- 1Department of Methods of Teaching Mathematics, Physics and Informatics, Abai Kazakh National Pedagogical University, Almaty, Kazakhstan
- 2Department of Science and Mathematics Education, SDU University, Kaskelen, Kazakhstan
- 3Department of Plasma Physics, Nanotechnology and Computer Physics, Al-Farabi Kazakh National University, Almaty, Kazakhstan
- 4Faculty of Education, University of Lethbridge, Lethbridge, AB, Canada
Science, technology, engineering, and mathematics (STEM) fields play a critical role in the advancement of society and are expected to grow rapidly in the coming years. This study examines the development of a STEM education course and its impact on teachers’ self-efficacy and course experiences. The study involves a mixed-methods approach, using survey and course assignment results gathered from 52 mathematics master’s degree candidates who took an online STEM education course. Teachers’ self-efficacy, STEM knowledge, reflections from reading materials, and lesson plans were quantitatively analyzed while content analyses was employed for the teachers’ opinions on the role of each STEM subject and overall course evaluation. Results showed a significant increase in teachers’ self-efficacy in teaching STEM subjects after completing the course. Additionally, teachers reported positive experiences related to course content, activities, and assignments. The study provides insights into the design and implementation of effective STEM courses and provides practical implications for designing operative STEM courses.
Introduction
Teacher self-efficacy and professional growth
Teacher self-efficacy refers to a teacher’s belief in their ability to effect student outcomes through instructional practices (Bandura, 1997). Self-efficacy is not at a constant level throughout one’s career and there are many factors that influence the increase or decrease of one’s self-efficacy. A teacher’s self-efficacy changes over time through a career peaking at approximately 23 years of experience and declining in later career stages (Klassen and Chiu, 2011). Day and Gu (2010) reported that a teacher’s self-efficacy wanes and increases within a given school year depending on the expectations that are placed on a teacher during a specific time of year. Klassen and Chiu (2011) also found that one’s social work environment and how one is perceived within a teaching or school community can both positively and negatively impact one’s self-efficacy. Cooper and Carr (2019) noted that “low levels of teacher self-efficacy in teaching STEM-related disciplines is linked to poor teaching practices such as teacher-centred pedagogy, poor questioning and avoidance of teaching concepts considered too difficult” (p. 182). Ensuring that a teacher’s self-efficacy is increased with respect to teaching science, technology, engineering, and mathematics (STEM) courses is imperative to improving the STEM experience for K-12 students.
To increase one’s self-efficacy, a teacher might engage in enhancing their instructional strategies or increasing their content knowledge of a discipline. Ways that teachers choose to increase their professional and content knowledge through formal means like engaging in mandated or optional professional development programs or taking courses at a post-secondary institution or through informal means like self-directed learning or conversations with colleagues. Palmer (2011) found that cognitive mastery in understanding a concept was more impactful on a teacher’s self-efficacy than past hands-on experience or enactive mastery. Avalos (2011) reviewed articles on teacher professional development and identified that both cognitive theories, including self-efficacy, and socio-cultural theory, including teaching context, play a role in teacher growth through a variety of forms of professional learning. In addition, Kayan-Fadlelmula et al. (2022) relates self-efficacy to positive outcome expectations and the achievement of those positive outcomes.
A conceptual model of teacher professional learning that worked to positively influence teacher self-efficacy was developed by Beauchamp et al. (2014). Their model included mastery experience, verbal persuasion, vicarious experience, and affective/physio states within a collaborative environment that works together to enhance teaching practice and, as a result, improve teacher self-efficacy. Beauchamp et al. (2014) found that “teacher efficacy was fostered by professional learning that allows teachers time to meet and talk, and spaces that promote conversation and collaboration” (p. 48). They recommended that professional development for teachers that increases one’s self-efficacy be built around “sharing curriculum ideas and best practices, co-creating and sharing learning and teaching resources and learning new teaching strategies” (p. 59). Milner-Bolotin (2018) argued that “in order to change how students engage with STEM we have to change how we educate K-12 teachers and how we support them during their careers” (p. 2). Increasing teacher self-efficacy through professional development is of importance to STEM education as ensuring current teachers have the skill and knowledge to work in innovative ways to support student learning.
STEM education
Science, technology, engineering, and mathematics education is a relatively new discipline in K-12 education and, as such, in pre-service education and in-service teacher professional development. As K-12 schools are implementing STEM programs and looking for teaching practices that integrate the fields of science, technology, engineering, and mathematics in multidisciplinary ways, “Educators at all levels are grappling with the complexities and issues that are emerging in what is a relatively new, and some might argue, ill-defined field” (Barkatsas et al., 2018, p. 93). With a call for a growing need for multi-disciplinary innovators in an increasingly global world, Barkatsas et al. (2018) recognized the need for current teachers to have support in the form of resources and knowledge to effectively implement STEM programs. Kayan-Fadlelmula et al. (2022) add that there is a need for teacher professional development programs that support one’s efficacy and interest in teaching STEM. Mutseekwa (2021) proposes that practices such as field trips, work visits, and partnerships can facilitate increased collaboration among colleges, schools, professional scientists, and industry.
STEM course development
The impact of various instructional strategies and course design features appears to differ. To facilitate the learning of course content in online STEM courses, designers should take into account a variety of instructional strategies and course design features. This may include fostering a learning community and providing opportunities for experienced students to impart their knowledge and share resources with their peers (Yang, 2017).
In order to develop a course to support the growth of teacher efficacy in teaching in a STEM context, courses at both the pre-service and in-service teacher levels were examined. In-service courses considered both courses offered at a master’s level or orchestrated professional development experiences that were more than a one-time event. Though the designing of pre-service and in-service teacher education courses considers different characteristics of the audience and level of experience in a classroom or with a curriculum, both can offer insights into structures that can be identified as successful for different audiences (Nurbaeva et al., 2023).
Byrd et al. (2022) noted that, with respect to pre-service teachers, “meaningful experiences as a learner and an educator in integrated STEM methods courses” (p. 188) would lead to teachers that are more able to integrate standards from different disciplines and would lead to increased self-efficacy in planning and implementing STEM lessons. What constitutes a meaningful experience depends on the level of engagement of the students in the potential teaching of a STEM lesson to experiencing a STEM lesson as a student. Richmond et al. (2017) found that integrating “active learning strategies to model reform-based classroom practice” including “case studies and cooperative learning” (p. 20) activities that engage teachers in experiences that help them make sense of teaching in a way that potentially different than what they have been engaged in previously.
Beyond what was identified in the literature with respect to teacher professional development and self-efficacy, the context of STEM education poses an additional threat to a teachers’ self-efficacy because the field is complex, multi-faceted, and ill-defined. Jong et al. (2021) noted that there is a need for teacher professional development in integrated and cross-disciplinary ways that support current teachers in developing their capacity beyond a singular discipline. Jong et al. (2021) identified that to build one’s capacity in teaching, a professional development program should include the following elements “(a) content focus, (b) use of models and modelling, (c) active learning, (d) collaboration, (e) coaching and expert support, (f) feedback and reflection, and (g) sustained duration” (p. 81). Each of these elements serves to increase teachers’ capacity for planning, teaching, and assessing in innovative ways that underlie STEM education.
Experiences in STEM courses
Pre-service and in-service teacher experiences through engaging in courses designed to support the development of confidence and skills in teaching a STEM course have been explored in a variety of settings. In pre-service teacher education, Cooper and Carr (2019) found that pre-service teachers valued experiencing and learning more about disciplinary integration within and between the STEM fields and engaging in “authentic learning and inquiry-based pedagogies” (p. 180). Having elements of a course where participants fully experience the learning that happens through authentic experiences further supports future integration of those practices within one’s context (Nielsen et al., 2019; Marynowski et al., 2021).
In-service teacher professional development also considers that the participants in the learning have professional experience that they draw on to position new learning within their experience of teaching. Rajbanshi et al. (2020) reported that in-service teacher educators need to be able to share their experiences alongside having opportunities to integrate new practices into their current context. Ensuring that there is both specific disciplinary content and pedagogy included for in-service teachers alongside opportunities for reflection about the implementation was noted by Nadelson et al. (2012). They stated that both content and pedagogy were important, however, teachers needed “time to think about STEM teaching and learning” (Nadelson et al., 2012, p. 166) so that they could develop a stronger sense of how to apply what they were learning.
One additional key component of experiences for both in-service and pre-service teachers was ensuring that the process of scientific inquiry (Nadelson et al., 2012) or the engineering design process (Shernoff et al., 2017) as a way to integrate seemingly separate disciplines in an integrated manner. As many teachers are trained in the pedagogy and/or in the content of separate disciplines, working in an integrated manner on potentially complex contexts or problems. Working in an integrated way on complex problems with in-service and pre-service teachers supports the later integration of disciplines in practice which is a goal of STEM education programs (Nadelson et al., 2012; Shernoff et al., 2017).
Several researchers have noted that there is a continued need to explore ways to enhance pre-service and in-service teacher professional development in unique knowledge and pedagogy in order to teach STEM in an integrated way (Teo and Ke, 2014; Milner-Bolotin, 2018; Margot and Kettler, 2019; Hill et al., 2020; Özer et al., 2020). The question that still remains is how can teachers be supported to improve their self-efficacy and knowledge of STEM education? There are many incentives for STEM education, but there are no effective courses to train teachers to provide this education. This study makes contribution to both literature and education as it investigates the effectiveness of a developed and implemented STEM course.
Research purpose and research questions
One of the ways to design a new course is to look at teachers’ perceptions and experiences which affect learning outcomes and satisfaction with a course. It is also essential to examine teachers’ interest and feedback regarding the instructional strategies and course design features in new courses. This research investigated effective instructional approaches and course design elements in an online mathematics class provided to master’s degree students. Teacher experiences in courses help in designing future courses and being able to identify what aspects of the course impacted knowledge, integration of STEM concepts, and self-efficacy (Nadelson et al., 2012; Rajbanshi et al., 2020; Abylkassymova et al., 2021).
The participants in this study were mathematics teachers who were teaching full-time, while enrolled in the online class. Their instruction experience and knowledge of teaching approaches let them give distinctive evaluations and comments associated with efficient teaching strategies and course design characteristics that contributed to their learning. Engaging in the course online is different from the other models of professional development for in-service teachers. In particular, the online context of the course was purposefully designed to ensure that the elements of effective teacher education were integrated. This research questions do not specifically attend to the fact that this course is online, however, this study serves to show that teacher self-efficacy, STEM content knowledge, and integration of STEM concepts into teaching can be improved through an online course.
The following research questions were identified for this study.
1. Are there any changes in the teachers’ self-efficacy after participating in the course?
2. Are there changes in the participants’ STEM education knowledge after participating in the course?
3. To what extent do mathematics teachers integrate STEM subjects into their lesson plans?
4. How do participants rate the role of each STEM subject?
5. What is the participants’ overall evaluation of the course?
Materials and methods
Participants and context of the study
The study involved 52 mathematics teachers pursuing their master’s degree at SDU University in Almaty, Kazakhstan. Among them, 13 (25%) were male, and 39 (75%) were female teachers. The participants had an average age of 26 and hailed from 12 different cities, with the majority (46%) originating from Almaty. Their teaching assignments covered grades 1 through 12, with approximately half instructing at high schools and the other half at middle and elementary schools. As these individuals were teachers enrolled in the “STEM Education” course, the sampling method employed can be characterized as convenient sampling. The context of the study is a complete semester program on STEM education for master’s degree students in mathematics (September–December 2022). This course comprised a 50-min lesson each week of theoretical lectures and two 50-min lessons per week of practical periods each. The course was taught online for teachers who were pursuing a master’s degree in mathematics education at a Kazakhstan rural university. The course had no face-to-face meetings and was hosted in Webex (an online course management system).1
The theoretical part of the course covered an introduction to STEM education (2 weeks), integrated STEM education (1 week), gender differences in STEM (1 week), project-based learning (1 week), engineering design loop process (1 week), research and trends in STEM education (1 week), challenges in STEM education (1 week), STEM curriculum design (1 week), the role of each silo of STEM in STEM education (4 weeks), STEAM (science, technology, engineering, art, and mathematics) education (1 week). On the other hand, the practical part of the course focused on sharing STEM lesson plans and design challenges along with having quizzes and reflecting on reading materials.
The course was assessed across teachers’ portfolios that consist of STEM lesson plans, design challenges, quizzes, participation, reflections on the reading materials, and presentation of produced works. Teachers filled in a pre- and a post-test survey respectively in the initial and the final week which investigated their STEM education knowledge and self-efficacy. All 52 teachers in the course gave their approval for this study.
Instrument
The research questionnaire was designed to assess teachers’ self-efficacy in STEM education. At the beginning of the questionnaire, the aims and background of the study were presented to the respondents. It was emphasized to the respondents that the research focus was on their views rather than on the specific content of the subject. The questionnaire was prepared using Socrative, an online assessment tool2 and consisted of three sections, which included both open-ended and closed-ended questions assessed on a five-point Likert scale. The first part included demographic items (such as gender), and in the second section, the teachers demonstrated their self-efficacy in STEM education with 20 Likert items (such as I am good at projects involving STEM). The third section addressed teachers’ knowledge about STEM education with four open-ended (such as What is STEM Education?) items (See Appendix). The Cronbach alpha coefficient was calculated as 0.804 and 0.860 respectively for pre-test and post-test scores on the second section of the questionnaire. Moreover, according to both pre-test and post-test scores, dropping none of the items increased the reliability coefficient. Along with standard Cronbach alpha coefficient we calculated the ordinal alpha coefficients for pre and posttests. They were calculated to be 0.86 for the posttest and 0.76 for the pretest, indicating high internal consistency in the ranking of responses for the posttest and slightly lower consistency for the pretest.
For the validity of the instrument, we sent it to two professors at SDU University for their views on the individual items. They evaluated the items in terms of relevance, clarity, and understandability. Depending on their feedback two items were revised and one item was dropped from the instrument.
Design and procedure
This is the second time this course has been offered online. The first offering was for undergraduate students, and the third author adapted the course and is teaching the course to master’s students for the first time. There were no prerequisites for this course, and the learning outcomes of the course were for participants: (1) develop scientific thinking strategies, (2) demonstrate basic knowledge in the fields of science, mathematics, and technology education, (3) utilize the vocabulary, primary concepts, definitions, and models applicable to STEM education, (4) develop innovative and alternative teaching methods and learning activities, and (5) analyze attributes, strengths, and weakness of current STEM education programs. These learning outcomes were measured informally at the beginning (pre-test) and at the end of the course (post-test) and formally through STEM lesson plans, STEM activities prepared by teachers, quizzes, participation and reflections from reading materials.
The main structure of the course design and the instructional strategies adopted were developed by the researchers based on the teacher professional development and STEM literature noted earlier. Instructional strategies included online discussion about the reading material, video demonstrations of design challenges, and discussion of STEM lesson plans. Teachers were awarded 10 points (around 6% of total grades) for participation in the online discussions. The reflection from reading materials asked teachers to summarize their understanding. Additionally, each teachers prepared four design challenges and four STEM lesson plans. Before preparing the design challenges and lesson plans, examples of these activities were presented to teachers. During the practice classes, teachers presented their lessons and design challenges with the instructor providing immediate feedback. The revised versions of both the design challenges and lesson plans, that is after the feedback, were uploaded to Moodle for prefinal and final grade evaluations. Participants provided MS Word documents for the lesson plans and YouTube links for the design challenges. The aim behind the YouTube videos was to have teachers use technology as an instructional strategy for the STEM course. No textbook was determined for the course and all reading materials were selected from journals indexed in SCOPUS. A summary of the designed course is presented in Figure 1.
Data analysis
To evaluate teachers’ self-efficacy, and knowledge before and after the course, a pre- and post-questionnaire was applied with a five-point Likert scale, ranging from 1 (strongly disagree) to 5 (strongly agree). Participants’ demographic information was also collected from the administered survey. T-statistics were used to test the proposed hypotheses for the group differences such as pre-posttests. Wilcoxon W test was carried out to compare the scores from the first two and last two lesson plans.
Teachers were required to write reflections for each week’s reading material. Each teacher’s reflection ranged from one to two single-spaced pages. Except for the midterm and the final week, teachers were assigned reading articles. Each teacher provided 13 reflections. There were 52 teachers and a total of 615 reflections (61 reflections were not submitted). There was a rubric for the reading reflections which was composed of three sections; summary of the reading (70 points), top three Take-Aways (20 points), and questions I still have (10 points).
The rubric designed for evaluating the lesson plans developed by teachers were as follows: face validity and organization, that is the appearance of the lesson plan if it contains elements, such as date, aim, duration, school level, etc., of a standard lesson plan (10 points), objectives related to STEM education (20 points), description of the STEM activity (30 points), integration of the STEM subjects in the activity (20 points), assessment (10 points), and presentation (10 points). The first two lesson plans were prepared for the prefinal and were submitted for evaluation together, similarly, the last two lesson plans were submitted together and were graded for the final. That is why, we merged the scores from the first and second lesson plans and did the same for the third and fourth lesson plans. In other words, for each teacher, the average scores from the first two and last two lesson plans were compared for the effect of the course.
Similarly, design challenges were evaluated on the following bases: organization and quality (20 points), description of the activity (50 points), integration of the STEM subjects in the activity (20 points), and presentation (10 points). Regarding analyses of qualitative data, we utilized a content analysis, where views were categorized within teachers’ responses to open-ended questions.
Data collected from quizzes, participation, reflections from reading materials and the design challenges were not analyzed in this study because they were beyond the research questions of the current study.
Results
Changes in teachers’ self-efficacy
To respond to the first research question, that is, are there any changes in the teachers’ self-efficacy after participating in the course? we used the results from the self-efficacy questionnaire. With the teachers responses, the following analyzes were performed.
A paired sample t-test (Table 1) was carried out to locate the difference between teachers’ pre- and post-test scores about their self-efficacy in STEM education. The normality of the data was assessed by the Shapiro–Wilk test and a normal distribution was detected (p > 0.05).
The t-test indicated that teachers’ post-test mean score (3.95) is significantly higher than the pre-test mean score (3.77). The means of pre and post-test scores are visualized in Figure 2.
Changes in STEM education knowledge
For the second research question, teachers’ STEM knowledge (second research question) was assessed through participant responses to the following open-ended questions on the questionnaire: What is STEM? What is STEM Education? What is integrated STEM Education? What is STEAM?
To see the effect of the STEM education course on teachers’ STEM knowledge their initial and final (that is before the course and after the course) responses to these questions were compared. The comparisons were based on both the number of responses and a number of correct responses provided. Firstly, it was hypothesized that the more blank responses the more they do not know about STEM education. The number of teachers who responded to these four questions before the course was 46, 44, 27, and 29 respectively while after the course it was 47, 48, 46, and 47 respectively. This is visualized in Figure 3.
Teachers’ responses (correct or incorrect) for the first two questions are almost the same while for the last two questions after the course they provided significantly more answers. The statistical significance test was done through the Chi-Square Goodness of Fit Test where for the third question χ2 = 4.95, p = 0.026, and the fourth question χ2 = 4.26, p = 0.039.
Secondly, it was hypothesized that the more correct responses the more they do have knowledge about STEM education. A number of correct responses for the items assessing teachers’ knowledge about STEM education before and after the course are depicted in Figure 4.
Figure 4 indicates that the number of correct answers substantially increased after the course. The Chi-Square Test of Goodness of Fit was run to locate statistically significant differences. For what is STEM the statistics were calculated as χ2 = 4.90, p = 0.027, for what is STEM Education χ2 = 1.65, p = 0.199, for what is integrated STEM Education χ2 = 10.40, p = 0.001, and for what is STEAM χ2 = 6.42, p = 0.011. These statistics demonstrate that except for what is STEM, teachers’ correct responses after the course are significantly higher than their initial responses.
Integration of STEM subjects
To determine a response to the third research question (To what extent do mathematics teachers integrate STEM subjects into their lesson plans?) participants’ lesson plans were examined. They prepared first and second lesson plans for the prefinal and third and fourth lessons plans for the final exam. We hypothesized that as the course progresses participants will prepare better STEM lesson plans. Lesson plans were examined on six variables; face validity and organization, objectives, STEM activity, integration of STEM subjects, assessment, and school level at which the lesson plans were prepared for.
There were 52 participants and from the first, second, third, and fourth lesson plans prepared by participants, we randomly selected 20 from each category (totally 80 lesson plans). The statistics such as the number of lesson plans examined, the mean of scores from each variable, and the standard deviation are presented in Table 2.
According to Figure 5, participants’ first two and last two lesson plans seem to differ for integration of the STEM subjects, and school level while others such as face validity of the lesson plans remain similar. The statistically significant differences between the mean of the scores are tested through the Wilcoxon W test because our data indicated non-normal distribution.
Table 3 indicates that participants’ first two and last two lesson plans were significantly different for the integration of STEM subjects and school level. In other words, the provided STEM education course has an effect on participants’ integration of STEM subjects in their lesson plans (p < 0.001) and also has an effect on the school level for which they prepare the lesson plans for (p = 0.005). In other words, as the course progressed teachers prepared STEM lesson plans for higher grades. Which implies that they prepared more composite lesson plans.
Rating the role of each STEM subject
At the end of the course, teachers were asked to rate (the fourth research question), from 5 to 1 where 5 corresponds to very important, the position of the role of each silo in integrated STEM education. A bar graph (Figure 6) with a stacked column was created to indicate this rating. This graph was created based on the responses from 43 teachers.
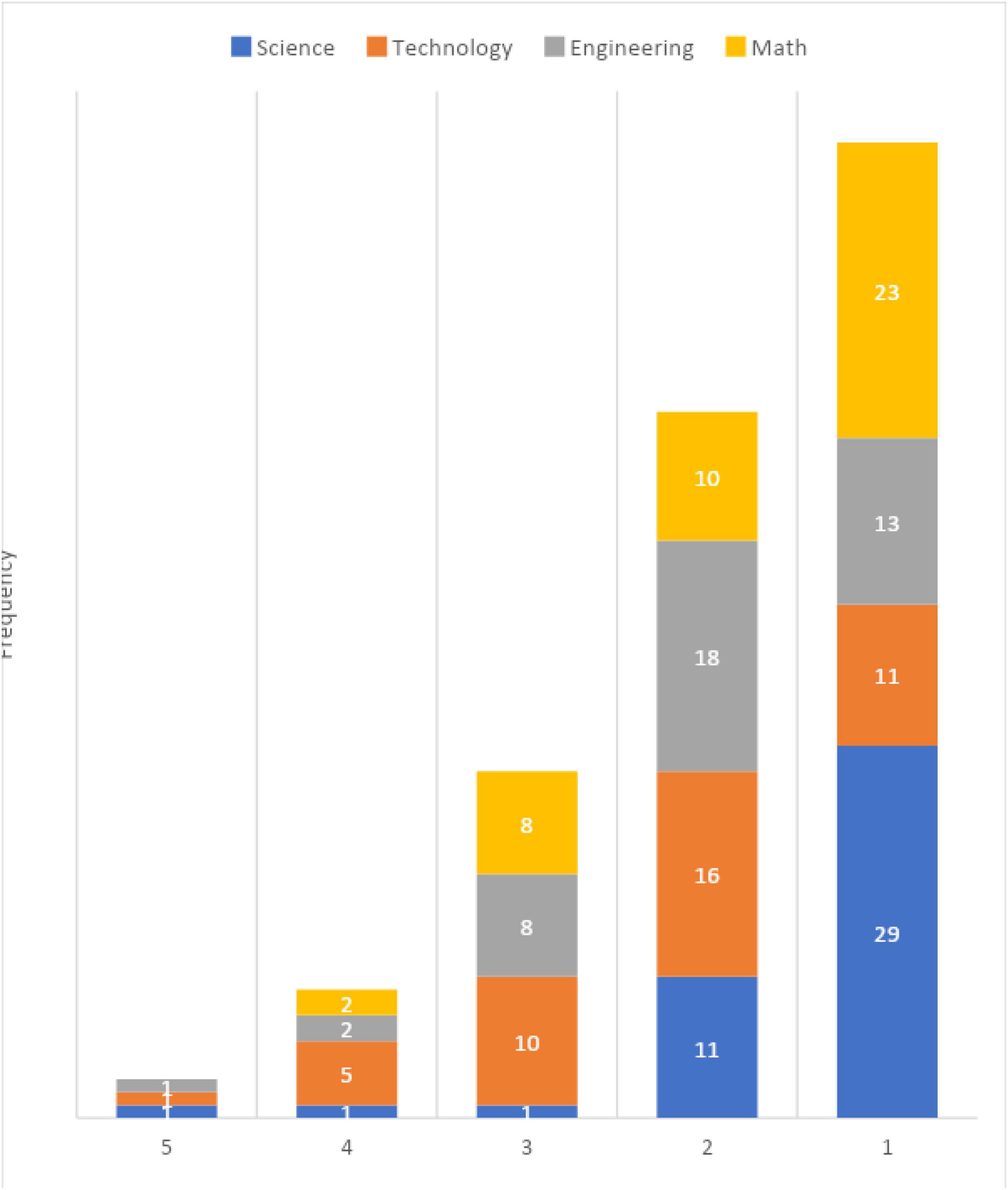
Figure 6. The role of each science, technology, engineering, and mathematics (STEM) subject within integrated STEM education.
Figure 6 demonstrates that teachers rated the role of STEM silos as science, math, engineering, and technology from the most important to the least important. For instance, of the 43 teachers, 29 of them put science in the first place within STEM subjects, and 11, 1, 1, and 1 teachers put science in the second, third and fourth places, respectively. Similarly, math was inserted in the first place by 23 teachers and inserted in second, third and fourth places respectively by 10, 8, 2, and 0 teachers.
A supplementary question to rating the importance of each STEM subject was teachers’ opinions on the role of each STEM subject in STEM education. Below some of the examples from teachers’ views are presented. According to teachers’ views, science is the foundation of STEM education. They stated that science provides the fundamental principles and concepts that students need to understand the natural world and how it works.
Teacher 43: Science is the most important element of STEM. Science asks questions about nature and gives answers in a fact-based and explanatory way. Science is mainly to promote students to design their works according to scientific principles, verify hypotheses based on evidence, and discover and come up with solutions to problems.
Teacher 8: The main role of science in STEM education is to enable students to strengthen their education of scientific literacy and apply scientific knowledge, for example, in the subjects of physics, chemistry, and biological sciences.
In pointing out the role of math, teachers emphasized the effect of math on critical thinking and its role in combining STEM subjects. Here is an example:
Teacher 21: I think the role of mathematics is important in STEM education because we necessarily use mathematical calculations when we make engineering models. Even if we use technology, we cannot work with technology without building a mathematical model.
Teacher 29: Mathematics is a knowledge that can connect other subjects with each other. At the same time, mathematics increases the functional literacy of the student. Students can learn a variety of problem-solving techniques by solving various mathematical problems. We use mathematical concepts in both engineering and technology development. That is why it can be said that mathematics takes the first place in the STEM education system.
Teachers pointed out the role of engineering in designing creative solutions and solving real-life problems. Following is an example which explains the role of technology.
Teacher 44: Engineering plays an important role in STEM education and serves as a bridge between mathematics, technology, and science. Engineering tries to solve problems and applies mathematics, science and technology to design.
Teacher 13: In general, engineering not only increases our ability to solve problems but also helps us to look at the problem in a comprehensive way. In the process of solving the problem, critical thinking leads to increased interest in the work. It opens the way to strengthen inter-disciplinary communication and to look at each other from a new angle.
Finally, teachers pointed out the role of technology in making stuff easier and affordable and saving time. The following example emphases the role of technology in providing good life conditions.
Teacher 2: Nowadays, the use of technology is widespread due to the advanced age. That is, with the help of technology, we make the very difficult work easier. At the same time, the student’s technological literacy will increase. Learning by using technology in life increases the free time of a person. Currently, technology is making the work that cannot be done by humans easier. STEM technology teaches students how to solve complex problems effectively and quickly. With the help of technology, the student’s thinking ability develops, and the student also has the opportunity to invent new techniques. People can use technology to make things easier and easier. That is to say, we can use technology to make complex and difficult things easier, simpler and better to do.
Over 4 weeks, teachers expressed their views regarding the role of one subject in STEM education per week. Since each week they provided responses for a different subject, they praised the role of each subject a lot. However, there was an agreement between writing their views and rating their views. In other words, the role of science was expressed explicitly and expressed a lot in agreement with the stacked graph in Figure 6.
Overall evaluation of the course
At the end of the course, teachers were also asked what they liked and what they did not like in this course (fifth research question). In other words, they were asked to evaluate the course as a whole. Generally, teachers expressed positive feedback on the gains they received from the course.
An example of a totally positive view is as follows (Teacher 32): I really liked this course because this course had a good impact on my experience. As a teacher, I realized that it is necessary to teach children not only the knowledge provided by the program but also to explain the use and benefits of these courses in life. I understood the relationship between mathematics with many directions. I have observed that it allows children to develop their thinking widely.
An example of both negative and positive views together is as follows (Teacher 9): I really like this course. I learn a lot about STEM, and I really excited to use it in future in my classes. Sometimes it was difficult for me to do design challenges. Cause, of the many ideas that I had, I couldn’t realize them because of the tools that required. Many tools I couldn’t afford. That’s my DCs were so simple. I really liked Socrative from previous lessons, it helps us to review and repeat the topic. Thanks for everything. It was an amazing course.
An example of a totally negative view is as follows (Teacher 18): I didn’t like assessment methods. I think it’s too much for one person to make 4 lesson plans and 4 design challenges. And also, every week we wrote a quiz, and also every week we had to read an article and write a reflection. There are too many assignments, I think. Nothing I liked in this course. After this course, I have a negative attitude toward STEM.
Many stated that it was useful and interesting and they will use STEM activities in their future classes. They also expressed that they learnt how to prepare STEM lesson plans. Besides, teachers complained about a high number of weekly assignments and difficulty in finding materials for developing STEM activities along with ideas about STEM activities.
Discussion
This study examined the development and implementation of a STEM course designed to investigate effective instructional approaches and course design elements in an online mathematics class provided to master’s degree students. The course focus and design responds to calls for the development of teacher efficacy in teaching STEM disciplines (Brown, 2012; Barkatsas et al., 2018; Kayan-Fadlelmula et al., 2022).
The online course design was deemed effective when it incorporated several key features, such as well-defined course objectives, appropriate alignment between objectives and assessments, consistent module structure, a range of assignments and learning activities, and a suitable balance between theoretical concepts and practical applications (Yang, 2017). Similarly, we incorporated STEM lesson plans, design challenges, quizzes, participation, reflections on the reading materials, and presentations to increase the effectiveness of the designed course. This is also consistent with what Huang et al. (2022) where they suggest to go beyond assessing teachers’ attitudes and opinions and investigating their participation in STEM events by analyzing their online records of participation and interaction with colleagues, as well as examining the results of their work, such as lesson plans and projects.
Our study demonstrated that involvement in the STEM course substantially elevated the self-efficacy of teachers in teaching and creating STEM courses at the K-12 level. This finding was in parallel with Gardner et al.’s (2019) finding that teachers improved their self-efficacy and made productive changes in their classroom practices after participating in STEM professional development programs.
One of the aims of this course was to have teachers gain competencies in integrating STEM subjects. In the first two lesson plans participants generally integrated two subjects where math was one of the subjects usually. Of the 20 lesson plans we examined only three did not include math for the first and three for the second lesson plans. On the other hand, in the last two lesson plans they generally integrated three subjects where science was the most frequent. For instance, in the third lesson plan, only four did not include science while in the fourth only five did not include science. This finding is consistent with Jong et al. (2021) comment that teachers are not necessarily comfortable creating integrated opportunities for students to experience STEM as intended in a cross-disciplinary way and there is a need for teacher professional development which supports teacher planning in a wholistic way.
The effect of the course on teachers’ STEM knowledge was also assessed. Four simple questions were asked before and after the course. We found that while teachers were familiar with the acronym STEM they were not able to differentiate between STEM, STEM education, and integrated STEM education before the course. Similarly, Teo and Ke (2014) noted that many pre-service teaches had an understanding of their individual discipline, but not the way STEM has been conceptualized and that there should be specialized programs or courses to support inservice teachers in STEM.
One of the most successful implementations in the course that effected teachers’ learning products was that watching what their colleagues have prepared inspired them to produce better works, that is, lesson plans and design challenges. As expected, in their overall course evaluation teachers explicitly mentioned the effect of the video demonstrations by their peers on their own task developments. Designing, video recording, and uploading them to YouTube allowed teachers to develop their engineering design skills as well as their technology skills. Besides, preparing activities allowed a real-world application for the course and added value and meaning throughout the course work for the participants.
In their overall course evaluation teachers generally expressed positive views. According to Karpudewan et al. (2023), research supports the idea that knowledge and perceived effectiveness have a positive impact on STEM teaching.
An important part of the course time was devoted to discussing the reading materials assigned weekly. From the course evaluation data, the teachers indicated that the assignments were useful for their conceptualization of STEM. However, writing reflections on what they learnt from the reading materials did not seem enjoyable for them. This finding corroborates Mzoughi (2015) study who established that the majority of teachers did not find writing reflections from reading materials useful.
The participants in this study were mathematics teachers who were teaching full-time, while enrolled in the online class. Their instruction experience and knowledge of teaching approaches let them give distinctive evaluations and comments associated with efficient teaching strategies and course design characteristics that contributed to their learning.
Although our sample was mathematics teachers and mathematics is a fundamental subject surprisingly, they chose science to be the leading subject among the four silos of STEM. The explicit motive why teachers perceived science as the chief silo is that first, science compromises many subjects taught in schools, that is, physics, chemistry, astronomy, geology and biology, second our experience with the developed STEM lesson plans and activities indicate that it is easier to integrate other subjects into science. Moreover, STEM education is closely related to project-based learning (PBL) (Redmond et al., 2011) and asking a leading question such as what is the effect of microwaves on different organs of the body is the first step in PBL and the leading questions are usually science-based.
The teachers reported that the course was challenging but engaging, and they appreciated the use of real-world examples and hands-on activities to reinforce the course concepts. Despite the overall success of the course, because of heavy assignments, several teachers find it difficult and discouraging. These findings are consistent with the literature that highlights the importance of providing sufficient support and resources for teachers when implementing STEM education (Margot and Kettler, 2019).
The effect of the course is evident that as the course progressed the quality of the tasks prepared by teachers increased. In other words, the lesson plans and design challenges prepared as well as the quality of the reflections written from reading materials improved toward the end of the course. The findings suggest that a well-designed STEM course can improve teachers’ self-efficacy, knowledge and experience providing STEM courses.
Conclusion
This study delivers the findings of a semester-long STEM education course with 52 teachers pursuing master’s degrees in mathematics. The study assessed participants’ self-efficacy, STEM education knowledge, ability to integrate STEM subjects, rating the role of STEM subjects, and overall STEM course experiences. Pre- and post-test surveys and open-ended questions, STEM lesson plans, design challenges, and reading reflections were assessed to locate the effect of the course.
We showed participating in the STEM course significantly increased teachers’ self-efficacy in teaching and designing STEM courses at the K-12 level. Moreover, our results convey the fact that the provided STEM education course has an effect on participants’ STEM knowledge, and integration of STEM subjects in their lesson plans, and also has an effect on the quality of the developed STEM lesson plans. Furthermore, we demonstrated that teachers rated the role of science in STEM as the highest and that of technology as the least important. Finally, participants generally expressed that the STEM education course was useful and interesting and that they will use STEM activities in their future classes.
This study has specific limitations. The context was a single course over a full semester, the findings could be made more applicable to a broader context by including multiple courses and instructors in the analysis. In future research, it would be beneficial to explore the potential benefits of preparing lesson plans and design challenges and how they could motivate teachers in designing STEM lessons in schools.
A second limitation is that teachers video-recorded the design challenges that they prepared and they showed their work remotely. A face-to-face demonstration of the activities would be more effective in terms of understanding and engagement.
A third limitation is that the sample size was insufficient to evaluate nuanced group variations and potential interaction effects, such as those between females and males or advanced and novice participants. Future studies may see whether the suggested course design plays out in a parallel route for such subdivisions.
A fourth limitation of the study pertains to the quasi-experimental design employed, which lacked a control group, a factor that merits attention and acknowledgment. It is imperative to recognize that quasi-experimental designs without control groups inherently present challenges to internal validity. While such designs offer valuable perceptions into real-world settings, their limitations should be considered, as they may compromise the ability to draw causal inferences with a high degree of certainty. To enhance the robustness of future research, it is advisable to explore more intricate designs that incorporate control groups, thereby bolstering the internal validity and strengthening the overall methodological rigor of the investigation.
We suggest STEM teachers and course developers work with or at least discuss with researchers who have proficiency in teaching methods when electing and applying instructional approaches in STEM classes. The results presented in this article have already supported the proposed course design. That is why, we strongly recommend this design for those who seek to teach STEM education as a course at the tertiary level.
Our findings are expected to promote further research and development of STEM courses in the future. This study has found preparing lesson plans and designing STEM activities to be strong evidence of STEM course development. Overall, this study provides valuable insights into the development and implementation of effective STEM courses that can positively impact teachers’ self-efficacy and experience in STEM fields.
Data availability statement
The raw data supporting the conclusions of this article will be made available by the authors, without undue reservation.
Ethics statement
Ethical approval was not required for the studies involving humans because the participants were from corresponding authors’ own class and the data were from instructors’ own course notes, materials, and exams. The studies were conducted in accordance with the local legislation and institutional requirements. The participants provided their written informed consent to participate in this study. Written informed consent was obtained from the individual(s) for the publication of any potentially identifiable images or data included in this article.
Author contributions
NZ: Data curation, Writing – original draft. ZY: Methodology, Writing – original draft. NB: Supervision, Writing – original draft. AA: Conceptualization, Writing – original draft. TB: Formal analysis, Writing – original draft. RM: Writing – original draft, Writing – review & editing.
Funding
The author(s) declare that no financial support was received for the research, authorship, and/or publication of this article.
Conflict of interest
The authors declare that the research was conducted in the absence of any commercial or financial relationships that could be construed as a potential conflict of interest.
Publisher’s note
All claims expressed in this article are solely those of the authors and do not necessarily represent those of their affiliated organizations, or those of the publisher, the editors and the reviewers. Any product that may be evaluated in this article, or claim that may be made by its manufacturer, is not guaranteed or endorsed by the publisher.
Footnotes
References
Abylkassymova, A. E., Kalybekova, Z. A., Zhadrayeva, L. U., Tuyakov, Y. A., and Iliyassova, G. B. (2021). Theoretical foundations of the professional direction of teaching mathematics course in higher Educational Institutions. Glob. Stoch. Anal. 8, 311–322.
Avalos, B. (2011). Teacher professional development in teaching and teacher education over ten years. Teach. Teach. Educ. 27, 10–20. doi: 10.1016/j.tate.2010.08.007
Barkatsas, T., Carr, N., and Cooper, G. (2018). STEM education: An emerging field of inquiry. Leiden: Koninklijke Brill NV.
Beauchamp, I., Klassen, R., Parsons, J., Durksen, T., and Taylor, L. (2014). Exploring the development of teacher efficacy through professional learning experiences. Edmonton, AB: Alberta Teachers’ Association.
Brown, J. (2012). The current status of STEM education research. J. STEM Educ. Innov. Res. 13, 7–11.
Byrd, K. O., Herron, S., Robichaux-Davis, R., Mohn, R. S., and Shelley, K. (2022). Elementary preservice teacher preparation to teach mathematics and science in an integrated STEM framework. J. Res. Sci. Maths. Technol. Educ. 5, 173–193. doi: 10.31756/jrsmte.531
Cooper, G., and Carr, N. (2019). “Primary pre-service teachers’ perceptions of STEM education: Conceptualisations and psychosocial factors,” in STEM education: An emerging field of inquiry, eds T. Barkatsas, N. Carr, and G. Cooper (Leiden: Koninklijke Brill NV), 168–189.
Gardner, K., Glassmeyer, D. M., and Worthy, R. (2019). Impacts of STEM professional development on teachers’ knowledge, self-efficacy, and practice. Front. Educ. 4:26. doi: 10.3389/feduc.2019.00026
Hill, H. C., Lynch, K., Gonzalez, K. E., and Pollard, C. (2020). Professional development that improves STEM outcomes. Kappan 101, 50–56.
Huang, B., Jong, M. S. Y., Tu, Y. F., Hwang, G. J., Chai, C. S., and Jiang, M. Y. C. (2022). Trends and exemplary practices of STEM teacher professional development programs in K-12 contexts: A systematic review of empirical studies. Comput. Educ. 189:104577.
Jong, M. S.-Y., Song, Y., Soloway, E., and Norris, C. (2021). Editorial note: Teacher professional development in STEM education. Educ. Technol. Soc. 24, 81–85.
Karpudewan, M., Krishnan, P., Roth, W. M., and Ali, M. N. (2023). What research says about the relationships between Malaysian teachers’ knowledge, perceived difficulties and self-efficacy, and practicing STEM teaching in schools. Asia-Pac. Educ. Res. 32, 353–365.
Kayan-Fadlelmula, F., Abdellatif, S., Nada, A., and Salman, U. (2022). A systematic review of STEM education research in the GCC countries: Trends, gaps and barriers. Int. J. STEM Educ. 9:2. doi: 10.1186/s40594-021-00319-7
Klassen, R. M., and Chiu, M. M. (2011). Teachers’ job commitment and intention to quit: Impacts of career stage, self-efficacy, job stress, and teaching context. Contemp. Educ. Psychol. 36, 114–129.
Margot, K. C., and Kettler, T. (2019). Teachers’ perception of STEM integration and education: A systematic literature review. Int. J. STEM Educ. 6, 1–16.
Marynowski, R., Wismath, S., Mabin, V., Campmans, M., Suttie, L., and Millard, A. (2021). Engaging K-12 teachers in mathematical puzzles and problems. Delta K 57, 21–28.
Milner-Bolotin, M. (2018). Evidence-based research in STEM teacher education: From theory to practice. Front. Educ. 3:92. doi: 10.3389/feduc.2018.00092
Mutseekwa, C. (2021). STEM practices in science teacher education curriculum: Perspectives from two secondary school teachers’ colleges in Zimbabwe. J. Res. Sci. Maths. Technol. Educ. 4, 75–92. doi: 10.31756/jrsmte.422
Mzoughi, T. (2015). An investigation of student web activity in a “flipped” introductory physics class. Proc. Soc. Behav. Sci. 191, 235–240.
Nadelson, L., Seifert, A., Moll, A., and Coats, B. (2012). i-STEM summer institute: An integrated approach to teacher professional development in STEM. J. STEM Educ. 13, 69–83.
Nielsen, W., Georgiou, H., Howard, S., and Forrester, T. (2019). “Perspectives on STEM education in preservice primary teachers education,” in STEM education: An emerging field of inquiry, eds T. Barkatsas, N. Carr, and G. Cooper (Leiden: Koninklijke Brill NV), 168–189.
Nurbaeva, D. M., Abylkassymova, A. E., Nurmukhamedova, Z. M., and Erzhenbek, B. (2023). The role of educational programs in the development of secondary education (on the example of training mathematics teacher). Mind Brain Educ. 17, 1–6.
Özer, B., Can, T., and Duran, V. (2020). Development of an individual professional development plan proposal that is based on continuing professional development needs of teachers. Eur. Educ. Res. 3, 139–172. doi: 10.31757/euer.334
Palmer, D. (2011). Sources of efficacy information in an in-service program for elementary teachers. Sci. Educ. 1, 1–24.
Rajbanshi, R., Brown, S., Ali Ozer, M., and Delgardo, N. (2020). A case study on professional development: Improving STEM teaching in K – 12 education. Qual. Rep. 25, 4209–4223.
Redmond, A., Thomas, J., High, K., Scott, M., Jordan, P., and Dockers, J. (2011). Enriching science and math through engineering. Sch. Sci. Maths. 111, 399–408.
Richmond, G., Dershimer, R. C., Ferreira, M., Maylone, N., Kubitskey, B., and Meriweather, A. (2017). Developing and sustaining an educative mentoring model of STEM teacher professional development through collaborative partnership. Ment. Tutor. Partner. Learn. 25, 5–26.
Shernoff, D. J., Sinha, S., Bressler, D. M., and Ginsburg, L. (2017). Assessing teacher education and professional development needs for the implementation of integrated approaches to STEM education. Int. J. STEM Educ. 4, 1–16.
Teo, T. W., and Ke, K. J. (2014). Challenges in STEM teaching: Implication for preservice and inservice teacher education program. Theory Into Pract. 53, 18–24.
Yang, D. (2017). Instructional strategies and course design for teaching statistics online: Perspectives from online students. Int. J. STEM Educ. 4, 1–15. doi: 10.1186/s40594-017-0096-x
Appendix
Instrument used in this study is as follows:
A. Sociodemographic data
Type of school you work at is private or public?
City you live in currently?
Grade levels you teach?
B. Self-efficacy in STEM education
We need science education for the future of our country.
We need mathematics education for the future of our country.
We need technology education for the future of our country.
We need engineering education for the future of our country.
I can prepare STEM lesson plans.
I like preparing STEM lesson plans.
I can create STEM activities.
I like creating STEM activities.
I can integrate math into science.
I can integrate math into technology.
I can integrate math into engineering.
I can integrate math into science, technology and engineering.
I like to read about STEM Education.
I am good at projects involving STEM.
I believe there is a need for STEM Education.
I care about developments in STEM Education.
I would like more/advanced courses in STEM Education.
I intend to further develop my abilities in STEM Education.
STEM activities increases creativity.
STEM activities increases critical thinking.
C. Open-ended questions
What is STEM?
What is STEM Education?
What is integrated STEM Education?
What is STEAM?
Keywords: STEM education, course development, self-efficacy, course experiences, Kazakhstan
Citation: Zhumabay N, Yelemessova Z, Balta N, Abylkassymova A, Bakytkazy T and Marynowski R (2024) Designing effective STEM courses: a mixed-methods study of the impact of a STEM education course on teachers’ self-efficacy and course experiences. Front. Educ. 9:1276828. doi: 10.3389/feduc.2024.1276828
Received: 13 August 2023; Accepted: 14 February 2024;
Published: 13 March 2024.
Edited by:
Vanda Santos, University of Aveiro, PortugalReviewed by:
Delfín Ortega-Sánchez, University of Burgos, SpainCucuk Wawan Budiyanto, Sebelas Maret University, Indonesia
Copyright © 2024 Zhumabay, Yelemessova, Balta, Abylkassymova, Bakytkazy and Marynowski. This is an open-access article distributed under the terms of the Creative Commons Attribution License (CC BY). The use, distribution or reproduction in other forums is permitted, provided the original author(s) and the copyright owner(s) are credited and that the original publication in this journal is cited, in accordance with accepted academic practice. No use, distribution or reproduction is permitted which does not comply with these terms.
*Correspondence: Nuri Balta, YmFsdGFudXJpQGdtYWlsLmNvbQ==