were done through a 5-point Likert scale spanning from 1 (very low) to 5 (very high). Questions highlighted in blue (A-G, J) were administered in the Mid-test, while questions highlighted in yellow (H, I) were administered in the Post-test. Error bars represent the 95% confidence interval, while asterisks indicate statistically significant differences between the two groups.
3.2 Study 2: virtual reality laboratory simulation in addition to the traditional laboratory program
In Study 2, 64% of the participants performed a VR laboratory simulation before an organic chemistry hands-on laboratory in addition to the traditional laboratory program. The participants who also attended the VR laboratory simulation reported a statistically significant increase in their theoretical knowledge, from 3.7 in the Pre-test up to 6.6 correct answers out of 10 questions in the Post-test (Figure 6A). After 30 days, the theoretical knowledge of these students did not drop significantly (Ret-test). The participants who attended the traditional program also reported a statistically significant increase in their theoretical knowledge, from 3.7 in the Pre-test up to 5.7 correct answers in the Post-test, and no significant knowledge drop after 30 days. When the two didactical approaches were compared, the students who attended the VR simulation reported a statistically significant higher knowledge level in the Post-test with respect to the students who attended the traditional program (Figure 6A), while no significant difference was observed in the retention test due to the high variability of the data.
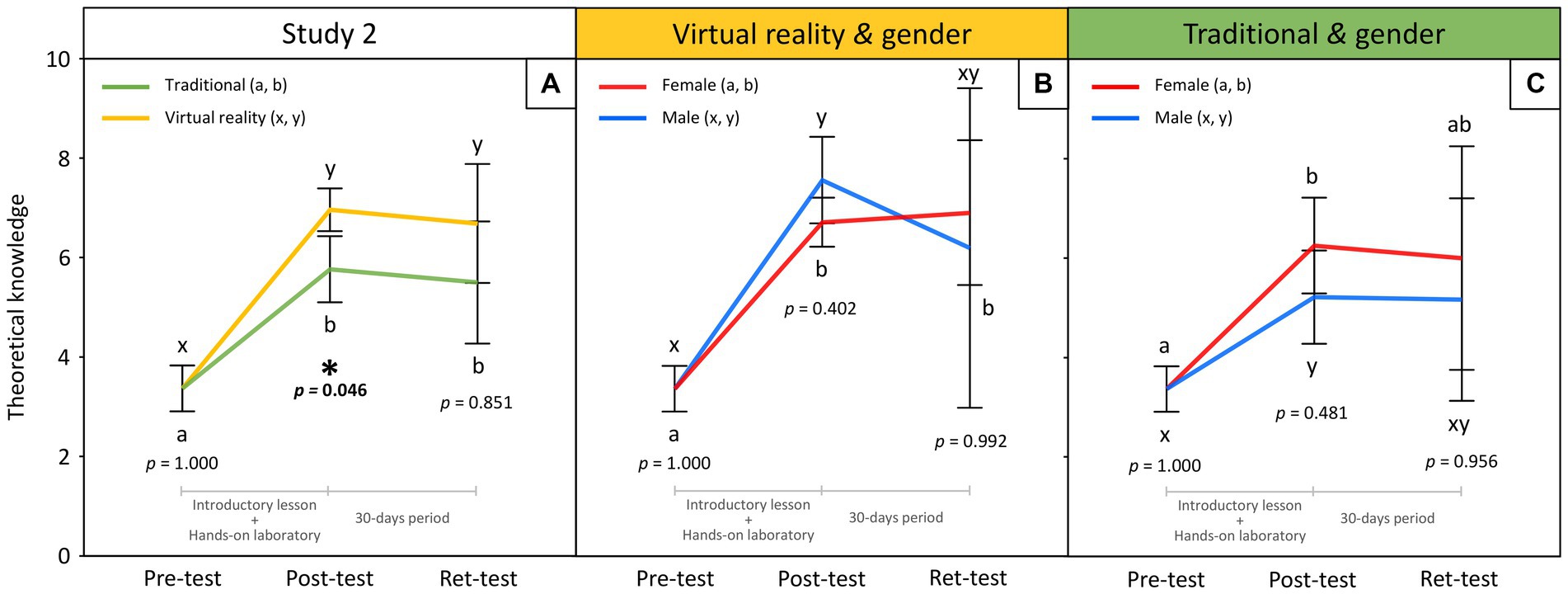
Figure 6. (A) Change in theoretical knowledge with the progress of the laboratory experience for the two groups of participants to Study 2. In panels (B,C), the data of the virtual reality and traditional groups are analyzed singularly to highlight gender differences. Error bars represent the 95% confidence interval, while asterisks indicate statistically significant differences between the two groups.
The performances of males and females were also analyzed separately, showing that no significant differences in the knowledge gained by the participants can be observed due to gender, both in the VR and the traditional approach (Figures 6B,C). No other differences in the students’ learning outcomes were found when the other demographic factors were analyzed separately (data not shown).
Figure 7 shows the responses to questions aimed at measuring the participants’ interest in biotechnology (Figures 7A,B) and self-efficacy (Figures 7C–E). Within the questions to measure the participants’ interest in biotechnology, we observed no statistically significant differences both with the progress of the laboratory experience and between the two participants’ groups (Figures 7A,B). A similar result was also observed for the self-efficacy question C (Figure 7C). Moreover, we observed a slight increase between the Pre- and Post-test in the student’s perceived ability to perform caffeine extraction alone, although not significant in the traditional group, followed by a decrease after 30 days (i.e., Ret-test; Figure 7D). On the contrary, in both groups, we observed a marked increase between the Pre- and Post-test in the student’s perceived ability to explain to a classmate the laboratory procedure, followed by a decrease after 30 days (Figure 7E). However, in all questions analyzed no statistically significant differences were observed between the two groups of participants (Figures 7A–E).
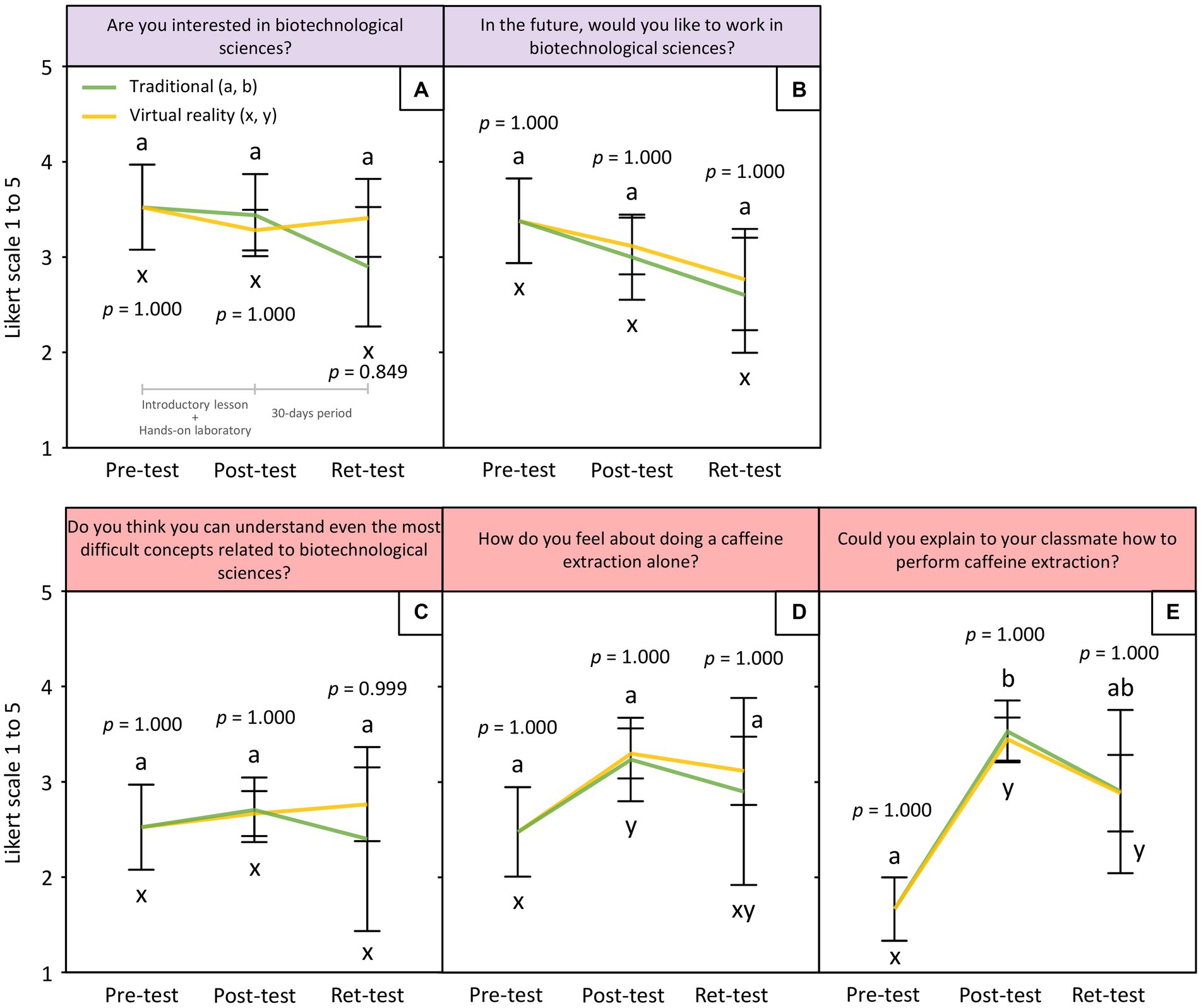
Figure 7. Change in the responses to questions related to (A, B) interest in biotechnology (purple) and (C-E) self-efficacy (red) with the progress of the laboratory experience and for the two groups of participants. Measurements were done through a 5-point Likert scale spanning from 1 (very low) to 5 (very high). Error bars represent the 95% confidence interval.
Further questions were administered in the Post-test to assess the participants’ perception regarding different aspects of the laboratory experience (Figure 8). The students who attended the VR simulation felt to have better acquired the concepts needed to understand the hands-on experience with respect to the students who attended the Traditional approach (Figure 8A). No statistically significant differences were observed regarding the difficulty of following the different steps of the hands-on experience (Figure 8B), and the usefulness of a theoretical lesson before doing the hands-on laboratory (Figure 8C). Furthermore, the students who attended the VR simulation felt less need for further teaching tools to better understand the hands-on laboratory experience (Figure 8D). Finally, the students who attended the VR simulation scored 3.65 points out of 5 when asked if carrying out the VR laboratory simulation was useful before performing the hands-on experience in the laboratory (Figure 8E). Among the participants who used the VR headsets, 90.0% of the students think that immersive VR should be used more often for teaching laboratory techniques, while 10.0% of the students responded negatively to this question (Figure 8F).
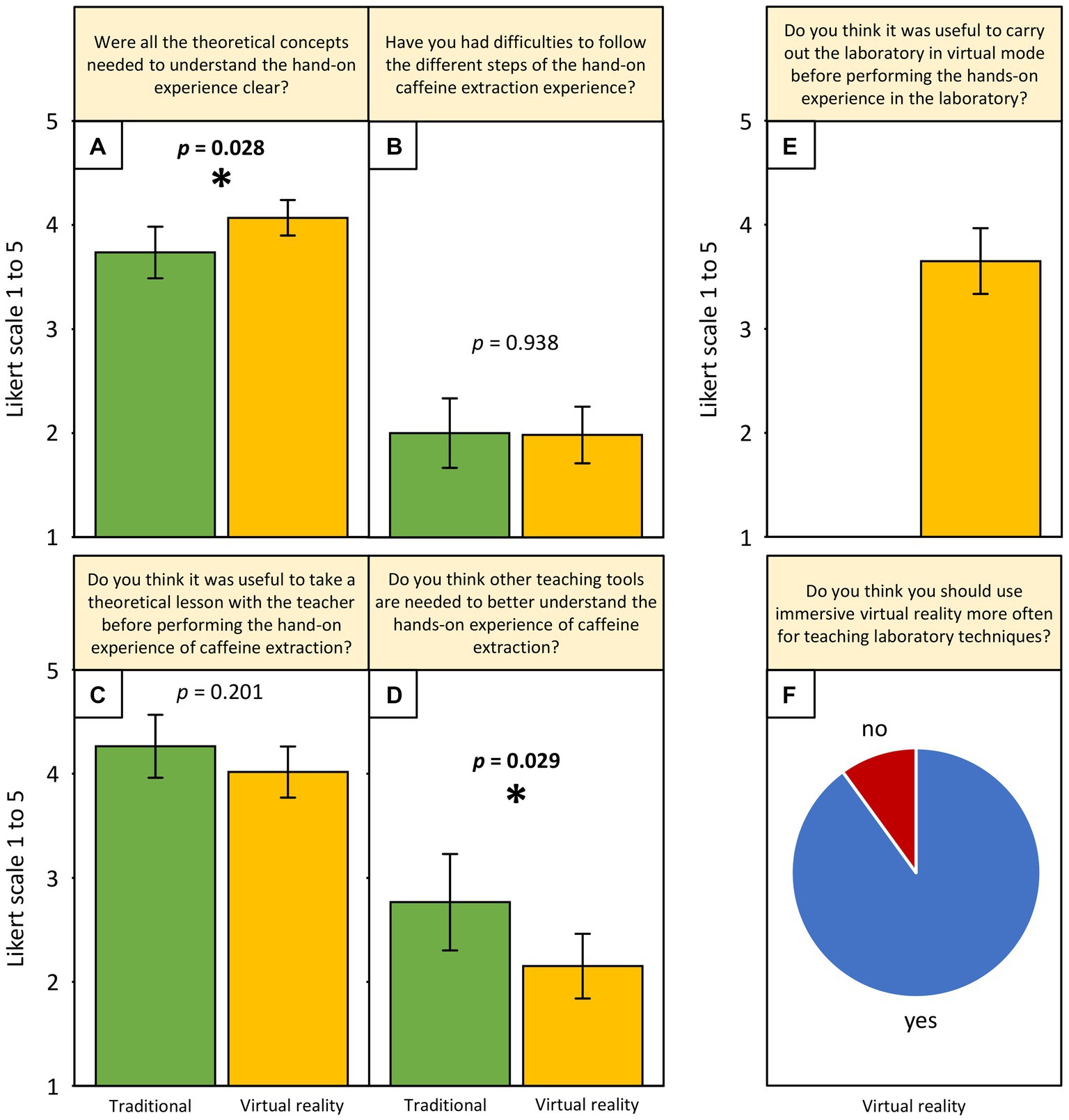
Figure 8. (A-F) Responses of the two groups to questions related to the participants’ perception regarding different aspects of the laboratory experience. Measurements were done through a 5-point Likert scale spanning from 1 (very low) to 5 (very high). These questions were administered in the Post-test. Error bars represent the 95% confidence interval, while asterisks indicate statistically significant differences between the two groups.
4 Discussion
4.1 Theoretical knowledge
Study 1 investigated the effects on motivational and learning outcomes of high school students when the introductory lesson to a hands-on laboratory is replaced with an equivalent virtual reality (VR) simulation. Diversely from what was hypothesized, our data show that the traditional lesson, consisting of an oral speech supported by a slideshow, was more effective in the participants’ learning of theoretical knowledge with respect to the VR simulation. The results reported in the literature, comparing traditional and innovative didactic approaches, are still poorly investigated and controversial, making our findings both in line and in contrast with previous studies conducted with similar approaches. In particular, Makransky et al. through the use of desktop VR simulations observed no differences in knowledge of microbiology between groups that attended either a virtual laboratory or a face-to-face tutorial (Makransky et al., 2016). More recently, Wismer et al. using immersive VR simulations for the biopharma industry training observed no differences in the knowledge scores of the group that attended the VR simulation with respect to the group that attended a real-life training (Wismer et al., 2021). On the contrary, Parong and Mayer found that a group of students that studied on a slideshow scored significantly better than the VR group and attributed this difference to the high load of non-essential visual inputs that characterize VR simulations (Parong and Mayer, 2018), diverting the student from the main narration and the important material (Chan et al., 2021). A further study compared the learning outcomes resulting from science desktop simulations vs. immersive VR simulations, showing that students who attended the immersive VR simulations learned less due to a higher cognitive load based on ECG measurements (Makransky et al., 2019c). This latter study concluded that learning science in immersive VR modality may overload and distract the learner, resulting in less opportunity to build solid learning outcomes (Makransky et al., 2019c).
However, each of these studies operates within a specific setting and methodology, which may influence the outcomes observed. One key aspect to consider is the diversity in VR implementation across studies, which can significantly impact the learning outcomes (Akpan and Shanker, 2019). The differences in VR applications for educational purposes could lead to variations in cognitive load, immersion levels, and nature of interaction, ultimately affecting learning outcomes (Suh and Prophet, 2018; Klingenberg et al., 2023). A further aspect that could influence the efficacy of VR content is the subject matter of the intervention (e.g., chemistry, biopharma, microbiology, biology, etc.) and the specific educational objectives that have been set. The application of VR simulations to a didactic approach could be more effective in a specific subject matter with respect to others depending on the conceptual complexity, the nature of the content, and the practical applications. Finally, also the demographic characteristics of the students, like age, level of education, and gender could play a role in shaping the efficacy of the VR intervention (Makransky et al., 2020). Regarding this latter, we observed a gender difference with regard to students who attended the immersive VR simulation, as female students reported significantly higher theoretical knowledge scores than male students following the VR simulation. Similar results were previously observed by Makransky et al. in regard to science career aspiration, with only females reporting a significant increase following the immersive VR simulation, demonstrating the potential of VR simulations to contribute to balancing out the gender gap in STEM fields (Makransky et al., 2020). Subsequent studies found that when there is a transition from face-to-face to digital learning, as happened during the pandemic-induced school closures, females might adjust their methods and be more adaptable to the new learning environment (Liu et al., 2021), being also more perseverant (Yu, 2021) and engaged than males (Korlat et al., 2021), thus, getting more benefits from digital learning. Regarding the age of the beneficiaries of the VR intervention, given the small age gap between the high school students participating in our study (17–19 years old), we observed no differences in the student’s learning outcomes related to age (data not shown). Considering the age and level of education of the participants in the studies currently available in the literature [i.e., university students with a mean age of 23.8 years in Makransky et al. (2019c), university students with mean age of 19.2 years in Parong and Mayer (2018), university students with mean age of 20.2 years in Makransky et al. (2016), and secondary education students aged 16–53 in Wismer et al. (2021)], there seems to be no correlation between the efficacy of VR laboratory simulations with respect to traditional methods and these two demographic factors. At present, only one study addressed the influence of age and industry experience on self-reported learning outcomes consequently to training for mine rescue with virtual reality, finding that age and experience do not affect learning outcomes (Pedram et al., 2022). Nevertheless, in the field of biotechnology and STEM education, a comprehensive study on the efficacy of VR laboratory simulations in relation to the student’s age and level of education is still lacking.
In the case of Study 2 the immersive VR simulation was considered as an additional tool to improve the educational method used so far, and, thus, was added to the traditional program instead of substituting part of the program (e.g., the introductory lesson) as tested in Study 1 and by the majority of the literature currently available (Makransky et al., 2016; Parong and Mayer, 2018; Wismer et al., 2021). Our findings show that this different approach may significantly increase the theoretical knowledge of the students who were attending the laboratory experience, contributing to building a solid learning outcome. These data support our initial hypothesis and provide a significant starting point for developing effective and efficient strategies to incorporate VR laboratory simulations in educational programs. In a recent review concerning virtual chemical laboratories, Chan et al. reported that combining virtual laboratories with a conventional lesson and/or a hands-on laboratory seems to result in a greater learning improvement than the traditional approach alone as it reinforces the previously learned concepts (Chan et al., 2021). It is suggested that a high level of interactivity engages the learner to develop a deeper understanding of the learning content (Davenport et al., 2018).
4.2 Interest in science
Diversely from what was expected, in Study 1 the participants of both groups (VR and Traditional) showed no increase in interest in biotechnology following the introductory lesson and the laboratory activity, demonstrating that with both didactical approaches a single educational guidance experience was not enough to make high-school students more passionate about biotechnology. Similarly, Study 2 showed no increase in the interest in biotechnology in first-year B.S. students following the whole educational experience proposed. These results reject our hypothesis and are not consistent with previous research. Other authors reported an overall increase in interest in science following a single didactical activity with VR simulations (Makransky et al., 2019a, 2020), while Andersen et al. found that immersive VR was more effective than 2D video in fostering science interest (Andersen et al., 2023).
4.3 Self-efficacy
With the questions aimed to measure the participants’ self-efficacy, we observed, in both studies and groups of participants, a significant increase with the progress of the laboratory experience in the perceived ability to explain to a classmate the laboratory procedures, indicating that the students are developing a clear idea of what they were taught. Also, in students who used VR simulations in Study 2, we observed an increase in the perceived ability to perform caffeine extraction alone, however not statistically different from the data obtained from students who have not used VR simulations. Altogether, these results support our hypothesis only partially, as we were expecting an increased self-efficacy in all items tested. Furthermore, our data suggest that the use of VR simulations does not affect the student’s perceived self-efficacy when compared to traditional didactical methods. Makransky et al. observed a similar pattern, i.e., an increase in self-efficacy but no difference between the two groups, when comparing students who used a virtual simulation with students who attended a face-to-face tutorial (Makransky et al., 2016). The finding that both groups of students report the same self-efficacy outcomes when virtual simulations are used as an alternative to traditional methods is of particular importance as non-cognitive skills are emerging to play an essential role in academic success and life outcomes and, consequently, with a significant long-term impact (Garcia, 2014). Thus, when testing new technologies for educational purposes it is important to assess that the new didactical methods are not impairing the development of non-cognitive skills (Kautz et al., 2014).
4.4 Engagement and perceived learning
In Study 1 we found that at the end of the introductory lesson the participants who joined the traditional lesson felt to have better understood the concepts that had been explained to them with respect to the participants who attended the VR simulation. Also, at the end of the laboratory experience, these participants scored higher ratings when asked if the concepts necessary to understand the hands-on experience were clear. These data reflect what was observed with the theoretical knowledge test, which showed that participants who attended the traditional introductory lesson actually obtained a higher learning outcome both in the Mid- and Post-test. On the contrary, the students who attended the VR simulation reported a higher enjoyment and felt that the introductory lesson was less boring with respect to the students who attended the traditional lesson. No wonder that 90% of the participants in Study 1 believe that immersive VR should be used more often for school learning. These results support the hypothesis that VR simulations can improve students’ engagement. Other studies reported similar results, for instance, Parong and Mayer obtained more enjoyment and less boredom in the VR group and suggested that students have more positive feelings when learning in immersive VR than from a slideshow, despite showing a lower learning outcome (Parong and Mayer, 2018). The authors concluded that immersive VR appears to create situational interest, but adding interesting features to a lesson may not be enough to enhance learning outcomes (Dewey, 1913). Since VR simulations motivate students more than conventional media, Parong and Mayer suggest using these new technologies in tandem with the existing strategies to spark interest among learners while maintaining high learning outcomes (Parong and Mayer, 2018).
In light of these data, Study 2 has tested the use of VR simulations as an additional tool to improve the existing educational method. Students who attended also the VR simulation felt to have better understood the concepts needed and felt less need for further teaching tools to understand the hands-on laboratory experience, showing that VR simulations contributed to building a better background to deal with the subsequent hands-on laboratory. These data are further supported by the increased theoretical knowledge observed in the students who took advantage of VR simulations, showing that the students’ feelings align with their effective knowledge about the topic at the end of the laboratory experience.
5 Conclusion
Overall, it can be concluded that immersive virtual reality (VR) laboratory simulations are a promising technology to improve traditional educational methods. However, care should be taken to the approach used to integrate these new technologies with the existing methods, as very different outcomes can be obtained depending on the chosen strategy. This research showed that when the introductory lesson to a biotechnology hands-on laboratory is replaced with an equivalent immersive VR simulation, the student’s learning outcomes suffer a reduction with respect to the traditional approach. On the contrary, when VR simulations are integrated as an additional tool to the existing learning methods, higher learning outcomes were observed demonstrating a deeper understanding of the learning contents. Furthermore, our study showed that learning with immersive VR simulations motivates students more than the traditional methods, thus, using this new technology in addition to the existing educational methods could be considered as a win-win strategy to raise the attention of the students while increasing the learning outcomes.
In the coming years, the constant improvement of the software will allow the development of VR simulations increasingly tailored to the student’s learning needs, promising to further revolutionize the use of VR simulations in educational programs. Further research will be necessary to investigate new strategies to integrate immersive VR simulations with the existing learning methods and demonstrate their effectiveness or limitations. Finally, one of the limitations of this study was the failure to accurately assess the participants’ practical and manual skills. These skills are supposed to rise when students exercise with immersive VR laboratory simulations, preparing them for the subsequent hands-on laboratory experience. Therefore, future research should focus on measuring these skills to assess if the participants’ practical and manual skills benefit from practicing with immersive VR laboratory simulations.
Data availability statement
The original contributions presented in the study are included in the article/Supplementary material, further inquiries can be directed to the corresponding author.
Ethics statement
The research was reviewed and approved by the ethics committee “Comitato Etico per la Ricerca” of the University of Insubria. Moreover, written informed consent was not required from the participants or the participants’ legal guardians/next of kin in accordance with the European legislation (EU GDPR – Key Issue Consent and Art. 7, Recitals 32) implemented by the national legislation (art. 2 Cod. civ. and D.Lgs 101/2018) because all participants were over 16 years old.
Author contributions
PB: Data curation, Formal analysis, Investigation, Methodology, Software, Visualization, Validation, Writing – original draft, Writing – review & editing. AG: Conceptualization, Project administration, Resources, Writing – review & editing. SB: Conceptualization, Funding acquisition, Writing – review & editing. EC: Conceptualization, Project administration, Investigation, Writing – review & editing. MB: Project administration, Funding acquisition, Writing – review & editing. AM: Conceptualization, Funding acquisition, Methodology, Project administration, Resources, Supervision, Validation, Writing – original draft, Writing – review & editing.
Funding
The author(s) declare that financial support was received for the research, authorship, and/or publication of this article. PB is a postdoctoral scholar funded by the Teaching and Learning Center (TLC) of the University of Insubria. The research was funded by the Department of Biotechnology and Life Sciences of the University of Insubria. The funders played no role in the study design, data collection, analysis and interpretation of data, or the writing of this manuscript.
Acknowledgments
The authors acknowledge Pierlorenzo Peruzzo, Alberto Zavattoni, and Simone Zamparini (OpenLab S.R.L.) for providing virtual laboratory simulations and regular technical support. The authors are also grateful to Rossella Roncoroni and Nicolò Antonelli (University of Insubria) for their valuable help in tutoring the virtual simulations and hands-on laboratory experiences.
Conflict of interest
The authors declare that the research was conducted in the absence of any commercial or financial relationships that could be construed as a potential conflict of interest.
Publisher’s note
All claims expressed in this article are solely those of the authors and do not necessarily represent those of their affiliated organizations, or those of the publisher, the editors and the reviewers. Any product that may be evaluated in this article, or claim that may be made by its manufacturer, is not guaranteed or endorsed by the publisher.
Supplementary material
The Supplementary material for this article can be found online at: https://www.frontiersin.org/articles/10.3389/feduc.2024.1354526/full#supplementary-material
References
Akpan, I. J., and Shanker, M. (2019). A comparative evaluation of the effectiveness of virtual reality, 3D visualization and 2D visual interactive simulation: an exploratory meta-analysis. Simulation 95, 003754971875703–003754971875170. doi: 10.1177/0037549718757039
Andersen, M. S., Klingenberg, S., Petersen, G. B., Creed, P. A., and Makransky, G. (2023). Fostering science interests through head-mounted displays. J. Comput. Assist. Learn. 39, 369–379. doi: 10.1111/jcal.12749
Bodekaer, M . (2016). This virtual lab will revolutionize science class. Available at: https://www.ted.com/talks/michael_bodekaer_this_virtual_lab_will_revolutionize_science_class/transcript
Bonde, M. T., Makransky, G., Wandall, J., Larsen, M. V., Morsing, M., Jarmer, H., et al. (2014). Improving biotech education through gamified laboratory simulations. Nat. Biotechnol. 32, 694–697. doi: 10.1038/nbt.2955
Carnevale, A., Mannocchi, I., Sassi, M. S. H., Carli, M., De Luca, G., Longo, U. G., et al. (2022). Virtual reality for shoulder rehabilitation: accuracy evaluation of oculus quest 2. Sensors 22:5511. doi: 10.3390/s22155511
Center for Self-Determination Theory Intrinsic Motivation Inventory (IMI). Available at: https://www.selfdeterminationtheory.org/intrinsic-motivation-inventory/ (Accessed February 16, 2024).
Chan, P., Van Gerven, T., Dubois, J.-L., and Bernaerts, K. (2021). Virtual chemical laboratories: a systematic literature review of research, technologies and instructional design. Comput. Educ. Open 2:100053. doi: 10.1016/j.caeo.2021.100053
Davenport, J. L., Rafferty, A. N., and Yaron, D. J. (2018). Whether and how authentic contexts using a virtual chemistry lab support learning. J. Chem. Educ. 95, 1250–1259. doi: 10.1021/acs.jchemed.8b00048
Deci, E. L., Eghrarl, H., Patrick, B. C., and Leone, D. R. (1994). Facilitating internalization: the self-determination theory perspective. J. Pers. 62, 119–142. doi: 10.1111/j.1467-6494.1994.tb00797.x
Eastwood, J. L., and Sadler, T. D. (2013). Teachers’ implementation of a game-based biotechnology curriculum. Comput. Educ. 66, 11–24. doi: 10.1016/j.compedu.2013.02.003
Garcia, E. (2014). “The Need to Address Non-Cognitive Skills in the Education Policy Agenda,” in Non-cognitive Skills and Factors in Educational Attainment (Rotterdam: SensePublishers), 31–64.
Kautz, T., Heckman, J. J., Diris, R., ter Weel, B., and Borghans, L. (2014). Fostering and measuring skills: improving cognitive and non-cognitive skills to promote lifetime success. SSRN Electron. J. 119, 1–5. doi: 10.2139/ssrn.2543890
Klingenberg, S., Fischer, R., Zettler, I., and Makransky, G. (2023). Facilitating learning in immersive virtual reality: segmentation, summarizing, both or none? J. Comput. Assist. Learn. 39, 218–230. doi: 10.1111/jcal.12741
Korlat, S., Kollmayer, M., Holzer, J., Lüftenegger, M., Pelikan, E. R., Schober, B., et al. (2021). Gender differences in digital learning during COVID-19: competence beliefs, intrinsic value, learning engagement, and perceived teacher support. Front. Psychol. 12, 1–12. doi: 10.3389/fpsyg.2021.637776
Jumbri, K., and Ishak, M. A. I. (2022). Can Virtual Reality Increases Students Interest in Computational Chemistry Course? A Review. J. Penelit. dan Pengkaj. Ilmu Pendidik. e-Saintika 6, 190–201. doi: 10.36312/esaintika.v6i3.885
Liu, X., He, W., Zhao, L., and Hong, J. C. (2021). Gender differences in self-regulated online learning during the COVID-19 lockdown. Front. Psychol. 12, 1–8. doi: 10.3389/fpsyg.2021.752131
Makransky, G., Borre-Gude, S., and Mayer, R. E. (2019a). Motivational and cognitive benefits of training in immersive virtual reality based on multiple assessments. J. Comput. Assist. Learn. 35, 691–707. doi: 10.1111/jcal.12375
Makransky, G., Mayer, R. E., Veitch, N., Hood, M., Christensen, K. B., and Gadegaard, H. (2019b). Equivalence of using a desktop virtual reality science simulation at home and in class. PLoS One 14, 1–14. doi: 10.1371/journal.pone.0214944
Makransky, G., Petersen, G. B., and Klingenberg, S. (2020). Can an immersive virtual reality simulation increase students’ interest and career aspirations in science? Br. J. Educ. Technol. 51, 2079–2097. doi: 10.1111/bjet.12954
Makransky, G., Terkildsen, T. S., and Mayer, R. E. (2019c). Adding immersive virtual reality to a science lab simulation causes more presence but less learning. Learn. Instr. 60, 225–236. doi: 10.1016/j.learninstruc.2017.12.007
Makransky, G., Thisgaard, M. W., and Gadegaard, H. (2016). Virtual simulations as preparation for lab exercises: assessing learning of key laboratory skills in microbiology and improvement of essential non-cognitive skills. PLoS One 11, 1–11. doi: 10.1371/journal.pone.0155895
Parong, J., and Mayer, R. E. (2018). Learning science in immersive virtual reality. J. Educ. Psychol. 110, 785–797. doi: 10.1037/edu0000241
Pedram, S., Palmisano, S., Miellet, S., Farrelly, M., and Perez, P. (2022). Influence of age and industry experience on learning experiences and outcomes in virtual reality mines rescue training. Front. Virtual Real. 3, 1–12. doi: 10.3389/frvir.2022.941225
Peruzzo, P., Zavattoni, A., and Zamparini, S. (2023). OpenLabVR - virtual reality laboratory simulations. Available at: https://www.openlabvr.com/ (Accessed February 16, 2024).
Pintrich, P. R., Smith, D. A. F., Garcia, T., and McKeachie, W. J. (1991). A manual for the use of motivated strategies for learning questionnaire (MSLQ, NCRIPTAL-91-B-004). Retrieved from National Measuring Self-Regulation in Self-Paced Open and Distance Learning Environments Kocdar, Karadeniz, Bozkurt, and Buyuk Center for Research to Improve Postsecondary Teaching and Learning. Ann Arbor, University of Michigan. Available at: https://files.eric.ed.gov/fulltext/ED338122.pdf
Schnieder, M., Williams, S., and Ghosh, S. (2022). Comparison of in-person and virtual labs/tutorials for engineering students using blended learning principles. Educ. Sci. 12:153. doi: 10.3390/educsci12030153
Soliman, M., Pesyridis, A., Dalaymani-Zad, D., Gronfula, M., and Kourmpetis, M. (2021). The application of virtual reality in engineering education. Appl. Sci. 11, 1–14. doi: 10.3390/app11062879
Suh, A., and Prophet, J. (2018). The state of immersive technology research: a literature analysis. Comput. Human Behav. 86, 77–90. doi: 10.1016/j.chb.2018.04.019
Sviridova, E., Yastrebova, E., Bakirova, G., and Rebrina, F. (2023). Immersive technologies as an innovative tool to increase academic success and motivation in higher education. Front. Educ. 8, 1–10. doi: 10.3389/feduc.2023.1192760
Thisgaard, M., and Makransky, G. (2017). Virtual learning simulations in high school: effects on cognitive and non-cognitive outcomes and implications on the development of STEM academic and career choice. Front. Psychol. 8, 1–13. doi: 10.3389/fpsyg.2017.00805
Wismer, P., Lopez Cordoba, A., Baceviciute, S., Clauson-Kaas, F., and Sommer, M. O. A. (2021). Immersive virtual reality as a competitive training strategy for the biopharma industry. Nat. Biotechnol. 39, 116–119. doi: 10.1038/s41587-020-00784-5
Keywords: theoretical knowledge, engagement, questionnaire, VR headset, hands-on laboratory, science laboratory, improving students’ learning, self-efficacy
Citation: Beatrice P, Grimaldi A, Bonometti S, Caruso E, Bracale M and Montagnoli A (2024) Adding immersive virtual reality laboratory simulations to traditional teaching methods enhances biotechnology learning outcomes. Front. Educ. 9:1354526. doi: 10.3389/feduc.2024.1354526
Edited by:
Patsie Polly, University of New South Wales, AustraliaReviewed by:
Jodi Asbell-Clarke, TERC, United StatesMilan Kubiatko, J. E. Purkyne University, Czechia
Copyright © 2024 Beatrice, Grimaldi, Bonometti, Caruso, Bracale and Montagnoli. This is an open-access article distributed under the terms of the Creative Commons Attribution License (CC BY). The use, distribution or reproduction in other forums is permitted, provided the original author(s) and the copyright owner(s) are credited and that the original publication in this journal is cited, in accordance with accepted academic practice. No use, distribution or reproduction is permitted which does not comply with these terms.
*Correspondence: Peter Beatrice, peter.beatrice@uninsubria.it