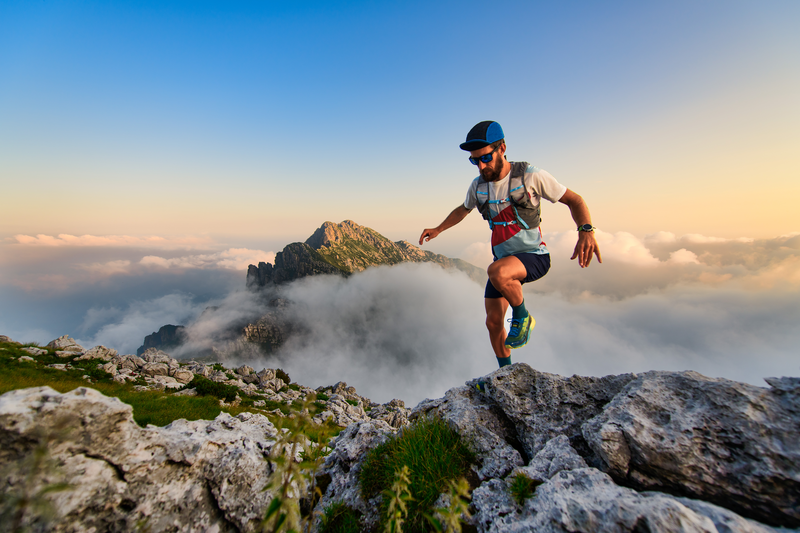
95% of researchers rate our articles as excellent or good
Learn more about the work of our research integrity team to safeguard the quality of each article we publish.
Find out more
CURRICULUM, INSTRUCTION, AND PEDAGOGY article
Front. Educ. , 13 February 2024
Sec. STEM Education
Volume 9 - 2024 | https://doi.org/10.3389/feduc.2024.1287521
This article is part of the Research Topic Invention Education and STEM: Perspectives and Possibilities View all 8 articles
Invention education is an emerging field that shows promise for fostering equitable student engagement, especially related to disciplines of science, technology, engineering, arts, and mathematics (STEAM), in both classroom and informal learning. A central concept for practitioners, researchers, and evaluators, student engagement connects with academic, socioemotional, career, and civic success. Nonetheless, more work is needed to ensure more equitable approaches to educational design for student engagement, especially with youth of one or more minoritized identity markers (e.g., Black, Brown, or Indigenous youth; female or non-binary youth; youth from lower socioeconomic statuses; etc.). This Curriculum, Instruction, and Pedagogy article describes six iterations of educational designs for invention education with grades 6–8 classes and camps. Three of the iterations revised the same curriculum for grade 7 classes (N ~ 160 students/year), and the other three iterations involved similar yet distinct curricula for grades 6–8 camps (N ~ 25 students/year). Taking a cultural psychology approach to design-based research, we conducted a phenomenological mixed-methods study for convergence. That is, we iteratively refined educational designs within given microcultures, and we sought to better understand participants’ lived experiences. We share evidence of high affective, behavioral, and cognitive engagement, within and between both individual and social levels, alongside development of self-efficacy with respect to ability beliefs and anxiety management. With a few exceptions, our findings suggest equitable participation of youth. These findings we connect with educational design considerations, including individual vs. social supports, explicit attention to youths’ hobbies and peer-inventors, and formative assessment that broadens response scales and gradations of challenge. Our work supports a more nuanced and socially-situated six-dimensional framework for student engagement, expanding upon commonly-used three-and four-dimensional models. We conclude with local and transferrable implications, towards the main goal of fostering equitable student engagement in science and engineering through invention education.
User-and activity-centered design, including invention education, can foster meaningful learning experiences in science, technology, engineering, arts, and mathematics (STEAM; National Science and Technology Council, 2018; Bevan et al., 2019; Invention Education Research Group, 2019). Such transdisciplinary, project-based curriculum can be powerful for both in-school-time and out-of-school-time contexts, especially through partnerships of local, regional, and national organizations (Bevan et al., 2010; Njoo et al., 2018; Fraser et al., 2021). It can be challenging to design curriculum and evaluate interventions across contexts for student outcomes related to interest, identity, academic performance, and career attainment; however, student engagement has shown potential in linking a variety of topics of interest to communities, policy makers, and practitioners (Bell et al., 2019; Sneider and Allen, 2019; Reschly and Christenson, 2022; Radil et al., 2023). In particular, more work is needed to adapt curricula from primarily out-of-school-time contexts, for in-school-time implementations, and to ensure equitable engagement across identity markers such as race and ethnicity, gender, socioeconomic class, linguistic repertoire, (dis)ability, and more (Cho et al., 2013; Fraser et al., 2021; Pérez-Salas et al., 2021; Galindo et al., 2022; Reschly and Christenson, 2022).
We share understandings from over 5 years in a community-practice-research partnership based in Mills City,1 a semi-urban city in the Northeast US. The partnership included members from Boston College, the Lemelson-MIT Program, and Mills City (including local businesses, professional organizations, and Mills City Public Schools). For in-school-time classes and out-of-school-time camps and clubs, we co-designed and co-created learning environments with middle-school youth, who engaged in user-and activity-centered design through invention projects. The classes and camps in particular have included youth of one or more populations historically marginalized from participation in STEAM. We hope that in sharing our understandings we will join a broader conversation about co-designing formal, informal, and hybrid environments—as well as connections and transitions between these models—an especially important conversation during times of increased remote and hybrid learning (Allen et al., 2020; Fraser et al., 2021). Though engagement may manifest in different ways over time and space, it remains a key predictor and outcome of academic, career, civic, and personal importance.
Our pedagogical approach leverages the flexibility of invention education to vary emphasis on disciplines of science, technology, engineering, arts, and mathematics (STEAM; Bevan et al., 2019). For example, our in-school-time interventions tend to prioritize per practitioner needs, while still providing opportunities for youth to leverage their interests in technology, engineering, arts, and mathematics, as described in the Learning Environments section below. On the other hand, our out-of-school-time interventions take a more pluridisciplinary approach (Hofstetter, 2012), wherein no discipline dominates the others. In these ways, invention education has provided a versatile framework that can promote in- and out-of-school-time learning, as well as synergies between them (Bevan et al., 2010).
Our specific approach to invention education includes principles of the Lemelson-MIT JV InvenTeams Program, which strives to “cultivate new ways of thinking and develop technical skills,” especially for grades 7–10 youth “with limited access to hands-on STEM enrichment activities” (Massachusetts Institute of Technology (MIT), 2016, p. P4). The “JV” stands for “Junior Varsity,” positioning the program as a stepping-stone to the “Varsity” program for youth in grades 9–12. Each of the curriculum units has a main project, as well as a follow-up activity for free-choice inventing. Within each unit, all sessions conclude with a self-assessment, in which youth reflect on their development of self-efficacy in science, user-and activity-centered design/engineering, and inventing. Overall, the program considers (technological) inventing to be the creation of a “useful and unique” contrivance, which can potentially be patented and produced in large quantities through entrepreneurship.
Each unit includes the Types of Team Members framework, developed by the Lemelson-MIT JV InvenTeams program. Youth self-identify with four roles: Doodler (of sketches, diagrams, etc.), Organizer (of materials, tasks, and persons); Talker (within and between teams, as well as with community members); and Tinkerer (with materials, supplies, prototypes, and final products). Table 1 has a summary of enacted curricula, including relevant pedagogical standards.
Mills City is situated on the traditional lands of the Massachusett and Pawtucket peoples. These first inhabitants were displaced by colonists first for farmland, then for mills during the US Industrial Revolution, and most recently by offices for businesses and housing for both immigrant and gentrifying populations. The present-day population is diverse in terms of race/ethnicity, gender, cultural and linguistic background, national origin, and socioeconomic status. Thus, Mills City in many ways is a microcosm of past and current life in what is currently called the US (See Table 2 for rounded racial/ethnic data; additional details are in Supplementary material).
Though our work centers on middle-school youth, it also includes high-schoolers as “counselors”; in-service educators as “teachers,” “co-advisors,” or “co-facilitators” (of classes, clubs, and camps, respectively); curriculum designers for creation and modification of curricula, along with some co-facilitation; university-based researchers as “participant-observers” who work at the intersection of research and practice; school-district leaders who ensure alignment with Mills City Public Schools’ district improvement plan; and local workers and residents who participate in all aspects of programming. This strong foundation in local communities connects with broader cultural and professional communities, as detailed below.
Our research-practice-community partnership began in November 2016, when members of the Innovation in Urban Science Education (IUSE) lab met members of the Lemelson-MIT Program (LMIT) at the Massachusetts STEM Summit. The following January we held a 5 h meeting on the Boston College campus, to explore possible synergies between existing and potential programs. Agendas were set by IUSE and LMIT leads, but were semi-structured to permit emergent discussions. The meeting included five, one-hour sub-meetings: (1) camp learning environments; (2) emergent multilingual learning; (3) evaluation; (4) standards alignment; and (5) grant writing. Ultimately we decided that a February Vacation Camp would be a good starting point for our work together, incorporating elements of all five 1 h sub-meetings.
After that first meeting, we met roughly for 1 h weekly during the academic years. Agendas were set by an IUSE lab member who also was an employee of the partner school-district. Further, we held half-week winter and summer professional development sessions with participating teachers, to collaboratively adapt the curriculum for their student populations. In particular, we considered adaptations to make the curriculum more accessible to emergent multilingual learners. This work included specialists in the five categories previously mentioned, including the After-school STEM Coordinator from Mills City’s two middle schools; an Associate Professor and a PhD student in language-learning; a PhD student in psychometrics; a PhD student in curriculum development; and a Professor and a Senior Research Scientist with grant writing expertise. Also, the IUSE lab manager was instrumental in facilitating logistics related to meetings, materials, scheduling, and tasks.
Mindful that this assemblage of personnel might be atypical, we note that high-school, undergraduate, and Master’s students have also made valuable contributions on similar projects. In other settings, department chairs, team leads, curriculum coordinators, or assistant superintendents might contribute more than was needed in our partnership. Finally, our connections with business partners have predominantly been formed through professional organizations, such as a nearby technology company consortium and a local chapter of the Society of Hispanic Professional Engineers.
In policy, the district improvement plan includes a specific priority for science, technology, engineering, and math (STEM) at the middle school level, as well as more general priorities of additional learning-time that can be partially realized through partnerships with external organizations. In practice, the grades 6–8 STEM camps tend to provide enrichment and extension, allowing students to go both broader and deeper into topics of interest to them.
The research team from Boston College prioritizes student (re-)engagement in STEM, particularly for youth from marginalized populations. The researchers include students spanning grades 13–20, a lab manager, a senior research scientist (post-doctoral), and a university Professor, all of whom work towards fostering youths’ confidence, interests, and identities, while developing transferrable skills of communication, critical thinking, and problem solving.
In describing the individual interventions, we proceed from out-of-school-time (OST) to in-school-time (IST), keeping chronological order in each.
The first camp was February 20–24, 2017, at Central Middle School in Mills City. Campers worked in teams of between two to four members, to make shoe sole prototypes in a process that involved researching, drawing, sculpting, molding, and casting. The camp culminated in a public Showcase of student work.
We expanded a 14 h curriculum2 towards the 26 h of camp activity-time, allowing additional opportunity for free-choice inventing and for videoconferences with invention professionals (An agenda for the camp and a list of supplies can be found in the Supplementary material). A total of 27 campers attended at least 1 day of camp, with an average of 23 campers per day (4.4 days per camper). Eleven campers were from races and ethnicities underrepresented in STEM, and nine self-identified as female.
Camp topics and activities included rapid prototyping (see Figures 1A,B); biomimicry (i.e., design inspired by organisms); principles of shoe-sole design; crafting clay models of shoe soles (see Figure 1D); creating rubber molds; casting from the rubber molds; activities about professional inventors and the role of empathy in invention; and planning-time for free-choice inventions. On the final day, campers presented their prototypes in a public Showcase. Throughout the week there were generally three or more adult facilitators and two or more university participant-observers present at any given time.
Figure 1. Representative photos of Designed Learning Environments. Required safety equipment included safety glasses, latex-free gloves, and close-toed shoes. (A) Rapid prototyping I: Stands for tablets and/or mobile phones; (B) Rapid prototyping II: “Problem Strips” for common situations; (C) Chill Out for small, insulating devices; (D) Shoe Soles for outsoles of shoes (clay models; pre-molding and -casting); (E) Noise Makers for electronic musical instruments; (F) U Control for electronic doors.
In the second camp, youth worked in teams of between three to four members, to make electronic musical instruments. The standard instrument design included a single electromagnet that converted mechanical vibration of strings into electrical signals (i.e., a single electrical “pickup”). However, some campers chose not to incorporate a pickup into their final design, while other campers made double-pickup designs. The camp culminated in a public Showcase of student work.
We once again expanded the activities for the additional time-on-learning, relative to the original curriculum.3 Further, based on feedback from campers and co-facilitators, we planned for more time to be devoted to free-choice inventing (about 4.5 h in 2018, compared to about 3 h in 2017; see Supplementary material for details). A total of 32 campers attended at least 1 day of camp, with an average of 26.2 campers per day (4.09 days per camper). Of the 30 campers who opted-in to the research component of the camp, 16 were from races/ethnicities underrepresented in STEM (with three declining to self-identify), 14 self-identified as female, and nine completed their applications in Haitian Creole or Spanish.
Camp topics and activities included rapid prototyping; taking apart headphones and building electromagnets; assembly of speakers; fabrication of musical instruments (see Figure 1E); a youth-led activity on empathy; construction of electrical pickups; free-choice inventing; a videoconference with a drummer-and-inventor and a high-school-age musical inventor; and creation of informational pamphlets or slideshows. As in the previous year, campers presented their work in a public Showcase. Throughout the week there were again usually three or more adult facilitators and two or more participant-observers.
In our third camp, youth worked in teams of between three to four members, to make electronic doors, using electronic prototyping boards (i.e., “breadboards”; Figures 2A,E) that interfaced with a three-position switch and a servo motor to open and close doors made of foamboard (Figure 2B). The curriculum4 called for a 61 cm × 46 cm frame with a 30 cm x 30 cm opening (24″ × 18″ frame and 12″ × 12″ opening, Figure 2D). However, some campers created larger or smaller designs. For example, Figure 1F’s design is for a small pet, and Figure 2C’s is for a younger sibling. The camp again concluded with a public Showcase.
Figure 2. Creating Electronic Doors, using Principles of Direct Current (DC) Electricity & Simple Machines. (A) Using an electronic prototyping board (“breadboard”) to include a three-position switch for controlling the door (forward-off-reverse); (B) Close-up photo of servo motor when connected to metal arm, for increasing torque on the door during opening; (C) Scale of door roughly doubled, relative to the student curriculum guide; (D) Scale of door when replicating the instructions in the student curriculum guide (see Figure 1F for a reduced-scale door); (E) Camper-drawing of door assembly (breadboard not yet detailed).
Due to a weather delay, we had 24 total hours of activities, or two fewer than previous years. A total of 31 campers attended at least 1 day, with an average of 27.8 campers per day (4.48 days per camper). All campers opted-in to the research component of the camp, 12 of whom were from races and ethnicities underrepresented in STEM (with one declining to self-identify), six self-identified as female, and seven were emergent multilingual campers. We were concerned by the disparity of female and male campers, a phenomenon we partially attribute to low interest in the topic, which was likely exacerbated by culturally and socially gendered behavior norms, as will be elaborated in the “Successes & Challenges” section.
Camp activities and topics included rapid prototyping; simple machines; fabrication of the door and its frame; wiring an electrical circuit to control a servo motor; free-choice inventing; two videoconferences (one with an intellectual-property attorney, and the other with an Assistant Professor of Art); pamphlet-making, with some specific guidance around procedure-writing; and the culminating community Showcase.
Our in-school-time partnership is detailed elsewhere (Zhang et al., 2021), with a scope-and-sequence, student work artifacts, alignment to the Next Generation Science Standards, interdisciplinary connections, safety concerns, classroom management tips, and material costs (See Supplementary material for costs of all projects). The student and teacher guides are available online5, free of charge. In brief, the curriculum involves students designing and building shoebox-size insulating devices, using principles of heat transfer (conduction, convection, and radiation), biomimicry (e.g., of penguins and seals), and thermoelectric effects (including a Peltier tile for converting electrical energy to thermal energy). Alongside curriculum-embedded connections like urban heat islands, students identified needs for everyday lunchboxes, longer-term food storage, and medical transport, especially in situations where electrical grid access is scarce (e.g., in rural areas and/or due to natural disasters). See Figures 1C, 3 for representative photos.
Figure 3. Creating Shoebox-size Insulating Devices, Testing Them in a Contest, and Sharing via Posters. (A) In addition to the primary goal of minimizing temperature, there were goals and prizes for efficiency, collaboration, and creativity; (B) The control box had minimal insulation; (C) Teams created boxes with both aesthetics and functionality in mind; (D) Each of four classes had its own “contest”, followed by a cluster-wide competition of the top performing designs from each class plus two “wild card” entries; (E) Youth created posters for science, engineering, and invention concepts.
We partnered with both middle schools in Mills City, Northwest Middle School (NMS) and Central Middle School (CMS). The racial/ethnic demographics of the two middle schools are shown in Table 2. CMS has tended to have more multilingual students and families, so the members of the research team with specialties in language-learning worked more closely with CMS. On the other hand, research specialists in science education worked more with NMS.
The racial/ethnic and linguistic (alternatively, raciolinguistic) dynamics manifested differently across the two schools. For example, at CMS the in-service educators emphasized the value of literacy-based activities such as procedure- and patent-writing. At NMS, in-service educators noticed both US- and internationally-born students making connections with their ancestors from up to several generations ago (e.g., when talking about how their “home cultures” keep things hot or cold). In sum, the curriculum showed flexibility in adapting to two very different school and community contexts within the same city, as implemented by classroom teachers with researcher-participant support.
To evaluate our interventions, we have mixed qualitative and quantitative methods to converge towards deeper understandings of campers’ and counselors’ lived experiences (Creswell and Plano Clark, 2018; Jackson and Bendiksen, 2020; Jackson and Semerjian, 2020; Zhang et al., 2021). On average, for camps we sourced data from over 100 daily questionnaires (~20 students/day * 5 days), around a dozen pre- and post-interviews, and around a dozen hours of video and audio recordings supplemented with field notes by in-person observers. For classes, we had several hundred student questionnaires per year (~160 students * ~3 questionnaires per year); about a dozen each of pre- and post-interviews; and around a dozen hours of field notes, several of which were supplemented with audio and video recordings. Observation protocols were focused on student engagement and invention practices, in ways that sought to elicit qualitative mechanisms of any quantitative changes in self-efficacy.
We analyzed quantitative data using t-tests of means if only pre- and post-questionnaires were available, or analyses of variance (ANOVAs) if mid-questionnaires were also available (Field, 2013). For qualitative data, we conducted two rounds of coding: first, coding for processes, emotions, and endogenous or “in vivo” codes; and second, looking for commonalities or connections through pattern and axial coding (Miles et al., 2014). Our quantitative scales routinely achieved reliabilities of between 0.78 and 0.92 for Cronbach’s α, an acceptable range for the interdisciplinary natures of inventing and STEAM (Field, 2013). For qualitative analyses, we worked in pairs until reaching interrater reliabilities between 0.85–0.90 or greater, per criteria from Saldaña (2009). Further details are omitted due to space constraints, yet they are available free-of-charge elsewhere (Jackson and Bendiksen, 2020; Jackson and Semerjian, 2020; Zhang et al., 2021).
For the purposes of this article, we share some key successes and challenges below. Across the three in-school-time interventions of one invention project and the three out-of-school interventions of different invention projects, some patterns have emerged for student engagement, along with processes of developing self-efficacy for science, design, and inventing. Though the long-term nature of our partnership has enabled us to change some initial challenges into eventual successes, other challenges still remain.
Overall, the interventions have been successful at engaging students in affective, behavioral, cognitive, and social ways (Fredricks et al., 2016; Zhang et al., 2021). One major finding is that social engagement seems to counteract individually-based anxiety, especially for in-school-time interventions. In one representative quotation, seventh-grader Daniela stated that “…there was other people working with me that could help me, and it wasn’t really something to get anxious about.” Another key finding is that there was increased engagement during out-of-school-time implementations compared to students’ typical experiences in science classes. This difference is supported by quantitative data, such as the item, “I pay more attention in vacation camp than I do in school science,” for which responses averaged 5.5/7 for the entire camp and 5.9/7 for days 2–5, the more hands-on and project-focused days. Those averages, which met criteria for normal distribution, indicate a slight to moderate agreement that students paid more attention in vacation camp, as equal engagement would have been a score of 4.0/7 on the 1–7 Likert-style scale of “strongly disagree” to “strongly agree.” For finer-grained analyses of individual students, see Jackson (2022). Though we recognize that in-school-time and out-of-school-time environments come with different affordances and limitations (Bevan et al., 2010), we agree with eighth-grade camper Pedro that in-school-time environments could benefit from “more freedom” without teachers checking-in “every 5 s.”
In terms of self-efficacy, we found evidence that students developed through their conceptions of ability, as well as through their management of anxiety. Unsurprisingly, the most pronounced developments are the most closely connected to a given curriculum unit (e.g., “I can demonstrate heat transfer,” “I can apply my understanding of electromagnetism to build an electric door,” etc.). On the other hand, we have seen little development related to more abstract or domain-general skills (e.g., “I can work in MANY different ways on my own invention project” and “I can work in MANY different ways as part of an invention team,” etc.). Though the high turnover rate for our middle-school camps has thus far hampered efforts to examine possible long-term development of the more abstract skills, we are optimistic in part due to findings about self-efficacy and identity development on high-school invention teams (Invention Education Research Group, 2019). Perhaps most importantly, we are encouraged that differences in self-efficacy development have not widened any gaps with respect to gender or race/ethnicity, and occasionally have narrowed such gaps.
Over time we have made a revised curriculum, increasing accessibility through differentiating for (dis)abilities, interests, modalities, and linguistic registers. In expanding the Chill out problem framing from a “lunchbox” to an “insulating device,” we created space for students to generate culturally relevant problems like medicine transportation and food preservation. For Noise makers, we allowed two or zero electronic pickups, instead of mandating precisely one pickup. During U Control, we supported designs that doubled or halved the expected door-size, including some designs that had doors-within-doors. In addition to those educational design choices towards leveraging interest, we did a labor-intensive conversion of the Chill out curriculum from PDF to Google Doc format, enabling students to use dictionary, translation, highlighting, and screen-reader tools. For students sensitive to loud noises, we gave extra space and separately performed woodworking per their instructions. Some youth with diagnosed disabilities for processing or executive function required more frequent check-ins. Finally, in offering summative assessments like posters, presentations, pamphlets, and video advertisements, we encouraged the use of colloquial language that nonetheless addressed canonical science.
Despite quantitative evidence that gender was not a statistically significant factor for engagement and self-efficacy (detailed in Jackson and Bendiksen, 2020), some qualitative findings suggest that more work is needed to ensure equitable participation with students of all genders. For example, during the U Control camp for electric doors, a mixed-gender group had two boys doing most of the tinkering and two girls doing most of the doodling. In post-interviews, the two girls expressed a desire for more intra-team talking, which could have been facilitated by high-school counselors and adult advisors. We have since adjusted our training sessions to include more pedagogical knowledge and pedagogical content knowledge, whereas previous trainings overemphasized content knowledge.
One minor challenge remains survey response rates, especially during out-of-school-time. In hindsight, we may have been overly cautious in our efforts to avoid taking too much space for student questionnaires, especially when trying to preserve a less “school-like” environment during out-of-school-time. Distributing surveys earlier in camp days should reduce the number of missed responses due to students leaving early or hastily. For in-school-time, more streamlined formats like Google Forms could yield the same information as the more complicated survey software we have used in the past, whose advanced features are not necessary for these interventions.
Finally, we still have work to do to support students in considering inventing as a career option. In pre-/post-measures from in-school-time interventions, career intentionality towards inventing has either remained stagnant or decreased. Though in part we can attribute this to more realistic conceptions of inventing (e.g., disrupting the myth of “lone wolf” inventors), we could do a better job of showing diverse approaches to inventing. One teacher in particular has taken extra time and energy to ensure that examples include amateur and/or adolescent inventors, an initiative that she reports has resulted in increased student engagement during some of the more reading-, video-, and writing-intensive activities.
Our experiences suggest that educational designers—whether they work as curriculum specialists, classroom teachers, camp or club facilitators, or in other roles—could benefit from thinking with a six-dimensional framework for student engagement, which we believe to be an improvement upon existing three-and four-dimensional models (Jackson, 2022; Reschly and Christenson, 2022). Namely, we found that affective, behavioral, and cognitive engagement are qualitatively different when approached individually or socially. Specifically, we recommend individual-affective, individual-behavioral, individual-cognitive, social-affective, social-behavioral, and social-cognitive dimensions. This is not to say that categories should be approached exclusively in isolation; rather, educational design should consider how various dimensions support each other (Jackson, 2022; Reschly and Christenson, 2022). Recalling an earlier example, the mixed-gender group members from U Control were engaged or disengaged in individual-behavioral and individual-affective senses (i.e., boys consistently excited with tinkering and girls eventually bored with doodling), yet they were not engaged in social-behavioral or social-affective ways (i.e., group members neither talking much with each other nor substantially supporting each other emotionally). We found that this example and others suggest that invention education – with its personal relevance and team-based approach—is a particularly fertile field for exploring the six-dimensional model of student engagement.
Though it might appear that the out-of-school-time implementations prioritized engagement and interest at the expense of conceptual understanding and canonical practices (and vice versa for in-school-time implementations), we agree with prior work showing that each setting can and does promote both affective and cognitive outcomes (Bevan et al., 2010; Sneider and Allen, 2019). For example, we found that in-school-time learning could foster joy while mitigating anxiety, and that out-of-school-time learning can promote deeper cognitive engagement with topics previously explored through casual hobbies (e.g., deepening concepts of electricity or simple machines, which were initially approached with toy trains or furniture-making). In terms of STEAM practices, it is not only in-school-time that can promote holding commitments to standards of the field, nor is it only out-of-school-time that can foster more expansive approaches to learning such as engaging multiple modalities (Bevan et al., 2019).
In terms of measurement, we recommend that student self-assessments are expanded both in response scale and in gradations of challenge (Bandura, 2006). For example, we found that seven-point scales worked better than five-point scales, which in turn worked better than our initial three-point scales. Also, we noticed that adding modifiers like “very” and “many” allowed us to see finer-grained changes in students developing self-efficacy (e.g., “I can work very well…,” “I can think of many uses…”). Further, these self-assessments should be given well before the end of a session, as we have found that many students run out of time or leave a little early, especially during camps. These self-assessments can aid not only in youths’ personal reflection and meta-cognition, but also as formative assessments for educational design and implementation (Bell et al., 2019; Sneider and Allen, 2019).
Finally, a concern that we anticipated would be important yet we nonetheless underestimated, is the importance of topics being high-interest to youth, including what Bevan et al. (2019) call a STEAM practice of “finding relevance.” Though it can be resource-intensive to update existing curricula for more recency and relevance, in our experience such investment has been worthwhile. One way to share the workload, which also adds a diversity of experience, is to include near-peer youth in the planning process (e.g., high-school youth as co-designers of interventions for middle-school youth). For us, it took a full year to establish strong connections between adults and high-schoolers in terms of co-design, which ultimately proved to be beneficial for middle-schoolers.
Moving forward, we plan to design for three-student groups as much as supplies will allow, despite the four-part Types of Team Members framework. We believe that such a decision will allow for both individual responsibility and collective flexibility. Namely, each teammate will have a primary role alongside a share of the one undesignated role. We hope that this design consideration will encourage a firmness yet fluidity in task-sharing, as we saw in varying degrees during the U Control implementation in particular (as mentioned in Key successes and Key challenges, and further detailed in Jackson, 2022). Also, it will enable students to more deeply engage in the STEAM practice of exploring materiality (Bevan et al., 2019), as smaller groups will have more physical access to supplies.
Another change we will make is diversifying the awards or recognition for in-school-time interventions. While we acknowledge the general-scientific and standards-specific importance of an insulating device’s effectiveness (i.e., minimizing temperature change; NGSS Lead States, 2013), we also seek to encourage sustainability by awarding prizes or other attention for efficiency (i.e., minimal temperature change AND minimal and/or sustainable materials use; Gunckel and Tolbert, 2018). Further recognition could expand to disciplines related to technological inventing adjacent to the “STEM” umbrella, such as precision of patent writing (language arts), attention to sociocultural concerns of users/clients/benefactors (social studies), and aesthetic appeal (visual arts).
Finally, we plan to extend our work into developing fields like sustainable chemistry and physical computing. Already we have piloted units on earth-friendly bioplastics (Green Chemistry) and microcontroller-connected toys (Toy Design, with BBC micro:bit), developing or maintaining partnerships with local businesses. Revisions of the pilot curricula are ready for future camps, and these new curricula will replace units that stimulated less interest in past years.
Supported by strong connections amongst community members, district-level policy-makers, practitioners, researchers, and youth, our collaboration has resulted in Shoe soles becoming part of the district-wide 7th grade curriculum; an annual oversubscription to the February camp; and several units in after-school clubs that we omit here for conciseness. In addition to these practical contributions in the emerging field of invention education, our work has contributed to conceptualizations of student engagement, supporting a new six-dimensional framework that better harmonizes individual/psychological and collective/social dynamics. Further, we contribute to literature on finer-grained analyses of self-efficacy development, broadening the participant pool and subject areas for self-efficacy studies (Schunk and DiBenedetto, 2022). As the in-school-time and out-of-school-time implementations continue to inform each other, the research-practice-community partnership seems poised to generate design considerations to foster equitable student engagement in Mills City and beyond.
The datasets presented in this article are not readily available because the datasets for this study are kept confidential per agreement with the Institutional Review Board of Boston College, including informed consent with all adult participants/guardians and informed assent with all youth participants. Requests to access the datasets should be directed to GB, YmFybmV0Z2VAYmMuZWR1.
The studies involving humans were approved by the Boston College Institutional Review Board. The studies were conducted in accordance with the local legislation and institutional requirements. Written informed consent for participation in this study was provided by the participants’ legal guardians/next of kin.
DJ: Conceptualization, Data curation, Formal analysis, Investigation, Methodology, Project administration, Software, Validation, Visualization, Writing – original draft, Writing – review & editing. HZ: Conceptualization, Data curation, Formal analysis, Investigation, Methodology, Project administration, Supervision, Validation, Writing – review & editing. CA: Conceptualization, Formal analysis, Investigation, Methodology, Writing – review & editing. AS: Conceptualization, Data curation, Formal analysis, Investigation, Methodology, Software, Validation, Writing – review & editing. GB: Funding acquisition, Resources, Supervision, Validation, Writing – review & editing. SC: Funding acquisition, Investigation, Project administration, Resources, Supervision, Writing – review & editing. LE: Investigation, Project administration, Resources, Supervision, Writing – review & editing. JK: Investigation, Project administration, Resources, Writing – review & editing. NK: Investigation, Project administration, Resources, Writing – review & editing.
The author(s) declare financial support was received for the research, authorship, and/or publication of this article. This work was financially supported by the Lemelson-MIT JV InvenTeams Program and a Collaborative Fellows Grant from Boston College.
The authors appreciate the enthusiasm, curiosity, and participation of middle-school students and campers; the co-designing, co-leadership, and near-peer mentoring of high-school-age camp “counselors”/co-facilitators; the co-designing, lead-teaching, and assistant-teaching of Mills City faculty/staff; Mills City educational administrators for their continued collaboration; and current and past members of the Lemelson-MIT JV InvenTeams Program for creating and revising the curricula.
The authors declare that the research was conducted in the absence of any commercial or financial relationships that could be construed as a potential conflict of interest.
All claims expressed in this article are solely those of the authors and do not necessarily represent those of their affiliated organizations, or those of the publisher, the editors and the reviewers. Any product that may be evaluated in this article, or claim that may be made by its manufacturer, is not guaranteed or endorsed by the publisher.
The Supplementary material for this article can be found online at: https://www.frontiersin.org/articles/10.3389/feduc.2024.1287521/full#supplementary-material
1. ^All K-12 city, district, educator, school, and youth names are pseudonyms.
2. ^ https://lemelson.mit.edu/curriculum-invention/jv-inventeams-shoe-soles
3. ^ https://lemelson.mit.edu/curriculum-invention/jv-inventeams-noise-makers
4. ^ https://lemelson.mit.edu/curriculum-invention/jv-inventeams-u-control
5. ^ https://lemelson.mit.edu/curriculum-invention/jv-inventeams-chill-out
Allen, A., Ball, M., Bild, D., Boone, D. N., Briggs, D., Davis, D., et al. (2020). “Leveraging out-of-school STEM programs during COVID-19” in Connected science learning Available at: https://www.nsta.org/connected-science-learning/connected-science-learning-october-december-2020/leveraging-out-school
Bandura, A. (2006). “Guide for constructing self-efficacy scales” in Self-efficacy beliefs of adolescents (Greenwich, CT: Information Age Publishing), 307–337.
Bell, J., Crowley, K., Storksdieck, M., Besley, J., Cannady, M. A., Nelson, A. G., et al. (2019). “Defining and measuring STEM identity and interest in STEM learning” in Connected science learning Available at: https://www.nsta.org/connected-science-learning/connected-science-learning-october-december-2019/defining-and-measuring
Bevan, B., Dillon, J., Hein, G. E., Macdonald, M., Michalchik, V., Miller, D., et al. (2010). “Making science matter: collaborations between informal science education organizations and schools” in A CAISE inquiry group report (Washington, DC: Center for Advancement of Informal Science Education)
Bevan, B., Peppler, K., Rosin, M., Scarff, L., Soep, E., and Wong, J. (2019). “Purposeful pursuits: leveraging the epistemic practices of the arts and sciences” in Converting STEM into STEAM programs: environmental discourses in science education. eds. A. J. Stewart, M. P. Mueller, and D. J. Tippins (Cham: Springer International Publishing), 21–38.
Cho, S., Crenshaw, K. W., and McCall, L. (2013). Toward a field of intersectionality studies: theory, applications, and praxis. Signs J. Women Cult. Soc. 38, 785–810. doi: 10.1086/669608
Creswell, J. W., and Plano Clark, V. L. (2018) Designing and conducting mixed methods research. 3. Los Angeles, CA: SAGE.
Fraser, S., Barnes, N., Kilpatrick, S., Guenther, J., and Nutton, G. (2021). Considering young people’s dislocation from STEM education: looking beyond the narrow focus of teaching and learning practice within school. Front. Educ. 6:678613. doi: 10.3389/feduc.2021.678613
Fredricks, J. A., Wang, M. T., Schall Linn, J., Hofkens, T. L., Sung, H., Parr, A., et al. (2016). Using qualitative methods to develop a survey measure of math and science engagement. Learn. Instr. 43, 5–15. doi: 10.1016/j.learninstruc.2016.01.009
Galindo, C. L., Brown, T. M., and Lee, J. H. (2022). “Expanding an equity understanding of student engagement: the macro (social) and micro (school) contexts” in Handbook of research on student engagement. eds. A. L. Reschly and S. L. Christenson (Cham: Springer International Publishing), 383–402.
Gunckel, K. L., and Tolbert, S. (2018). The imperative to move toward a dimension of care in engineering education. J. Res. Sci. Teach. 55, 938–961. doi: 10.1002/tea.21458
Hofstetter, R. (2012). Educational sciences: evolutions of a pluridisciplinary discipline at the crossroads of other disciplinary and professional fields (20th century). Br. J. Educ. Stud. 60, 317–335. doi: 10.1080/00071005.2012.729666
Invention Education Research Group. (2019). Researching invention education: a white paper. Available at: https://lemelson.mit.edu/sites/default/files/2020-04/ResearchingInventEdu-WhitePaper-2.21.2020.pdf. (Accessed February 27, 2023)
Jackson, D. W. (2022). “Magic” or “maybe … other years”: designing for young adolescents’ engagement and self-efficacy in an invention camp. Int. J. Sci. Educ. B 12, 374–393. doi: 10.1080/21548455.2022.2120781
Jackson, D. W., and Bendiksen, P.. (2020). Youths’ self-efficacy and practices in a vacation camp for team-based inventing of electronic doors (structured poster session). AERA Annual Meeting. San Francisco, CA. Available at: http://tinyurl.com/y6qwjqua
Jackson, D. W., and Semerjian, A. R. (2020). Self-efficacy, identity, and interest amid an invention contest: a phenomenological study in required seventh-grade classes (roundtable session). AERA Annual Meeting. San Francisco, CA. Available at: http://tinyurl.com/vftltn2
Massachusetts Institute of Technology (MIT). (2016). Chill out: educator guide. Cambridge, MA: Lemelson-MIT JV InvenTeams Program.
Miles, M. B., Huberman, M. A., and Saldaña, J.. (2014). Qualitative data analysis: a methods sourcebook. 3. Los Angeles, CA: SAGE Publications.
National Governors Association. (2010). Common core state standards for English language arts & literacy in history/social studies, science, and technical subjects. Washington, DC: Center for Best Practices, Council of Chief State School Officers. Available at: http://www.corestandards.org/wp-content/uploads/ELA_Standards1.pdf. (Accessed March 23, 2022)
National Science and Technology Council. (2018). Charting a course for success: America’s strategy for STEM education. Available at: https://www.energy.gov/sites/prod/files/2019/05/f62/STEM-Education-Strategic-Plan-2018.pdf
NGSS Lead States. (2013). Next generation science standards: for states, by states. Washington, DC: The National Academies Press.
Njoo, E., Narain, S., Pabbisetty, L., and Agrawal, M. (2018). “Emerging connections: resourceful and effective ways of bridging afterschool STEM programs and local community” in Connected science learning Available at: https://www.nsta.org/connected-science-learning/connected-science-learning-october-december-2018-0/emerging-connections
Pérez-Salas, C. P., Parra, V., Sáez-Delgado, F., and Olivares, H. (2021). Influence of teacher-student relationships and special educational needs on student engagement and disengagement: a correlational study. Front. Psychol. 12:708157. doi: 10.3389/fpsyg.2021.708157
Radil, A. I., Goegan, L. D., and Daniels, L. M. (2023). Teachers’ authentic strategies to support student motivation. Front. Educ. 8:1040996. doi: 10.3389/feduc.2023.1040996
Reschly, A. L., and Christenson, S. L. (2022) Handbook of research on student engagement. 2. Cham: Springer International Publishing
Saldaña, J.. (2009). The coding manual for qualitative researchers. Thousand Oaks, CA: SAGE Publications, Inc.
Schunk, D. H., and DiBenedetto, M. K. (2022). “Self-efficacy and engaged learners” in Handbook of research on student engagement. eds. A. L. Reschly and S. L. Christenson (Cham: Springer International Publishing), 155–170.
Sneider, C., and Allen, S. (2019). “Formative assessment of STEM activities in afterschool and summer programs” in Connected science learning Available at: https://www.nsta.org/connected-science-learning/connected-science-learning-july-september-2019/formative-assessment-stem
Keywords: student engagement, invention education, science education, engineering education, middle school, classroom learning, informal learning, educational design
Citation: Jackson DW, Zhang H, Asante CK, Semerjian AR, Barnett GM, Couch S, Estabrooks L, Kiel J and Kulkarni N (2024) Inventors emerging in-school and out-of-school: six iterations of educational design to promote equitable student engagement. Front. Educ. 9:1287521. doi: 10.3389/feduc.2024.1287521
Received: 01 September 2023; Accepted: 29 January 2024;
Published: 13 February 2024.
Edited by:
Joanna K. Garner, Old Dominion University, United StatesReviewed by:
Antonio Luque, University of Almeria, SpainCopyright © 2024 Jackson, Zhang, Asante, Semerjian, Barnett, Couch, Estabrooks, Kiel and Kulkarni. This is an open-access article distributed under the terms of the Creative Commons Attribution License (CC BY). The use, distribution or reproduction in other forums is permitted, provided the original author(s) and the copyright owner(s) are credited and that the original publication in this journal is cited, in accordance with accepted academic practice. No use, distribution or reproduction is permitted which does not comply with these terms.
*Correspondence: David W. Jackson, ZGF2aWR3akBidWZmYWxvLmVkdQ==
Disclaimer: All claims expressed in this article are solely those of the authors and do not necessarily represent those of their affiliated organizations, or those of the publisher, the editors and the reviewers. Any product that may be evaluated in this article or claim that may be made by its manufacturer is not guaranteed or endorsed by the publisher.
Research integrity at Frontiers
Learn more about the work of our research integrity team to safeguard the quality of each article we publish.