- 1The Interdisciplinary Center of Educational Innovations, University of Latvia, Riga, Latvia
- 2Department of Learning, KTH Royal Institute of Technology, Stockholm, Sweden
- 3School of Electrical and Electronic Engineering, Technological University Dublin, Dublin, Ireland
- 4Science and Engineering Education Group, Delft University of Technology, Delft, Netherlands
- 5College of Engineering and Applied Science, University of Cincinnati, Cincinnati, OH, United States
- 6Department of Mathematics, Delft University of Technology, Delft, Netherlands
- 7Academic Affairs – City Campus, Technological University Dublin, Dublin, Ireland
- 8School of Mechanical and Design Engineering, Technological University Dublin, Dublin, Ireland
- 9Faculty of Engineering and Informatics, Technological University of the Shannon: Midlands Midwest, Athlone, Ireland
Extensive research has established that spatial ability is a crucial factor for achieving success in Science, Technology, Engineering, and Mathematics (STEM). However, challenges that educators encounter while teaching spatial skills remain uncertain. The purpose of this study is to develop a research framework that examines the interrelationships, barriers, and enablers amongst various educational components, including schools, teachers, students, classrooms, and training programs, that are encountered when teaching for spatial ability development. A thorough examination of international research, in combination with a detailed review of the primary Science and Mathematics curricula in Ireland, Latvia, Sweden, and the Netherlands, is undertaken to acquire a more concentrated comprehension of the incorporation of spatial components in the curriculum. The review seeks to establish the fundamental factors that enable or hinder teachers in terms of curriculum, pedagogy, pedagogical content knowledge, and spatialized classroom practices.
1 Introduction
Spatial ability is a fundamental cognitive ability that is critical in both educational and everyday contexts. It is a manifold factor of intelligence, comprising of several individual spatial factors. These spatial factors, encompass a range of narrow cognitive abilities associated with, for example, comprehending and thinking about space, distance, direction, and object relationships, and reflect a diverse range of mental operations such as mental rotation, folding, and cutting (Uttal et al., 2013; Newcombe and Shipley, 2014; Buckley et al., 2018). Spatial intervention at an early age has garnered increasing attention in educational research due to its profound implications for cognitive development in primary school children. Research on infants indicates that the foundation for cognitive development is built at an early stage, through the development of basic spatial transformation skills (Newcombe and Frick, 2010). Based on longitudinal and cross-sectional data, it has been observed that individuals with better spatial abilities exhibit superior performance in mathematics and science starting from early childhood, in contrast to those with inferior spatial abilities (Mix et al., 2016; Gilligan et al., 2017; Hodgkiss et al., 2018; Gilligan et al., 2019; Bower et al., 2020). Moreover, studies indicate that spatial transformation abilities continue to progress during early childhood. Therefore, interventions targeting various age groups might still greatly influence children’s cognitive development (Newcombe and Frick, 2010).
While there is ample evidence supporting the positive impact of spatial ability on students’ cognitive development, the process of developing spatial ability is intricate due to the interrelated nature of different educational factors, including the curriculum, institution, classroom, teacher, and students. These components themselves have complex inter-relationships as well as several sub-components, influences, and dependents. A narrative map representing a partial account of the complexity of educational environments is depicted in Figure 1.
Given the many components of an educational system that impact an educator’s pedagogical flexibility and capacity to spatialize the curriculum, and thus on students’ associated learning experience, this manuscript aims to contribute in-part to the creation of a spatial ability pedagogical framework to support teaching for spatial ability development with an emphasis on primary level education. There are ongoing efforts across Europe to identify where, within existing national curricula, there is potential for spatial ability development through the use of spatial pedagogies (e.g., Whittaker et al., 2022; Lin et al., 2023). This current work aims to support these efforts by highlighting aspects of the education system which have been examined relative to spatial ability development and have been highlighted as pertinent such that collectively this on-going agenda can first lead to more specific investigations on how spatial ability development should be incorporated into existing educational practice and then to evidence-informed practice recommendations. Importantly, this work has been conducted primarily through a European lens based on the experiences of the research team although international research is reviewed which is considered useful for informing educational practice in Europe. It also is presented in the context of spatializing the existing curriculum (embedded approaches to spatial ability development) as opposed to the integration of supplementary interventions (isolated approaches to spatial ability development) (cf. Hawes et al., 2023; Zhu et al., 2023), although again it is acknowledged that such interventions have elements which can be abstracted and generalized to inform regular practice. The reason for this view is that, in many jurisdictions, curricula are already quite full making it difficult to apply resources specific to the development of spatial ability. As such, the use of isolated interventions is not always possible, and a spatialized curriculum can be more feasible.
Specifically, this report provides a narrative review of the barriers and enablers that influence the components of educational systems which have been related to spatial ability development. A narrative review, unlike a systematic review, does not involve an exhaustive search and consolidation of the extant literature. Instead, the purpose of this manuscript is to use a selection of relevant works to illustrate a narrative account of the facets of primary level mathematics and science education where spatial thinking could be incorporated in general, and to provide an overview of identified barriers and enablers to this potential action. According to Schoepp (2005, p. 57), a barrier is any condition that makes it difficult to make progress or achieve a goal, whereas an enabler is any student and environmental factors that promote the development of academic skills (DiPerna and Elliott, 2002, pp. 294–295). As noted by Doyle et al. (2018), there exist various types of barriers and enablers, including situational and systemic. The inherent characteristics of education, including its structural, cultural, and contextual aspects, present certain challenges that are difficult to overcome. Nevertheless, situational obstacles are more susceptible to alteration.
2 Background: associations between spatial ability and mathematics and science education
The term spatial ability refers to an individual’s cognitive aptitude to mentally visualize, manipulate, and comprehend objects and concepts that possess a spatial dimension (Uttal et al., 2013). Its significance lies in its ability to aid in activities such as problem-solving (Buckley et al., 2018, 2019; Sorby et al., 2020; Rohmah and Indriati, 2021), designing (Türkmenoglu Berkan et al., 2020; Dilling and Vogler, 2021; Darwish et al., 2023), and data analysis (Cook et al., 2002; Rodriguez and Rodriguez-Velazquez, 2020; Arundel and Li, 2021). As shown by Uttal et al. (2013) spatial ability is malleable and can be developed through practice and experience, particularly at a young age. This malleability, coupled with its predictive and causal relationship with STEM education retention and achievement (Sorby et al., 2018), makes spatial ability a particularly auspicious cognitive ability to focus on for those interested in STEM education development.
Whilst generally relevant to STEM education, the role of spatial ability within science and mathematics education is particularly pertinent for this review as the context is to support teaching and learning at the primary school level where many countries do not formally offer dedicated technology and engineering curricula (Buckley, 2023). With respect to mathematics, numerous studies (e.g., Mix et al., 2016, 2017; Verdine et al., 2017; Geer et al., 2019) have examined the relationship between spatial ability and mathematics achievement and revealed a strong correlation between them across all educational levels. Specifically, amongst primary school students, recent longitudinal studies have found that spatial ability is a significant predictor of mathematics performance (Geer et al., 2019; Gilligan et al., 2019), and the work of Lowrie and colleagues in Australia has been seminal in examining the embedding of spatial ability development into practice (Lowrie et al., 2019; Lowrie and Logan, 2023; Resnick and Lowrie, 2023). Hawes et al. (2023) present three rationales for this relationship being that spatial ability and mathematics engage analogous brain regions and neurological pathways, that spatial ability make enhance mathematical problem solving by enabling the representation and illustration of mathematical concepts, and pedagogically that mathematical concepts are often and lends themselves to being communicated spatially. With respect to science education, multiple studies (e.g., Kozhevnikov et al., 2007; Jee et al., 2013; Miller and Halpern, 2013; Shipley et al., 2013; Gagnier et al., 2017; Verdine et al., 2017; Mix, 2019) have demonstrated that spatial thinking plays a crucial role in students’ general comprehension and reasoning about scientific phenomena. Understanding and logical analysis of spatial characteristics such as position, size, and capacity are essential for students to effectively solve scientific challenges, as demonstrated by these studies. That said, in many jurisdictions, science education provision is structured across physics, chemistry, and biology separately, and it is therefore worth noting the evidence linking spatial ability to each of these fields. For this reason, amongst others, the role of spatial ability across different age groups in physics education has been widely studied in recent years. Träff et al. (2019), for example, investigated the extent to which logical reasoning, spatial ability, and working memory at age 9–10 are predictive of physics skills at age 12–13. Mental rotation was found to be a long-term predictor of physics skills in 12- to 13-year-olds, which is consistent with earlier findings of a link between spatial processing and physics in adults (Kozhevnikov et al., 2007; Webb et al., 2007; Wai et al., 2009; Kell et al., 2013). Evidence from chemistry education research indicates that students have been found to struggle even at the university level due to their inability to visualize submicroscopic structures (Tuckey et al., 1991; Chittleborough and Treagust, 2007). This has resulted in the hypothesis that spatial ability plays a crucial role in learning chemistry (National Research Council, 2005). As such, the effects of spatial ability on learning chemistry have been extensively studied (Cole et al., 2020; Fatemah et al., 2020; Applebee et al., 2021; Horikoshi, 2021; Kiernan et al., 2021; Rahmawati et al., 2021; Salame and Kabir, 2022). Recent observations include that students with higher levels of spatial ability performed better than their peers with lower levels when assessed on their understanding of the conservation of matter (Cole et al., 2020) and that students use both analytical and spatial reasoning when making predictions about molecular geometry (Kiernan et al., 2021) Finally, given the importance of both textual and visual elements in biology education, spatial skills are an essential asset in the subject (Castro-Alonso and Uttal, 2019) with existing research with adults demonstrating a link between spatial ability and conceptual understanding of specific biological concepts (Garg et al., 1999; Hodgkiss et al., 2018). Pedagogically, the use of different types of visual representations (e.g., images, drawings, diagrams, graphs) in biology influences how children learn (Bergey et al., 2015) and these have been examined with respect to their interaction with students’ levels of spatial ability.
3 Aim of the review
Given the clear associative evidence between spatial ability and science and mathematics education, the purpose of the current review is to contribute to a pedagogical framework for spatial ability, predominantly for use at the primary level, which encompasses the interconnections among the various systemic components of teaching and learning and recognizes the barriers and facilitators that impact on each of these components. It is envisioned that an understanding and highlighting of these barriers and enablers will enhance the capacity to develop fit-for-purpose and evidence-informed pedagogical approaches that further spatialize existing curricula and thus foster the development of spatial ability in students’ whilst they develop scientific and mathematical competencies. To guide this review, the following research question was used:
What are the enablers and barriers when implementing spatial activities in primary level science and mathematics across different countries?
In order to obtain a broader range of the barriers and enablers that teachers can encounter when teaching for the development of spatial ability, the primary research question was divided into sub-questions to construct a path of all of the components that are interrelated when teaching spatial abilities These included:
1. For context, how do different countries’ primary level curricula address spatial ability development in science and mathematics education?
2. From a review of the selected curricula, what barriers and enablers are structurally apparent with respect to curriculum organization?
3. What are the current teacher training practices for developing spatial ability skills in pupils, and how effective are they?
4. How can teacher training programs provide opportunities for teachers to develop their pedagogical content knowledge in spatial ability through collaboration and reflection with other teachers?
5. How can primary level educators “spatialize” their mathematics and science classrooms?
6. The findings from the review are structured around each of these sub-questions.
4 Findings
4.1 How do different countries’ curricula address spatial ability development in science and mathematics education, and what factors facilitate or impede this process?
To contextualize the insights gained in response to this question, initial work involved qualifying the nature of primary-level curricula for mathematics and science in four European countries: Ireland, the Netherlands, Latvia, and Sweden. The selection of countries was based on this review being conducted by researchers involved in the EU-funded SellSTEM project, who are based in these respective locations and are thus able to provide accurate accounts of the respective educational systems. Narrative accounts of these curricula are provided below, with Supplementary Tables S1–S7 providing structured summaries of the Irish, Latvian, Dutch and Swedish curricula. The subsequent review is not limited to research from these countries or to primary level education given that evidence and experiences from different contexts (jurisdictions and educational levels) can be informative. Rather, these countries serve as exemplary to what spatial ability currently can look like in primary education across Europe, at least from these countries perspectives.
4.1.1 The mathematics and science curricula in primary schools in Ireland
In Ireland, school is mandatory from age six until sixteen, or until 3 years of schooling at the secondary level are complete. Primary-level education is an eight-year-long program. The curriculum consists of 12 subjects including Mathematics, Gaeilge (Irish language), English, History, Geography, Science, Visual arts, Music, Drama, Social, personal and health education, and Religious education. The National Council for Curriculum and Assessment (1999) introduced the current Mathematics curriculum in 1999, which is composed of five strands: Number, Algebra, Shape and space, Measures, and Data. The curriculum places significant emphasis on spatial ability, particularly in the Shape and space strand. Despite being presented in distinct sections, these strands are not considered independent domains with comprehension of one strand being contingent upon and reinforcing notions and principles in other strands. The strand of Shape and space dives into the concept of spatial awareness and its practical implementation in various real-world scenarios. The curriculum comprises modules that cover topics related to geometric shapes in two and three dimensions, symmetrical figures, as well as lines and angles.
The current Science curriculum was also introduced in 1999. Teaching science in the primary curriculum requires the development of two categories of comprehension: conceptual comprehension and procedural comprehension. Children’s conceptual understanding is concerned with the growth of their scientific knowledge and comprehension of fundamental scientific concepts. The four strands of the science program are Living things, Materials, Energy and forces, and Environmental awareness and care. Beginning in the infant classes, students begin to develop a sense of observation based on characteristics such as shape, size, color, pattern, texture, sound, and familiar things in the local environment, as well as the ability to predict what will occur in structured situations. In addition, estimation and measurement skills are developed by describing mass and length through comparison words such as – ‘is bigger than’ and, ‘is heavier than.’ In addition, through scientific investigation, students engage in a variety of design and make activities, employing tools and materials in both structured and unstructured situations.
4.1.2 The mathematics and science curricula in primary schools in Latvia
Children in Latvia begin primary education at the age of six or seven. Primary education is compulsory in Latvia and has a duration of 9 years. The recent curriculum and educational methodology (National Centre for Education Latvia, 2019a,b), implemented in 2019/2020, has placed increased emphasis on the topic of socio-emotional preparedness for academic pursuits. The curriculum comprises modules that cover a range of topics related to geometric shapes in two and three dimensions, construction and deconstruction, symmetrical figures, lines, and angles, as well as graphical representations.
The presence of spatial ability-related learning in the primary mathematics curriculum is observed across all classes. Beginning in the first class, students begin to visually develop an understanding of figure composition, grouping based on similar and dissimilar characteristics, and distinguishing symmetrical objects in their environment. The acquisition of knowledge is a gradual process, with a significant focus on the construction and deconstruction of concepts beginning in the second grade. To facilitate this process, various activities, including planning and sketching, are employed. Science learning in primary school is methodical and sequential. The curriculum’s content is organized according to the concentric principle where at each level of education, the material is revisited in greater depth. From first to sixth class, students study a single subject - natural science - and in seventh class and beyond, students’ study separate subjects - biology, geography, chemistry, and physics.
In Latvia’s primary science curriculum, there are numerous spatial ability components, and in each class students can potentially develop spatial ability through a variety of strands. For example, in the initial year of primary education, students engage in the practice of observing and contrasting various organisms and objects, drawing upon their perceptual abilities to identify and label the most salient characteristics of indicated objects. In the second class, students learn about motion and how to compare the movement of various objects using the correct terminology, such as “slower, faster” as well as how to explain, based on visual observation, the forces acting on an object that alter its motion. They enforce such knowledge through the upper classes where students develop projects about force and movements and utilize statistical data to interpret such findings.
4.1.3 The mathematics and science curricula in primary schools in the Netherlands
The Minister of Education, Culture and Science establishes the legal and administrative framework within which individual institutions must operate. In the Netherlands, children begin an eight-year long primary education at the age of four. The curriculum consists of the following learning domains: Dutch, English (Frisian language), Mathematics, Personal and World Orientation, Arts and Physical Education. For each of these learning domains core goals were set in 2006 by the Ministry of Education, Culture and Science. New core goals are under development, and it is expected that the versions will be published in 2025. However, based on work to an initial presentation of the new Mathematics core goals to the outgoing Minister for Education Culture and Science in 2023, the new version contains almost the same mathematics concepts as are in the 2006 curriculum. However, the core goals will now be explained in more detail for the mandatory part of the curriculum. The Mathematics curriculum for primary school comprises 11 core objectives (Mullis et al., 2016). These describe the intended learning outcomes, but not how they should be attained. The pedagogical approach is explained in the learning lines. These learning lines are not obligatory but illustrate to teachers and makers of educational resources how they can reach the core goals step by step. For the current Mathematics core goals from 2006, six content lines for various math concepts (Number, Number Operations, Relations, Measuring, Geometry, and Proportions) have been developed. Particularly the Geometry line is spatial in nature through the subgoals of Orientation in Space, Constructing, and Operations with Forms and Figures.
In primary education, Science is incorporated into the Personal and World Orientation learning domain (Mullis et al., 2016). As part of Personal and World Orientation, children learn about the natural world and phenomena present (Mullis et al., 2016). Currently, a committee has been formed to develop new core goals for Science with the anticipation that these will be published in the second half of 2024. The core goals for science are currently found in the sub-domain Nature and Technology and include various goals and topics from biology, chemistry and physics such as light, sound, magnetism. In addition, Design and Technology goals are present in the Dutch curriculum and students should learn to solve problems through designing. However, only a few schools include Design and Technology and the time schools provide for science is in general decreasing. Again, for all core goals related to Science, content lines are available to explain how schools could reach the core goals and these frequently include activities with a spatial nature. For example, observing and comparing plants and animals based on appearance (including form), or exploring nature inspired technological developments such as Velcro are relevant content lines. Many of the design-related activities are potentially spatial in nature such as thinking about the form-function of products and discovering patterns in the environment based on shapes.
4.1.4 The mathematics and science curricula in primary schools in Sweden
The national mathematics curriculum (Skolverket, 2018) for compulsory school begins with a statement of purpose that describes the role of mathematics in society and human activity and presents arguments defending the significance of learning and teaching mathematics. In addition, the syllabus provides an overview of the objectives for creating mathematics learning opportunities for students, such as the opportunity to develop the ability to formulate, apply, and analyze mathematical concepts, as well as use appropriate mathematical expressions, through mathematics instruction. The curriculum is separated into three levels: Grades 1 through 3, Grades 4 through 6, and Grades 7 through 9. The descriptions of core content are relatively brief, and the course outline does not specify the order in which each tier’s content should be covered or introduced. Understanding and Use of Numbers, Algebra, Geometry, Probability and Statistics, Relationships and Change, and Problem-Solving are the six categories of the curriculum and are the same for all three levels.
The core subject, “geometry,” encompasses a diverse range of spatial tasks that target students in the first to third-grade levels. These exercises involve the comprehension of basic geometrical properties, which include points, lines, and distances, as well as the construction of geometrical objects. Additionally, students are taught the principles of scaling for simple enlargement and reduction, common terminologies used to describe an object’s position in space, symmetry, and the ability to make comparisons and estimates of mathematical quantities. Furthermore, spatial elements are observed in the fundamental subject of “relationships and changes,” where various proportional relationships, such as doubling and halving, are developed. In the fourth to sixth grade, the use of visuals, such as graphs, for expressing different types of proportional relationships in simple investigations, as well as the coordinate system and strategies for scaling coordinate axes, are developed in the core subject “relationships and changes.”
Science is divided into three subjects in the Swedish primary curriculum: biology, chemistry, and physics (Skolverket, 2018). In the first level, from grade 1 to grade 3, physics, biology, and chemistry have the same core content, and spatial competencies are found in force and motion where students observe and engage in play and movement, such as on swings and slides. Moreover, the development of spatial visualization is fostered by comprehending unseen forces, such as gravity. Furthermore, the content “materials and substances in our surroundings” includes spatial elements such as the properties of materials and how materials and objects can be categorized based on characteristics such as appearance, magnetism, and conductivity. From grades 4–6, novel learning material pertaining to biology and chemistry is introduced. The content of “biology its methods and ways of working” contains indications of spatial elements, in which students devise and implement simple studies and experiments, and classify and group the ways by which animals, plants, and other organisms can be identified. In the field of chemistry, specifically in the topic of “chemistry in nature,” students are tasked with devising and implementing basic, methodical analyses utilizing tables and images.
4.2 From a review of the selected curricula, what barriers and enablers are structurally apparent with respect to curriculum organization?
Following the in-depth examination of the curricula of Ireland, Latvia, the Netherlands, and Sweden four systemic enablers for spatial ability development have been identified:
▪ Systematic curricular design for spatial ability development
▪ Adoption of spatial technology
▪ Adoption of hands-on activities
▪ Alternative assessment
In addition, two barriers were identified which could impede the development of spatial abilities in pupils:
▪ Insufficient duration
▪ Inadequate consideration of learner diversity in curriculum design.
4.2.1 Curriculum enablers
4.2.1.1 Systematic curricular design for spatial ability development
A systematic curriculum design could facilitate the development of spatial ability in students. What was immediately apparent from the curricular review is the variance between countries in making spatial ability development explicit. For the most part, the curricula do not speak directly to spatial ability. The Irish primary level mathematics curriculum however, through the strand of Space and shape, does. This level of specificity and associated description or advice can serve as a substantive enabler for spatial ability development. When stakeholders design a curriculum with the intent of developing spatial ability, the curriculum should facilitate the gradual progression from simple one-dimensional structures to more complex three-dimensional structures (Salimi et al., 2020). The pedagogical approach known as “Concrete-Pictorial-Abstract” is widely utilized in the Irish primary mathematics curriculum, as illustrated in Figure 2.
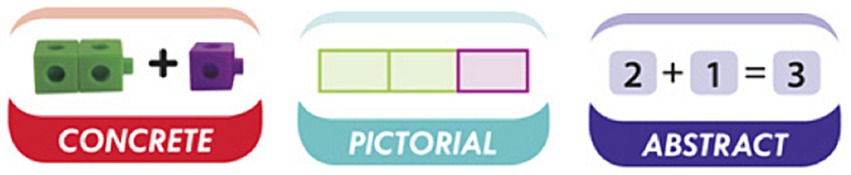
Figure 2. Concrete – pictorial – abstract approach. https://www.maryvaleacademy.ca/academics/math/Concrete-Pictorial-AbstractLearningProgression.
This approach involves the introduction of concepts first through experiential learning with the aid of manipulatives. Subsequently, students engage in visualizing these concepts through pictorial models such as number bonds and bar models, which serve as a means of creating a mental image. Lastly, students employ abstract numerical and symbolic representations when they possess sufficient contextual knowledge to comprehend their significance (Gujarati, 2013). While a pedagogical approach, considering such scaffolding at a curriculum level could not only enable students to acquire spatial ability through a more structured approach (Lee and Kim, 2018) but it could also help teachers to gain further experience through this progression (Taylor and Hutton, 2013). More importantly, this type of systematic design aligns with students’ cognitive development, which could further enhance their learning and retention of concepts (Taylor and Hutton, 2013).
4.2.1.2 Adoption of spatial technology
An appropriate technology-supported spatial curriculum could better improve students’ spatial ability when it relates to object identification and image interpretation (Bodzin, 2011). For example, in the geography curriculum, geospatial information technologies such as Google Earth can be effective in supporting the development of students’ spatial ability in terms of aerial and remotely sensed imagery interpretation. An additional benefit of the implementation of appropriate technology within the curriculum could be an increase in students’ engagement and interest (Endreny, 2010). Bodzin (2011) found students to be more engaged in spatial tasks as they perceived them as more meaningful and relevant to their real-life. According to a study conducted by ten Brummelhuis et al. (2010), the utilization of technology is prevalent in The Netherlands, with a significant proportion of primary school teachers incorporating technology into their daily classroom activities.
4.2.1.3 Adoption of hands-on activities
It is very useful to include concrete objects in the learning materials when designing a spatial curriculum for young pupils. Previous studies have suggested that young children need manipulatives such as blocks to develop their spatial and geometric abilities (Başak and Davasligil, 2014). For example, by assembling, stacking, and inserting blocks into slots, pupils can manipulate the physical objects in their minds. Hence, their thinking and the objects can be connected and transformed which can also further stimulate their creative thinking and self-learning (Lee and Kim, 2018). The curricula of Ireland and Latvia strongly suggest the use of a diverse range of hands-on activities, with a particular emphasis on geometry, including 2D and 3D shapes, as well as spatial awareness.
4.2.1.4 Alternative assessment
It is important to develop alternative assessment mechanisms that allow teachers to gain insight into their pupils’ levels of spatial ability (Jadallah et al., 2017). The establishment of these as formal and high stakes or not is a separate discussion. Such assessment should help pupils relate what they have learned to their real life, which could increase pupils’ engagement in their acquisition of spatial ability. Educators could link the assessment to pupils’ practical life when they design a spatial curriculum. Examples of alternative assessment for spatial ability can include way finding in the real world with map reading, assembling furniture, and packing a trunk or case to maximize capacity (Lee and Bednarz, 2012).
4.2.2 Curriculum barriers
4.2.2.1 Insufficient duration
Previous studies have reported that insufficient curriculum hours hinder children’s growth in spatial ability (Collins, 2018). Some curricula do not make reference to spatial ability development at all, and others only include spatial development on an hourly or daily basis, which is less than enough to witness young pupils’ growth in spatial ability. Where the curriculum does not make such explicit reference to spatial ability development it is left to interpretation or potentially chance whether or not practice will impact spatial development. In the review of curricular documents it was apparent that the Mathematics curricula were either explicit about the development of spatial ability, or had activities more intuitively associated with spatial thinking, while the Science curricula had learning that could be interpreted to be spatial in nature but this seems likely to require more consideration. Research suggests that to observe children’s gains in spatial ability, more time should be allotted in the curriculum to conduct spatial interventions or to focus on spatial thinking development (Jadallah et al., 2017). This barrier was identified as one of the biggest challenges for teachers in primary schools in Latvia when implementing spatial ability developmental activities (Bufasi et al., 2022).
4.2.2.2 Inadequate consideration of learner diversity in curriculum design
Researchers found that consideration of talented children’s needs is, unfortunately, missing in today’s school curriculum (Webb et al., 2007). Spatially talented children have unique intellectual strengths. These children might have their personal preferences during the acquisition of spatial ability. For example, some talented children hope to develop spatial ability by learning robotics, increasing lab work, or reading biographies of scientists (Webb et al., 2007). Educators should modify the curriculum to tailor the learner diversity to increase students’ engagement and motivation for their own learning.
4.3 What are the current teacher training practices for developing spatial ability in pupils, and how effective are they?
Extensive research by Lowrie and colleagues in Australia has been conducted to understand pedagogical approaches to spatial ability development in primary level Mathematics. For example, Lowrie et al. (2019) conducted an embedded three-week intervention study with grade 5 and 6 pupils and found a significant positive impact on spatial ability and geometry and word-based mathematics problems. A critical dimension to this was a professional development program for the involved teachers and provision of purposefully design resources including lesson plans, physical manipulatives, and digital resources. In a subsequent study, Lowrie and Logan (2023) observed significant additive effects of an embedded spatial intervention when delivered in addition to an isolated intervention. They highlighted the criticality of teacher knowledge and confidence with teaching for spatial ability development. As a final example from this group, Resnick and Lowrie (2023), in a large-scale play-based spatial ability intervention study focused on improving numeracy in preschool, emphasized the importance of pedagogical tools and activities such as gestures and sketching for spatial ability development and mathematics transfer. The collective contribution from this group offers significant guidance for other international efforts with respect to primary level reform or pedagogical guidance for the development of spatial ability.
Outside of Lowrie’s research group, others have also contributed to an understanding of spatial-related pedagogy. Burte et al. (2020) identified significant obstacles to the achievement of mathematical and spatial competencies that are associated with pedagogical practices. They suggest that interventions that prioritize the enhancement of spatial ability and its potential transfer to mathematics tend to neglect other variables that impact the learning process. Specifically, the focus is on individuals’ perceptions of their own mathematical and spatial abilities. The role of stereotypical thinking is a crucial factor that impacts the effectiveness of teachers in their instructional practices. In addition, Hawes et al. (2022) conducted a comprehensive meta-analysis of the existing literature on spatial training and its correlation with mathematical performance. The study revealed that it is feasible to observe a transfer effect from spatial ability training to mathematical performance. Furthermore, Adams et al. (2022) propose a number of recommendations that could enhance pedagogy, including the provision of adaptable resources that enable educators to seamlessly incorporate them into their instructional practices and strategies that take into account the longitudinal growth of spatial ability. The authors also suggest the possibility of implementing constructivist methodologies for enhancing spatial ability, which involves the utilization of material objects to facilitate the development of spatial skills. According to their argument, a pedagogical framework that provides substantive scaffolding can effectively assist children with lower academic performance. According to Gagnier et al. (2022), fostering children’s spatial ability would be advantageous, especially in the context of science education. This intervention may serve to assist educators with limited spatial aptitude in promoting spatial proficiency through pedagogy and emphasizing spatial components within instructional materials. In addition, Gagnier et al. (2022) have provided evidence that professional development programs play a crucial role in enabling teachers to successfully incorporate spatial ability pedagogy into children’s education.
4.3.1 Pedagogical enablers
• Pedagogical frameworks aid educators in effectively supporting learning through the utilization of constructivist methodologies that involve the use of physical objects (Adams et al., 2022).
• The most effective way to achieve a transfer from spatial ability training to mathematics performance may be through an “in situ” approach. In addition, the significance of utilizing hands-on materials is in facilitating effective spatial instruction (Hawes et al., 2022; Resnick and Lowrie, 2023).
• Assisting teachers with lower spatial ability by emphasizing spatial components within instructional materials (Lowrie et al., 2019; Gagnier et al., 2022).
• Professional development programs play a crucial role in enabling educators to proficiently incorporate spatial ability pedagogy into the academic curriculum of children (Lowrie et al., 2019; Gagnier et al., 2022).
• The significance of professional development lies in its ability to facilitate the application of research findings in practical settings (Pollitt et al., 2020).
4.3.2 Pedagogical barriers
• In most research pedagogy is not a prominent factor, and there is a gap in the literature regarding the integration of educational and cognitive science research findings into pedagogy (Adams et al., 2022).
• Focusing on mathematics outcomes may lead to overlooking the vital role spatial ability plays in other STEM-related learning (Hawes et al., 2022).
• Overlooking factors that affect learning, such as perceptions about one’s own abilities and stereotypical thinking (Burte et al., 2020).
• Spatial ability and mathematics instruction methodology and could be negatively impacted by low teacher beliefs regarding their mathematics and spatial ability (Pollitt et al., 2020).
4.4 How can teacher training programs provide opportunities for teachers to develop their pedagogical content knowledge in spatial ability through collaboration and reflection with other teachers?
In the context of children’s spatial ability development, it is beneficial for educators to tailor their instructional approaches to accommodate diverse levels of spatial ability in children. For example, students with lower levels may need additional support to engage with more complex visual materials than those with higher levels. Park and Oliver (2008) assert that the adaptation of lessons to suit the abilities and interests of children necessitates both knowledge-on-action and knowledge-in-action. The concept of knowledge-on-action pertains to the knowledge base utilized in the planning and implementation of instruction that is specific to a particular topic. Knowledge-in-action refers to the actions undertaken by teachers in the classroom, which is commonly referred to as enactment. This type of implementation entails instantaneous decision-making, which embodies a more fluid form of expertise (Schön, 1983; Park and Oliver, 2008). Pedagogical Content Knowledge (PCK) then encompasses both internal and external reflections that mutually influence one another within the classroom setting. High levels of PCK within teachers would enable them to better tailor their pedagogy for the development of spatial ability and to suit the diversity in their children’s ability levels. Magnusson et al. (1999) outline four components of PCK that assist educators in addressing knowledge-in-action and knowledge-on-action:
1. Goals and objectives for teaching a specific topic in the curriculum,
2. Children’s understanding of the topic,
3. Instructional strategies concerning the topic,
4. Ways to assess children’s understanding of the topic.
For each of these 4 components, there are multiple data points that can be collected to identify the PCK of teachers. Such data points address how particular topics, problems, or issues are organized, represented, and adapted to the diverse interests and abilities of learners (Shulman, 1987). These data points could include:
1. Lesson plans, interview transcripts, lesson artifacts, video of classroom teaching, and field notes from observation as secondary data sources (Friedrichsen et al., 2009)
2. Analysis of lesson plans developed by participants based on a taxonomy of spatial thinking (Jo and Bednarz, 2014; Newcombe et al., 2016).
3. Classroom observation (semi-structured interview/ videos) for insights on teachers’ PCK development (enactment coding schemes are available).
According to Hong et al. (2019), teacher training programs have the potential to facilitate the enhancement of teachers’ PCK. This can be achieved through the implementation of diverse Professional Development (PD) strategies, which involve collaborative and reflective practices among teachers. Effective PD should prioritize the needs of both educators and learners and consider various factors that impact them, including individual and contextual elements.
4.4.1 Pedagogical content knowledge enablers
• School leaders can play an important role in enabling opportunities for collaboration and reflection by providing resources and support for professional development and collaborative activities (Desimone, 2002).
• Professional learning communities can provide opportunities for teachers to collaborate and learn from one another about effective spatial reasoning teaching strategies (Fulton and Britton, 2011; Van Driel and Berry, 2012).
• Providing coaching and mentoring can help teachers to develop teachers PCK by providing one-on-one support and feedback (Zubaidah and Johar, 2018).
• Engaging in lesson study can help teachers to develop teachers PCK by providing opportunities for collaboration and experimentation with new teaching strategies (Juhler, 2016; Leavy and Hourigan, 2016; Coenders and Verhoef, 2019).
• Providing teachers with access to necessary resources, such as technology and funding, can help to support their participation in collaborative activities and reflective practice (Hennessy et al., 2022; Okoye et al., 2022).
• Providing incentives and recognition for teachers who engage in collaborative activities and reflective practice can help to motivate and encourage participation (Chen Musgrove et al., 2022; Matahela and van Rensburg, 2022).
4.4.2 Pedagogical content knowledge barriers
• It may be challenging for teachers to develop their PCK in spatial ability if they have limited time to engage in collaborative activities or engage in reflective practice (Bufasi et al., 2022).
• Teachers may not have access to the necessary resources and support to engage in collaborative activities and reflective practice, such as technology, funding, and release time from teaching duties.
• Certain teachers may exhibit reluctance toward altering their pedagogical approaches, particularly if they possess extensive teaching experience or lack familiarity with spatial reasoning principles (Christidou et al., 2022).
• Teachers may not have had the opportunity to engage in professional development activities centered on spatial reasoning or to acquire effective teaching strategies in this area (Gagnier et al., 2022).
4.5 How can primary level educators “spatialize” their mathematics and science classrooms?
From first grade, science and mathematics teachers use symbolic spatial resources including graphs, maps, animations, and diagrams. These tools can significantly support spatial thinking processes. Consequently, the implementation of more spatial activities throughout lessons is one of the most crucial components when teaching for spatial skill development. Most teachers grasp the concept of spatial ability, but some may not know how to teach the topic in a way that emphasizes spatial competence. Therefore, it is essential to comprehend the challenges and benefits teachers face when designing and implementing spatial tasks. According to Costales et al. (2015), incorporating spatial activities that promote spatial thinking into the classroom does not require additional materials or instruction. Rather, existing methods and resources can be utilized to “spatialize” classrooms and programs. Such approaches are:
4.5.1 Using more spatial language
Educators can easily incorporate spatial language into the classroom. For instance, during clean-up time. by instructing a child to “place that block on the top shelf between the triangular and rectangular blocks,” they are emphasizing a spatial location (i.e., top), a spatial relation between two objects (i.e., between), and two different shapes (i.e., rectangle, triangle) (Casasola et al., 2020). Such spatial terms are prevalent in the primary mathematics curricula of Ireland and Latvia. For instance, in Ireland statements are included indicating that children should be able to explore, discuss, develop, and employ the vocabulary of spatial relations related to positioning (over, under, up, down, on, besides, in) and direction (moving in straight/curved lines, in a circle, finding own space) by the end of the first grade. In addition, the acquisition of spatial language is furthered during the initial and subsequent years of primary education, as the vocabulary pertaining to spatial relationships concerning location is refined and enhanced. This includes prepositions such as “between,” “underneath,” “on top of,” “around,” “through,” “left,” and “right,” as well as directional language denoting basic navigational instructions within the classroom and school environment, such as “from desk to window,” “from classroom to school hall,” and “from classroom to school yard.” Furthermore, the primary mathematics curriculum in Latvia places significant emphasis on spatial vocabulary from the first grade onwards. This is particularly evident in the curriculum strand pertaining to the “location and directions of objects in the plane, in space,” where children are expected to develop their observation skills and articulate spatial concepts using appropriate terminology such as “right/left,” “top/bottom,” “front/back,” “closer/further,” “first/then,” “earlier/later,” among others.
4.5.2 Playing with blocks and puzzles
As there are cubes, pyramids, cylinders, and rectangular prisms in blocks, children’s recognition, sorting, and labeling of three-dimensional shapes are also reinforced by block play. Children get to exercise their pattern recognition and pattern-making skills, both of which are key spatial thinking skills when they are asked to build a structure out of blocks that is the same as an educator’s construction. For instance, when Caldera et al. (1999) observed the construction activities of 51 preschoolers, they discovered a pattern: the children who demonstrated a greater interest in construction — and who constructed more complex structures — performed better on a standardized test of spatial intelligence. Similar observations have been made by others (Oostermeijer et al., 2014; Richardson et al., 2014; Jirout and Newcombe, 2015).
The Irish curriculum extensively employs blocks, particularly the dienes blocks, as concrete numerical representations that are proportionate to each other. This enables children to represent all powers of ten, including ones, tens, hundreds, and thousands. The utilization of blocks facilitates children’s comprehension of the correlation between place value columns and the rationale behind exchanging, such as exchanging one ten for ten ones (Kurumeh et al., 2010).
4.5.3 Multiple spatial examples
Comparison of regular and irregular examples enables children to recognize the distinguishing characteristics of the spatial concept. Such comparison activities are evident in all curriculums, especially in the strands of Geometry (2D and 3D shapes). According to a study conducted by Burte et al. (2020), the efficacy of visualizations was evaluated by teachers for different types of mathematical problems. The results indicated that visualizations were rated as most helpful for visual problems, followed by word problems, and least helpful for notation problems. According to the findings, teachers perceived visual aids to be the most beneficial in real-life scenarios, followed by abstract situations, and least effective in notation-based contexts.
4.5.4 The use of tangrams
In strands/topics such as geometry, ‘tangram’ puzzles can be utilized as a tool for presenting specific mathematical concepts, stimulating children’s observation, imagination, shape analysis, creativity, and logical reasoning (Olkun et al., 2005; Yang and Chen, 2010). By sorting, comparing, and solving the puzzle, children can deepen their understanding of geometric ideas and solve issues in geometric situations. Furthermore, the study conducted by Bufasi et al. (2022) revealed that the incorporation of tangrams in primary education was highly advantageous, as it enhanced the effectiveness of instruction and resulted in greater pupil engagement compared to traditional teaching methods. Furthermore, the activity fostered pupil collaboration as proficient pupils provided assistance and demonstrated techniques to their peers who encountered difficulties with the tangram puzzle. However, in the context of the task that pertained to geometric patterns, the pupils encountered difficulties in committing to memory-diverse patterns; while their memory capacity was occasionally satisfactory, they encountered challenges in reproducing the patterns through drawing.
4.5.5 Extending the discussion of the solution with ideas and expressions from children
An effective way to promote problem-solving skills in children is to extend the conversation with another idea and let them figure out the solution. This approach encourages children to think critically and creatively, as well as to develop their communication and collaboration skills. By providing them with opportunities to explore different perspectives and come up with their own solutions, educators can help them become more confident and independent problem-solvers (Chan et al., 2020).
4.5.6 Talk out loud and model
Teachers can spatialize their classroom effectively by using talk-out-loud and modeling techniques (Chan et al., 2020). This involves verbally explaining and demonstrating various spatial concepts to students. For instance, they can articulate how to arrange objects in space, like stacking blocks in ascending order, by explaining the process step by step.
4.5.7 Spatialization enablers
• The use of spatial language can serve as a means of enhancing and strengthening spatial cognition (Newcombe, 2010)
• The utilization of blocks and puzzles presents possibilities for children to engage in problem-solving and investigate fundamental early mathematical concepts such as shape, size, scale, location, and structure (Chan et al., 2020).
• Extend the conversation with another idea and let your child figure out the solution (Chan et al., 2020).
• Utilization of various representations in a versatile and interconnected manner, alongside the establishment of connections among these representations and the ability to translate between and within them, plays a crucial role in the development of comprehensive and profound mathematical comprehension (Teixidor-i-Bigas et al., 2013; Bautista et al., 2015).
• One way to help kids learn how to solve problems is to add another idea to the talk and let them figure out the answer. This method helps kids learn how to communicate and work together, as well as how to think critically and creatively (Chan et al., 2020).
• Explain the steps taken to reach a solution for a mathematical problem in order to illustrate the problem-solving procedure (Chan et al., 2020).
4.5.8 Spatialization barriers
• Educators are often constrained by time limitations that may hinder their ability to incorporate diverse instructional strategies involving manipulatives and visual aids (Bufasi et al., 2022).
• Assessing the progress of children in manipulative tasks and providing parents with corresponding grades and evidence can be a challenging task (Bufasi et al., 2022).
4.6 Summary of identified barriers and enablers
Through a comprehensive examination of each of the sub-questions outlined in the present study, the groundwork is laid for the development of an overall framework detailing the enablers and barriers encountered by teachers when teaching spatial abilities. The response to the primary research question is summarized in Table 1.
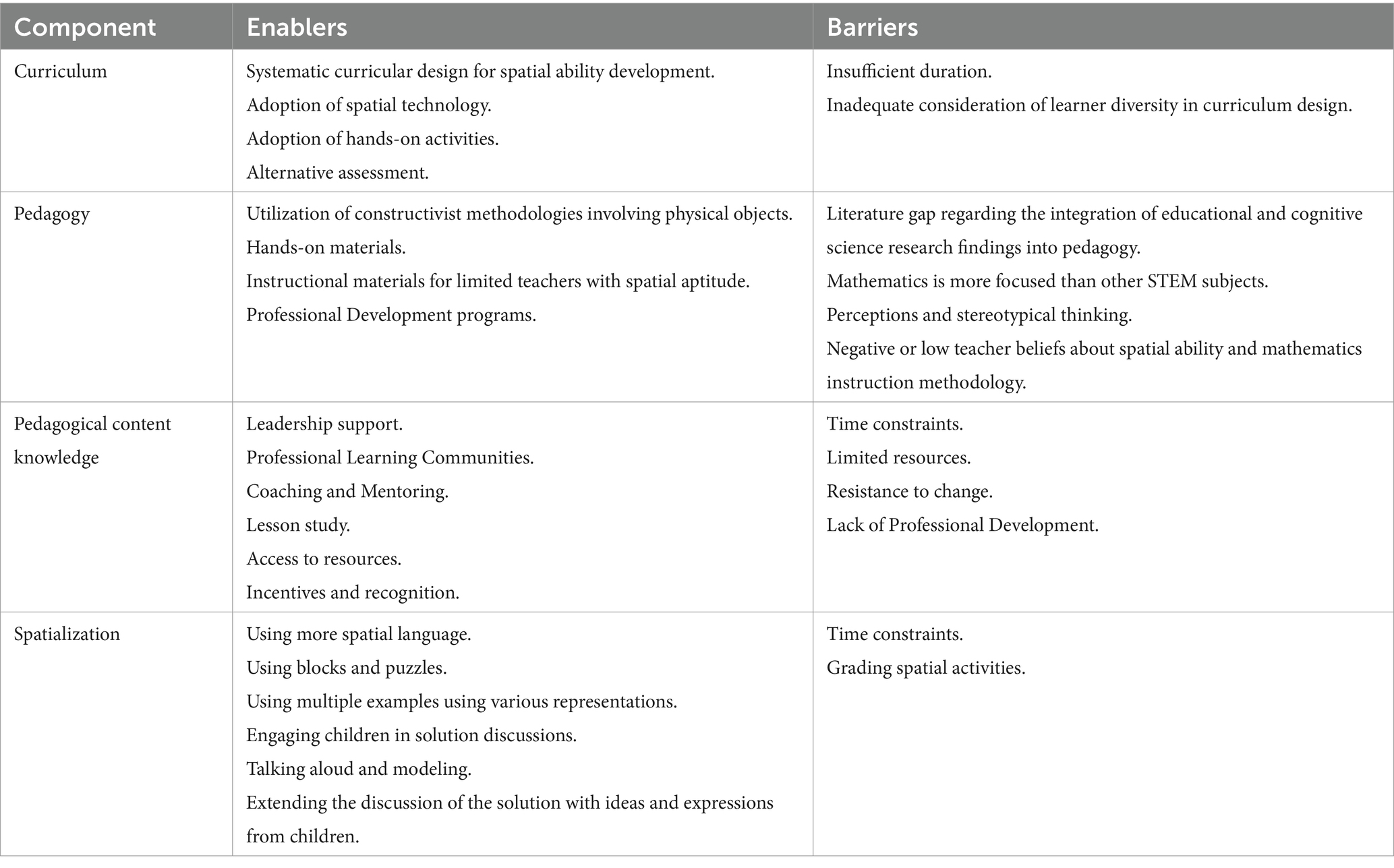
Table 1. Summary of enablers and barriers identified for integrating spatial activities into primary level science and mathematics education.
5 Discussion
The present study involved conducting a detailed narrative examination of the literature, resulting in the initial development of a framework that facilitates a comprehensive understanding of the manifold elements implicated in the teaching of spatial abilities. This framework encompasses the complex interaction among these elements, as well as the factors that facilitate or hinder teachers’ efforts to teach spatial abilities. The study utilized sub-questions to facilitate responding to the main research question, which was addressed by providing evidence for each of the enablers and barriers presented in response to the sub-questions.
5.1 The mathematics and science curricula in primary schools
To develop a comprehensive understanding of the integration of spatial elements in the curriculum, an initial examination of the primary mathematics and science curricula of Ireland, Latvia, the Netherlands, and Sweden was conducted. The findings indicate that spatial components are incorporated in these curricula, although how explicit these are made varies. The most explicit case was in the Irish mathematics curriculum which features a well-defined and detailed strand dedicated to spatial ability, known as “Shape and space” beginning at the infant level. In addition, the Irish science curriculum incorporates spatial components, particularly in the development of practical skills related to design and construction. These skills include the ability to plan through visualization and communication with others, exploration through structured and unstructured material manipulation, craft-handling skill development for fabrication, and positive peer evaluation and reporting. The Latvian mathematics curriculum incorporates a diverse range of spatial components. In contrast to the Irish mathematics curriculum, the spatial strand is not explicitly delineated, but rather is integrated throughout the other strands. In addition, the science curriculum incorporates spatial components as well, which are dispersed throughout the topics of forces and motions, as well as the observation of various material properties. It is worth noting that the Swedish mathematics curriculum places comparatively less emphasis on spatial components. Specifically, only two strands, namely Geometry and Relationships, and changes are highlighted, with a focus on spatial orientation. Meanwhile, the science curriculum in Sweden displays noticeable spatial components that are distributed throughout the coverage of topics in physics, biology, and chemistry. In the Netherlands, the broader national curriculum necessitates reliance on general education achievement goals, which may complicate the identification of spatial components in pedagogy. Nevertheless, recent research has demonstrated that technology is widely employed by educators in the country, with nearly all teachers incorporating it into their daily instructional routines.
Gökçe (2015) argues that the current spatial curriculum is inadequate in terms of allocated learning hours and fails to provide effective strategies to accommodate the diverse needs of learners. One potential approach to increase learning time could involve expanding the curriculum through the addition of instructional hours and/or days to the current schedule. Furthermore, Gökçe (2015) argues that prioritizing the improvement of teachers’ classroom management abilities and addressing children’s learning challenges within the constraints of limited instructional time is of greater importance. However, Leutner et al. (2009) suggest that incorporating spatial elements into the curriculum may facilitate the development of children’s spatial skills without imposing additional cognitive demands.
5.2 Pedagogical practices
Several issues were identified which can impede the teaching for spatial ability development. A primary concern that is closely associated with teaching practice pertains to the insufficient emphasis on associated pedagogy within educational training programs. The issue has resulted in an absence of research, as the majority of these investigations are primarily grounded in the field of cognitive science. Adams et al. (2022) have noted that most spatial training studies, albeit at high school level, especially those that examine the correlation between spatial training and mathematics achievement, do not incorporate pedagogical considerations. As such, Lowrie and colleagues (e.g., Lowrie et al., 2019; Lowrie and Logan, 2023; Resnick and Lowrie, 2023) have invested significant efforts in investigating the embedding of spatial ability into pedagogical practices particularly in primary level Mathematics. The general absence of this information in literature has resulted in a constraint for educators to proficiently apply knowledge gained from academic sources in their instructional settings more broadly. Literature has placed a significant emphasis on the cognitive aspect of learning, which has led to the disregard of other factors that may impact learning outcomes (Tan et al., 2021). One such factor is the self-perception of children regarding their own abilities (Chevalier et al., 2009). Psychometric literature illustrates the impact of stereotypical thinking on spatial ability test performance, which is frequently overlooked in training studies. The documented performance disparities between genders on psychometric tests, as well as the influence of external factors such as stereotypical thinking, are frequently overlooked in training studies and spatial ability training reports (Campbell and Collaer, 2009; Pennington et al., 2016; Sanchis-Segura et al., 2018). The development of pedagogical content knowledge (PCK) among teachers is necessary to effectively implement spatial ability training programs (Cordova and Linaugo, 2022).
5.3 Pedagogical content knowledge and professional development
Current PCK theories are focused on subject-specific instruction, such as the teaching of Mathematics. Spatial ability development, conversely, affects various academic fields that provide pupils with prospects for acquiring knowledge in the areas of patterning, operations, shapes, and spatial relationships. Several instances of transdisciplinary pedagogical content knowledge (TPCK) can be identified, including TPCK as proposed by Koehler and Mishra (2009), Design and Technology PCK as described by Doyle et al. (2018), and STEM PCK as explored by Sahin-Topalcengiz and Yildirim (2019). However, there remains a lack of understanding regarding the attributes of teachers’ PCK that can effectively facilitate the development of spatial thinking skills in children (Jo and Bednarz, 2014). Furthermore, there is a lack of research that elucidates teachers’ PCK in the context of teaching spatial thinking in design-oriented classrooms. In a study conducted by Lee (2017), the PCK of preschool teachers in mathematics was examined through a scenario-based approach. The findings revealed that the participants exhibited greater proficiency in PCK pertaining to number sense, measurement, and classification, as compared to their proficiency in PCK related to arranging, procedures, shapes, and spatial relationships. Hong et al. (2019) suggest that teacher training programs possess the capacity to facilitate the augmentation of teachers’ PCK. The attainment of this objective may be realized by means of varied professional development approaches, which encompass cooperative and introspective methodologies among educators. The prioritization of the needs of both educators and learners is a crucial aspect of effective professional development. This prioritization should take into account a range of factors that can impact on these stakeholders, including individual and contextual elements. The active engagement of educators in investigating their own practices is a productive approach to supporting their professional growth, as it encourages reflection (Cochran-Smith and Lytle, 1999; Cabaroglu, 2014). According to Darling-Hammond (2006), the concept of teachers as reflective practitioners implies that through conducting research, educators assume the role of active knowledge generators. This approach enables them to consistently tackle challenges encountered during practical teaching experiences, with the aim of fulfilling the educational requirements of their pupils. According to recent research conducted by Cabaroglu (2014) and Bufasi et al. (2022), action research has demonstrated efficacy as a professional development tool that facilitates active engagement, reflection, and the cultivation of problem-solving skills, ultimately resulting in transformative change.
5.4 The spatialization of classrooms
Within the context of spatial activities, educators frequently voice concerns regarding the necessity for thorough lesson plan preparation that integrates spatial concepts, the absence of concrete instructional materials, and the resulting disregard for prospects to infuse such activities into classroom pedagogy. Costales et al. (2015) have suggested that the integration of spatial activities that facilitate spatial thinking in the classroom can be achieved without the need for Supplementary materials or instruction. Instead, available techniques and resources can be employed to incorporate spatial elements into classrooms and educational programs. The integration of various teaching strategies has been suggested to enhance the learning experience of children. This includes the utilization of a diverse spatial vocabulary within the classroom setting (Newcombe and Frick, 2010), encouraging the exchange of ideas between children through the extension of conversations (Chan et al., 2020), and the use of multiple examples, such as visuals and representations, to aid in the comprehension of abstract concepts (Teixidor-i-Bigas et al., 2013; Bautista et al., 2015). Additionally, teachers can engage pupils in solution discussions by asking them to explain their reasoning and thought processes, and by modeling problem-solving strategies through verbalization of the steps taken to reach a solution (Chan et al., 2020).
6 Conclusion
The complicated nature of teaching spatial ability development is multifaceted, as numerous components are interrelated. These components may include important factors such as school administration, teacher attitudes toward change, availability of instructional materials, and professional training for teachers. One significant observation from this review is that there appears a challenge, inferred through a paucity in literature, in the integration of these elements for spatial ability development. There are for example pedagogical enablers, but these can be limited in impact through a lack of curricular level detailing. Further, and illustrating the value of considering mathematics and science collectively, there appears more research and curricular specificity relating to spatial ability in primary level Mathematics than Science. Given than primary level education is typically a general education, this siloing may not be to the detriment of children if spatial ability development is incorporated into Mathematics then children will still receive the benefit. However, the relationship between spatial ability and science education has been observed so it would appear valuable at policy and practice levels to acknowledge this such that (1) this and other curricular areas may also serve for embedded spatial ability development and that (2) pedagogical approaches across the curriculum may better acknowledge levels of spatial ability to better support learning where relevant. Our main findings provide a path for further research to develop each of these components based on the enablers and barriers established. The consolidation of these barriers and enablers can also contribute as a lens to a broader framework for the integration of spatial ability development into primary level education in terms of enacted practice. Additionally, our findings emphasize the necessity of providing teachers with professional development opportunities to enhance and strengthen their knowledge about spatial ability and spatial activities.
7 Recommendations
Several curricula exhibit an extensive level of organization in integrating spatial components, with the explicit distinction of objectives and goals. Conversely, other curricula incorporate spatial components in a diffused or vague manner across various topics, leading educators to perceive spatial abilities as a distinct subject that requests inclusion in the curriculum. Therefore, it is crucial to provide adequate professional development to teachers to enhance their comprehension of the significance of spatial skills, their correlation with science and mathematics accomplishments, and the potential of such training to enhance teachers’ content knowledge through collaborative efforts and the development of innovative pedagogical frameworks. Additionally, the introduction of a well-defined rubric that teachers can utilize to assess the progress of individual pupils would be immensely beneficial, given that teachers may encounter difficulties in providing parents with outcomes while implementing spatial activities. Moreover, the integration of diverse manipulative resources, such as blocks, tangrams, and dienes blocks, into the pre-existing instructional practices that teachers address during the lesson may serve as a possible solution for one of the difficulties that teachers commonly encounter with respect to time limitations and the need to generate new tasks. Furthermore, teachers need to employ a diverse range of illustrations as this enhances pupils’ spatial visualization skills through exposure to multiple visual representations.
Author contributions
EB: Conceptualization, Data curation, Formal analysis, Investigation, Validation, Visualization, Writing – original draft, Writing – review & editing. TL: Data curation, Formal analysis, Investigation, Writing – review & editing. UB: Data curation, Formal analysis, Investigation, Writing – review & editing. MW: Data curation, Formal analysis, Investigation, Writing – review & editing. RM: Data curation, Formal analysis, Investigation, Writing – review & editing. DN: Conceptualization, Investigation, Methodology, Project administration, Resources, Supervision, Validation, Writing – review & editing. ID: Data curation, Resources, Supervision, Writing – review & editing. SS: Data curation, Project administration, Resources, Supervision, Writing – review & editing. LG: Data curation, Resources, Supervision, Writing – review & editing. RK: Conceptualization, Project administration, Resources, Supervision, Writing – review & editing. JS: Conceptualization, Resources, Supervision, Writing – review & editing. BB: Conceptualization, Resources, Supervision, Writing – review & editing. CO'K: Conceptualization, Resources, Supervision, Writing – review & editing. GD: Conceptualization, Investigation, Project administration, Resources, Supervision, Writing – review & editing. MP: Conceptualization, Resources, Supervision, Writing – review & editing. JB: Conceptualization, Resources, Supervision, Writing – review & editing, Data curation, Investigation, Methodology, Visualization, Writing – original draft.
Funding
The author(s) declare financial support was received for the research, authorship, and/or publication of this article. This research is part of the SellSTEM - Spatially Enhanced Learning Linked to STEM - Marie Skłodowska-Curie Innovative Training Network to investigate the role of spatial ability in and for STEM learning. It has received funding from the European Union’s Horizon 2020 research and innovation programme under the Marie Skłodowska-Curie grant agreement No. 956124.
Conflict of interest
The authors declare that the research was conducted in the absence of any commercial or financial relationships that could be construed as a potential conflict of interest.
Publisher’s note
All claims expressed in this article are solely those of the authors and do not necessarily represent those of their affiliated organizations, or those of the publisher, the editors and the reviewers. Any product that may be evaluated in this article, or claim that may be made by its manufacturer, is not guaranteed or endorsed by the publisher.
Supplementary material
The Supplementary material for this article can be found online at: https://www.frontiersin.org/articles/10.3389/feduc.2024.1306189/full#supplementary-material
References
Adams, J., Resnick, I., and Lowrie, T. (2022). Supporting senior high-school students’ measurement and geometry performance: does spatial training transfer to mathematics achievement? Math. Educ. Res. J. 35, 879–900. doi: 10.1007/s13394-022-00416-y
Applebee, D., Bennett-Day, B., Ferrari, J., Pritchard, P., and Boettger-Tong, H. (2021). Multimodal training improves spatial reasoning skills in female college students. J. Sci. Educ. Technol. 30, 539–549. doi: 10.1007/s10956-020-09898-6
Arundel, S. T., and Li, W. (2021). The evolution of geospatial reasoning, analytics, and modeling. In The geographic information science & technology body of knowledge 3rd Quarter 2021 Edition, Wilson, John P. (Ed.), 2021. 57. 1–32
Başak, K. Ö. K., and Davasligil, Ü. (2014). The effect of teaching geometry which is differentiated based on the parallel curriculum for gifted/talented students on spatial ability. J. Educ. Gifted Young Scientists 2, 40–52. doi: 10.17478/JEYSG.201419012
Bautista, A., Cañadas, M. C., Brizuela, B. M., and Schliemann, A. D. (2015). Examining how teachers use graphs to teach mathematics during a professional development program. J. Educ. Train. Stud. 3, 91–106. doi: 10.11114/jets.v3i2.676
Bergey, B. W., Cromley, J. G., and Newcombe, N. S. (2015). Teaching high school biology students to coordinate text and diagrams: relations with transfer, effort, and spatial skill. Int. J. Sci. Educ. 37, 2476–2502. doi: 10.1080/09500693.2015.1082672
Bodzin, A. M. (2011). The implementation of a geospatial information technology (GIT)-supported land use change curriculum with urban middle school learners to promote spatial thinking. J. Res. Sci. Teach. 48, 281–300. doi: 10.1002/tea.20409
Bower, C., Odean, R., Verdine, B. N., Medford, J. R., Marzouk, M., Golinkoff, R. M., et al. (2020). Associations of 3-year-olds’ block-building complexity with later spatial and mathematical skills. J. Cogn. Dev. 21, 383–405. doi: 10.1080/15248372.2020.1741363
Buckley, J. (2023). “Historical and philosophical origins of technology education” in The Bloomsbury handbook of technology education: perspectives and practice. eds. D. Gill, D. Irving-Bell, M. McLain, and D. Wooff (London: Bloomsbury), 14–27.
Buckley, J., Seery, N., and Canty, D. (2018). A heuristic framework of spatial ability: a review and synthesis of spatial factor literature to support its translation into STEM education. Educ. Psychol. Rev. 30, 947–972. doi: 10.1007/s10648-018-9432-z
Buckley, J., Seery, N., and Canty, D. (2019). Investigating the use of spatial reasoning strategies in geometric problem solving. Int. J. Technol. Des. Educ. 29, 341–362. doi: 10.1007/s10798-018-9446-3
Bufasi, E., Cakane, I., Dudareva, I., and Namsone, D. (2022). A Preliminary study on spatial ability for primary school teachers' professional development. 14th annual International Conference on Education and New Learning Technologies
Burte, H., Gardony, A. L., Hutton, A., and Taylor, H. A. (2020). Elementary teachers’ attitudes and beliefs about spatial thinking and mathematics. Cogn. Res. 5, 17–18. doi: 10.1186/s41235-020-00221-w
Cabaroglu, N. (2014). Professional development through action research: Impact on self-efficacy. System 44, 79–88. doi: 10.1016/j.system.2014.03.003
Caldera, Y. M., Culp, A. M., O’Brien, M., Truglio, R. T., Alvarez, M., and Huston, A. C. (1999). Children’s play preferences, construction play with blocks, and visual-spatial skills: are they related? Int. J. Behav. Dev. 23, 855–872. doi: 10.1080/016502599383577
Campbell, S. M., and Collaer, M. L. (2009). Stereotype threat and gender differences in performance on a novel visuospatial task. Psychology of Women Quarterly 33, 437–444. doi: 10.1111/j.1471-6402.2009.01521.x
Casasola, M., Wei, W. S., Suh, D. D., Donskoy, P., and Ransom, A. (2020). Children’s exposure to spatial language promotes their spatial thinking. J. Exp. Psychol. Gen. 149, 1116–1136. doi: 10.1037/xge0000699
Castro-Alonso, J. C., and Uttal, D. H. (2019). “Science education and visuospatial processing” in Visuospatial processing for education in health and natural sciences, Cham: Springer (Ed. Castro-Alonso, J.C.) 53–79
Chan, J. Y. C., Praus-Singh, T. L., and Mazzocco, M. M. (2020). Parents’ and young children’s attention to mathematical features varies across play materials. Early Child. Res. Q. 50, 65–77. doi: 10.1016/j.ecresq.2019.03.002
Chen Musgrove, M. M., Nied, S., Cooley, A., Schinske, J. N., and Corwin, L. A. (2022). Engaging with CC bio INSITES: experiences of barriers, supports, and belonging in community college faculty participating in biology education research. CBE—life sciences. Education 21:ar16. doi: 10.1187/cbe.21-09-0246
Chevalier, A., Gibbons, S., Thorpe, A., Snell, M., and Hoskins, S. (2009). Students’ academic self-perception. Economics of Education Review 28, 716–727. doi: 10.1016/j.econedurev.2009.06.007
Chittleborough, G., and Treagust, D. F. (2007). The modelling ability of non-major chemistry students and their understanding of the sub-microscopic level. Chem. Educ. Res. Pract. 8, 274–292. doi: 10.1039/B6RP90035F
Christidou, D., Voulgari, I., Tisza, G., Norouzi, B., Kinnula, M., Iivari, N., et al. (2022). Obstacles and challenges identified by practitioners of non-formal science learning activities in Europe. Int. J. Sci. Educ. 44, 514–533. doi: 10.1080/09500693.2022.2035466
Cochran-Smith, M., and Lytle, S. L. (1999). Chapter 8: Relationships of knowledge and practice: Teacher learning in communities. Review of research in education 24, 249–305. doi: 10.3102/0091732X024001249
Coenders, F., and Verhoef, N. (2019). Lesson study: professional development (PD) for beginning and experienced teachers. Prof. Dev. Educ. 45, 217–230. doi: 10.1080/19415257.2018.1430050
Cole, M., Wilhelm, J., Vaught, B. M. M., Fish, C., and Fish, H. (2020). The relationship between spatial ability and the conservation of matter in middle school. Educ. Sci. 11:4. doi: 10.3390/educsci11010004
Collins, L. (2018). The impact of paper versus digital map technology on students' spatial thinking skill acquisition. J. Geogr. 117, 137–152. doi: 10.1080/00221341.2017.1374990
Cook, T. D., Campbell, D. T., and Shadish, W. (2002). Experimental and quasi-experimental designs for generalized causal inference (Vol. 1195). Boston, MA: Houghton Mifflin.
Cordova, W. M., and Linaugo, J. D. (2022). Pedagogical content knowledge practices of public school science teachers. Technium Soc. Sci. J. 37:37. doi: 10.47577/tssj.v37i1.7584
Costales, A., Abad, C., Odean, R., Pruden, S. M., and Scarlett, I. G. (2015). “Spatial activities and manipulatives for early education classrooms” in Ed. G. Scarlett The SAGE encyclopedia of classroom management, Thousand Oaks, CA: Sage Publication, 768–771.
Darling-Hammond, L. (2006). Constructing 21st-century teacher education. Journal of teacher education 57, 300–314. doi: 10.1177/0022487105285962
Darwish, M., Kamel, S., and Assem, A. (2023). Extended reality for enhancing spatial ability in architecture design education. Ain Shams Eng. J. 14:102104. doi: 10.1016/j.asej.2022.102104
Desimone, L. (2002). How can comprehensive school reform models be successfully implemented? Rev. Educ. Res. 72, 433–479. doi: 10.3102/00346543072003433
Dilling, F., and Vogler, A. (2021). Fostering spatial ability through computer-aided design: a case study. Digit. Exp. Math. Educ. 7, 323–336. doi: 10.1007/s40751-021-00084-w
DiPerna, J. C., and Elliott, S. N. (2002). Promoting academic enablers to improve student achievement: an introduction to the mini-series. Sch. Psychol. Rev. 31, 293–297. doi: 10.1080/02796015.2002.12086156
Doyle, A., Seery, N., Gumaelius, L., Canty, D., and Hartell, E. (2018). Reconceptualising PCK research in D&T education: proposing a methodological framework. In Research and Practice in Technology Education-Perspectives on Human Capacity and Development–Proceedings of the 2018 Pupils’ Attitude Towards Technology (PATT) conference 363–370
Endreny, A. H. (2010). Urban 5th graders conceptions during a place-based inquiry unit on watershed. Journal of Research in Science Teaching 47, 501–517. doi: 10.1002/tea.20348
Fatemah, A., Rasool, S., and Habib, U. (2020). Interactive 3D visualization of chemical structure diagrams embedded in text to aid spatial learning process of students. J. Chem. Educ. 97, 992–1000. doi: 10.1021/acs.jchemed.9b00690
Friedrichsen, P. J., Abell, S. K., Pareja, E. M., Brown, P. L., Lankford, D. M., and Volkmann, M. J. (2009). Does teaching experience matter? Examining biology teachers' prior knowledge for teaching in an alternative certification program. J. Res. Sci. Teach. 46, 357–383. doi: 10.1002/tea.20283
Fulton, K., and Britton, T. (2011). STEM teachers in professional learning communities: From good teachers to great teaching National Commission on Teaching and America's Future. (Washington, DC)
Gagnier, K. M., Atit, K., Ormand, C. J., and Shipley, T. F. (2017). Comprehending 3D diagrams: Sketching to support spatial reasoning. Topics in cognitive science 9, 883–901. doi: 10.1111/tops.12233
Gagnier, K. M., Holochwost, S. J., and Fisher, K. R. (2022). Spatial thinking in science, technology, engineering, and mathematics: elementary teachers' beliefs, perceptions, and self-efficacy. J. Res. Sci. Teach. 59, 95–126. doi: 10.1002/tea.21722
Garg, G. N., Spero, L., and Ian Taylor, A. (1999). Learning anatomy: do new computer models improve spatial understanding? Med. Teach. 21, 519–522. doi: 10.1080/01421599979239
Geer, E. A., Quinn, J. M., and Ganley, C. M. (2019). Relations between spatial skills and math performance in elementary school children: a longitudinal investigation. Dev. Psychol. 55, 637–652. doi: 10.1037/dev0000649
Gilligan, K. A., Flouri, E., and Farran, E. K. (2017). The contribution of spatial ability to mathematics achievement in middle childhood. J. Exp. Child Psychol. 163, 107–125. doi: 10.1016/j.jecp.2017.04.016
Gilligan, K. A., Hodgkiss, A., Thomas, M. S., and Farran, E. K. (2019). The developmental relations between spatial cognition and mathematics in primary school children. Dev. Sci. 22:e12786. doi: 10.1111/desc.12786
Green, B. N., Johnson, C. D., and Adams, A. (2006). Writing narrative literature reviews for peer-reviewed journals: secrets of the trade. J. Chiropr. Med. 5, 101–117. doi: 10.1016/S0899-3467(07)60142-6
Gujarati, J. (2013). Deepening mathematics teaching and learning through the concrete-pictorial-abstract approach. Strategies Successful Learn. 6, 1–9.
Hawes, Z. C., Gilligan-Lee, K. A., and Mix, K. S. (2022). Effects of spatial training on mathematics performance: a meta-analysis. Dev. Psychol. 58, 112–137. doi: 10.1037/dev0001281
Hawes, Z. C., Gilligan-Lee, K. A., and Mix, K. S. (2023). “Infusing spatial thinking into elementary and middle school mathematics: what, why, and how?” in Ed. Robinson, K., Dubé, A., & Kotsopoulos, D. Mathematical cognition and understanding: Perspectives on mathematical minds in the elementary and middle school years (Cham: Springer International Publishing), 13–33.
Hennessy, S., D'Angelo, S., McIntyre, N., Koomar, S., Kreimeia, A., Cao, L., et al. (2022). Technology use for teacher professional development in low-and middle-income countries: a systematic review. Comput. Educ. Open 3:100080. doi: 10.1016/j.caeo.2022.100080
Hodgkiss, A., Gilligan, K. A., Tolmie, A. K., Thomas, M. S., and Farran, E. K. (2018). Spatial cognition and science achievement: the contribution of intrinsic and extrinsic spatial skills from 7 to 11 years. Br. J. Educ. Psychol. 88, 675–697. doi: 10.1111/bjep.12211
Hong, H. Y., Lin, P. Y., Chai, C. S., Hung, G. T., and Zhang, Y. (2019). Fostering design-oriented collective reflection among preservice teachers through principle-based knowledge building activities. Comput. Educ. 130, 105–120. doi: 10.1016/j.compedu.2018.12.001
Horikoshi, R. (2021). Teaching chemistry with LEGO® bricks. Chem. Teach. Int. 3, 239–255. doi: 10.1515/cti-2020-0017
Jadallah, M., Hund, A. M., Thayn, J., Studebaker, J. G., Roman, Z. J., and Kirby, E. (2017). Integrating geospatial technologies in fifth-grade curriculum: impact on spatial ability and map-analysis skills. J. Geogr. 116, 139–151. doi: 10.1080/00221341.2017.1285339
Jee, B. D., Uttal, D. H., Gentner, D., Manduca, C., Shipley, T. F., and Sageman, B. (2013). Finding faults: analogical comparison supports spatial concept learning in geoscience. Cogn. Process. 14, 175–187. doi: 10.1007/s10339-013-0551-7
Jirout, J. J., and Newcombe, N. S. (2015). Building blocks for developing spatial skills: evidence from a large, representative US sample. Psychol. Sci. 26, 302–310. doi: 10.1177/0956797614563338
Jo, I., and Bednarz, S. W. (2014). Developing pre-service teachers' pedagogical content knowledge for teaching spatial thinking through geography. J. Geogr. High. Educ. 38, 301–313. doi: 10.1080/03098265.2014.911828
Juhler, M. V. (2016). The use of lesson study combined with content representation in the planning of physics lessons during field practice to develop pedagogical content knowledge. J. Sci. Teach. Educ. 27, 533–553. doi: 10.1007/s10972-016-9473-4
Kell, H. J., Lubinski, D., Benbow, C. P., and Steiger, J. H. (2013). Creativity and technical innovation: spatial ability’s unique role. Psychol. Sci. 24, 1831–1836. doi: 10.1177/0956797613478615
Kiernan, N. A., Manches, A., and Seery, M. K. (2021). The role of visuospatial thinking in students’ predictions of molecular geometry. Chem. Educ. Res. Pract. 22, 626–639. doi: 10.1039/D0RP00354A
Koehler, M., and Mishra, P. (2009). What is technological pedagogical content knowledge (TPACK)? Contemporary issues in technology and teacher education, 9, 60–70.
Kozhevnikov, M., Motes, M. A., and Hegarty, M. (2007). Spatial visualization in physics problem solving. Cogn. Sci. 31, 549–579. doi: 10.1080/15326900701399897
Kurumeh, M. S., Chiawa, M. A., and Ibrahim, M. O. (2010). Dienes multibase Blocks' approach an effective strategy for improving Students' interest in number bases among secondary school students in mathematics. Res. J. Math. Stat. 2, 101–104.
Leavy, A. M., and Hourigan, M. (2016). Using lesson study to support knowledge development in initial teacher education: insights from early number classrooms. Teach. Teach. Educ. 57, 161–175. doi: 10.1016/j.tate.2016.04.002
Lee, B., and Kim, Y. (2018). “The effects of S-Block curriculum on kindergartenchildren’s logical-mathematical and spatial ability” in TENCON 2018-2018 IEEE Region 10 Conference (IEEE), 0802–0805. doi: 10.1109/TENCON.2018.8650161
Lee, J., and Bednarz, R. (2012). Components of spatial thinking: evidence from a spatial thinking ability test. J. Geogr. 111, 15–26. doi: 10.1080/00221341.2011.583262
Leutner, D., Leopold, C., and Sumfleth, E. (2009). Cognitive load and science text comprehension: Effects of drawing and mentally imagining text content. Computers in Human Behavior 25, 284–289. doi: 10.1016/j.chb.2008.12.010
Lin, T.-J., Buckley, J., Gumaelius, L., and Ampadu, E. (2023). “Situating spatial ability development in the craft and technology curricula of Swedish compulsory education” in The 40th international pupils’ attitudes towards technology conference proceedings 2023 (Liverpool, UK: Liverpool John Moores University)
Lee, J. E. (2017). Preschool teachers’ pedagogical content knowledge in mathematics. International Journal of Early Childhood 49, 229–243. doi: 10.1007/s13158-017-0189-1
Lowrie, T., and Logan, T. (2023). Spatial visualization supports students’ math: mechanisms for spatial transfer. J. Intelligence 11:127. doi: 10.3390/jintelligence11060127
Lowrie, T., Logan, T., and Hegarty, M. (2019). The influence of spatial visualization training on students’ spatial reasoning and mathematics performance. J. Cogn. Dev. 20, 729–751. doi: 10.1080/15248372.2019.1653298
Magnusson, S.J., Krajcik, J.S., and Borko, H. (1999). Nature, sources, and development of pedagogical content knowledge for science teaching In Examining pedagogical content knowledge: The construct and its implications for science education 95–132. Dordrecht: Springer Netherlands.
Matahela, V. E., and van Rensburg, G. H. (2022). Motivation as a facilitator of self-leadership in nurse academics. Heliyon 8:e09580. doi: 10.1016/j.heliyon.2022.e09580
Miller, D. I., and Halpern, D. F. (2013). Can spatial training improve long-term outcomes for gifted STEM undergraduates? Learn. Individ. Differ. 26, 141–152. doi: 10.1016/j.lindif.2012.03.012
Mix, K. S. (2019). Why are spatial skill and mathematics related? Child Development Perspectives 13, 121–126. doi: 10.1111/cdep.12323
Mix, K. S., Levine, S. C., Cheng, Y. L., Young, C. J., Hambrick, D. Z., and Konstantopoulos, S. (2017). The latent structure of spatial skills and mathematics: a replication of the two-factor model. J. Cogn. Dev. 18, 465–492. doi: 10.1080/15248372.2017.1346658
Mix, K. S., Levine, S. C., Cheng, Y. L., Young, C., Hambrick, D. Z., Ping, R., et al. (2016). Separate but correlated: the latent structure of space and mathematics across development. J. Exp. Psychol. Gen. 145, 1206–1227. doi: 10.1037/xge0000182
Mullis, I. V. S., Martin, M. O., Foy, P., and Hooper, M. (2016). TIMSS 2015 international results in mathematics. Retrieved from Boston College, TIMSS & PIRLS International Study Center website: Available at: https://timssandpirls.bc.edu/timss2015/international-results/
National Centre for Education Latvia, (2019b). Skola2030. Latvian primary mathematics curriculum. Available at: https://mape.skola2030.lv/resources/159 (Accessed April 16, 2023).
National Centre for Education Latvia, (2019a). Skola2030. Latvian primary science curriculum. Available at: https://mape.skola2030.lv/resources/124 (Accessed April 16, 2023).
National Council for Curriculum and Assessment. (1999). Primary mathematics curriculum. Available at: https://www.curriculumonline.ie/getmedia/9df5f3c5-257b-471e-8d0f-f2cf059af941/PSEC02_Mathematics_Curriculum.pdf
National Research Council. (2005). Division on Earth, Life Studies, Board on Earth Sciences, Geographical Sciences Committee, Committee on Support for Thinking Spatially, & The Incorporation of Geographic Information Science Across the K-12 Curriculum. (2005). Learning to think spatially. National Academies Press.
Newcombe, N. S. (2010). Picture this: increasing math and science learning by improving spatial thinking. Am. Educ. 10, 1–6. doi: 10.1016/j.cobeha.2016.04.010
Newcombe, N. S., and Frick, A. (2010). Early education for spatial intelligence: why, what, and how. Mind Brain Educ. 4, 102–111. doi: 10.1111/j.1751-228X.2010.01089.x
Newcombe, N. S., and Shipley, T. F. (2014). “Thinking about spatial thinking: new typology, new assessments” in Ed. Gero, J. Studying visual and spatial reasoning for design creativity (Dordrecht: Springer Netherlands), 179–192.
Okoye, K., Hussein, H., Arrona-Palacios, A., Quintero, H. N., Ortega, L. O. P., Sanchez, A. L., et al. (2022). Impact of digital technologies upon teaching and learning in higher education in Latin America: an outlook on the reach, barriers, and bottlenecks. Educ. Inf. Technol. 28, 2291–2360. doi: 10.1007/s10639-022-11214-1
Olkun, S., Altun, A., and Smith, G. (2005). Computers and 2D geometric learning of Turkish fourth and fifth graders. Br. J. Educ. Technol. 36, 317–326. doi: 10.1111/j.1467-8535.2005.00460.x
Oostermeijer, M., Boonen, A. J., and Jolles, J. (2014). The relation between children’s constructive play activities, spatial ability, and mathematical word problem-solving performance: a mediation analysis in sixth-grade students. Front. Psychol. 5:782. doi: 10.3389/fpsyg.2014.00782
Park, S., and Oliver, J. S. (2008). Revisiting the conceptualisation of pedagogical content knowledge (PCK): PCK as a conceptual tool to understand teachers as professionals. Research in science Education 38, 261–284. doi: 10.1007/s11165-007-9049-6
Pennington, C. R., Heim, D., Levy, A. R., and Larkin, D. T. (2016). Twenty years of stereotype threat research: A review of psychological mediators. PloS one 11:e0146487. doi: 10.1371/journal.pone.0146487
Pollitt, R., Cohrssen, C., and Seah, W. T. (2020). Assessing spatial reasoning during play: Educator observations, assessment and curriculum planning. Mathematics Education Research Journal 32, 331–363. doi: 10.1007/s13394-020-00337-8
Rahmawati, Y., Dianhar, H., and Arifin, F. (2021). Analysing students’ spatial abilities in chemistry learning using 3D virtual representation. Education Sciences 11:185. doi: 10.3390/educsci11040185
Resnick, I., and Lowrie, T. (2023). Spatial reasoning supports preschool numeracy: findings from a large-scale nationally representative randomized control trial. J. Res. Math. Educ. 54, 295–316. doi: 10.5951/jresematheduc-2022-0051
Richardson, M., Hunt, T. E., and Richardson, C. (2014). Children's construction task performance and spatial ability: controlling task complexity and predicting mathematics performance. Percept. Mot. Skills 119, 741–757. doi: 10.2466/22.24.PMS.119c28z8
Rodriguez, J., and Rodriguez-Velazquez, L. G. (2020). Data analytics on improvement of spatial visualization skills. Proced. Comput. Sci. 172, 106–111. doi: 10.1016/j.procs.2020.05.015
Rohmah, M., and Indriati, D. (2021). “Hass’s theory: how is the students’ spatial intelligence in solving problems?” in International conference of mathematics and mathematics education (I-CMME 2021) (Netherlands: Atlantis Press), 169–175.
Sahin-Topalcengiz, E., and Yildirim, B. (2019). The development and validation of Turkish version of the elementary teachers’ efficacy and attitudes towards STEM (ET-STEM) scale. Journal of Education in Science Environment and Health 5, 12–35. doi: 10.21891/jeseh.486787
Salame, I. I., and Kabir, S. A. (2022). Examining students’ spatial ability and its impact on the learning of stereochemistry. Interdiscip. J. Environ. Sci. Educ. 18:e2288. doi: 10.21601/ijese/12099
Salimi, M., Suhartono, S., Hidayah, R., and Fajari, L. E. W. (2020). Improving mathematics learning of geometry through the concrete-pictorial-abstract (CPA) approach: collaborative action research. J. Physics 1663:12046.
Sanchis-Segura, C., Aguirre, N., Cruz-Gómez, Á. J., Solozano, N., and Forn, C. (2018). Do gender-related stereotypes affect spatial performance? Exploring when, how and to whom using a chronometric two-choice mental rotation task. Frontiers in Psychology 9:398111. doi: 10.3389/fpsyg.2018.01261
Schoepp, K. (2005). Barriers to technology integration in a technology-rich environment. Learn. Teach. Higher Educ. 2, 56–79. doi: 10.18538/lthe.v2.n1.02
Schön, D. (1983). The reflective practitioner: How professionals think in action. London: Temple Smith.
Shipley, T. F., Tikoff, B., Ormand, C., and Manduca, C. (2013). Structural geology practice and learning, from the perspective of cognitive science. J. Struct. Geol. 54, 72–84. doi: 10.1016/j.jsg.2013.07.005
Shulman, L. (1987). Knowledge and teaching: foundations of the new reform. Harv. Educ. Rev. 57, 1–23. doi: 10.17763/haer.57.1.j463w79r56455411
Skolverket (2018). Curriculum for the compulsory school, preschool class and school-age educare. Available at: https://www.skolverket.se/download/18.31c292d516e7445866a218f/1576654682907/pdf3984.pdf (Accessed April 13, 2023)
Sorby, S. A., Duffy, G., and Loney, N. (2020). An examination of the role of spatial ability in the process of problem solving in chemical engineering. Australas. J. Eng. Educ. 25, 55–65. doi: 10.1080/22054952.2020.1785653
Sorby, S., Veurink, N., and Streiner, S. (2018). Does spatial skills instruction improve STEM outcomes? The answer is “yes.”. Learn. Individ. Differ. 67, 209–222. doi: 10.1016/j.lindif.2018.09.001
Tan, J., Mao, J., Jiang, Y., and Gao, M. (2021). The influence of academic emotions on learning effects: a systematic review. International journal of environmental research and public health 18:9678. doi: 10.3390/ijerph18189678
Taylor, H. A., and Hutton, A. (2013). Think3d!: training spatial thinking fundamental to STEM education. Cogn. Instr. 31, 434–455. doi: 10.1080/07370008.2013.828727
Teixidor-i-Bigas, M., Schliemann, A. D., and Carraher, D. W. (2013). Integrating disciplinary perspectives: the poincaré institute for mathematics education. Math. Enthusiast 10, 519–562. doi: 10.54870/1551-3440.1279
ten Brummelhuis, A., Wijngaards, G., Swager, P., and van Goozen, B. (2010). ICT in initial teacher training. Paris: Organisation For Economic Co-operation and Development. Available at: https://www.oecd.org/sweden/45046846.pdf
Träff, U., Olsson, L., Skagerlund, K., Skagenholt, M., and Östergren, R. (2019). Logical reasoning, spatial processing, and verbal working memory: longitudinal predictors of physics achievement at age 12–13 years. Front. Psychol. 10:1929. doi: 10.3389/fpsyg.2019.01929
Tuckey, H., Selvaratnam, M., and Bradley, J. (1991). Identification and rectification of student difficulties concerning three-dimensional structures, rotation, and reflection. J. Chem. Educ. 68:460. doi: 10.1021/ed068p460
Türkmenoglu Berkan, S., Öztas, S. K., Kara, F. İ., and Vardar, A. E. (2020). The role of spatial ability on architecture education. Design Technol. Educ. 25, 103–126.
Uttal, D. H., Meadow, N. G., Tipton, E., Hand, L. L., Alden, A. R., Warren, C., et al. (2013). The malleability of spatial skills: a meta-analysis of training studies. Psychol. Bull. 139, 352–402. doi: 10.1037/a0028446
Van Driel, J. H., and Berry, A. (2012). Teacher professional development focusing on pedagogical content knowledge. Educ. Res. 41, 26–28. doi: 10.3102/0013189X11431010
Verdine, B. N., Golinkoff, R. M., Hirsh-Pasek, K., Newcombe, N. S., and Bailey, D. H. (2017). Links between spatial and mathematical skills across the preschool years. Monogr. Soc. Res. Child Dev. 82, 71–80. doi: 10.1111/mono.12283
Wai, J., Lubinski, D., and Benbow, C. P. (2009). Spatial ability for STEM domains: aligning over 50 years of cumulative psychological knowledge solidifies its importance. J. Educ. Psychol. 101, 817–835. doi: 10.1037/a0016127
Webb, R. M., Lubinski, D., and Benbow, C. P. (2007). Spatial ability: a neglected dimension in talent searches for intellectually precocious youth. J. Educ. Psychol. 99, 397–420. doi: 10.1037/0022-0663.99.2.397
Whittaker, D., Dunbar, R., and Buckley, J. (2022). “An analysis of the Irish junior cycle graphics specification to explore the treatment of spatial cognition” in Ed. Gill, D., Kennedy, T., Pendergast, S., & Jamil, S. PATT2022: PATT on the edge: technology, innovation, and education (Newfoundland & Labrador, Canada: Memorial University)
Yang, J. C., and Chen, S. Y. (2010). Effects of gender differences and spatial abilities within a digital pentominoes game. Comput. Educ. 55, 1220–1233. doi: 10.1016/j.compedu.2010.05.019
Zhu, C., Leung, C. O.-Y., Lagoudaki, E., Velho, M., Segura-Caballero, N., Jolles, D., et al. (2023). Fostering spatial ability development in and for authentic STEM learning. Front. Educ. 8:1138607. doi: 10.3389/feduc.2023.1138607
Keywords: spatial ability, barriers, enablers, primary school, mathematics education, science education, curriculum
Citation: Bufasi E, Lin TJ, Benedicic U, Westerhof M, Mishra R, Namsone D, Dudareva I, Sorby S, Gumaelius L, Klapwijk RM, Spandaw J, Bowe B, O'Kane C, Duffy G, Pagkratidou M and Buckley J (2024) Addressing the complexity of spatial teaching: a narrative review of barriers and enablers. Front. Educ. 9:1306189. doi: 10.3389/feduc.2024.1306189
Edited by:
Chunxia Qi, Beijing Normal University, ChinaReviewed by:
Hui Luan, National Taiwan Normal University, TaiwanSara Germani, Sapienza University of Rome, Italy
Tracy Logan, University of Canberra, Australia
Copyright © 2024 Bufasi, Lin, Benedicic, Westerhof, Mishra, Namsone, Dudareva, Sorby, Gumaelius, Klapwijk, Spandaw, Bowe, O’Kane, Duffy, Pagkratidou and Buckley. This is an open-access article distributed under the terms of the Creative Commons Attribution License (CC BY). The use, distribution or reproduction in other forums is permitted, provided the original author(s) and the copyright owner(s) are credited and that the original publication in this journal is cited, in accordance with accepted academic practice. No use, distribution or reproduction is permitted which does not comply with these terms.
*Correspondence: Ergi Bufasi, ZXJnaS5idWZhc2lAbHUubHY=