- 1School of Engineering and Sciences, Tecnologico de Monterrey, Monterrey, NL, Mexico
- 2Writing Lab, Institute for the Future of Education, Tecnologico de Monterrey, Monterrey, Nuevo Leon, Mexico
Traditionally, laboratory work has been a common approach to facilitate the acquisition of practical skills and familiarize the undergraduate students, particularly engineering students, with specialized tools and equipment. However, the conventional in-person labs often experience challenges such as limited resources and space, instructor availability, and inflexible schedules. The emergence of digital tools and the recent COVID-19 pandemic have prompted educators to reconsider their teaching methods. To this effect, this paper introduces an innovative approach and teaching methodology to address the challenges in traditional engineering education within the laboratory. It presents a methodology that combines cost-effective instructional videos and portable kits to promote autonomous development of practical skills in undergraduate engineering students. The proposed teaching methodology was grounded on the Descriptive Decision theory and Learning-Oriented Assessment (LOA), which are theoretical framework and type of students’ learning outcome (SLO) model that studies the rationality behind the decisions that the users are disposed to make, as well as level of outcome of the students’ learning process or performance. Motivation among the students was assessed using the Model of Academic Motivation Inventory (MUSIC Inventory) to evaluate the impact of the proposed teaching method and learning intervention on the interest of the students. The method was implemented in four independent courses at two different campuses of Tecnologico de Monterrey. The results show that the proposed learning approach was effective in helping students develop hard skills with reduced instructor intervention. Moreover, high levels of motivation was reported through the MUSIC methodology and test administered to the participating students at the end of the courses. The outcome of this study can be used to inform and support the curriculum design by the educators, promote effective policy and decision making by the university leaders, and encourage wide adoption of the digitalized-education.
1 Introduction
Undergraduate engineering education relies heavily on instructional laboratories as an indispensable platform for substantial part of the instruction and course delivery (Dym et al., 2005; Jiang and Pang, 2023; Lara-Prieto et al., 2023). For example, in a January 2002 colloquy organized by the Sloan Foundation at the request of ABET, engineering educators outlined thirteen objectives for instructional laboratories (Feisel and Peterson, 2002). These objectives includes, to name but a few, especially in connection to the work done in this study, how to familiarize students with instrumentation and experimental analysis to allow them identify strengths and limitations of theoretical models, expose the students to product/system design processes with aim to promote their creativity and to allow them to learn from failure, and to develop proficiency in using specialized tools while ensuring safety (Feisel and Rosa, 2005). Given the significance of these objectives, it can be said that while the engineering education is beginning to adopt the benefits of instructional laboratories. However, traditional instructions in engineering laboratories still face some drawbacks, mainly due to limited space and human resources, as pointed out in some works (Judge, 2017). Additionally, the schedules established by universities and colleges to control the hours during which students can make use of laboratories, along with the available time a student can work in a practice, could limit the availability of the students to progress at their own pace, potentially hindering their skill acquisition and development. Along these lines, the authors note that the advent of portable computers and the widespread and use of the internet have brought about significant changes in the learning process (IEEE, 2020; Okoye et al., 2021; UNESCO, 2021), offering an opportunity to address and overcome the obstacles faced by the traditional engineering laboratories (Dym et al., 2005; Jiang and Pang, 2023; Lara-Prieto et al., 2023). Consequently, engineering educators are challenged with the task of redefining instructional activities to align with the availability of digital tools and the evolving interests and proficiencies of the next generation of engineering students (Feisel and Rosa, 2005). On the other hand, the recent COVID-19 pandemic has also underscored the significance of implementing new pedagogical strategies that enable students to cultivate practical skills even in the absence of face-to-face training (Malhotra et al., 2020; OECD, 2021; Okoye et al., 2021; World Bank, 2020). This crisis has highlighted the necessity of alternative approaches to learning that provide the students with hands-on experiences for development of hard skills. Some researchers have already demonstrated that achieving favorable learning outcomes is possible through remote practical activities (Okoye et al., 2021; Ray and Srivastava, 2020).
Along these lines, in this study, we present the development, implementation, and assessment of a teaching methodology aimed at fostering the autonomous acquisition of hard skills and promotion of motivation among undergraduate engineering students. By recognizing the effectiveness of instructional videos in training engineering and medical students, the study focused on incorporating the Computer-Based Video Training and Tools into the studied educational system and innovation. This was done to help optimize the outcome of the learning process of the students. The outcome of the study contributes to the design of new strategies that enables efficient training of engineering students, optimizing both time and space utilization. Simultaneously, we aimed to find a solution that reduces the excessive workload on instructors when dealing with a large number of students, while also allowing students to develop hard skills at their own pace. In addition, the study provides a strategy that can be easily adapted to remote learning environments when in-person training is not feasible. Lastly, the study proved to improve the students’ motivation when they are tasked with completing complex tasks or learning challenging concepts within short timeframes.
The research questions of this study are as follows:
• Does computer-based video training and tools improve the learning outcomes and motivation of undergraduate engineering students?
• What are the implications of using the digitalized teaching method as blended alternative approach in facilitating the learning process of the students, and its implication for sustainable educational practice?
2 Literature review
Various educational research works have been conducted toward the development of strategies that leverage digital tools to overcome the learning drawbacks identified in this study, and to generate effective approaches for remote learning. These strategies aim to enhance the learning experiences of the undergraduate students in training scenarios, regardless of whether they are pursuing in-person or remote education.
Video-based training has been effective in overcoming challenges posed by limited instructor availability and has shown positive results in various studies. For example, Kumins et al. (2021) developed a video-based course for preclinical medical students, teaching knot-tying and suturing skills. The course proved effective in improving surgical familiarity, knowledge, and proficiency among novice medical students. Stefanova (2014) investigated the use of video training as a supplement for undergraduate telecommunications students, enhancing their performance in operating specialized devices. Narayan et al. (2022) compared video-based training on controlling hemorrhage with traditional in-person training for first-year medical students. The video-based training successfully achieved the objectives and instilled confidence in performing critical tasks. Bravo et al. (2011) studied the impact of low-cost educational videos on undergraduate engineering students, resulting in increased motivation and satisfaction. Videos offered advantages such as cost and time efficiency, improved comprehension of complex concepts, an engaging classroom environment, reduced reliance on face-to-face tutoring, enhanced autonomous learning, and encouraged collaborative discussions. De la Flor-López et al. (2016) developed tools, including video summaries and self-assessment activities, to enhance autonomous learning in practical sessions for mechanical engineering students. These tools led to significant improvements in their grades, reduced dropout rates, increased pass rates, and higher student satisfaction.
The development of kits that can be shipped and assembled by students without access to formal labs is another training alternative that have been explored in the scientific research. Tran et al. (2022) propose a take-home control engineering laboratory kit, leading to superior learning outcomes and increased final exam scores. Sotelo et al. (2022) developed an affordable kit for constructing a real-time data recording system, resulting in confident understanding and engagement. Reck and Sreenivas (2016) designed a modular, portable kit replicating a traditional control lab, achieving the same learning objectives as traditional labs. Positive feedback was received for both modalities. Rossiter et al. (2019) presented take-home kits for teaching embedded systems, reporting high student satisfaction, cost savings, and efficient resource utilization. Accessible software and support resources were emphasized for enhanced learning experiences.
Remote labs have also emerged as an alternative to provide access to formal laboratories for students who may not have physical access to them (Hyder et al., 2009; Debacq et al., 2021; Fabregas et al., 2011; Monzo et al., 2021; Reid et al., 2022). However, this type of experience lacks the hands-on component, which is crucial for the comprehensive development of engineering students (Rossiter et al., 2019) which forms part of the methodology and teaching approach described in this present study.
3 Methodology
3.1 Course description and research framework
The study employed the participatory research design (Spinuzzi, 2005; Vaughn and Jacquez, 2020), a qualitative research methodology that involves the collection, analyzing, and interpreting of data about the students’ learning outcome and assessment. The methodology of the study is grounded on the Descriptive Decision theory (Baucells and Katsikopoulos, 2011; Chandler, 2017) and Learning-Oriented Assessment (LOA) framework (Çakmak et al., 2023; Davidson and Coombe, 2022) which are theoretical framework and type of students’ learning outcome (SLO) model that studies the rationality behind the decisions that the users are disposed to make, as well as the level of outcome of the students’ learning process or performance. The study was implemented through a 20-h short course named “Engineering in Action” at two campuses of Tecnologico de Monterrey: Campus Toluca and Campus Puebla. Each course had an average enrollment of 25 students, and a total of four courses were conducted between February and December 2022. We designed an affordable kit that students can assemble with the assistance of the instructional videos. The resources were provided to the undergraduate students in their fourth to fifth semester from diverse engineering disciplines, including Industrial Engineering, Mechanical Engineering, and Mechatronics Engineering. The students had the option to develop the activity in the laboratory with the guidance of the professor, if needed, or to take the kit with them and complete the activity at their own pace.
Table 1 provides a brief overview and descriptive statistics of the distribution of the students, and the analyzed data to include the gender, degree, and enrollment status of the students in each course. The primary objective of this course is to introduce students to fundamental concepts of engineering design and enhance their skills in reading and interpreting engineering standards. Additionally, the course emphasizes the practical application of acquired knowledge in real-world scenarios, such as the fabrication of a force transductor, the experimental evaluation of the safety factor in a portable jib crane or the experimental estimation of stress in complex components like vehicle chassis or suspension arms.
The research study followed a structured course format, commencing with a conference-style lecture on the first day. The lecture covers essential topics for the upcoming activities, such as stress evaluation in simple cross-section beams and the working principle of Wheatstone bridges. On the second day, students delve into the ASTM E1237 Standard Guide for Installing Bonding Resistance Strain Gages, focusing on critical aspects of strain gauge installation and result reporting. Following this, students receive a kit and instructional videos to undertake two activities. The first activity involves assembling a Wheatstone bridge circuit capable of measuring the resistance change of a strain gauge, while the second activity entails preparing and installing a strain gauge on an aluminum strip, following the steps outlined in the instructional videos and in the previously reviewed engineering standard. The third day is dedicated to completing the tasks demonstrated in the instructional videos, allowing flexibility in the order of task development. However, both activities must be accomplished to receive evaluation. On the fourth day, student work is examined, and a practical examination is conducted to assess the successful completion of the activities. Finally, on the last day of the course, students are encouraged to apply their acquired knowledge and skills to solve engineering problems, such as constructing a force transducer or experimentally evaluating stress in a mechanical system.
3.2 Tool: portable kits design
When designing the portable kit for the study, the following measurement factors and instructional/scientific impact were considered:
• Educational goals: The kit should enable students to apply engineering materials and techniques effectively, addressing engineering problems while adhering to relevant standards.
• Component availability and cost-effectiveness: Consideration should be given to selecting components that are readily accessible and affordable, making them easy to obtain for the institution.
• Simplified assembly of electronic components: While the installation of a strain gauge may present some complexity, the remaining components should be easy and straightforward to assemble.
• Utilization of open-source software: To facilitate strain measurement, the kit should be compatible with free, safe, and easy-to-install software.
• Portability: The kit should be designed with portability in mind, ensuring that all components can be conveniently stored within a compact box measuring a maximum of 25 cm × 25 cm × 25 cm.
By considering the instructional factors, the portable kit was tailored to meet the educational objectives of the course while ensuring ease of use, affordability, and portability. The portable kit consisted of two modules: one for the assembly of a Wheatstone bridge and another for the installation of a strain gauge. Table 2 provides a detailed list of the materials included in each module of the kit. The total cost of each kit was approximately $75 USD. Figure 1 shows a representative diagram of the circuit that the students were required to assemble using the materials provided in the Wheatstone bridge module. In addition, the students were tasked with installing the strain gauge included in the installation gauge module on the aluminum strip, following the guidelines outlined in the previously reviewed ASTM E1237 Standard. A visual representation of the designed portable kit, as distributed to the students, is presented in Figure 2.
3.3 Digital intervention: instructional videos
Supplementary instructional videos were provided to assist students in understanding the assembly process for both modules of the kits. The videos aim to illustrate the step-by-step procedures involved in assembling the electronic circuit to create a Wheatstone bridge and installing a strain gauge in an aluminum strip according to engineering standards. The videos feature the instructor manipulating tools and materials while explaining the actions. Both videos were planned with the help of a simple script to guide the instructor, ensuring no important explanations were omitted. The content is designed to support the development of a relatively complex technical task for an inexperienced student. Students can replay the explanations as needed, and the materials in the video match those included in the kits. To minimize direct and indirect costs, in terms of resources and time for creating the instructional videos and to minimize the budget for implementing this educational proposal, a “low-cost educational video approach” was adopted. This approach has been proven to be an effective educational resource or practice (Simo et al., 2010). A low-cost educational video is a concise demonstration video that serves a specific purpose and can be produced in a short period of time using minimal resources. It can be easily integrated or embedded into other course materials. Such videos allow lecturers to address common video-related challenges by reducing the need for extensive resources, simplifying the video upgrading process, and seamlessly incorporating the video into the course based on the lecturer’s preferences (Bravo et al., 2011). A low-cost educational video does not require advanced video editing skills and can be created using freely available or commonly used video editing software, along with standard recording equipment such as a smartphone camera. Although for this study, the videos were meticulously crafted to ensure clear narration, satisfactory resolution, concise duration, and a well-structured explanation of the procedures, including an introduction to the materials used at the beginning of each video. The actions depicted in the videos were always clear and easy to replicate.
The learning management system of Tecnologico de Monterrey (Canvas) provided the students with access to the instructional videos. Figure 3 showcases a representative view of the content and upgrades offered by each professor through the same learning management system. Students were given access to these videos starting from the first day of the course. It is worth noting that instructors preferred uploading a series of videos instead of a single one to avoid the need for extensive editing.
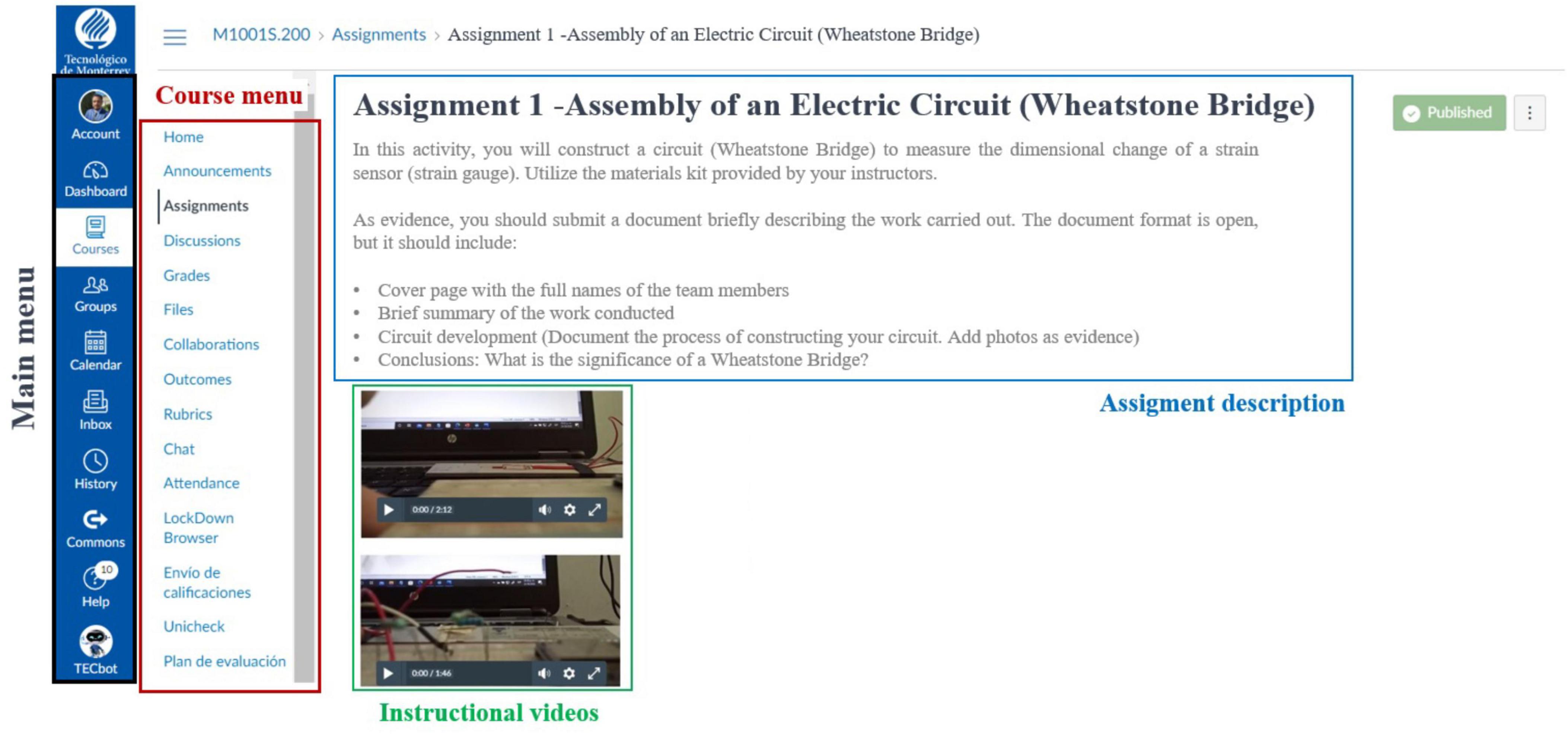
Figure 3. Insight into students’ perception of the instructional videos shared by the course instructors through CANVAS.
3.4 Learning outcomes evaluation
To assess the effectiveness of the combined use of portable kits and instructional videos in developing hard skills among enrolled students, a practical examination was conducted. From the second day of the course onward, students were equipped with portable kits and directed to review the instructional videos. They had the option to work on assigned activities in the laboratory, guided by videos and instructor support, or take the kit to a location of their choice and proceed at their own pace. The flexible location offered an open-ended completion time, requiring assembly of both modules by the following day. The time allocated for in-person laboratory activities remained consistent with the traditional four-hour format. Most students preferred conducting activities in the laboratory. During this time, each student selected which of the two portable kits to initiate. The instructor permitted students to progress independently, with some directing questions to the instructor. Notably, only one instructor could assist the entire group, thanks to detailed video explanations. Questions mainly aimed to confirm task progress or clarify the use of materials, explained in the videos but not yet seen by the students.
During the practical examination, students were required to demonstrate the functionality of the Wheatstone bridge they constructed by measuring the change in resistance of the installed strain gauge. This evaluation focused on two critical aspects: the operation of the Wheatstone bridge circuit and the correct installation of the strain gauge. To assess the results obtained by the students at the end of the third day, a Product Quality Score (PQS) similar to the one presented in the work developed by Kumins et al. (2021) was utilized. The PQS was rated on a scale of 0 to 5 as outlined in Table 3.
3.5 Motivation evaluation
In order to evaluate the motivation of the students, the Model of Academic Motivation Inventory (MUSIC Inventory) was employed. This inventory is a validated questionnaire that can be freely used by instructors and researchers to assess the motivational climate of a course or activity. It measures the perception of the students based on the five components of the MUSIC model: Empowerment/Autonomy, Usefulness, Success, Interest, and Caring (Brett, 2009). A brief description of each component is shown in Table 4. The Spanish version of the questionnaire, which is available in the “User Guide for Assessing the Components of the MUSIC Model of Motivation” (Brett, 2023), was utilized for this evaluation. The questionnaire consists of 26 items and the students were required to indicate their level of agreement using a 6 point ranked Likert scale measurement, where 1 being Strongly Disagree and 6 being Strongly Agree, respectively. The empowerment/autonomy and usefulness components was assessed using five items each, while interest and caring are evaluated using six items. The success component was evaluated with four items. On the last day of class, the questionnaire was distributed to the students using a Google Drive form. While all students were invited to participate, it should be noted that completing the questionnaire was not obligatory and did not factor into their final grade.
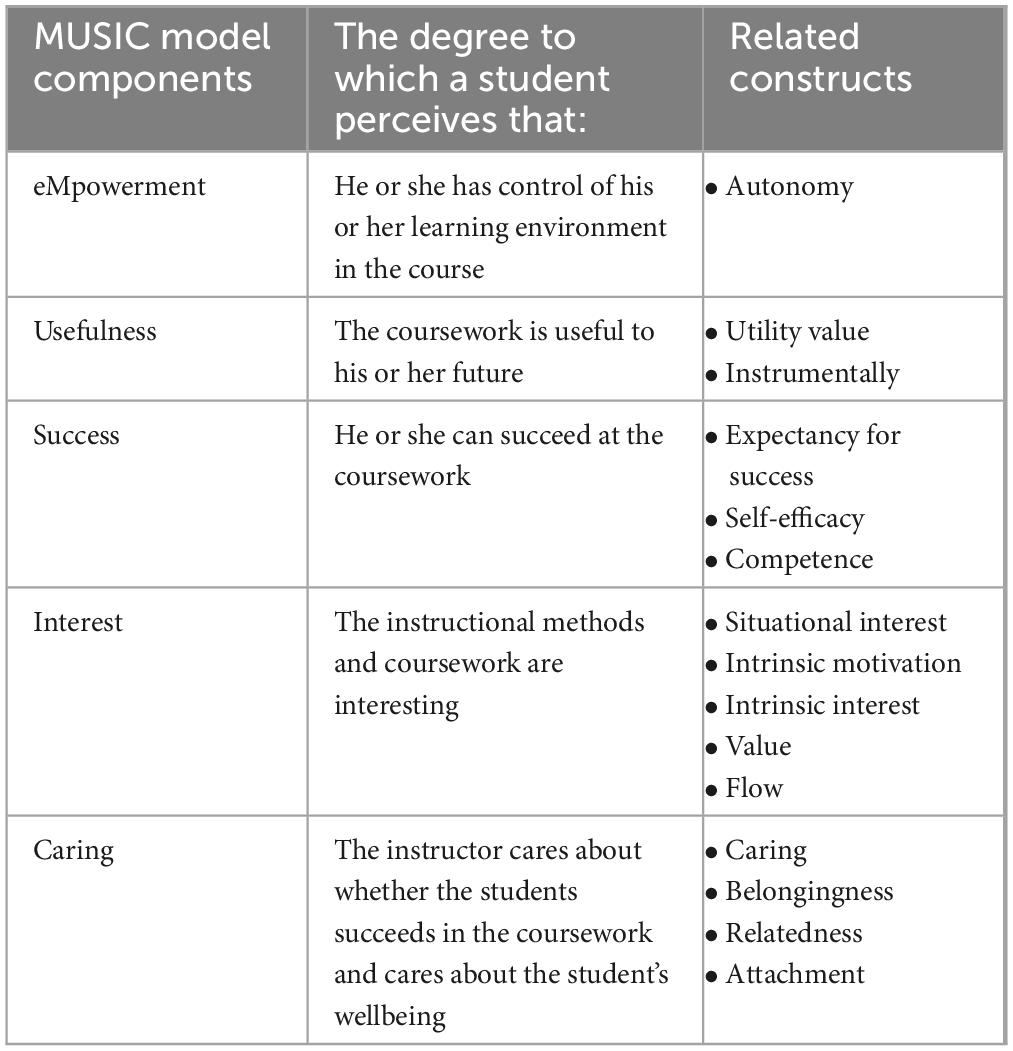
Table 4. Definition of the MUSIC model components and related constructs (Brett and Skaggs, 2016).
4 Results and outcomes
4.1 Learning outcomes
The practical examination and results for the four courses conducted at the two campuses of the host institution between February and December 2022 are presented in Table 5, and visually represented in Figure 4. Figures 5, 6 offer graphical depictions that illustrate the levels of achievement of some students in assembling the Wheatstone bridge circuit module and installing the strain gauge, respectively. The installation process of the strain gauge adhered to the guidelines outlined in the ASTM E1237 Standard, and the development of both modules was facilitated by instructional videos. During the validation phase, the accurate functioning of the strain gauge was confirmed through visual analysis and the utilization of a standard commercially available Wheatstone bridge, as depicted in Figure 6. Similarly, the instructors assessed the accuracy of the assembly of the Wheatstone bridge circuit developed by the students by measuring the signal from the installed strain gauge, as demonstrated in Figure 7. This figure provides insight into the measurement of the electrical resistance of the strain gauge when the aluminum plate underwent loading, serving as a validation technique to evaluate the proficiency of the students in assembling both modules.
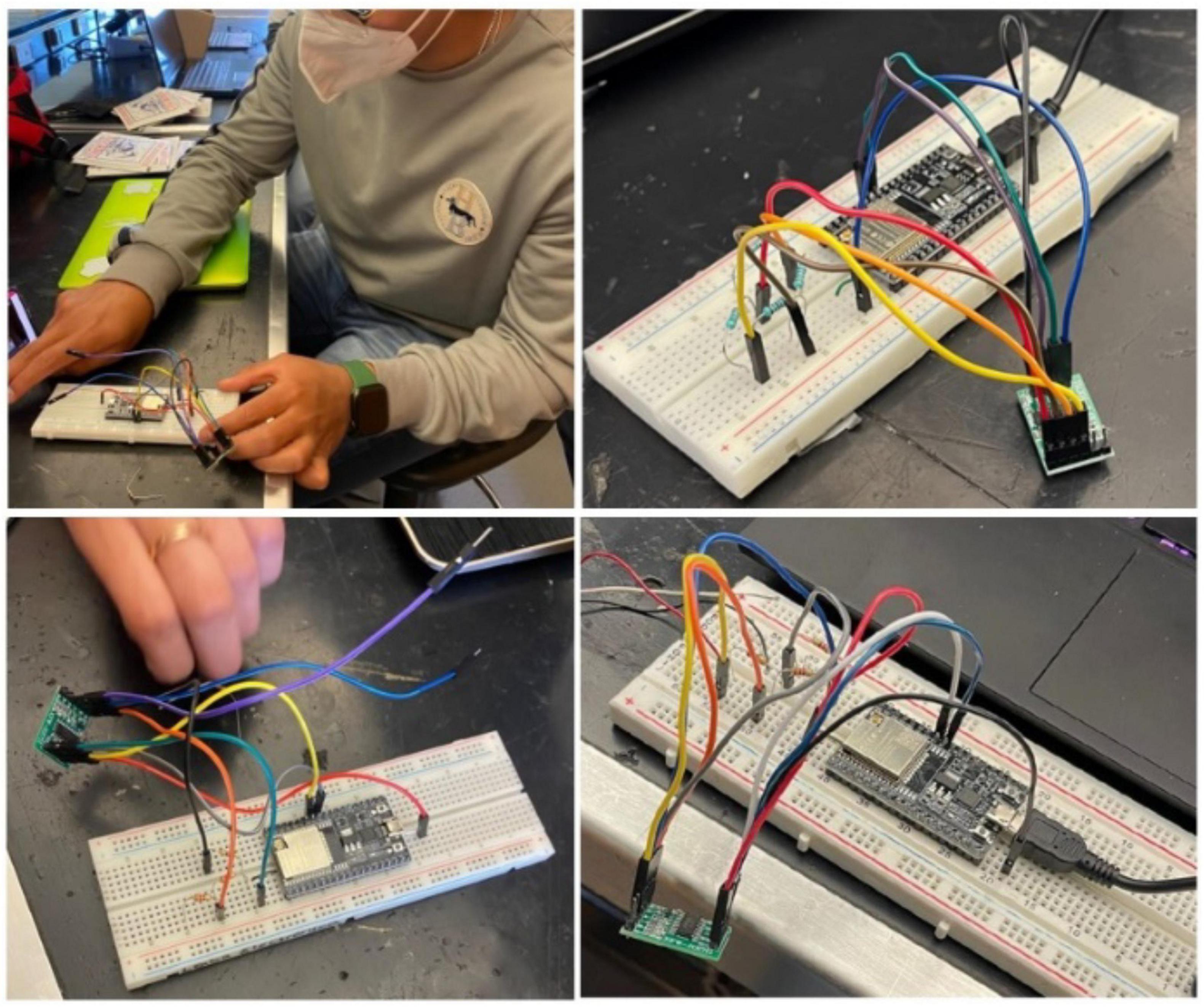
Figure 5. Visual representation showcasing the outcomes attained by some students in the assembly of the Wheatstone bridge circuit module.
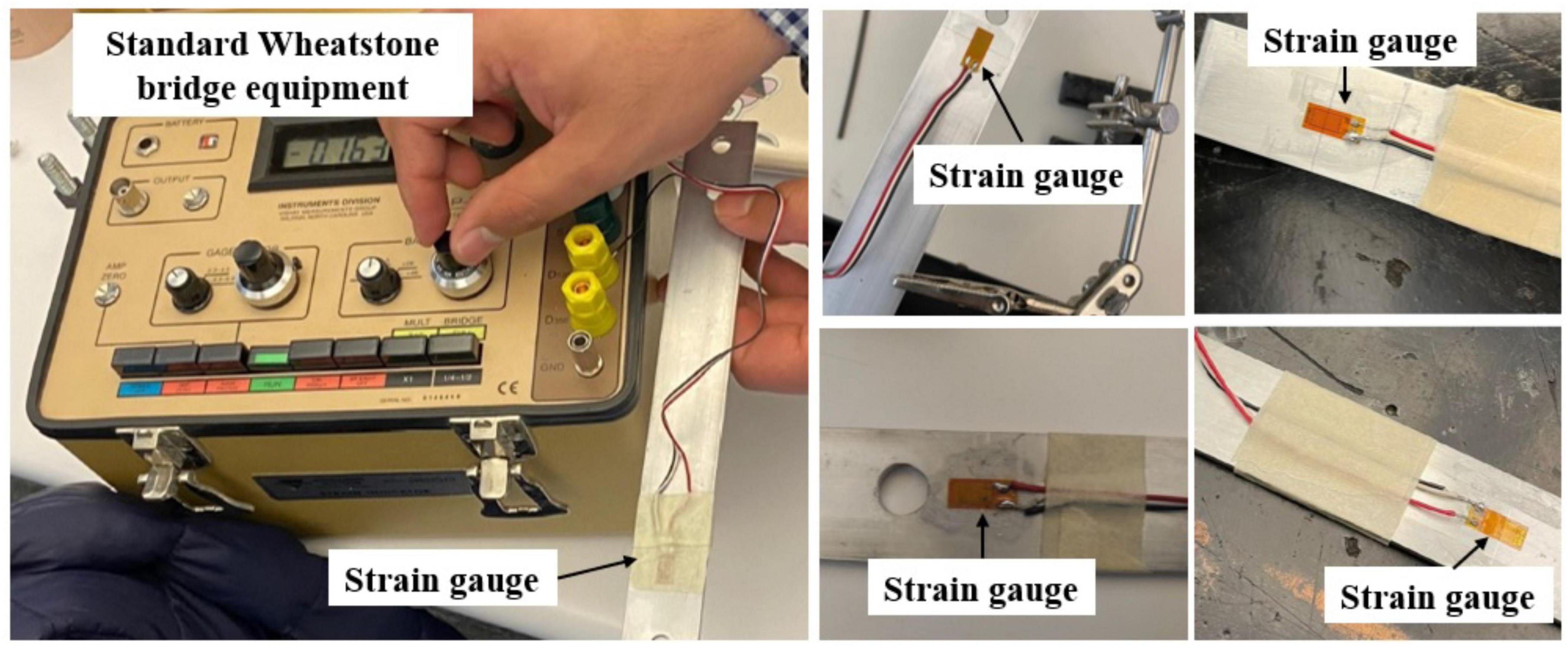
Figure 6. Visual representation of the practical evaluation of the installation of a strain gauge using a standard Wheatstone bridge equipment.
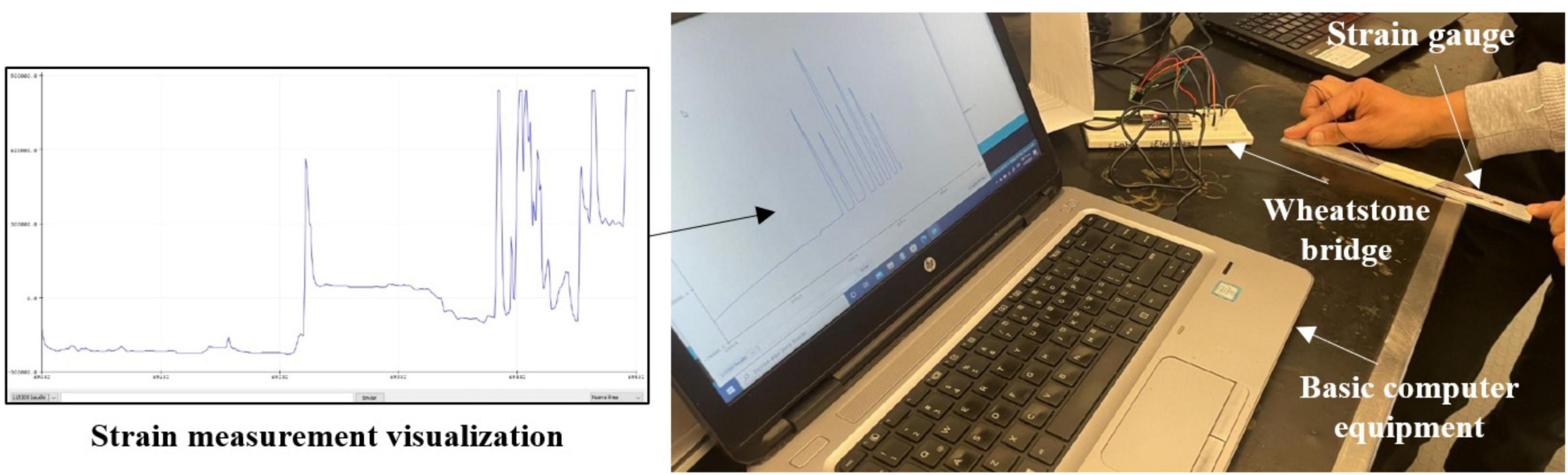
Figure 7. Visual depiction of the validation process conducted to verify the operational functionality of the electronic circuit.
In the first period, out of the 84 evaluated students, 16 students (19% of the evaluated students) required additional assistance to complete the tasks and achieved scores of 1 (below average) or 2 (average) when their individual work was evaluated. Most students (51.2%) were able to complete the tasks independently and achieved scores of 4 (very good) or 5 (excellent).
The combined use of instructional videos and lab kits was a novel approach for the professors involved, especially in determining and answering questions about uncertainty of the students performance. The initial implementation allowed instructors to gain experience regarding potential mistakes students might make in assembling the kits and how students should appropriately use the instructional videos. For the second application of this approach, the instructor provided recommendations regarding the use of videos and kits. Students were advised to watch the complete video before starting the assembly, as many in-person lab questions from students were related to the use of materials explained later in the video that students had not watched yet. This approach reduced the number of questions related to information already covered in the videos and made students more aware of the entire task they had to accomplish. Additionally, the instructor paid more attention to the theoretical content covered in the first four-hour lesson that required special emphasis to help students understand the application of the task they were working on. This approach allowed students to contextualize the practical task, enhancing their understanding of why they needed to place the strain gauge in a specific direction or choose the best location for their strain gauge. Even though these directions were indicated in the videos, better results were achieved when students were self-aware of the reasons behind their tasks. Certainly, in the second period course conducted from August to December, all the evaluated students (19) successfully completed the practical tasks without the need for instructor assistance, achieving scores ranging from 3 to 5 for their work. The authors believe that this improvement can be attributed to the experience of the instructor with this approach.
During the first period, students achieved an average score ranging from 3.38 to 3.52, placing their performance between 3 (Good) and 4 (Very good). This outcome implies the effectiveness of the educational approach employed in the course, as completing the assigned tasks necessitated the application of hard skills. Moreover, the experience of the instructor with these tools highlighted the potential for further enhancements, given that students attained an average score above 4 (4.12) during the second period course. This suggests that students not only completed the tasks but also demonstrated proficiency in executing procedures and utilizing the tools.
4.2 Motivation
Table 6 and Figure 8 show the results obtained from the Model of Academic Motivation Inventory (MUSIC) methodology. A voluntary participation of 88% was achieved from the four conducted courses. Across all courses, a consistent trend of scores reaching up to 5 was observed in all five components of the MUSIC model.
The Empowerment component of the MUSIC methodology assesses the perception of the students about their level of control over their learning process during the course. It is somewhat linked to the self-determination theory (Deci and Ryan, 1991). According to this theory, individuals who feel they have some measure of control over their learning are more likely to experience enjoyment when carrying out prescribed activities. In the applied test, the Empowerment component yielded scores ranging from 5.2 to 5.5, suggesting that the students generally agreed that they exercised at least some degree of control over their learning environment.
Within the framework of the MUSIC methodology, the Usefulness component assesses the perception of students regarding the practical applicability of the knowledge and skills acquired during the course. This score is more closely associated with the effectiveness of the instructional activities designed by instructors than with the instructional format itself. Nevertheless, this component also yielded favorable results, with values falling within the 5.1 to 5.6 range.
The success component of the MUSIC methodology is closely tied to the concept of self-perceived competence. It is widely acknowledged that human beings possess an innate desire for competence, striving for proficiency in their endeavors (Elliot and Dweck, 2005). Success holds paramount importance for students, and those who believe they can succeed in an activity are more likely to exhibit higher motivation compared to those anticipating failure when undertaking a task (Brett, 2009). The outcomes of the applied test demonstrate that students perceived a sense of accomplishment while completing the assigned tasks of the course using the blended learning approach proposed in this study. This is supported by scores ranging from 5.2 to 5.6 in this component, even when the quantity and complexity of the practical task are relatively high.
Perhaps, the most straightforward component to comprehend within the MUSIC methodology is Interest. This component is closely related to the instructor’s skill in developing activities that capture the attention of the students. Given that each individual possesses varying levels of interest in diverse subjects, maintaining engagement in a particular activity is a challenging endeavor. Nonetheless, it is crucial to recognize the impact of the learning environment on interests of students. In this study, students scored within the range of 5.4 to 5.6 for this component, suggesting that they found the designed activities and learning format engaging to participate in.
Lastly, the Caring component of the MUSIC methodology is linked to the perception of the students in the level of care and concern displayed by their instructors toward their learning. Consistent research indicated that motivation is positively correlated with the presence of caring relationships between instructors and students (Brett, 2009). Initially, the authors were concerned about the potential for students to feel neglected if a more impersonal approach was introduced through instructional videos and use of the portable kits. However, notably high scores ranging from approximately 5.6 to 5.8 in the Caring component indicates that the students perceived strong support and guidance throughout the exercises. The authors attributed the high scores obtained in this component to the continuous guidance provided by the instructors to the students, despite instructional videos serving as the primary means of support for performing the prescribed activities.
These results serve as evidence that not only does the educational approach implemented in this study proved valuable in nurturing the practical engineering skills of the students, but also proved effective in sustaining high levels of student motivation.
5 Discussion
Engineers heavily rely on specialized instruments and analytical techniques for their daily tasks. Therefore, it is crucial for students to gain proficiency in using those tools, e.g., through laboratory exercises, during their professional studies. Traditional laboratory practices in engineering education face several challenges, such as limited lab space, available instructors, and equipment, especially when accommodating a large number of students for in-person sessions. Consequently, there is significant value in enabling engineering students to develop fundamental technical skills using resources that offer flexibility in terms of training schedules and locations, even beyond the confines of traditional laboratories.
Numerous studies have underscored the effectiveness of incorporating low-cost instructional videos as educational resource in engineering and medical education for undergraduate students (Kumins et al., 2021; Stefanova, 2014; Narayan et al., 2022; Bravo et al., 2011; De la Flor-López et al., 2016). Portable kits have also been researched and evaluated in various research investigations, consistently yielding positive results as an educational tool that can be used to enhance the development of practical (hands-on) skills for engineering students (Tran et al., 2022; Sotelo et al., 2022; Reck and Sreenivas, 2016; Rossiter et al., 2019). However, some researchers have highlighted certain disadvantages associated with such approaches. For instance, the use of instructional videos outside the laboratory may lack immediate feedback from instructors, potentially limiting the students’ engagement and active participation in the learning process (Stefanova, 2014; Narayan et al., 2022). On the other hand, while portable kits incorporate hands-on experiences and offer scheduling and spatial flexibility within the learning process, unclear instructions may still necessitate substantial instructor support for assembling components, underscoring the challenge posed by limited instructor availability for lab practices.
In these perspectives, the objective of this study was to validate a blended approach that leverages the advantages of instructional videos and lab kits while addressing the documented drawbacks of this type of implementation by integrating immediate feedback from an instructor. This approach proved to aid the instructor in supporting the work of an entire group of students by minimizing the need for extensive explanations and student questions. Additionally, it allows students to proceed with the tasks at their own pace, independent of a single instructor’s explanation. It’s important to note that this approach does not aim to overhaul the common practice of learning hard skills in laboratories but rather intends to incorporate additional tools to enhance the efficiency of the student learning process in the lab.
Moreover, it is essential to emphasize the consistent availability of instructors in the lab to assist students with their queries during the proposed learning approach in this study. The authors firmly believe that an optimal approach to learning, especially in the engineering education, must involve a combination of traditional in-person guidance, instructional videos, and portable kits. This approach not only reduces the individualized time dedicated to each student but also minimizes the frequency of demonstrations and the reliance on specialized equipment, leading to remarkable outcomes.
Based on the results obtained in the learning outcomes/intervention and the motivation evaluations in this study, it can be said that the proposed educational approach proved to facilitate the development of practical skills in engineering students, including those with limited or no experience working with electronic items, instruments, or strain gauge measurements. The total cost of the proposed kit was only $75 US, making it an affordable investment in undergraduate students’ education, considering that most of the components can be reused semester after semester. Furthermore, motivation levels remained consistently high among all the groups that participated in the proposed learning approach, even when instructed by individuals with varying profiles or personalities. Indeed, the outcome of the study aligns with the findings of similar research works that also endorse instructional videos as valuable educational resources (Bravo et al., 2011; De la Flor-López et al., 2016).
To summarize the discussion, we note by the end of the course and the learning intervention that the students exhibited confidence in their ability to perform the specific practical skills outlined in this study (see Section “3 Methodology”). Consequently, the learning approach has the potential to be applied to numerous other engineering courses, assisting engineering students in the development of practical skills, provided that the laboratory practices can be adapted to incorporate the creation of portable kits and cost-effective instructional videos.
5.1 Implications of this study
In our literature review and discussion sections (see Sections “2 Literature review” and “5 Discussion”), we explained how the use of instructional videos and lab kits has proven to be useful. However, each approach has its drawbacks, including the lack of immediate feedback from instructors. It is important to mention that our approach does not aim to eliminate the support of instructors in the learning process but rather seeks to complement it by relying on instructional videos and lab kits in conjunction with personal feedback. The goal is to contribute to the development of tools that enable support for in-person lab learning while overcoming obstacles related to space and human resources.
For example, in this exercise, access to a lab was possible, but it was not necessary to have one to carry out the activity. Any space with sufficient tables and electrical outlets would suffice for task development, eliminating the need for specialized spaces, at least for some tasks. The use of instructional videos benefited both students and instructors. Students could be aware in advance of the tasks they had to develop, while instructors reduced (though did not eliminate) the amount of explanations they had to provide, making efficient use of available time and human resources.
Moreover, in emergencies such as the recent global COVID-19 pandemic in which the students are compelled to learn remotely, we note that this implementation can be more easily adapted to remote learning situations where in-person feedback is not possible. Although the authors are conscious that more work is needed to prove its effectiveness for a remote learning approach, we believe it is important to share our findings on the use of such type of blended approach as an alternative to the current traditional instructional lab paradigm.
5.2 Limitations and future directions of the study
The developed educational tools in this study can be readily adapted to remote learning environments, as demonstrated by the small percentage of students (less than 5%) who chose to complete the tasks at home and achieved scores between 3 and 4. However, due to the limited sample size, definitive conclusions may not be drawn especially considering the other settings or institutions in which this type of study can be replicated or performed. Therefore, necessitating further research for clarity on this topic.
Another limitation of this work lies in the absence of a comparison between the outcomes obtained with the proposed alternative learning approach and the outcomes achieved with a traditional approach. The authors note that while the design and testing of the alternative approach and methodology in this study proved to lead to successful learning outcomes, contribute significantly to the advancement of engineering education and instructional laboratories. It is noteworthy to mention that a direct comparison of the learning outcomes of the traditional engineering lab instructions in the same learning scenario could not be done at the time of this study or settings. However, it is also important to point out that the same in-person lab sessions developed in this study can be replicated in any space that has enough space to allow students to work and with enough electrical inlets, making it easier to condition a simple space for use as a lab and eliminating the obstacle of a lack of specialized spaces for the development of lab practical sessions, at least for some tasks.
6 Conclusion
This study introduced a blended methodology to help undergraduate engineering students develop hard skills. Based on the participatory design approach grounded on Descriptive Decision theory and Learning-Oriented Assessment (LOA) framework, an affordable kit was designed for a short course, resulting in low-cost instructional videos that are created to guide students in using the kits.
Results from the implemented teaching approach and practical exams showed the effectiveness of the learning approach in developing hard skills in engineering students. In the first period, students across various courses achieved an average score ranging from 3.38 to 3.52 (on a scale of 0 to 5) in the practical examination. Only 19% of students required assistance and scored 1 or 2. In the second period, students achieved an average score exceeding 4 (4.12), showcasing proficiency in tasks, procedures, and tool usage. No students needed significant support or scored below 3 in the practical examination during this period. This improvement can be attributed to instructor experience and better preparation, highlighting the potential for further enhancement of the educational approach with instructors’ expertise.
The Model of Academic Motivation Inventory (MUSIC) methodology consistently revealed high motivation levels across all courses, with scores reaching up to 5 in all five components of the MUSIC model. Notably, the caring component of the MUSIC methodology, which measures the perception of the students regarding the concern of their instructor in their learning process, received the highest evaluation. This is significant, especially considering that the time dedicated to each student was lower compared to a traditional in-person lab practice. The approach has the potential to be applied in various engineering courses to help students develop practical skills cost-effectively.
Data availability statement
The raw data supporting the conclusions of this article will be made available by the authors, without undue reservation.
Author contributions
CR-C: Writing – original draft, Project administration, Methodology, Investigation, Funding acquisition, Formal analysis, Conceptualization. LF-C: Writing – review & editing, Project administration, Methodology, Funding acquisition, Formal analysis, Conceptualization. IC-R: Writing – review & editing, Project administration, Methodology, Funding acquisition, Formal analysis, Conceptualization. PN-G: Writing – review & editing, Investigation, Funding acquisition. KO: Writing – review & editing, Supervision.
Funding
The authors declare financial support was received for the research, authorship, and/or publication of this article. This research work and project was supported by NOVUS, a grant initiative of Tecnologico de Monterrey, under Grant no. NOVUS (N21-210).
Acknowledgments
We would like to acknowledge the technical and financial support provided by the Writing Lab, Institute for Future of Education, Tecnologico de Monterrey in the publication of this work.
Conflict of interest
The authors declare that the research was conducted in the absence of any commercial or financial relationships that could be construed as a potential conflict of interest.
Publisher’s note
All claims expressed in this article are solely those of the authors and do not necessarily represent those of their affiliated organizations, or those of the publisher, the editors and the reviewers. Any product that may be evaluated in this article, or claim that may be made by its manufacturer, is not guaranteed or endorsed by the publisher.
References
Baucells, M., and Katsikopoulos, K. V. (2011). Descriptive models of decision making: Wiley encyclopedia of operations research and management science. Hoboken, NJ: John Wiley & Sons, Inc, doi: 10.1002/9780470400531.eorms0249
Bravo, E., Amante, B., Simo, P., Enache, M., and Fernandez, V. (2011). “Video as a new teaching tool to increase student motivation,” in Proceedings of the 2011 IEEE global engineering education conference (EDUCON), (Amman), 638–642. doi: 10.1109/EDUCON.2011.5773205
Brett, J. (2009). Motivating students to engage in learning: The MUSIC model of academic motivation. Int. J. Teach. Learn. High. Educ. 21, 272–285.
Brett, J. (2023). The music model of motivation, questionnaires. Available online at: https://www.themusicmodel.com/questionnaires (accessed August 08, 2023).
Brett, J., and Skaggs, G. (2016). Measuring students’ motivation: Validity evidence for the music model of academic motivation inventory. IJ SOTL 10:107. doi: 10.20429/ijsotl.2016.100107
Çakmak, F., Ismail, S. M., and Karami, S. (2023). Advancing learning-oriented assessment (LOA): Mapping the role of self-assessment, academic resilience, academic motivation in students’ test-taking skills, and test anxiety management in Telegram-assisted-language learning. Lang. Test. Asia 13:20. doi: 10.1186/s40468-023-00230-8
Chandler, J. (2017). Descriptive decision theory: Stanford encyclopedia of philosophy (Winter 20). Available online at: https://plato.stanford.edu/entries/decision-theory-descriptive/ (accessed May, 2023).
Davidson, P., and Coombe, C. (2022). “Practical applications of learning-oriented assessment (LOA),” in Local research and glocal perspectives in english language teaching, eds R. Khan, A. Bashir, B. L. Basu, and M. E. Uddin (Singapore.: Springer Nature), 329–344. doi: 10.1007/978-981-19-6458-9_21
De la Flor-López, S., Ferrando, F., and Fabregat-Sanjuan, A. (2016). Learning/training video clips: An efficient tool for improving learning outcomes in mechanical engineering. Int. J. Educ. Technol. High. Educ. 13:6. doi: 10.1186/s41239-016-0011-4
Debacq, M., Almeida, G., Lachin, K., Lameloise, M., Lee, J., Pagliaro, S., et al. (2021). Delivering remote food engineering labs in COVID-19 time. Educ. Chem. Eng. 34, 9–20. doi: 10.1016/j.ece.2020.10.002
Deci, E., and Ryan, R. (1991). “A motivational approach to self: Integration in personality,” in Nebraska symposium on motivation, ed. R. Dienstbier (Lincoln, NE: University of Nebraska Press), 237–288.
Dym, C. L., Agogino, A. M., Eris, O., Frey, D. D., and Leifer, L. J. (2005). Engineering design thinking, teaching, and learning. J. Eng. Educ. 94, 103–120. doi: 10.1002/j.2168-9830.2005.tb00832.x
Elliot, A., and Dweck, C. (2005). “Competence and motivation,” in Handbook of competence and motivation, eds A. Elliot and C. Dweck (New York, NY: Guilford), 3–12.
Fabregas, E., Farias, G., Dormido-Canto, S., Dormido, S., and Esquembre, F. (2011). Developing a remote laboratory for engineering education. Comput. Educ. 57, 1686–1697. doi: 10.1016/j.compedu.2011.02.015
Feisel, L. D., and Rosa, A. J. (2005). The role of the laboratory in undergraduate engineering education. J. Eng. Educ. 94, 121–130. doi: 10.1002/j.2168-9830.2005.tb00833.x
Feisel, L., and Peterson, G. (2002). “A colloquy on learning objectives for engineering education laboratories,” in Proceedings of the 2002 annual conference, (Berlin), doi: 10.18260/1-2-11246
Hyder, A., Thames, J., and Schaefer, D. (2009). “Enhancing mechanical engineering distance education through IT-enabled remote laboratories,” in Proceedings of the ASME 2009 international design engineering technical conferences and computers and information in engineering conference, (San Diego, CA), 491–500. doi: 10.1115/DETC2009-87456
IEEE (2020). COVID-19 made internet access, broadband and hotspots an educational necessity. IEEE transmitter. New York, NY: IEEE.
Jiang, C., and Pang, Y. (2023). Enhancing design thinking in engineering students with project-based learning. Comput. Appl. Eng. Educ. 31, 814–830. doi: 10.1002/cae.22608
Judge, M. (2017). Large-scale laboratory teaching for 1st year EEE undergraduates. Int. J. Electr. Eng. Educ. 54, 164–177.
Kumins, N., Qin, V., Driscoll, E., Morrow, K., Kashyap, V., Ning, A., et al. (2021). Computer-based video training is effective in teaching basic surgical skills to novices without faculty involvement using a self-directed, sequential and incremental program. Am. J. Surg. 221, 780–787. doi: 10.1016/j.amjsurg.2020.08.011
Lara-Prieto, V., Ruiz-Cantisani, M. I, Arrambide-Leal, E. J., de la Cruz-Hinojosa, J., Mojica, M., Rivas-Pimentel, J. R., et al. (2023). Challenge-based learning strategies using technological innovations in industrial, mechanical and mechatronics engineering programs. Int. J. Instruct. 16, 261–276. doi: 10.29333/iji.2023.16115a
Malhotra, S., Dutta, R., Amit Kumar, and Sagar Mahna, D. (2020). “Paradigm shift in engineering education during COVID 19: From chalkboards to talk boards,” in Proceedings of the 2020 12th international conference on computational intelligence and communication networks, CICN 2020, (Bhimtal), 287–293. doi: 10.1109/CICN49253.2020.9242617
Monzo, C., Cobo, G., Morán, J. A., Santamaría, E., and García-Solórzano, D. (2021). Remote laboratory for online engineering education: The RLAB-UOC-FPGA case study. Electronics 10:1072. doi: 10.3390/electronics10091072
Narayan, M., Chandramouli, M., De Angelis, P., Gupta, A., An, A., Dominguez, M., et al. (2022). Video-based stop the bleed training: A new era in education. J. Surg. Res. 280, 535–542. doi: 10.1016/j.jss.2022.07.014
Okoye, K., Rodriguez-Tort, J. A., Escamilla, J., and Hosseini, S. (2021). Technology-mediated teaching and learning process: A conceptual study of educators’ response amidst the Covid-19 pandemic. Educ. Inform. Technol. 26, 7225–7257. doi: 10.1007/s10639-021-10527-x
Ray, S., and Srivastava, S. (2020). Virtualization of science education: A lesson from the COVID-19 pandemic. J. Protein Proteomics 11, 77–80. doi: 10.1007/s42485-020-00038-7
Reck, R., and Sreenivas, R. (2016). Developing an affordable and portable control systems laboratory kit with a raspberry pi. Electronics 5:36. doi: 10.3390/electronics5030036
Reid, D., Burridge, J., Lowe, D., and Drysdale, T. (2022). Open-source remote laboratory experiments for controls engineering education. Int. J. Mech. Eng. Educ. 50, 828–848. doi: 10.1177/03064190221081451
Rossiter, J., Pope, S., Jones, B., and Hedengren, J. (2019). Evaluation and demonstration of take home laboratory kit. IFAC PapersOnline 52, 56–61. doi: 10.1016/j.ifacol.2019.08.124
Simo, P., Fernandez, V., Algaba, I., Salan, N., Enache, M., Albareda-Sambola, M., et al. (2010). Video stream and teaching channels: Quantitative analysis of the use of low-cost educational videos on the web. Proc. Soc. Behav. Sci. 2, 2937–2941. doi: 10.1016/j.sbspro.2010.03.444
Sotelo, D., Sotelo, C., Ramirez-Mendoza, R., López-Guajardo, E., Navarro-Duran, D., Niño-Juárez, E., et al. (2022). Lab-Tec@Home: A cost-effective kit for online control engineering education. Electronics 11:907. doi: 10.3390/electronics11060907
Stefanova, T. (2014). Using of training video films in the engineering education. Proc. Soc. Behav. Sci. 116, 1181–1186. doi: 10.1016/j.sbspro.2014.01.366
Tran, L., Radcliffe, P., and Wang, L. (2022). A low budget take-home control engineering laboratory for undergraduates. Int. J. Electr. Eng. Educ. 59, 158–175. doi: 10.1177/0020720919852784
UNESCO (2021). UNESCO strategy on technological innovation in education (2022–2025) - UNESCO digital library. Paris: UNESCO.
Vaughn, L. M., and Jacquez, F. (2020). Participatory research methods – choice points in the research process. J. Particip. Res. Methods 1:44. doi: 10.35844/001c.13244
Keywords: blended learning, digitized education, motivation, higher education, Tec21, professional education, educational innovation, portable kit
Citation: Resendiz-Calderón CD, Farfan-Cabrera LI, Cazares-Ramírez IO, Nájera-García P and Okoye K (2024) Assessing benefits of computer-based video training and tools on learning outcomes and motivation in mechanical engineering education: digitalized intervention and approach. Front. Educ. 9:1292405. doi: 10.3389/feduc.2024.1292405
Received: 11 September 2023; Accepted: 09 September 2024;
Published: 24 September 2024.
Edited by:
Clifford A. Shaffer, Virginia Tech, United StatesReviewed by:
Wahyu Widada, University of Bengkulu, IndonesiaRob Cassidy, Concordia University, Canada
Patrícia Albergaria Almeida, University of Aveiro, Portugal
Copyright © 2024 Resendiz-Calderón, Farfan-Cabrera, Cazares-Ramírez, Nájera-García and Okoye. This is an open-access article distributed under the terms of the Creative Commons Attribution License (CC BY). The use, distribution or reproduction in other forums is permitted, provided the original author(s) and the copyright owner(s) are credited and that the original publication in this journal is cited, in accordance with accepted academic practice. No use, distribution or reproduction is permitted which does not comply with these terms.
*Correspondence: Kingsley Okoye, a2luZ3NsZXkub2tveWVAdGVjLm14