- Kwansei Gakuin University, Nishinomiya, Japan
In the field of childcare, the focus has primarily been on executive function (EF) (working memory [WM]), which is a critical factor in daily life. The Wechsler Intelligence Scale for Children’s (WISC-IV) WM Index subtests of the digit span (forward and backward) and letter–number sequencing tasks are used to assess EF (WM). Theoretically, the letter–number sequencing task is more demanding on EF (WM) than the digit span task. Additionally, there is some suggestion of a relationship between EF (WM) and mental abacus (MA) and that MA can easily be performed in daily life. One challenge of supporting EF (WM) is that it is hard to conduct general evaluations and daily activities at home. This study examines the following questions through an empirical study: (1) Can Mental Abacus be used to support executive function in family education? In this study, I measured the cerebral blood flow of one child at home using the HOT-2000 for the tasks of MA and the WISC-IV. As a result, I was able to show that for that child, MA could have an effect on EF(WM). Although this is only one example, for parents, it has been important in showing that it is not impossible in the field of home education where there are many different kinds of children. The findings provide a new perspective regarding evaluating EF (WM) in cognitive psychology and neuropsychology and are valuable in breaking through previous research.
1. Introduction
Executive function (EF) is goal-directed and involves controlling thoughts, actions, and emotions (Miyake et al., 2000; Moriguchi, 2015). EF usually refers to working memory (WM), cognitive flexibility, shifting, and inhibition (Zelazo and Carlson, 2012; Diamond, 2013). The neural basis of EF has been noted as the prefrontal cortex (Stuss and Alexander, 2000; Banich, 2009; Duncan, 2010, 2013; Burgess and Stuss, 2017). Additionally, EF significantly impacts intellectual development and various everyday situations (Dempster, 1992; Harnishfeger and Bjorklund, 1993; Bjorklund and Harnishfeger, 1995; Bjorklund and Kipp, 2002; Hofmann et al., 2012; Miyake and Friedman, 2012).
A concept related to EF is WM. WM is a temporary storage system under attentional control that supports complex thinking abilities (Baddeley, 2012). WM is crucial for planning, problem-solving, and acquiring new skills in general (Miyake and Shah, 1999; Phye and Pickering, 2006). Therefore, it can be considered the basis of new intelligence that opens new ways of life (Alloway and Alloway, 2013).
Regarding the relationship between EF and WM, there are two positions: (1) EF is a subset of WM (Hofmann et al., 2012) and (2) WM is a subset of EF (Zelazo and Carlson, 2012; Diamond, 2013). Because these are insufficient explanations, some studies have recently indicated that EF and WM are complementary (Yuzawa and Yuzawa, 2014). Nevertheless, in the end, supporting the development of WM will support EF. Therefore, the general concept of EF is labeled as EF, and the general concept of WM is labeled as WM, WM, which is largely involved in EF, is labeled as EF (WM).
The Wisconsin Card Sorting Test (Berg, 1948), the Stroop Task Test (Stroop, 1935), the disentangling dimensions in the dimensional change card sorting task (Zelazo, 2006), and the Marshmallow Test (Mischel et al., 1972) are examples of behavioral tasks with EF. In contrast, WM tasks include the N-back task (Kirchner, 1958) and the Wechsler Intelligence Scale for Children-IV’s (WISC-IV) (Wechsler, 2003) sub-tasks (digit span (forward and backward digit span) and letter–number sequencing tasks). Regarding WISC-IV’s Working Memory Index (WMI) subtests, from the perspective of WM, the forward digit span task responds to the phonological loop, and the backward digit span task responds to the central executive (Era et al., 2006). Besides this, the letter–number sequencing task responds strongly to visuospatial WM and processing speed (Crowe, 2000). Therefore, the letter–number sequencing task is more demanding on WM than the digit span task. Previous studies have shown that the WM is closely related to mathematics (LeFevre et al., 2005).
Further, many scholars have argued that the study of mathematics has a huge impact on the academic performance of children (Freeman, 2014). However, does mental abacus (MA) have the same effect? MA is used to perform mental arithmetic with the mental image of an abacus. Notably, MA differs from ordinary mental arithmetic. For example, “Mitorizan” of MA is a type of addition and subtraction in the form of written arithmetic. Usually, these calculations are performed one digit at a time; however, in the MA calculation method, all digits are calculated simultaneously in order. In other words, we can imagine the movement of the beads of an abacus as we proceed. The learning effect of this method is not limited to mathematics; it can also enhance WM (Wang, 2020). MA is based on a visuospatial strategy that loads the process of storing and manipulating visuospatial WMs (Tanaka et al., 2002; Hanakawa et al., 2003). In addition, Dumontheil and Klingberg (2012) observed a particularly strong correlation between visuospatial working memory and mathematical ability. Long-term MA training improves math skills and exerts a positive impact on visuospatial WM (Wang et al., 2019). The current study suggests that MA and WM are closely related to increased academic achievement in mathematics. Therefore, considering that MA and letter–number sequencing tasks involve visuospatial WM processes, people who have received MA training may show similar brain activity when performing MA and letter–number sequencing tasks.
Further, many studies have already suggested that learning MA may be an effective way to support cognitive development (Stigler, 1984; Hanakawa et al., 2003; Tanaka et al., 2012; Yao et al., 2015; Zhou et al., 2019). MA, which was not originally designed to support WM, has been suggested to have a metastatic effect because it includes multiple components of WM (Wang, 2020).
There are several indicators of the relationship between EF and the prefrontal cortex. In other words, we can infer that the part of WM components related to EF can be measured from the brain activity in the prefrontal cortex. First, it has been indicated that EF is closely related to prefrontal cortex activity (Houdé and Borst, 2014), even during early childhood (Moriguchi, 2015). In contrast, WM is related to the prefrontal cortex (Rämä et al., 2001; Gruber and von Cramon, 2003; Alloway and Alloway, 2013). Studies have proposed that WM is related to the prefrontal cortex even in early childhood (Tsujimoto et al., 2004). Additionally, the prefrontal cortex has been implicated in MA (Tanida et al., 2004). Evidence suggests that mental abacus is associated with the activation of the prefrontal cortex in children (Du et al., 2013). In summary, these findings indicate that EF, WM, and MA are significantly related to brain activity in the prefrontal cortex.
Many of these studies have focused on the activation of cerebral blood flow. If the load is excessive or small, then the activation is small. If a task is moderately difficult, then activation is large (Watanabe, 2008). Brain activity can be visualized through the imaging of non-attacking brain functions. For example, magnetic resonance imaging (MRI), positron emission tomography, and near-infrared spectroscopy (NIRS) have been used to measure cerebral blood flow. However, functional near-infrared spectroscopy (fNIRS) is more valuable in the field because of its affordability and functionality (Shimizu, 2010). In addition, fNIRS is considered usable even in early childhood (Moriguchi, 2015). This technology has rapidly gained popularity in recent decades because of its safety, portability, and flexibility; further, it can be used by everyone—from newborns to elderly people—and can be operated inside and outside the laboratory (Pinti et al., 2015).
EF is often measured by behavioral outcomes alone. However, in recent years, brain activity has also been widely used, and efforts are actively made to improve the research of measured by behavioral outcomes (Karbach and Kray, 2009; Buschkuehl and Jaeggi, 2010; Klingberg, 2010; Best, 2012; Espinet et al., 2013). However, it is difficult to generalize and make practicable the results of these experiments because they were conducted at the laboratory level, and the results have many individual differences (Alloway and Alloway, 2013).
Support for children’s EF(WM) is important, and it would be a wishful thinking for parents if they could do it at home. If I can demonstrate that EF (WM) can be measured through brain activity—that is, if it is consistent with the generality of behavioral indices—then the developmental level within an individual is measurable and can be compared with other activities. Additionally, if this process can easily be conducted outside of the laboratory (at home), then it will be of great value in supporting children. In this respect, there is no problem in the direction of my research, since there is a recent case study on abacus by Watanabe (2021).
Against this background, this study examined the following research question possibilities through an empirical study. (1) Can Mental Abacus be used to support executive function in family education?
Specifically, the following processes (1) through (3) were tested through a pilot case study. (1) EF (WM) of the WISC-IV (WMI) test measure by brain activity in the prefrontal cortex (the more the load is moderately applied to WM, the more it is activated) (Alloway and Alloway, 2013). (2) To compare the activation level of EF (WM) in the prefrontal cortex of WMI with that of MA (presuming that brain activity is performed at a high level). (3) Both (1) and (2) are practiced at home using HOT-2000 (measuring the EF (WM) level of an individual is possible outside the laboratory). The HOT-2000 is a 2ch (two measurement points) wearable brain activity measurement device that can monitor changes in blood flow related to brain activity using near-infrared light. Changes in brain activity cause changes in brain hemodynamics, and blood flow increases near the area where the brain is activated. In HOT-2000, two sensor blocks are placed at the part of the brain that targets the prefrontal cortex, which enables measuring brain activity in the prefrontal cortex.
2. Materials and methods
2.1. Study design
To do the empirical study, a comparison was made based on the measurement of cerebral blood flow in the prefrontal cortex during the WISC- IV (WMI) tasks: the digit span (forward and backward) and letter–number sequencing tasks and MA. Each task was done as one set on the same day. This was done for nine sets (9 days). Brain activity was measured using fNIRS during each WISC- IV (WMI) task and while performing MA. A two-channel fNIRS device was used to explore the possibility of implementing it in homes. The measured value was the total left and right Hb (hemoglobin) (mMmm). A two-channel fNIRS device, HOT-2000 (NeU, Japan, price: 198,000 yen) (Figure 1), was used in this study. For the actual measurements, a wavelength light (approximately 800 nm) was used. The detectors were placed approximately 1 cm (to obtain blood flow information from the scalp to the shallow scalp) and 3 cm (to obtain blood flow information from the scalp to the shallow scalp) from the light irradiation. Brain activation was estimated based on velocity. Total Hb change (left and right) was present at each brain activity index (spike noise was removed; the signal at an SD distance of 3 cm minus the signal at an SD distance of 1 cm was subtracted at a constant rate). Total hemoglobin change (left and right) was adopted as the measured value. The baseline correction was set to 0 at the beginning, and the average brain activity during the task was examined {[Σ(f(x)-min f(x))/total number of milliseconds], x: time, and f(x): total hemoglobin}. Total hemoglobin was acquired every millisecond.
2.2. Target
The subject of the study was a 9-year-old 4th-grader who attended a typical public elementary school. Her grades were average. She needed no special assistance. She began attending an abacus school twice a week in the first grade and continued for about 3 years. She worked on practice problems for third-level MA and fourth-level abacus, an average level of achievement.
To reduce bias, a subject was chosen who was familiar with this type of experiment and brain activity survey, including participating in the measurements of cerebral blood flow. Furthermore, she had already built rapport with the researcher before the study.
An individual should practice MA at an appropriate level to allow the brain activity load to be moderate. If this stipulation is implemented consistently, no matter which child is studied, we can expect to see similar results, although the concrete issues may vary from individual to individual.
The study was presented (and explained) to the parents both verbally and in writing. I obtained informed consent from all participants. Additionally, I submitted a report to the Committee for Regulations for Behavioral Research with Human Participants at the author’s university. The experiment took place at the subject’s home—in her study room.
2.2.1. Ethical considerations
This study was performed per the Code of Ethics of the World Medical Association (Declaration of Helsinki). It had the approval of the Kwansei Gakuin University Committee for Regulations for Behavioral Research with Human Participants (Approval Numbers: 2020–06; Approval Dates: June 12, 2020). I received written informed consent for experimentation with human subjects. The privacy rights of all the participants were safeguarded.
2.3. Tasks
2.3.1. WISC-IV-WMI task
(1) Digit span task: This task includes forward digit span task and backward digit span task. Forward digit span task: There are 16 questions wherein the examiner read a set of numbers (number series) to the subject, and the subject respond verbally in order. There are eight big questions and two sub-questions within each big question. The first question has two numbers; for example, 2 and 8. Each sub-question has the same number of numbers. The number of numbers increases to 2, 3, 9, etc., in the big questions. The task is stopped when all two sub-questions in a big question are wrong. The question example is “Q1-1 (2, 8), Q1-2 (4, 7), Q2-1 (2, 4, 8), Q2-2 (8, 1, 3), … Q8-2 (4, 1, 6, 9, 2, 3, 7, 5, 8)”. Backward digit span task: There are 16 questions wherein the examiner read a set of numbers (number series) to the subject, and the subject respond verbally in reverse order. There are eight big questions and two sub-questions within each big question. The first question has two numbers, e.g., 3 and 1. Each sub-question has the same number of numbers. In the big questions, question 1 has 2 numbers and question 2 also has 2 numbers, but then the number of numbers increases each big question. The task is stopped when all two sub-questions in a big question are wrong. The question example is “Q1-1 (3, 1), Q1-2 (1, 7), Q2-1 (4, 8), Q2-2 (8, 1), Q3-1 (5, 6, 4), … Q8-2 (4, 1, 6, 9, 2, 3, 7, 5)”.
(2) Letter–number sequencing task: The examiner read a set of numbers and hiragana alternately to the subject, and the subject sort the numbers in ascending order and the letters in Japanese alphabetical order. The subject respond verbally in that order. There are 30 questions in total. There are 10 big questions and 3 sub-question within each big question. The first big question consists of one number and one hiragana, for example, “あ, 3.” Each sub-question has the same number of numbers and hiragana. The big first and second questions have one number and one hiragana, and the big third, fourth and fifth questions have a total three numbers and hiragana. Big questions 6 and 7 have a total of four numbers and hiragana, question 8 has a total of five numbers and hiragana, big question 9 has a total of six numbers and hiragana, and big question 10 has a total of seven numbers and hiragana. If all sub-questions in a big question cannot be respond, the task will be stopped. The example question is “Q1-1 (3, あ), Q1-2 (い, 7), Q1-3 (3, え), Q2-1 (う, 4, 5), … Q10-3 (7, う, 8, え, 3, あ, 5)”.
This task was performed according to the procedures outlined by Wechsler (2010). In the WMI task, the ordering of digit span task and letter–number sequencing task was performed in this order. Following the manual, the task was completed when the “Cancel” condition was met. In other words, if all the sub-questions were wrong, the task was terminated. Therefore, the time spent on task implementation also differed. Brain activity was measured from the beginning to the end of each task.
2.3.2. Mental abacus (MA)
The questions are based on the Japan Chamber of Commerce and Industry’s MA Test Level 3 textbook. The reason is the subject had already passed Level 4 of the Japan Chamber of Commerce and Industry’s MA Test and was currently practicing for Level 3. The content is to calculate 3-digit +3-digit questions. The number of questions are unlimited. The subject calculates as many questions as she can within the time limit. The example question is “428 + 169, 270 + 563, 619 + 405, 356 + 148, and 185 + 369”.
Specifically, the subject spent 5 min working on 3-digit +3-digit addition. Brain activity was measured from the beginning to the end of the task.
The above tasks were ordered as follows: MA, forward digit span and backward digit span task, and letter–number sequencing task. Between each task, breaks of at least 30 s were taken.
2.4. Calculations
The hypotheses were as follows: (1) Can Mental Abacus be used to support executive function in family education?
This study examined the possibility of the hypothesis. First, it is important for parents that they can implementing the method at home rather than in a laboratory. Next, there are considerable individual differences of EF(WM). Therefore it is valuable to show the possibility even if it’s just one person. Therefore, I used a pilot case study of one child. For validation, I measured the brain activity in the prefrontal cortex using fNIRS for each task. I also measured the values of the brain activity (total Hb in the left and right prefrontal cortex) for each task. Then, the average value for each task was calculated. The means were compared by Cohen’s d and Hedges’ g. In addition, a test of difference of means (t-test) and the Wilcoxon signed-rank test were used to test for significant differences. These values were calculated using IBM SPSS Statistics ver. 27.0.1.0.
3. Results
The subject performed tasks for 5 min during all sessions for the MA task. Further, the WISC-IV-WMI task was performed according to a general manual. In the WMI task, the ordering of digit span task and letter–number sequencing task was performed in this order. Following the manual, the task was completed when the subject met the “Stop” condition. In other words, the subject performed these tasks up to the “Stop” condition during all the sessions.
The subject performed each task nine times. The results are as follows.
Numerical and average values for each MA, forward digit span task, backward digit span task, and letter–number sequencing task are in Table 1. For the left lateral brain activity, the mean values were, in ascending order, the digit span task, MA, and the letter–number sequencing task. For the right lateral brain activity, the mean values were, in ascending order, the digit span task, the letter–number sequencing task, and MA. In other words, the degree of difficulty increases in this order. It can also be seen that the average values of MA and the letter–number sequencing task are similar.
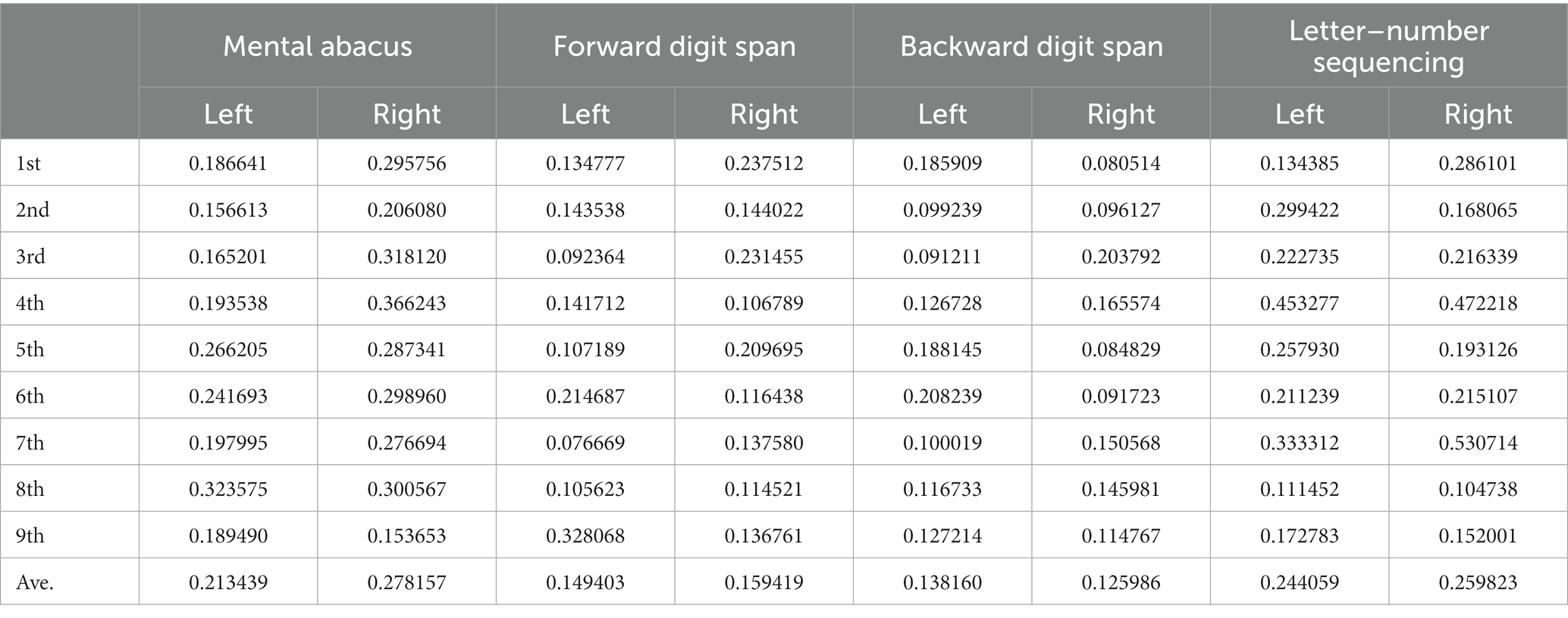
Table 1. Numerical values and average values for each MA, forward digit span, backward digit span, and letter–number sequencing tasks.
Table 2 illustrates the results of the Wilcoxon signed-rank test and t-test for the left and right brain activities in each task. First, the Wilcoxon signed-rank test indicated a significant difference between MA and the forward digit span and the backward digit span tasks. However, there was no significant difference between the letter–number sequencing task and MA. There was also no significant difference between the forward digit span task and the backward digit span task. Furthermore, there was a significant difference between the letter–number sequencing task and the backward digit span task. The t-test pointed to a significant difference between MA and the forward digit span and the backward digit span tasks. However, no significant difference was observed between the letter–number sequencing task and MA and between the forward digit span task and the backward digit span task. Alternatively, the study noted a significant difference between the letter–number sequencing task and the forward digit span task and between the letter–number sequencing task and the backward digit span task. These findings denote that there is a significant difference between MA and the forward digit span and backward digit span tasks.
Table 3 shows the effect size (Cohen’s d and Hedges’ g) corresponding to the differences between the mean values for the left and right brain activities in each task.
The effect size of Cohen’s d and Hedges’ g was considerable in the relationship between MA and the forward digit span task and the relationship between MA and the backward digit span task. This means that there is a difference between MA and the forward digit span and backward digit span tasks.
4. Discussion
4.1. Value of the research— an empirical study
To support children, it is critical to support their EF (WM). However, this kind of support is difficult to offer and is still under development. If possible, it is desirable to have a general-purpose approach that can easily be applied in homes rather than in the laboratory. Brain science research development has great potential to contribute to this.
In this study, the following possibilities were investigated through an empirical study. (1) Can Mental Abacus be used to support executive function in family education? Specifically, the following processes (1) through (3) were tested through a pilot case study. (1) EF (WM) of the WISC-IV (WMI) test measure by brain activity in the prefrontal cortex (the more the load is moderately applied to WM, the more it is activated) (Alloway and Alloway, 2013). (2) To compare the activation level of EF (WM) in the prefrontal cortex of WMI with that of MA (presuming that brain activity is performed at a high level). (3) Both (1) and (2) are practiced at home using HOT-2000 (measuring the EF (WM) level of an individual is possible outside the laboratory).
The brain measurements revealed the following. First, it was possible to measure and evaluate the brain activity of EF (WM) even at home using HOT-2000. Moreover, it should be noted that there is a significant difference between the MA and the digit span task, and a significant difference between the digit span task and the letter–number sequencing task. This result can be inferred from the point-out that the letter-number sequencing task is more demanding on WM than the digit span tasks. It should also be noted that the means of the MA and the letter-number sequencing task were similar. This result can be inferred from the point-out that the letter–number sequencing task is also strongly responsive to visuospatial WM and processing speed, and that MA is based on visuospatial WM.
Considering these points, it is clear that MA is more valuable than training for the digit span task, and although similar to training for mere letter–number sequencing task, MA itself has a load value of being able to compute quickly and accurately, thus contributing to mathematics, and is of high educational value. However, in Japan, only a part of this method is used in school education; therefore, it will take some effort to popularize it.
Therefore this empirical study makes the following suggestions: It’s possible that Mental Abacus can be used to support executive function in family education.
And if I may add that this study also provides a new out-of-laboratory procedure for understanding EF (WM) among children and proposes a new line of research using technology to increase knowledge about the mechanism of mathematical learning among school-age children.
This is only one case study. However, at home where there is a wide variety of children, even if I show generalities, it cannot be said to be complete because it cannot be applied to everyone. Even if the effect of such support may be limited, it is very important to show some of the possibilities of support. Therefore, it can be said that even a case study is valuable in that it is able to show that there is nothing that cannot be done.
4.2. Limitations
One can assume that many researchers will point out the difficulty of introducing MA. In this regard, first, in Japan, MA education is officially handled in school education. However, it only takes a few hours (about 2–3 school hours each at the 3rd and 4th grades). Therefore, no effective results have been found. In Japan, however, the hurdle to adopting MA is low because it is not entirely zero-based (many adults and children, even in elementary school, learn for several hours).
Even if no difference was found between the MA and letter-number sequencing task, nor any differences in brain activity, MA is benefit over letter-number sequencing task for mathematics and for family education. The reason why in mathematics problem solving, the capacity of working memory is limited, so it is important to have that space available, because if calculations can be done easily by MA, then the working memory can be used for other solutions.
Additionally, it is presumed that measuring brain activity does not necessarily measure all activation related to EF (WM) because of the measurement of two channels in the prefrontal cortex. In this regard, first, the task used is the one wherein activation of EF (WM) is recognized by behavioral indices. Furthermore, there is a theoretical difference in the load of the tasks and the results of brain activity measurement. From these results, I can conclude that I was unable to measure all of the brain activity in EF (WM); however, I did accurately measure the part related to prefrontal cortex activity. Our findings align with those of previous studies showing that EF (WM) relates to prefrontal cortex activity. In other words, the two-channel measurement of brain activity in the prefrontal cortex is valuable.
Generalizations from a case study or a small set cannot be relied upon for broad conclusions. Therefore, it is critical to show whether EF (WM) can be measured as a first step because, after all, there are individual differences in EF (WM). The study subjects showed a significant difference between the digit span task and the letter–number task. Therefore, EF (WM) can be treated as an index, and by comparing it with MA, it suggests that MA has the same level of value as the letter–number sequencing task. In the case of other subjects, it is possible to determine the value of the support content by selecting tasks with significant differences in other behavioral indices and comparing the support content with the target.
This case study is relevant because it shows a strategy for supporting this process (I determined the characteristics of the activation of the brain activity from the task with behavioral indicators. Based on this criterion, the value of the task of the assumed support content is judged. If the task has a high value, it can be identified as support content). It is possible to flexibly and accurately set the support appropriate for the target child simply and easily in a manner suitable for individual differences. Of course, it is necessary to conduct several experiments to show such a pattern. However, in the end, as there are individual differences in the support for children, it is valuable to show the “measures” first.
Regarding the issues that still need to be addressed, it is necessary to show the generality by further expanding the target population. And more data need to be collected to obtain a general trend.
4.3. Contributions
In the context of cognitive psychology and neuropsychology, this study evaluates EF (WM) from a new perspective. By matching the psychological theories of the digit span task and the letter–number sequencing task with brain science and its application (comparison with MA), further research will be promoted. Additionally, in terms of supporting children, this study proposes new support for EF (WM), such as support that can easily be provided at home, which is valuable for the development of future support methods.
Data availability statement
The data that support the findings of this study are available on request from the author. The data are not publicly available due to privacy or ethical restrictions.
Ethics statement
The studies involving human participants were reviewed and approved by Kwansei Gakuin University Committee for Regulations for Behavioral Research with Human Participants (Approval Number: 2020-06; Approval Date: June 12, 2020). Written informed consent to participate in this study was provided by the participants’ legal guardian/next of kin.
Author contributions
The author confirms being the sole contributor of this work and has approved it for publication.
Funding
This work was supported by JSPS KAKENHI Grant Number 22K02535.
Conflict of interest
The author declares that the research was conducted in the absence of any commercial or financial relationships that could be construed as a potential conflict of interest.
Publisher’s note
All claims expressed in this article are solely those of the authors and do not necessarily represent those of their affiliated organizations, or those of the publisher, the editors and the reviewers. Any product that may be evaluated in this article, or claim that may be made by its manufacturer, is not guaranteed or endorsed by the publisher.
References
Alloway, T. P., and Alloway, R. G. (2013). Working Memory: The Connected Intelligence. New York: Psychology Press.
Baddeley, A. (2012). Working memory: theories, models, and controversies. Annu. Rev. Psychol. 63, 1–29. doi: 10.1146/annurev-psych-120710-100422
Banich, M. T. (2009). Executive function: the search for an integrated account. Curr. Dir. Psychol. Sci. 18, 89–94. doi: 10.1111/j.1467-8721.2009.01615.x
Berg, E. A. (1948). A simple objective technique for measuring flexibility in thinking. J. Gen. Psychol. 39, 15–22. doi: 10.1080/00221309.1948.9918159
Best, J. R. (2012). Exergaming immediately enhances children’s executive function. Dev. Psychol. 48, 1501–1510. doi: 10.1037/a0026648
Bjorklund, D. F., and Harnishfeger, K. K. (1995). “The evolution of inhibition mechanisms and their role in human cognition and behavior,” in Interference and Inhibition in Cognition, eds. F. N. Dempster and C. J. Brainerd (San Diego, CA: Academic Press), 141–173.
Bjorklund, D. F., and Kipp, K. (2002). “Social cognition, inhibition, and theory of mind: the evolution of human intelligence” in The Evolution of Intelligence. eds. R. J. Sternberg and J. C. Kaufman (Mahwah, NJ: Lawrence Erlbaum Associates Publishers), 27–54.
Burgess, P. W., and Stuss, D. T. (2017). Fifty years of prefrontal cortex research: impact on assessment. J. Int. Neuropsychol. Soc. 23, 755–767. doi: 10.1017/S1355617717000704
Buschkuehl, M., and Jaeggi, S. M. (2010). Improving intelligence: a literature review. Swiss Med. Wkly. 140, 266–272. doi: 10.4414/smw.2010.12852
Crowe, S. F. (2000). Does the letter number sequencing task measure anything more than digit span? Assessment 7, 113–117. doi: 10.1177/107319110000700202
Dempster, F. N. (1992). The rise and fall of the inhibitory mechanism: toward a unified theory of cognitive development and aging. Dev. Rev. 12, 45–75. doi: 10.1016/0273-2297(92)90003-K
Diamond, A. (2013). Executive functions. Annu. Rev. Psychol. 64, 135–168. doi: 10.1146/annurev-psych-113011-143750
Du, F., Chen, F., Li, Y., Hu, Y., Tian, M., and Zhang, H. (2013). Abacus training modulates the neural correlates of exact and approximate calculations in Chinese children: an fMRI study. Biomed. Res. Int. 2013, 1–12. doi: 10.1155/2013/694075
Dumontheil, I., and Klingberg, T. (2012). Brain activity during a visuospatial working memory task predicts arithmetical performance 2 years later. Cereb. Cortex 22, 1078–1085. doi: 10.1093/cercor/bhr175
Duncan, J. (2010). The multiple-demand (MD) system of the primate brain: mental programs for intelligent behaviour. Trends Cogn. Sci. 14, 172–179. doi: 10.1016/j.tics.2010.01.004
Duncan, J. (2013). The structure of cognition: attentional episodes in mind and brain. Neuron 80, 35–50. doi: 10.1016/j.neuron.2013.09.015
Era, S., Seto, H., and Ohba, S. (2006). Relationships among arithmetic, forward digit span, and backward digit span subtests of the Wechsler intelligence scales (WAIS-R and WISC-III). Jpn. J. Support Syst. Dev. Disabil. 5, 1–6.
Espinet, S. D., Anderson, J. E., and Zelazo, P. D. (2013). Reflection training improves executive function in preschool-age children: behavioral and neural effects. Dev. Cogn. Neurosci. 4, 3–15. doi: 10.1016/j.dcn.2012.11.009
Freeman, N. (2014). “Does the Japanese abacus improve underachieving children’s performance in mathematics?” in Proceedings of the British Society for Research into learning mathematics. ed. G. Adams (Leicester: BSRLM), 13–18.
Gruber, O., and von Cramon, D. Y. (2003). The functional neuroanatomy of human working memory revisited: evidence from 3-T fMRI studies using classical domain-specific interference tasks. NeuroImage 19, 797–809. doi: 10.1016/s1053-8119(03)00089-2
Hanakawa, T., Honda, M., Okada, T., Fukuyama, H., and Shibasaki, H. (2003). Neural correlates underlying mental calculation in abacus experts: a functional magnetic resonance imaging study. NeuroImage 19, 296–307. doi: 10.1016/s1053-8119(03)00050-8
Harnishfeger, K. K., and Bjorklund, D. F. (1993). “The ontogeny of inhibition mechanisms: a renewed approach to cognitive development” in Emerging Themes in Cognitive Development. eds. M. L. Howe and R. Pasnak (New York: Springer), 28–49. doi: 10.1007/978-1-4613-9220-0_2
Hofmann, W., Schmeichel, B. J., and Baddeley, A. D. (2012). Executive functions and self-regulation. Trends Cogn. Sci. 16, 174–180. doi: 10.1016/j.tics.2012.01.006
Houdé, O., and Borst, G. (2014). Measuring inhibitory control in children and adults: brain imaging and mental chronometry. Front. Psychol. 5:616. doi: 10.3389/fpsyg.2014.00616
Karbach, J., and Kray, J. (2009). How useful is executive control training? Age differences in near and far transfer of task-switching training. Dev. Sci. 12, 978–990. doi: 10.1111/j.1467-7687.2009.00846.x
Kirchner, W. K. (1958). Age differences in short-term retention of rapidly changing information. J. Exp. Psychol. 55, 352–358. doi: 10.1037/h0043688
Klingberg, T. (2010). Training and plasticity of working memory. Trends Cogn. Sci. 14, 317–324. doi: 10.1016/j.tics.2010.05.002
LeFevre, J. A., DeStefano, D., Coleman, B., and Shanahan, T. (2005). “Mathematical cognition and working memory” in Handbook of Mathematical Cognition ed. J. I. D. Campbell (New York: Psychology Press), 361–377.
Mischel, W., Ebbesen, E. B., and Zeiss, A. R. (1972). Cognitive and attentional mechanisms in delay of gratification. J. Pers. Soc. Psychol. 21, 204–218. doi: 10.1037/h0032198
Miyake, A., and Friedman, N. P. (2012). The nature and organization of individual differences in executive functions: four general conclusions. Curr. Dir. Psychol. Sci. 21, 8–14. doi: 10.1177/0963721411429458
Miyake, A., Friedman, N. P., Emerson, M. J., Witzki, A. H., Howerter, A., and Wager, T. D. (2000). The unity and diversity of executive functions and their contributions to complex “frontal lobe” tasks: a latent variable analysis. Cogn. Psychol. 41, 49–100. doi: 10.1006/cogp.1999.0734
Miyake, A., and Shah, P. (Eds.). (1999). Models of Working Memory: Mechanisms of Active Maintenance and Executive Control. New York: Cambridge University Press.
Moriguchi, Y. (2015). Early development of executive function, its neural mechanism and interventions. Jpn. Psychol. Rev. 58, 77–88.
Pinti, P., Aichelburg, C., Lind, F., Power, S., Swingler, E., Merla, A., et al. (2015). Using fiberless, wearable fNIRS to monitor brain activity in real-world cognitive tasks. J. Vis. Exp. 106:e53336. doi: 10.3791/53336
Rämä, P., Sala, J. B., Gillen, J. S., Pekar, J. J., and Courtney, S. M. (2001). Dissociation of the neural systems for working memory maintenance of verbal and nonspatial visual information. Cogn. Affect. Behav. Neurosci. 1, 161–171. doi: 10.3758/CABN.1.2.161
Shimizu, K. (2010). Non-invasive brain functional imaging. J. ITE 64, 794–798. doi: 10.3169/itej.64.794
Stigler, J. W. (1984). “Mental abacus”: the effect of abacus training on chinese children’s mental calculation. Cogn. Psychol. 16, 145–176. doi: 10.1016/0010-0285(84)90006-9
Stroop, J. R. (1935). Studies of interference in serial verbal reactions. J. Exp. Psychol. 18, 643–662. doi: 10.1037/h0054651
Stuss, D. T., and Alexander, M. P. (2000). Executive functions and the frontal lobes: a conceptual view. Psychol. Res. 63, 289–298. doi: 10.1007/s004269900007
Tanaka, S., Michimata, C., Kaminaga, T., Honda, M., and Sadato, N. (2002). Superior digit memory of abacus experts: an event-related functional MRI study. Neuroreport 13, 2187–2191. doi: 10.1097/00001756-200212030-00005
Tanaka, S., Seki, K., Hanakawa, T., Harada, M., Sugawara, S. K., Sadato, N., et al. (2012). Abacus in the brain: a longitudinal functional MRI study of a skilled abacus user with a right hemispheric lesion. Front. Psychol. 3:315. doi: 10.3389/fpsyg.2012.00315
Tanida, M., Sakatani, K., Takano, R., and Tagai, K. (2004). Relation between asymmetry of prefrontal cortex activities and the autonomic nervous system during a mental arithmetic task: near infrared spectroscopy study. Neurosci. Lett. 369, 69–74. doi: 10.1016/j.neulet.2004.07.076
Tsujimoto, S., Yamamoto, T., Kawaguchi, H., Koizumi, H., and Sawaguchi, T. (2004). Prefrontal cortical activation associated with working memory in adults and preschool children: an event-related optical topography study. Cereb. Cortex 14, 703–712. doi: 10.1093/cercor/bhh030
Wang, C. (2020). A review of the effects of abacus training on cognitive functions and neural systems in humans. Front. Neurosci. 14:913. doi: 10.3389/fnins.2020.00913
Wang, C., Xu, T., Geng, F., Hu, Y., Wang, Y., Liu, H., et al. (2019). Training on abacus-based mental calculation enhances visuospatial working memory in children. J. Neurosci. 39, 6439–6448. doi: 10.1523/JNEUROSCI.3195-18.2019
Watanabe, M. (2008). “Kodo no Ninchikagaku [Cognitive Science of Behavior]” in Ninshiki to Kodo no Nokagaku [Brain Science of Cognition and Behavior]. ed. K. Tanaka (Tokyo: University of Tokyo Press), 203–263.
Watanabe, N. (2021). Easy abacus calculation in early childhood to support executive function: an educational pilot case study comparing brain activity in the prefrontal cortex. Front. Educ. 6:757588. doi: 10.3389/feduc.2021.757588
Wechsler, D. (2003). The Wechsler Intelligence Scale for Children (4th). San Antonio, TX: Harcourt Assessment.
Wechsler, D. (2010). (Translated and Edited by the Japanese WISC- IV Publication Committee). Nihonban WISC- IV zisshi・saiten manual [Implementation and scoring manual for the Japanese version of the Wechsler intelligence scale for children]. Nihon Bunka Kagakusha Co., Ltd.
Yao, Y., Du, F., Wang, C., Liu, Y., Weng, J., and Chen, F. (2015). Numerical processing efficiency improved in children using mental abacus: ERP evidence utilizing a numerical stroop task. Front. Hum. Neurosci. 9:245. doi: 10.3389/fnhum.2015.00245
Yuzawa, M., and Yuzawa, M. (Eds.). (2014). Working Memory and Education. Kyoto: Kitaoji-shobou (in Japanese).
Zelazo, P. D. (2006). The dimensional change card Sort (DCCS): a method of assessing executive function in children. Nat. Protoc. 1, 297–301. doi: 10.1038/nprot.2006.46
Zelazo, P. D., and Carlson, S. M. (2012). Hot and cool executive function in childhood and adolescence: development and plasticity. Child Dev. Perspect. 6, 354–360. doi: 10.1111/j.1750-8606.2012.00246.x
Keywords: mental abacus, executive function, prefrontal cortex, fNIRS, working memory
Citation: Watanabe N (2023) An empirical study of supporting executive function in family education with mental abacus. Front. Educ. 8:851093. doi: 10.3389/feduc.2023.851093
Edited by:
Robert Perna, University of Michigan Medical Center, United StatesReviewed by:
Colleen Marino, TIRR Memorial Hermann Hospital, United StatesSonia Brito-Costa, Polytechnic Institute of Coimbra, Portugal
Copyright © 2023 Watanabe. This is an open-access article distributed under the terms of the Creative Commons Attribution License (CC BY). The use, distribution or reproduction in other forums is permitted, provided the original author(s) and the copyright owner(s) are credited and that the original publication in this journal is cited, in accordance with accepted academic practice. No use, distribution or reproduction is permitted which does not comply with these terms.
*Correspondence: Nobuki Watanabe, nobuki@kwansei.ac.jp