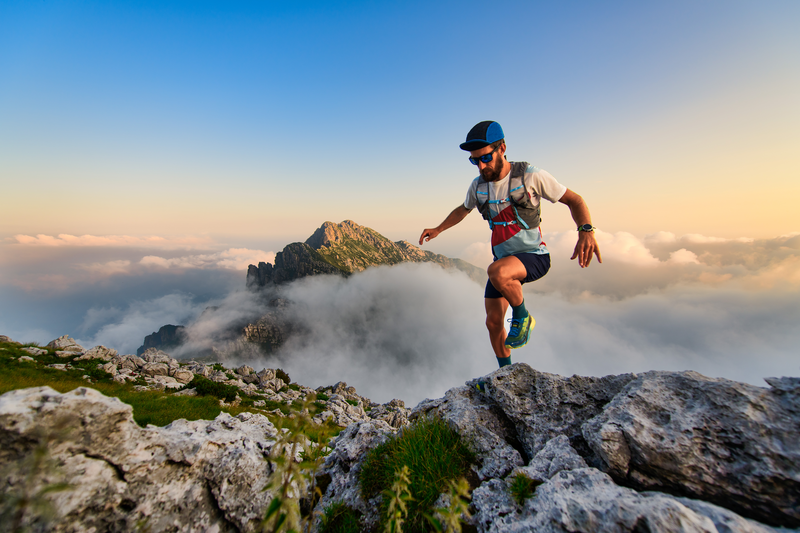
94% of researchers rate our articles as excellent or good
Learn more about the work of our research integrity team to safeguard the quality of each article we publish.
Find out more
ORIGINAL RESEARCH article
Front. Educ. , 22 February 2024
Sec. STEM Education
Volume 9 - 2024 | https://doi.org/10.3389/feduc.2024.1339957
Nanoscale Science and Technology (NST) is a rapidly evolving field with profound implications for various industries and our everyday lives. However, misconceptions among learners can hinder their ability to grasp the fundamental concepts of NST, thereby impeding their potential contributions to this advancing domain. Concept maps (CM) and conceptual change texts (CCT) are graphical and written representations of knowledge that enable learners to visualize relationships between concepts and assess the coherence of their understanding. In this pursuit, we engage with the concept of rehabilitation for misconceptions, viewing the learning process as a transformative journey akin to cognitive rehabilitation. Through this CM-CCT constructivist approach, learners are encouraged to engage in critical reflection, self-questioning, and peer discussions, which facilitate the identification of misconceptions. Moreover, CM-CCT provide a structured framework for presenting accurate information about NST, offering a clear depiction of the hierarchical and interconnected nature of nanoscale phenomena. The aim of this study was to evaluate the effectiveness of CM-CCT in correcting the misconceptions of undergraduate university students regarding nanotechnology and the taxonomy of nonmaterial. Prior to the implementation of the CM-CCT, an assessment of pre-existing knowledge of the students was performed through the structure of the observed learning outcomes (SOLO) taxonomy. A quasi-experimental research design was carried out. A total of 70 undergraduate university students, divided into two intact groups, were cross-examined for the study. Further, before and after the instructional tools, an achievement test based on nanotechnology and classification of nonmaterial was conducted, covering all six cognitive domains of the Bloom taxonomy of educational objectives. Data analysis revealed that the instructional tools based on constructivist approach had a statistically significant impact on students for elimination of their misconceptions about nanotechnology, nano science and classification of nonmaterial.
Acquiring a deep understanding of complex and controversial scientific concepts like atomic structure, space–time curvature and nanotechnology, require the reconstruction of intuitive notions to create a more robust scientific conceptual framework (Arabeyyat et al., 2022). The chemistry and physics education faces the challenge of ensuring that students fully grasp the concepts of nanotechnology that are being presented to them (Song and Long, 2022). Nanotechnology has emerged as an important research area in recent years, with applications in both traditional and advanced industries such as microprocessors, food, and textiles (Rudolf et al., 2020; Nazar et al., 2021; Najeeb et al., 2022). As a result, there is a growing demand for nanotechnology education at the undergraduate university level to equip students with the essential skills and knowledge needed to succeed in this field (Bartolucci et al., 2022). Teaching nanotechnology to undergraduate university students presents unique set of challenges due to interdisciplinary nature and the distinctive behavior of materials at the nanoscale (Quirola et al., 2018; Sakhnini and Blonder, 2018; Curreli and Rakich, 2020). Nevertheless, previous research studies suggest that nanotechnology education increases students’ motivation (Yolcua and Dyehouse, 2018), their inclination to pursue a scientific career (Rudolf et al., 2020) and encourages their curiosity and their commitment in the field of science. Moreover, it helps to improve students’ understanding of the nature of modern science (Blonder and Mamlok-Naaman, 2019).
Several factors can influence the development of critical conceptual understanding, such as the difficulty and complexity of the subject matter (Nugroho, 2021), the type of instructional materials used, and the level of student engagement (Madaiton et al., 2022), additionally, considering individual beliefs and existing knowledge (Dorsah and Okyer, 2020). These elements may contribute to the formation of misconceptions, which are individual ideas (Blonder and Mamlok-Naaman, 2019) views, and understandings that are inconsistent with commonly accepted scientific meaning. Students may hold into such misconceptions from their secondary education, moreover, these cognitive frameworks and experiences can impede their absorption of fresh ideas (Addido et al., 2022). People comprehend the world by constructing interpretations that make sense of their surroundings, drawing from their personal experiences and preexisting knowledge (Slater et al., 2018).
The integration of nanotechnology into the chemistry and physics curriculum has been accomplished via elective modules and a range of engaging learning experiences (Mushtaq et al., 2017, 2018; Koolen et al., 2022). The incorporation of nanotechnology presents distinct challenges due to the unique characteristics of nanoscale materials, which manifest variations both at the molecular and macroscopic levels, leading to the distinctive properties of nanomaterials. Nanoscience and technology (NST) encompass the manipulation of materials, devices, and systems to unveil entirely novel properties and functions, achieved by delving into their atomic, molecular, and macromolecular structures. NST represents an interdisciplinary field that amalgamates insights from chemistry, biology, physics, material science, medicine, and engineering (Rap et al., 2020).
The conceptual transformation technique is one of the well-known strategies and guidelines used to refute myths about scientific issues. The prior understanding of an idea substantially influences students’ capacity to understand it. As a result, when organizing classroom activities and instructional strategies, it is critical to grasp and comprehend the students’ conceptual frameworks (Gafoor and Akhilesh, 2010). Teachers should consider their students’ past knowledge in addition to how they present the topic. Thomas Khun’s ground-breaking notion of a “paradigm shift” in the history and philosophy of science in 1962 is credited with giving rise to conceptual change. As the basis for creating precise and solid scientific understandings, it involves a learning process that calls for the alteration of preexisting ideas (Barnett et al., 2015). Understanding concepts involves many different interpretations. Rather than depending solely on rote memorization, it refers to students’ ability to produce and integrate meaningful and practical interconnected ideas and facts (Mills, 2016).
The conventional approach to teaching chemistry and physics relies heavily on one-way instruction and often encourages memorization through rote learning. Consequently, in recent years, science educators have increasingly embraced student-centered and active learning methodologies (Sannathimmappa et al., 2022). Active-learning methods prioritize the involvement of learners, fostering their self-awareness regarding the learning process, ultimately cultivating lifelong learning skills (Slieman and Camarata, 2019). According to Cetin et al. (2015) an alternative approach to shifting instruction from shifting from a teacher-centered to a student-centered approach entails the adoption of a constructivist methodology.
According to Fatimah et al. (2022), the forerunners of constructivist theory in the early 20th century were Piaget, Bruner, and Vygotsky. They believed that knowledge and comprehension are actively acquired through personal experiences and experimental activities, rather than passively absorbed. Constructivism holds that science and culture are constructed, and do not exist inherently, in contrast to structuralism and positivism which view sociocultural elements as an unquestionable reality (Becerra and Castorina, 2018). This epistemological perspective, which is grounded in constructivism, posits that knowledge is a product of human construction (Candy, 1989; Yusdita et al., 2022; Nurhidayat et al., 2023). Constructivism holds that individuals are capable of constructing knowledge through their interactions with their environment (Amineh and Asl, 2015; Ginting et al., 2019). It focuses on the process of how individuals acquire knowledge rather than how teachers impart it (Sidabutar et al., 2023). Knowledge is seen as subjective and relative, shaped by the individual’s experiences and influenced by the socio-cultural environment, resulting in constantly evolving understanding based on interactions with the environment (Fatimah et al., 2022).
The constructivism learning paradigm emerged in response to the scientific revolution in the industrial era 4.0 (Fatimah et al., 2022), and it is characterized by several principles. Firstly, students personally and socially construct knowledge. Secondly, knowledge is not passively transferred from teachers to students, but is constructed through the students’ reasoning activities. Thirdly, active student construction leads to more complex and complete scientific concepts. Lastly, the teacher serves as a facilitator, supporting the student construction process (Huang, 2002; Murphy et al., 2005; Gordon, 2009; Fatimah et al., 2022). To effectively implement constructivism, teachers ought to possess specific traits, such as recognizing and valuing student autonomy, emphasizing the use of primary data and hands-on materials to improve cognitive abilities, and inspiring students to analyze and create. Moreover, teachers should take the time to comprehend their students’ perspectives before sharing their own insights, facilitate discussions, and nurture inquiry habits by posing open-ended questions (Kaufman, 2004; Koohang et al., 2014; Beerenwinkel and von Arx, 2017; Fatimah et al., 2022).
The application of constructivism learning theory in education involves utilizing five learning models for students: (1) Reasoning and Problem Solving; (2) Problem-Based Instruction; (3) Conceptual Learning; (4) Group Investigation; and (5) Inquiry Training (Fatimah et al., 2022). The conceptual learning model posits that knowledge is derived from interactions with the environment, and new knowledge can emerge from educational interventions, potentially leading to cognitive conflict (Fatimah et al., 2022). When cognitive conflict arises, students face choices such as maintaining their original intuition, revising it through assimilation, or changing their intuitive view to accommodate new knowledge (Rahmatya et al., 2019; Sakti et al., 2020; Fatimah et al., 2022). In this context, teaching is not about transmitting knowledge but facilitating and mediating the negotiation of meaning to foster conceptual change. The study in question uses the conceptual learning model.
Constructivism places significant emphasis on students’ existing conceptions and their individual experiences. Students construct their formal knowledge by building upon their pre-existing ideas and experiences within the educational setting. When new information or experiences are introduced in the classroom, students undergo a process of either rejecting or reshaping their existing cognitive structures, based on the alignment with their background knowledge. Ultimately, students’ own perceptions and novel ideas should seamlessly integrate into their memory as valuable components. Conversely, memorized facts or information that lack a connection to students’ pre-existing experiences tends to be forgotten easily. Hence, it is vital to connect new ideas or concepts to students’ prior experiences. This strategy assists in the creation of meaningful information within their current cognitive framework, promoting lasting knowledge retention (Balci et al., 2006).
The formation of a knowledge framework can be shaped by a range of factors, which include, but are not restricted to, students’ existing knowledge, personal experiences, information organization methods, perceptions, ideas, and their interactions with their surroundings (Putri et al., 2022b). The teacher’s responsibility is to guide students in cultivating new insights and connecting them with their existing knowledge. They should organize information around clusters of interconnected concepts and challenges to spark students’ interest in broader subjects. Initially, concepts should be introduced comprehensively before dissecting them into their constituent elements. Students should be encouraged to inquire, experiment, and arrive at their own conclusions with appropriate guidance (Putri et al., 2022a).
To foster meaningful learning of science concepts and promote a transformation in understanding, it is imperative to employ efficient strategies for addressing common misconceptions in science. One particularly successful approach for dispelling students’ misconceptions and improving their grasp of science concepts involves utilizing the “conceptual change approach” and incorporating concept mapping. A substantial body of research has been dedicated to examining the effectiveness of instructional techniques associated with the conceptual change approach in science, including the utilization of CM-CCT, with the aim of rectifying students’ alternative conceptions (Cetin et al., 2015).
Conceptual change texts represent a potent resource within the realm of science education. Instruction grounded in conceptual change texts has been shown to significantly enhance students’ academic performance in science (Gill et al., 2022). For instance, instruction based on conceptual change texts led to a more profound understanding of electrical concepts in comparison to traditional didactic texts (Sungur et al., 2001). Conceptual change texts and instruction incorporating concept mapping proved significantly more effective in enhancing students’ understanding of the human circulatory system in contrast to conventional teaching approaches (Sungur et al., 2001).
Joseph Novak and his research team were pioneers in the advancement of concept mapping as an assessment approach in the early 1970s (Novak and Cañas, 2008). This method is grounded in Ausubel’s theory of meaningful learning from 1968, which suggests that learners create knowledge by building upon their preexisting knowledge. This concept implies that links can be formed between prior knowledge and new information, and these connections can be visually depicted using a graphical tool commonly known as a CM. A concept map, therefore, connects concepts, with the foundation being formed by prior knowledge and new concepts expanding upon this foundation, not necessarily in a one-to-one correspondence. In a concept map, various elements such as words, objects, images, formulas, symbols, etc., are interconnected by lines, often accompanied by labels denoting propositions (Soika and Reiska, 2014).
A concept map comprises a set of propositions that elucidate the relationships among various concepts. When created thoughtfully, they have the capacity to visually represent meaningful connections between one or more distinct concepts. Concept maps find extensive utility in the educational context, where research indicates that this approach combats rote memorization, facilitates the synthesis of previously studied concepts, and captures concepts that evolve during class discussions (Soika and Reiska, 2014), they can be advantageous for crafting presentations and similar tasks. Nevertheless, CM also serve as a valuable assessment instrument, surpassing other assessment methods in providing insight into a student’s cognitive processes and their organization (Ghani et al., 2017). Educators have harnessed the potential of concept mapping as a potent educational tool to encourage active engagement, foster meaningful learning in students, and as a means of dispelling misconceptions (Maryam et al., 2021). Concept maps are visual tools in two dimensions, designed for the organization and representation of knowledge (Slieman and Camarata, 2019). Figure 1 represents the conceptual framework of the study.
Figure 1. Schematic showing the application of CM-CCT hybrid model elements involved in addressing the misconceptions in nanoscience and technology.
The conceptual frameworks that have been developed for this study focused on the idea that CM-CCT can be used as an effective tool for addressing misconceptions about nanotechnology and nano science. The conceptual framework showed that the misconceptions related to nanotechnology and nano science can be addressed by CM-CCT. As CM visually represent these concepts and their relationships, aiding in clarifying and organizing knowledge. Specific words or phrases can be used to establish connections between concepts, helping learners understand how these ideas relate to each other. The propositional structure part of the concept maps can be involved in breaking down complex ideas into propositions, which are statements that express relationships between concepts. It allows for a more detailed examination of the connections between concepts. The hierarchical structures might show how concepts can be organized hierarchically, illustrating the relationships from broad to specific within the context of nanotechnology and nano science. Focus questions are likely used to guide learners’ exploration of the concepts, encouraging critical thinking and active engagement with the material. Parking lot and cross links components may represent places to temporarily store related concepts or connections, ensuring that no important ideas are overlooked and allowing for later integration.
Through conceptual change texts problem presentation may introduce a problem or challenge related to nanotechnology or nano science. This can serve as a starting point for engaging students in the learning process. After identifying the common misconceptions, these will be addressed through effective teaching. Conceptual elaboration may involve providing in-depth explanations and insights into the correct concepts, helping students build a more accurate understanding of the subject matter. Students’ opinion and perspectives may be considered on the topic. This allows for an interactive and learner-centered approach, fostering engagement and a deeper understanding. Lastly, the effectiveness of the current approach was assessed. Are students’ misconceptions being corrected? Are they gaining a clearer understanding of nanotechnology and nano science? Evaluation helps refine the teaching methods.
In summary, the conceptual framework was designed to incorporate CM-CCT as complementary tools for addressing misconceptions in nanotechnology and nano science. CM visually organize key concepts and their relationships, while conceptual change texts guide students through a process of recognizing and correcting misconceptions, promoting deeper understanding, and encouraging student engagement and evaluation.
With the help of this kind of understanding, students can learn things that they can apply outside of the four walls of the classroom (Moser and Chen, 2016). From a broader standpoint (Vosniadou and Mason, 2012), connected conceptual knowledge with a transformational change in attitudes and values pertaining to a particular notion, which might stir up powerful feelings, prejudices, and personal views. In the end, this method of teaching has a substantial impact on how well students understand a concept and how well they can explain a specific phenomenon (Konicek-Moran and Keeley, 2015). When students understand the significance of the lesson and can apply it to real-world situations, learning becomes effective and lasting.
To effectively address the specifics of nanotechnology and eliminate misunderstandings, the CM and conceptual change texts both as constructivist approach were used in this study. CM typically consist of concepts, which are often enclosed within circles or various types of boxes, interconnected by lines that link two or more of these concepts (Novak and Cañas, 2008). The link between two concepts is denoted by the text displayed on the connecting line, often referred to as linking words or phrases. CM, excel in promoting higher-order thinking skills compared to many alternative instructional methods. Crafting concept maps demands a deep comprehension of the intricate relationships between various concepts, thereby enhancing the acquisition of knowledge that goes beyond mere rote learning or memorization.
Conceptual relationships are conveyed through propositions, and these propositions, when they form a coherent statement encompassing multiple concepts, are termed “semantic units.” Typically, these units are arranged hierarchically in CM, with broader, more general concepts positioned at the top, and more specific ones at the bottom. Strong propositions establish robust connections between these two levels of concepts (Katagall et al., 2015). Crosslinks can be established to provide responses to the central “focus question (Novak and Cañas, 2008). Concept maps offer numerous benefits to learners. They enable a structured and well-organized presentation of concepts, making it easier for students to grasp challenging ideas. Concept maps also create opportunities for students to establish relevant and pivotal connections within the subject matter, stimulating their interest, encouraging the generation of fresh insights, and fostering critical thinking, creativity, problem-solving skills, and the integration of theory into practical applications. Moreover, they facilitate collaboration among students, help identify areas of learning gaps, clarify misconceptions or uncertainties, and aid in establishing connections between prior knowledge and newly introduced concepts, ultimately promoting meaningful learning over mere rote memorization (Maryam et al., 2021). Concept maps are typically employed in the learning process through one of two methods: either students are tasked with independently creating CM on a given topic, or they are instructed to analyze CM provided by the instructor, drawing connections between terms or phrases. Both of these approaches have proven to be effective in improving students’ learning outcomes (Slieman and Camarata, 2019).
Our study aims to provide important insights into pedagogical practices that support effective learning in the rapidly developing field of nanoscale science and technology, where conceptual comprehension is critical. We aimed to determine the precise effect of the constructivist approach on the correction of misunderstandings by contrasting the results of the experimental group, which received the CM-CCT intervention, with those of the control group, which received traditional teaching approaches. In addition, our study sought to clarify, if this teaching approach may be applied to improve students’ understanding of complex nanoscale ideas. Our study sought to offer evidence-based recommendations for educators and curriculum developers looking for creative ways to solve misconceptions in the difficult field of nanoscale science and technology.
To address the prevailing alternative conceptions of nanoscience, we experimented through the concept maps and conceptual change texts as constructivist approach of instruction. Concept maps consisted of a number of stages and procedures. Teachers can help students in these stages to correct their misconceptions about certain topics. In detail, this research used different paradigms of teaching techniques at each stage of the framework and interventions to assess the instructional settings used in the lesson plans. Therefore, our research aims to respond all of the following research questions:
• Identifying misconceptions of chemistry students about nanotechnology, nanoscale, and classification of nonmaterial through SOLO taxonomy.
• What is the effect of the CM-CCT on students’ misconceptions about nanotechnology, nanoscale, and classification of nanomaterial?
A non-equivalent control group design was used. The lesson plans, on the other hand, were developed based on the constructivist approach. The experimental group was taught using CM-CCT while the control group was taught using the traditional instruction (TI) model. The impact of the intervention on students’ knowledge of nanotechnology, nanoscale, and classification of nanomaterial was assessed using the pre-test/post-test.
The intervention was conducted at university level in the University of Education, Lahore, Multan Campus, Pakistan. A total of 70 under graduate university students were asked questions about their concepts of nanotechnology, nanoscience and nanoparticles. The SOLO taxonomy was used to assess their knowledge. To assess the students’ grasp of nanotechnology; a 25-point achievement test was delivered. Item analysis was performed on the test to guarantee item difficulty and discrimination (Hamamoto Filho et al., 2020).
The students’ prior knowledge was evaluated using the “Structure of the Observed Learning Outcome” (SOLO) taxonomy (Biggs and Collis, 1989). The SOLO taxonomy is a framework for comprehending and quantifying the complexities of learning outcomes. It gives a framework for understanding how pupils progress from surface-level to deep-level understanding of a topic. It consists of five levels of comprehension that establish the taxonomy levels related to the configuration and content of nanotechnology, as shown in Table 1.
According to Richards et al. (2012), SOLO taxonomy offers a workable way to assess how well students advance from pre-structural competence to productive proficiency, which involves using higher levels of SOLO taxonomy. This shows the depth of learning that students can achieve at higher SOLO model levels, especially at the last level, extend abstract, which improves their ability to apply and conceptualize knowledge. It offers a vital starting point for creating curricula and lesson plans in the classroom that lead to profound, pertinent, and useful results. Teachers typically utilize SOLO Taxonomy to create experiences, learning objectives, and assessments at various levels so they may access and manage their teaching practices (Mahmood et al., 2020). According to Thompson et al. (2007), the SOLO taxonomy offers a framework for evaluating the caliber of responses from students as well as self-learning goals that they must meet to reach specific learning levels. According to Hook and Mills (2011), the SOLO taxonomy methodically aids in teachers’ and students’ comprehension of the learning process and learning objectives. In order to determine question levels, evaluate students’ quality of answers, and create learning objectives, SOLO Taxonomy has been widely applied in assessments (Groth, 2003 Teaching and Educational Development Institute, 2015).
This treatment spanned a period of 16 weeks. One class was designated as the experimental group, receiving instruction utilizing conceptual change texts and concept mapping, while the other group served as the control, undergoing traditional instruction. Both groups were taught same contents by the authors to control for potential teacher effects and biases, as well as to ensure consistent treatment. The control group received teacher-directed instruction, involving lectures and discussions to convey concepts, along with problem-solving techniques. Prior to each lesson, students were required to read the relevant topic from the course textbook. Concepts were introduced and defined, followed by discussions prompted by questions from the teacher. The majority of instructional time (70%–80%) was dedicated to these explanations and ensuing discussions. The remaining time was allocated for completing worksheets designed as practice activities, which included both mathematical and conceptual questions, tables to fill in, and space for students to create sketches and graphs. While students worked on these worksheets, the teacher facilitated the process, addressing questions and providing guidance as necessary. Worksheets were assessed and scored, with students reviewing their work following the correction process.
In the experimental group, students actively interacted with both conceptual change texts and concept mapping within a teacher-led lecture. The instructional approach, centered on conceptual change texts, operated under the assumption that students come into a new topic with pre-existing notions, which form the basis for their subsequent understanding. If these initial ideas are not addressed within the instructional process, they may endure largely unchanged and persist even after the instruction has concluded (Durmuş and Bayraktar, 2010). We should not automatically assume that preconceptions are always scientifically incorrect; indeed, they can sometimes act as “bridges” that help students transition to new understanding. Whether or not these preconceptions are accurate, it is essential to explicitly address them within the instructional process. In this study, both preconceptions and misconceptions were directly confronted within the conceptual change texts and during discussion segments. Students were actively encouraged to acknowledge their initial conceptions and openly share them with their peers in the class.
In the experimental group, students actively participated in both conceptual change texts and concept mapping activities within a lecture led by the instructors. Before the class, students were instructed to read the conceptual change texts independently, and during the class, they silently reviewed these texts with instructors available to offer assistance if needed. Following this, students engaged in discussions focused on the situations and statements presented in the texts. The instructor highlighted common misconceptions through questioning and provided the correct concepts, along with supporting evidence to debunk typical misconceptions. Subsequently, both students and researchers collectively examined the scenarios presented in the conceptual change texts, emphasizing students’ misconceptions and presenting scientifically accurate explanations. The results section of this study includes examples and figures for reference.
Students were given the job of making their own CM to show the relationships between these concepts after the conceptual change texts were discussed. Students were given an introduction to concept mapping techniques before starting the assignment. They learned about the qualities of CM and received instructions on how to make one. The students were given a list of important descriptors to use during the concept mapping tasks. They had to complete a partially filled map, create an idea map from scratch, and write an essay using a concept map, among other assignments. Students were provided a prepared structure with the necessary key descriptors and connecting lines for the “object-only” map exercise. However, it was frequently up to the students to decide which way the links pointed. In the “link-only” map exercises, students were given pre-structured maps but only the connecting lines and, in some cases, their directional arrows were provided; the critical descriptors were absent. Some of the students drawn concept maps are provided in Figure 2.
The word “proposition” refers to a claim that clarifies the relationship between concepts. The essential descriptions were to be incorporated by the students into their predetermined locations on the map. The fill-in map’s format was chosen based on the conceptual framework of the subject, with the main goal being to promote meaningful comprehension and help students organize their knowledge rather than just identifying and clearing up misconceptions. However, these maps also included some claims (propositions) that may clarify and correct misunderstandings. In the essay-only map activity, students were required to either write an essay based on a concept map that was provided or develop a concept map based on an essay that was provided. After that, the students took part in a variety of activities, such as filling up a map, creating a concept map from scratch, and writing an essay using a concept map as inspiration. The three methods were applied to each student in the experimental group in the same order. Each concept mapping approach was discussed separately throughout the instructional workshops on concept mapping. An illustration of the concept mapping application is shown in Figure 2. Both groups conducted post-tests to gauge their comprehension of the concepts in nano science and nano technology after the treatment.
To explore the impact of CM on students’ misconceptions about nanotechnology, nanoscale, and classification of nanomaterials, the data as-obtained were analyzed using a principal statistical data analysis involving repeated measures, a paired-sample t-test, and an independent-sample t-test. The paired-sample t-test was conducted to find the difference between the mean scores before and after the same group tested, and the independent-sample t-test was used to find the mean score difference between two independent groups. These statistics were used because the study variables were continuous, observations/tests were independent, variables were normally distributed with no outliers, and both groups were independent. Cohen’s d value is a convenient way to calculate the effect size of independent variable vs. a dependent variable. A “d” of 1 indicates that both groups differ by one standard deviation, and a “d” of 2 indicates that both groups differ by two standard deviations, and so on (Nima et al., 2020).
The SOLO taxonomy provides an extremely valuable framework for understanding and quantifying learning outcomes. Using the SOLO taxonomy, teachers designed effective assessments and instructional strategies that helped students develop a deep understanding of the topics they were studying. The knowledge of different students was evaluated through tasks, assignments, quizzes and tests on content related to nanotechnology, nanoscale and classification of nanomaterials. We got some alarming results and found that students have some serious, well-known misconceptions about nanotechnology, nanoscale and nanomaterials. Details of their misunderstandings are presented through a concept map. The students said that the 3D nanomaterials have three dimensions in nano-range and the 2D nanomaterials have two dimensions in nano-range. Similarly, following this conceptual approach, students think that 1D and 0D nanomaterials are those materials that, respectively, have 1 and 0 dimension in nano-range. In their concepts, the larger objects like stuff around us including books, chairs, trees and even buildings are composed of 0D materials as their no (0) dimension is in nano-range. These concepts are dead wrong in terms of the true scientific understanding of nanotechnology and nanomaterials. These misconceptions about the classification of nanomaterials have been illustrated in Figure 3.
Figure 3. Concept map for misconception and correct conception about nanotechnology, nanoscale, and nonmaterial.
Students believe that the nanoscale is a very small scale that we can barely see with the naked eye. For example, there are small grains of sand or very fine and small particles of wheat flour or much smaller particles of soil that we can see with the naked eye. In their concepts, such materials are 3D nanomaterials because all three dimensions are very small (at nanoscale). Similarly, various students think the very thinner human hairs and versatile fibers of similar thickness/radius are composed of 2D materials as their 2 dimensions are remarkably smaller (considered in nano-range). However, we also found some students who did not have any misconception about such materials and say that the materials which have 2 dimensions in nanoscale, are 1 dimensional material like very thinner fibers whose diameter is in nano-range.
When students were asked about the differences between nanoparticles and quantum dots. A group of students believe that there is no difference between quantum dots and nanoparticles. They think all nanoparticles are quantum dots. However, the other group of students thinks that the quantum dots are extraordinarily smaller (as small as we can think of) than nanoparticles. In addition, they believe that quantum dots are very rare and extremely difficult to prepare. The found misconceptions about classification of nonmaterial and the size of quantum dots are schematically illustrated in Figure 3.
Almost all students have the same misconceptions about the topics. We then developed an achievement test. A pre-test and post-test was carried out in both groups.
The table provides information about the means, standard deviations, t-values, degrees of freedom (df), and significance levels (Sig.) for the pre-test and post-test scores of the experimental and control groups. For the experimental group, the mean score on the pre-test was 12.68 with a standard deviation of 0.989. The t-value of −28.625 indicates a significant difference between the pre-test and post-test scores. The degrees of freedom (df) are 34, and the value of p (Sig.) is 0.000, suggesting a highly significant difference. The note indicates that the significance level (p) is set at 0.05, and the sample size (n) is 35. This means that a value of p below 0.05 is considered statistically significant.
For the experimental and control group, the pre-test mean score was 12.68and 12.53 with a standard deviation of 0.989 and 0.959. The t-value of 0.610 indicates a small difference between the pre-test scores of experimental and control group. The degrees of freedom (df) are 68, and the value of p (Sig.) is 0.526, which suggests that the difference is not statistically significant.
For the experimental and control group, the post-test mean score was 20.33 and 12.57 with a standard deviation 1.341 and 0.940. The t-value of 32.350 indicates a large and highly significant difference between the pre-test and post-test scores. The value of p (Sig.) is 0.000, indicating a statistically significant improvement. The effect size (Cohen’s d) is 7.5, which signifies a very large effect.
Information provided in the Tables 2 3 is about comparison regarding understanding of nano technology of experimental and control groups before and after the test. The values showed that the experimental group performed better in post-test scores. This improved score of the experimental group in the post-test to resolve the misconceptions related to nanotechnology, nanoscale and classification of nonmaterial was predicted due to the intervention of CM-CCT. The conclusions of Tables 2 3 have also been presented in Figure 4 to evidence the significance of CM-CCT as constructivist approach at the glance.
Table 3. Comparison of independent groups on the basis of pre-test and post-test scores for achievement test.
Figure 4. Comparison of student-ratio with misconceptions at pre- and post-test stages, and impact of CM-CCT as constructivist approach on elimination of misconceptions.
The CM-CCT as constructivist tool is widely used in various educational settings, particularly in science classrooms, where learners often have misconceptions that need to be dispelled in order to develop accurate scientific understanding. It is recognized that changing misconceptions is a complex process that requires active engagement, reflection and the reconstruction of learners’ mental models. In such crucial situations, we planned to eliminate these misunderstandings among the students and applied the CM-CCT as constructivist tool. By applying this approach, we have imparted the real knowledge and eliminated the students’ misconceptions about nanotechnology, nanoscale and nonmaterial. In teaching nanotechnology through conceptual change texts and CM, we have also used a context-based approach as it is believed to be effective in eliminating student alternative ideas and/or misunderstandings as it helps students to relate scientific concepts to context in daily life to connect.
By applying the concept maps and conceptual change texts as constructivist tools, we made clear to the students that nanotechnology is the study of certain materials or things at the nanoscale (1 nm = 10–9 m). To get an idea of how small the nanoscale is, let us look at an example. We consider our Earth which is ~12,742 km or 12,742,000 m in size (diameter). We also have a football with a size (diameter) of22 cm. Now, we half the size of earth and half the size of ball. We keep halving these two until the size of football becomes 22 nm (reduced 10 million times). Interestingly, in this state, the size of our giant Earth will become 1.2742 m, just about five times of the football. This example illustrates how small a nanometer and thus the nanoscale is. This concept is also depicted through a CM-CCT in Figure 5.
This way we made the students realize that the nanoscale is so much smaller! We corrected their misconception that the small particles that have all three dimensions are insignificant at the nanoscale are 0D materials, and not 3D materials. Likewise, the materials that have one dimension and two dimensions insignificant at nanoscale are, respectively, 1D and 2D materials, not 2D and 1D material. Furthermore, materials where no dimension is insignificant or nanoscale are not nanomaterial; they are bulk materials and scientifically and technically there are not 3D nanomaterial. Moreover, depending on the size of the materials, nanoparticles a few nm in size (approximately 1–10 nm) are called quantum dots (these are typically semiconductor materials), and the other particles larger than this and smaller than 100 nm are considered as nanoparticles.
To inspect the performance of CM-CCT, the interviews of students were conducted to find out the experiences and learning after the interventions. Following are the reflections of students about the studied misconceptions.
When the students were asked about the misconception in nanotechnology, following response was notable:
“I was inspired but confused about the nanotechnology before the interventions. However, now I understand well about it through CM-CCT. These helped me fruitfully to know more about nanoscale study in nanotechnology” P5.
Following response of a student was received about the misconception in nanoscale:
“I thought the small scale like millimeter or sub-millimeter will be equivalent to nanoscale. However, I was totally wrong and my misconception was eliminated when I was taught using CM-CCT approaches that it is the scale of billionth of a meter” P6.
Following response was also markable:
“In my understanding, the small objects like small particles and wheat flour grains would be the nanoparticles. However, I was misunderstanding the nanomaterials. Our teaching through CM-CCT helped me a lot to clearly understand the scale of nanomaterials. Actually, the individual nanoparticles are invisible to human eye and these are of the scale of millionth of a millimeter” P12.
The SOLO Taxonomy, developed by Biggs and Collis, serves as a valuable framework for analyzing the depth of understanding and cognitive complexity exhibited in students’ responses. In the context of our study, the taxonomy was employed as a systematic tool to categorize and assess the identified misconceptions related to nanoscale science and technology. The primary purpose of utilizing the SOLO Taxonomy was to go beyond a mere identification of misconceptions and delve into the nuanced levels of cognitive engagement exhibited by students. The taxonomy classifies responses into different levels of understanding, ranging from a surface level of knowledge to a deep conceptualization. By doing so, it allowed us to not only identify misconceptions but also to discern the depth and nature of these misconceptions within the cognitive framework.
Based on the findings from the analyses, it can be deduced that the incorporation of concept mapping instruction alongside conceptual change texts led to a notably enhanced grasp of scientific concepts and a reduction in alternative conceptions when compared to the more conventional instructional approach. These conceptual change texts were crafted with the aim of encouraging students to reflect on both their existing knowledge and scientific concepts, fostering the essential conditions for conceptual change as per the following (Putri et al., 2022a), To elucidate the reasons behind the inaccuracy of commonly held beliefs and offer accurate explanations of concepts, the approach involved triggering students’ prior knowledge and dispelling any misconceptions. In contrast, traditional instruction neglected the consideration of these misconceptions. The conceptual change texts, designed with the awareness of these misconceptions, aimed to employ methods of conceptual transformation. This approach facilitated the acquisition of new understandings by fostering the exchange, differentiation, and integration of existing concepts with new conceptions. When students read and discussed the text in class, it led to shifts in their ontological categories.
Concept maps were used to show the knowledge gaps for pupils who were unable to make the right connections. Unique learning environments were constructed using the conceptual transformation approach. In these settings, misconceptions about nanoscience were identified, students’ misconceptions were sparked by straightforward qualitative examples, descriptive evidence was provided in the text to refute common misconceptions, the situation was explained scientifically, and students were given the chance to practice giving the right answers through questions. Therefore, it can be concluded that students receiving traditional science training are less likely to learn scientific concepts because persistent misconceptions exist inside their conceptual framework.
Concept maps can reveal complex understanding and misconceptions in science education, help make ideas transparent, and illustrate conceptual change at both gross and specific levels, while highlighting personal relevance and activating prior knowledge for further learning (Kinchin, 2000). According to Edmondson and Smith (1996) the necessity of assisting students in amalgamating their knowledge and emphasized the utility of CM in rendering explicit conceptual connections, pinpointing errors and omissions, and exposing misconceptions. Concept mapping is recommended as an assessment or research method in science education due to its unique ability to visualize the structure of students’ conceptual achievements, according to three case studies that examined approaches to conceptual acquisition in science education (Soika and Reiska, 2014). According to Kaya (2008) the results of an investigation into the viability and validity of using CM as authentic assessment tools in a student-centered approach to describe the changes in prospective science teachers’ (PSTs’) conceptual understanding during general chemistry laboratory investigations showed positive views of their assessment practices in the laboratory course, providing pedagogical implications for the preparation of science teachers.
The current study showed how the newly developed instructional paradigm known as conceptual change texts and CM as constructivist tool helped students understand and accurately conceptualize scientific ideas. The experimental group’s degree of student comprehension of nanotechnology increased significantly. The purpose of concept map and conceptual change texts as constructivist tool was to give teachers and students another alternative when it came to lesson planning, which was similar to Kocakülah and Kural (2016) recommendation of a new teaching model called Teaching Model for Hot Conceptual Change (TMHCC), which comprises eight phases of learning syntaxes to encourage conceptual change.
The acquisition of 21st century abilities has been regarded as being facilitated by conceptual shift as a viable teaching strategy (Djudin, 2021) According to Strike and Posner (1982), relying on Posner as the main proponent of conceptual change theory, explained that for conceptual change to occur, four conditions must be met: (1) dissatisfaction with the (initial) conception that is owned; (2) the new concept is easier to understand; (3) the new concept is more plausible; and (4) the new concept is felt to be useful (fruitful). All of these conceptual change criteria have been taken into account by CM and conceptual change texts as constructivist tool, as shown in the various levels of instruction and in the students’ improved comprehension and altered concepts. CM-CCT specifically emphasized the significance of identifying the contributions that students make to the classroom. To encourage conceptual understanding, it is essential to acknowledge students’ past knowledge (Dorsah and Okyer, 2020). Additionally, it is crucial to keep in mind that in order for students to completely grasp the lesson, the activities must be successful in piquing their interest and making them want to engage in the discussion rather than just listen to the teacher (Slater and Gelderman, 2017).
The findings of the showed greater satisfaction with the fact that the CM-CCT as constructivist tool featured differentiated educational activities (Bakouli and Jimoyiannis, 2014). The reflection step may also be one of the both’s key features for encouraging conceptual change. The similar claim about the benefits of a constructivist approach in teaching science topics was made by Antonio and Prudente (2021).
The goal of employing instructional materials is for students to integrate the knowledge offered to them. As a result, CM-CCT presented students with a tool that allowed them to fully comprehend the concept of nanotechnology while also having fun. This study, however, does not claim that conceptual change growth occurred in a linear fashion in accordance with the stages of the instructional tools. Individual students’ conceptual change occurs at any level of their learning, and it may proceed gradually or more slowly than predicted given that the period of instruction is restricted (Hoppe and Gaβner, 2023). According to Djudin (2021) the same disclaimer about the emergence of conceptual change in learners, stating that even when conceptual change occurs, individuals do not entirely forsake their earlier notions. The fact that this study was able to confirm that CM-CCT as constructivist approach can improve conceptual knowledge and conceptual transformation among students is critical.
In order to thoroughly assess the efficacy of Concept Map (CM) and Conceptual Change Texts (CCT) as an instructional tools in resolving misconceptions among undergraduate university students about nanoscale science and technology concepts, we used a variety of approaches in this study. First, we applied the Structure of Observed Learning Outcomes (SOLO) Taxonomy to create a baseline knowledge of students’ misconceptions. We were able to recognize and classify misconceptions prior to the intervention, which also gave us a clear picture of the scope and of the conceptual difficulties that the students were facing. A crucial aspect of our research involved the intervention design, which involved applying conceptual change texts and concept maps to the experimental group. This design ensures a targeted and concentrated instructional approach by precisely addressing the misunderstandings found by the SOLO Taxonomy.
In our evaluation, quantitative analysis was essential. To compare the pre- and post-test results between the experimental and control groups, we used independent sample t-tests. We were able to ascertain the significance of the observed differences and conduct a thorough evaluation of the intervention’s efficacy. As a standardized measure of the effect size, we computed Cohen’s d values to quantify the practical relevance of the observed changes. This metric improved our capacity to understand the practical implications of our findings by offering a useful indicator of the extent of change brought about by the intervention. We used qualitative information from students’ interviews in addition to quantitative measurements. Students in the experimental group were able to consider how the intervention affected their conceptual knowledge. The inclusion of a qualitative component enhanced the overall interpretation of the study outcomes by providing additional context and depth to our findings. We sought to ensure a comprehensive and reliable assessment of the concept’s efficacy by combining these various sources of evidence—using the SOLO Taxonomy to identify baselines, putting in place a targeted intervention, carrying out meticulous statistical analyses, calculating effect sizes, and obtaining qualitative reflections through interviews. We believe that taking a diverse strategy to teaching nanoscale science and technology ideas to undergraduate university students strengthens the validity and reliability of our conclusion that both concept maps and conceptual change texts are useful teaching tools. Our study offers insightful information to educators and researchers in the field, highlighting the significance of using a thorough methodology to assess the effectiveness of educational interventions.
Here are some recommendations for future research:
• Longitudinal studies may be conducted to examine the long-term effects of implementing the CM-CCT as constructivist tool in teaching nanoscale science and technology concepts to students. This would help determine whether the conceptual changes achieved by the CM-CCT as constructivist tool are sustainable over time. Generalizability may be investigated of the CM-CCT as constructivist tool across different age groups and educational settings. Its effectiveness may be assessed in teaching nanoscale science and technology concepts to students in diverse populations and cultural contexts.
• may be training programs for science teachers to explore the effective implementation of the constructivist tools. Examine how science teachers’ knowledge, attitudes and instructional practices affect the outcomes of using these instructional tools to students.
• A mixed-methods research design may be employed that combines qualitative and quantitative measures to provide a comprehensive understanding of the effectiveness and implementation of the above stated instructional tools. This could include collecting both quantitative data on learning outcomes and qualitative data on students’ experiences, perceptions and attitudes toward the instructional approach.
• Investigation may be done to the integration of technology tools and digital resources into the instructional tools to improve engagement and learning outcomes of students. Discovery may be done for the potential of multimedia materials, simulations, and virtual labs to support conceptual changes in nanoscale science and technology education.
• Considering these research recommendations may provide additional insights into the effectiveness, generalizability, and instructional strategies associated with the CM-CCT as constructivist approach in teaching nanoscale science and technology concepts to students. These insights can help refine and improve STEM educational practices for students with diverse learning needs.
The original contributions presented in the study are included in the article/supplementary material, further inquiries can be directed to the corresponding authors.
MN: Conceptualization, Data curation, Validation, Writing – original draft, Investigation, Writing – review & editing. AS: Data curation, Writing – review & editing. ZU: Data curation, Software, Conceptualization, Validation, Writing – original draft. SR: Conceptualization, Methodology, Software, Writing – review & editing. SW: Methodology, Software, Writing – review & editing. MS: Funding acquisition, Resources, Software, Writing – review & editing. IE: Formal Analysis, Funding acquisition, Resources, Writing – review & editing. SK: Data curation, Formal Analysis, Methodology, Writing – review & editing. SQ: Methodology, Project administration, Supervision, Writing – review & editing. MF: Funding acquisition, Supervision, Writing – original draft, Writing – review & editing.
The author(s) declare financial support was received for the research, authorship, and/or publication of this article. The authors extend their appreciation to the King Salman center For Disability Research for funding this work through Research Group no KSRG-2023-267.
The authors declare that the research was conducted in the absence of any commercial or financial relationships that could be construed as a potential conflict of interest.
The author(s) declared that they were an editorial board member of Frontiers, at the time of submission. This had no impact on the peer review process and the final decision.
All claims expressed in this article are solely those of the authors and do not necessarily represent those of their affiliated organizations, or those of the publisher, the editors and the reviewers. Any product that may be evaluated in this article, or claim that may be made by its manufacturer, is not guaranteed or endorsed by the publisher.
Addido, J., Burrows, A. C., and Slater, T. F. (2022). Addressing pre-service teachers' misconceptions and promoting conceptual understanding through the conceptual change model. Prob Educ 21st Century 80, 499–515. doi: 10.33225/pec/22.80.499
Amineh, R. J., and Asl, H. D. (2015). Review of constructivism and social constructivism. J Soc Sci Lit Lang 1, 9–16.
Antonio, R. P., and Prudente, M. S. (2021). Metacognitive argument-driven inquiry in teaching antimicrobial resistance: effects on students’ conceptual understanding and argumentation skills. J Turk Sci Educ 18, 192–217. doi: 10.36681/tused.2021.60
Arabeyyat, Z. H., Jamaliah, M. M., and Khalaf, M. A. (2022). Public awareness of nanotechnology and its implications for health in Jordan. Sustain. For. 14:5786. doi: 10.3390/su14105786
Bakouli, V., and Jimoyiannis, A. (2014). Concept mapping as cognitive tool in science education: an analysis of students’ learning using SOLO taxonomy. In: 1st international conference on new developments in science and technology education, Corfu, Greece.
Balci, S., Cakiroglu, J., and Tekkaya, C. (2006). Engagement, exploration, explanation, extension, and evaluation (5E) learning cycle and conceptual change texts as learning tools. Biochem. Mol. Biol. Educ. 34, 199–203. doi: 10.1002/bmb.2006.49403403199
Barnett, T., Pearson, A. W., Pearson, R., and Kellermanns, F. W. (2015). Five-factor model personality traits as predictors of perceived and actual usage of technology. Eur. J. Inf. Syst. 24, 374–390. doi: 10.1057/ejis.2014.10
Bartolucci, C., Scognamiglio, V., Antonacci, A., and Fraceto, L. F. (2022). What makes nanotechnologies applied to agriculture green? Nano Today 43:101389. doi: 10.1016/j.nantod.2022.101389
Becerra, G., and Castorina, J. (2018). Towards a dialogue among constructivist research programs. Construct Found 13, 191–198.
Beerenwinkel, A., and von Arx, M. (2017). Constructivism in practice: an exploratory study of teaching patterns and student motivation in physics classrooms in Finland, Germany and Switzerland. Res. Sci. Educ. 47, 237–255. doi: 10.1007/s11165-015-9497-3
Biggs, J., and Collis, K. (1989). Towards a model of school-based curriculum development and assessment using the SOLO taxonomy. Aust. J. Educ. 33, 151–163. doi: 10.1177/168781408903300205
Blonder, R., and Mamlok-Naaman, R. (2019). Teaching chemistry through contemporary research versus using a historical approach. Chem Teach Int 2:20180011. doi: 10.1515/cti-2018-0011
Candy, P. C. (1989). Constructivism and the study of self-direction in adult learning. Stud Educ Adults 21, 95–116. doi: 10.1080/02660830.1989.11730524
Cetin, G., Hamide, E., and Omer, G. (2015). Effects of conceptual change texts based instruction on ecology, attitudes toward biology and environment. Educ. Res. Rev. 10, 259–273. doi: 10.5897/ERR2014.2038
Curreli, M., and Rakich, S. S. (2020). An integrated approach to teaching and learning nanotechnology: the Omni Nano model. J Res Innov Teach Learn 13, 141–145. doi: 10.1108/JRIT-02-2020-0012
Djudin, T. (2021). Promoting students’ conceptual change by integrating the 3-2-1 reading technique with refutation text in the physics learning of buoyancy. J Turk Sci Educ 18, 290–303. doi: 10.36681/tused.2021.66
Dorsah, P., and Okyer, M. (2020). Cultural factors affecting the teaching and learning of some science concepts. Eur J Educ Stud 7:3159. doi: 10.46827/ejes.v7i7.3159
Durmuş, J., and Bayraktar, Ş. (2010). Effects of conceptual change texts and laboratory experiments on fourth grade students’ understanding of matter and change concepts. J. Sci. Educ. Technol. 19, 498–504. doi: 10.1007/s10956-010-9216-9
Edmondson, K. M., and Smith, D. F. (1996). Concept mapping to facilitate veterinary Students' understanding of fluid and electrolyte disorders. American Educational Research Association.
Fatimah, S., Rosidin, D. N., and Hidayat, A. (2022). Student-based learning in the perspective of constructivism theory and Maieutics method. Int J Soc Sci Hum Res 5, 1632–1637. doi: 10.47191/ijsshr/v5-i5-10
Gafoor, K. A., and Akhilesh, P. (2010). Strategies for facilitating conceptual change in school physics. Innov Res Educ 3, 34–42.
Ghani, I. A., Ibrahim, N., Yahaya, N., and Surif, J. (2017). Enhancing students' HOTS in laboratory educational activity by using concept map as an alternative assessment tool. Chem Educ Res Pract 18, 849–874. doi: 10.1039/C7RP00120G
Gill, M., Trevors, G., Greene, J., and Algina, J. (2022). Don’t take it personally? The role of personal relevance in conceptual change. J. Exp. Educ. 90, 1–22. doi: 10.1080/00220973.2020.1754152
Ginting, R., Maryanto, M., Menarianti, I., and Buchori, A. (2019). Similarity: Implementation interactive whiteboard media with constructivism approach to public policy courses. J Engineer Appl Sci 14, 7248–7253. doi: 10.36478/jeasci.2019.7248.7253
Gordon, M. (2009). Toward a pragmatic discourse of constructivism: reflections on lessons from practice. Educ. Stud. 45, 39–58. doi: 10.1080/00131940802546894
Groth, R. (2003). High school students levels of thinking in regard to statistical study design. Mathematics Education Research Journal, 15, 252–268.
Hamamoto Filho, P. T., Silva, E., Ribeiro, Z. M. T., Hafner, M., Cecilio-Fernandes, D., and Bicudo, A. M. (2020). Relationships between Bloom’s taxonomy, judges’ estimation of item difficulty and psychometric properties of items from a progress test: a prospective observational study. Sao Paulo Med. J. 138, 33–39. doi: 10.1590/1516-3180.2019.0459.r1.19112019
Hook, P., and Mills, J. (2011). SOLO Taxonomy: A Guide for Schools. A Common Language of Learning. Essential Resources.
Hoppe, H. U., and Gaβner, K. (2023). Integrating collaborative concept mapping tools with group memory and retrieval functions. Computer Support for Collaborative Learning. (pp. 716–725). Routledge.
Huang, H. M. (2002). Toward constructivism for adult learners in online learning environments. Br. J. Educ. Technol. 33, 27–37. doi: 10.1111/1467-8535.00236
Katagall, R., Dadde, R., Goudar, R., and Rao, S. (2015). Concept mapping in education and semantic knowledge representation: an illustrative survey. Proc Comput Sci 48, 638–643. doi: 10.1016/j.procs.2015.04.146
Kaufman, D. (2004). 14. Constructivist issues in language learning and teaching. Annu. Rev. Appl. Linguist. 24, 303–319. doi: 10.1017/S0267190504000121
Kaya, O. N. (2008). A student-centred approach: assessing the changes in prospective science teachers’ conceptual understanding by concept mapping in a general chemistry laboratory. Res. Sci. Educ. 38, 91–110. doi: 10.1007/s11165-007-9048-7
Kinchin, I. M. (2000). Using concept maps to reveal understanding: a two-tier analysis. Sch. Sci. Rev. 81, 41–46.
Kocakülah, M. S., and Kural, M. (2016). The effect of Compton scattering teaching based on hot conceptual change on Students'conceptual change. J Educ Instruct Stud World. 6, 78–90.
Konicek-Moran, R., and Keeley, P. (2015). Teaching for conceptual understanding in science. NSTA Press, Arlington.
Koohang, A., Paliszkiewicz, J., Nord, J. H., and Ramim, M. (2014). Advancing a theoretical model for knowledge construction in e-learning. Online J Appl Knowl Manage 2, 12–25.
Koolen, C. D., Torrent, L., Agarwal, A., Meili-Borovinskaya, O., Gasilova, N., Li, M., et al. (2022). High-throughput sizing, counting, and elemental analysis of anisotropic multimetallic nanoparticles with single-particle inductively coupled plasma mass spectrometry. ACS Nano 16, 11968–11978. doi: 10.1021/acsnano.2c01840
Madaiton, N., Tomaquin, M. E., Visitacion, E. J., Villaver, J. R., Malingin, J. M., Nacua, S., et al. (2022). Conceptual change framework of instruction (CCFI): an instructional model in teaching eclipses. J Turk Sci Educ 19, 622–640. doi: 10.36681/tused.2022.141
Mahmood, A., Mahmood, S., and Butt, M. W. (2020). Comparative study of English textbook I of Federal and Khyber Pakhtunkhwa Boards at SSC Level: Application of Bloom’s taxonomy. Kashmir Journal of Language Research, 23, 1–2.
Maryam, A., Mohammadreza, D., Abdolhussein, S., Ghobad, R., and Javad, K. (2021). Effect of concept mapping education on critical thinking skills of medical students: a quasi-experimental study. Ethiop. J. Health Sci. 31, 409–418. doi: 10.4314/ejhs.v31i2.24
Mills, S. (2016). Conceptual understanding: a concept analysis. Qual. Rep. 21, 546–557. doi: 10.46743/2160-3715/2016.2308
Murphy, K. L., Mahoney, S. E., Chen, C. Y., Mendoza-Diaz, N. V., and Yang, X. (2005). A constructivist model of mentoring, coaching, and facilitating online discussions. Distance Educ. 26, 341–366. doi: 10.1080/01587910500291454
Mushtaq, M. W., Kanwal, F., Batool, A., Jamil, T., Zia-ul-Haq, M., Ijaz, B., et al. (2017). Polymer-coated CoFe2O4 nanoassemblies as biocompatible magnetic nanocarriers for anticancer drug delivery. J. Mater. Sci. 52, 9282–9293. doi: 10.1007/s10853-017-1141-3
Mushtaq, M. W., Kanwal, F., Imran, M., Ameen, N., Batool, M., Batool, A., et al. (2018). Synthesis of surfactant-coated cobalt ferrite nanoparticles for adsorptive removal of acid blue 45 dye. Mater Res Exp 5:035058. doi: 10.1088/2053-1591/aab6a4
Najeeb, J., Farwa, U., Ishaque, F., Munir, H., Rahdar, A., Nazar, M. F., et al. (2022). Surfactant stabilized gold nanomaterials for environmental sensing applications – a review. Environ. Res. 208:112644. doi: 10.1016/j.envres.2021.112644
Nazar, M. F., Saleem, M. A., Basharat, H., Nasrullah, A., Asif, H., Ashfaq, M., et al. (2021). Architecting water-dispersible organic nanopowder from volatile microemulsion: an emerging colloidal technology. Colloid Interface Sci Commun 45:100536. doi: 10.1016/j.colcom.2021.100536
Nima, A. A., Cloninger, K. M., Persson, B. N., Sikström, S., and Garcia, D. (2020). Validation of subjective well-being measures using item response theory. Front. Psychol. 10:3036. doi: 10.3389/fpsyg.2019.03036
Novak, J. D., and Cañas, A. J. (2008). The theory underlying concept maps and how to construct and use them. Florida Institute for Human and Machine Cognition (IHMC).
Nugroho, W. (2021). Analisis Kesalahan Siswa Dalam Penyelesaian Soal Kaidah Pencacahan. Aritmatika 2, 32–46. doi: 10.35719/aritmatika.v2i1.18
Nurhidayat, W., Surahman, E., and Sujarwanto, E. (2023). The effect of conceptual understanding procedures learning model on Students' higher level thinking skills. J Pend Indon 12, 386–394. doi: 10.23887/jpiundiksha.v12i2.58709
Putri, A. H., Samsudin, A., Purwanto, M. G., and Suhandi, A. (2022a). Examination of conceptual change research over a decade: a bibliometric analysis using science mapping tool. Indones J Learn Adv Educ 4, 171–190. doi: 10.23917/ijolae.v4i3.18249
Putri, A. H., Samsudin, A., and Suhandi, A. (2022b). Exhaustive studies before Covid-19 pandemic attack of students’ conceptual change in science education: a literature review. J Turk Sci Educ 19:151. doi: 10.36681/tused.2022.151
Quirola, N., Marquez, V., Tecpan, S., and Baltazar, S. E. (2018). Didactic proposal to include nanoscience and nanotechnology at high school curriculum linking physics, chemistry and biology. J. Phys. Conf. Ser. 1043:012050. doi: 10.1088/1742-6596/1043/1/012050
Rahmatya, O., Susilawati, S., and Erna, M. (2019). Development of constructivism-based student worksheets on free radical reaction material. J Educ Sci 3, 162–173. doi: 10.31258/jes.3.2.p.162-173
Rap, S., Feldman-Maggor, Y., Aviran, E., Shvarts-Serebro, I., Easa, E., Yonai, E., et al. (2020). An applied research-based approach to support chemistry teachers during the COVID-19 pandemic. J. Chem. Educ. 97, 3278–3284. doi: 10.1021/acs.jchemed.0c00687
Richards, D., Atif, A., and Busch, P. (2012). Towards an ontology-based approach to knowledge management of graduate attributes in higher education. n Knowledge Management and Acquisition for Intelligent Systems: 12th Pacific Rim Knowledge Acquisition Workshop, PKAW 2012, Kuching, Malaysia, September 5-6, 2012. Proceedings 12 (229–243). Berlin Heidelberg: Springer.
Rudolf, R., Majerič, P., Štager, V., and Golub, D. (2020). Nanotechnology as the technology of the future, nanowaste problems. Anali PAZU 10, 41–44.
Sakhnini, S., and Blonder, R. (2018). Insertion points of the essential nanoscale science and technology (NST) concepts in the Israeli middle school science and technology curriculum. Nanotechnol. Rev. 7, 373–391. doi: 10.1515/ntrev-2018-0026
Sakti, A., Jufri, J., and Abdul Gani, H. (2020). The development of learning models of speaking skills based on integrative-Constructivic approach on elementary students. Solid State Technol 63, 8911–8921.
Sannathimmappa, M. B., Nambiar, V., Aravindakshan, R., and Kumar, A. (2022). Are online synchronous team-based-learning (TBL) pedagogy effective?: perspectives from a study on medical students in Oman. J. Adv. Med. Educ. Prof. 10, 12–21. doi: 10.30476/JAMP.2021.92361.1481
Sidabutar, R., Napitupulu, S., and Sauduran, G. N. (2023). The effect of constructivism learning approach on Students' mathematics learning outcomes in material relations and functions of grade VIII SMP Negeri 11 Pematangsiantar. EduMatika 3, 15–19.
Slater, T. F., and Gelderman, R. (2017). Addressing students’ misconceptions about eclipses. Physiol. Teach. 55, 314–315. doi: 10.1119/1.4981046
Slater, E. V., Morris, J. E., and McKinnon, D. (2018). Astronomy alternative conceptions in pre-adolescent students in Western Australia. Int. J. Sci. Educ. 40, 2158–2180. doi: 10.1080/09500693.2018.1522014
Slieman, T. A., and Camarata, T. (2019). Case-based group learning using concept maps to achieve multiple educational objectives and behavioral outcomes. J. Med. Educ. Curric. Dev. 6:238212051987251. doi: 10.1177/2382120519872510
Soika, K., and Reiska, P. (2014). Using concept mapping for assessment in science education. J. Balt. Sci. Educ. 13, 662–673. doi: 10.33225/jbse/14.13.662
Song, M., and Long, Y. (2022). Scientometric review of transition metal oxides for hydrogen energy production. Energy Sourc Part A Recov Util Environ Effects 44, 3720–3734. doi: 10.1080/15567036.2022.2069884
Strike, K. A., and Posner, G. J. (1982). Conceptual change and science teaching. Eur. J. Sci. Educ. 4, 231–240. doi: 10.1080/0140528820040302
Sungur, S., Tekkaya, C., and Geban, Ö. (2001). The contribution of conceptual change texts accompanied by concept mapping to students' understanding of the human circulatory system. Sch. Sci. Math. 101, 91–101. doi: 10.1111/j.1949-8594.2001.tb18010.x
Teaching and Educational Development Institute, University of Queensland . (2015). Biggs’ structure of the observed learning outcome (SOLO) taxonomy. Available at: http://www.tedi.uq.edu.au/downloads/biggs_solo.pdf
Thompson, E., Whalley, J., Lister, R. F., Carbone, A., Hu, M., Sheard, J., et al. (2007). Reliably classifying novice programmer exam responses using the SOLO taxonomy. National Advisory Committee on Computing Qualifications.
Vosniadou, S., and Mason, L. (2012). Conceptual change induced by instruction: a complex interplay of multiple factors. APA Educ Psychol Handb 2, 221–246. doi: 10.1037/13274-009
Yolcua, H. H., and Dyehouse, M. A. (2018). Engineering major Students' perceptions of nanotechnology. Int. J. Progress. Educ. 14, 37–51. doi: 10.29329/ijpe.2018.154.4
Keywords: misconceptions, conceptual change text, nanotechnology, nanomaterial, constructivism, concept maps
Citation: Naeem Sarwar M, Shahzad A, Ullah Z, Raza S, Wasti SH, Shrahili M, Elbatal I, Kulsoom S, Qaisar S and Faizan Nazar M (2024) Concept mapping and conceptual change texts: a constructivist approach to address the misconceptions in nanoscale science and technology. Front. Educ. 9:1339957. doi: 10.3389/feduc.2024.1339957
Received: 17 November 2023; Accepted: 29 January 2024;
Published: 22 February 2024.
Edited by:
Gladys Sunzuma, Bindura University of Science Education, ZimbabweReviewed by:
Seamus Delaney, Deakin University, AustraliaCopyright © 2024 Naeem Sarwar, Shahzad, Ullah, Raza, Wasti, Shrahili, Elbatal, Kulsoom, Qaisar and Faizan Nazar. This is an open-access article distributed under the terms of the Creative Commons Attribution License (CC BY). The use, distribution or reproduction in other forums is permitted, provided the original author(s) and the copyright owner(s) are credited and that the original publication in this journal is cited, in accordance with accepted academic practice. No use, distribution or reproduction is permitted which does not comply with these terms.
*Correspondence: Zaka Ullah, emFrYS51bGxhaEB1ZS5lZHUucGs=; Muhammad Faizan Nazar, ZmFpemFuLm5hemFyQHVlLmVkdS5waw==
†ORCID: Zaka Ullah https://orcid.org/0000-0002-2321-8323
Disclaimer: All claims expressed in this article are solely those of the authors and do not necessarily represent those of their affiliated organizations, or those of the publisher, the editors and the reviewers. Any product that may be evaluated in this article or claim that may be made by its manufacturer is not guaranteed or endorsed by the publisher.
Research integrity at Frontiers
Learn more about the work of our research integrity team to safeguard the quality of each article we publish.