- Associate Professorship of Life Sciences Education, Department of Educational Sciences, TUM School of Social Sciences and Technology, Technical University of Munich, Munich, Germany
Digital media have played a central role in everyday school life, at least since the governments in various competence frameworks define the digital competence areas. With a view to experimentation in STEM lessons, digital media offers a variety of opportunities to promote learning processes. A benefit is expected from technological progress when visually imperceptible scientific processes are made visible with software and hardware systems. Augmented reality combines the real and virtual worlds so that the viewer physically moves in a real environment that contains virtual elements. Consequently, augmented reality offers good conditions for expanding students’ subject-specific knowledge regarding substance-particle concept understanding. When a technology like augmented reality is used in the classroom, the learning environment must be accepted by teachers. Teachers are thus actively involved in the modification of digital learning environments so that they can identify, evaluate, and select digital resources. Teachers’ acceptance, therefore, presupposes an upbeat assessment of the usability of the innovation. Attitudes and self-efficacy can influence digital literacy and, thus, acceptance. The study investigates whether chemistry teachers positively embrace augmented reality and accept them as learning tools. Considering the T(D) Pack model, the teachers’ digital competencies are examined concerning the subject- and media-didactic evaluation of digital media. First, self-efficacy and attitudes of teachers (N = 157) are assessed. After processing the (non-/HMD-)augmented reality learning environment, an acceptance and usability test (N = 122) follows. The data analysis provides reliability and correlation analyses according to classical test theory. The results demonstrated that chemistry teachers saw great potential in using digital media and AR and, in particular, positively evaluated the AR learning environment on the tablet for chemistry teaching. In this context, the analyses revealed significant correlations between attitudes and acceptance.
1 Introduction
Digital competencies are not only essential in everyday life but also in the modern world of work and especially in the school context (Fischer et al., 2015). Accordingly, with the publication of “Competencies in the Digital World” by the Kultusministerkonferenz (2017), the educational mandate at German schools became more apparent than ever. Digitization should reach all school subjects (Huwer and Banerji, 2020). Thus, the school community should aim to support learners in developing digital competencies (Sailer et al., 2017). Accordingly, learners and teachers must develop media skills (cf. Gu et al., 2013). During the recent coronavirus pandemic, it became clear that teachers must acquire competencies in teaching and learning with digital media, especially concerning new innovative technologies (Lachner et al., 2020). Therefore, several frameworks have been designed to map teachers’ professional knowledge. Referring to the TPACK model (Köhler and Mishra, 2009), this professional knowledge consists of three dimensions: content knowledge (CK), pedagogical knowledge (PK), and technical knowledge (TK). On this basis, the DPACK model addresses not only technology but also digitality (Stalder, 2018). Concerning the individual and societal digital transformation, the components are combined into a pedagogical and subject content knowledge (DPCK) that relates to digitality as a basis for a subject-specific and media-pedagogical reflected instructional design with technologies (Stalder, 2018; Huwer et al., 2019a,b). The DigCompEdu competence framework systematizes the various media-related competencies of teachers needed to implement their educational mission in a digitalized world (Redecker and Punie, 2017). Digitization is based on dynamic and fast-moving scientific developments that can be challenging for (prospective) teachers. Therefore, the DiKoLan competence framework has developed standards for teacher training based on the existing subject curricula. This framework is the first science education and practice-oriented approach that differentiates the TPACK model in a domain-specific way (Becker et al., 2020; Kotzebue et al., 2021).
With a view to experimentation in STEM lessons, digital media offers a wide range of possibilities to promote learning processes and activities (Nerdel and von Kotzebue, 2020). A benefit should result from the technical progress, especially when visually imperceptible scientific processes are made visible with soft- and hardware systems (Huwer et al., 2019a,b). Particle modeling techniques (e.g., tablet with video) contribute to the understanding of the substance-particle concept by explaining the real-world phenomenon on the substance level through appropriate forms of representation. Augmented reality (AR) has become popular as a forward-looking technology in recent years (Akçayır and Akçayır, 2017; Buchner and Freisleben-Teutscher, 2020). While in virtual reality technology, the viewer moves in a fully simulated environment, AR links virtual and real worlds (Azuma, 1997). For this reason, AR is also referred to as mixed reality (Milgram and Kishino, 1994) and can be used to visualize not only 2D objects but also 3D representations. In addition, AR information can be controlled interactively (Azuma, 1997; Dunleavy and Dede, 2014). It is possible to view the combination of the physical and digital world in two ways (Milgram and Kishino, 1994), either on a screen (e.g., PC, tablet, smartphone) or as AR glasses directly integrated into the field of view (e.g., head-mounted display, abbreviated HMD; cf. Lauer and Peschel, 2023). The unique nature of AR for the chemistry subject becomes evident when submicroscopic particles are virtually projected onto a real experiment while considering dynamics. According to Fiorella and Mayer’s coherence and contiguity principle Fiorella and Mayer (2021), this simultaneous presentation should foster positive learning effects. It also avoids split attention, often with conventional media (Ayres and Sweller, 2021). AR thus offers new pedagogical possibilities for multimedia learning (Buchner and Freisleben-Teutscher, 2020; Keller and Habig, 2022) and can provide promising support for the learning process as a promotional measure. Accordingly, AR concerning interactivity (cf. Dunleavy and Dede, 2014) should be particularly suitable for promoting self-regulated learning (Buchner and Freisleben-Teutscher, 2020). Furthermore, AR as a tool for interaction and communication can have a positive impact on learners’ attitudes and motivation (Bacca et al., 2014; Radu and Schneider, 2019) and, ultimately, on learning performance (Hellriegel and Čubela, 2018; Nechypurenko et al., 2018; Buchner and Freisleben-Teutscher, 2020; Keller and Habig, 2022).
AR technology can play a constructive role in teacher education, but this is closely linked to the TPCK of teachers (Buchner and Zumbach, 2020). In addition to the conditions of the school, such as IT infrastructure, the evaluation of the innovative teaching and learning material, as well as the open-mindedness toward it, has a significant influence on the perspective use of technology in teaching (Fischer et al., 2015). Teachers must be able to evaluate AR as an educational technology and adequately assess its technical possibilities (Buchner and Zumbach, 2020). Accordingly, teachers must be supported in their professional development to understand how innovations such as AR work and how they can select, reflect, and use them for their subject-specific and media pedagogical potential (Buchner and Zumbach, 2020). Only when the teacher is satisfied with the digital tool (Kopp et al., 2003) a successful implementation in classes at schools will be possible (cf. Kiraz and Ozdemir, 2006; Krause and Eilks, 2015; Dalle et al., 2021). Acceptance is a favorable adoption decision of an innovative technology (Simon, 2001) and describes the attitude toward a behavior (cf. Álvarez-Marín et al., 2021). Special attention is paid to didactic and media design when developing digital learning environments (Bürg, 2005; Holden and Rada, 2011). Thus, assessing their system quality is crucial when successfully integrating innovations such as AR into subject teaching (Silius et al., 2003; Kiraz and Ozdemir, 2006; Dalim et al., 2017). In this regard, the literature shows a correlation between the assessment of digital learning tools and interest as well as perspective learning success (Karapanos et al., 2018; Fitting et al., 2023). Venkatesh and Davis (2000) refer to Ease of Use or the more common term Usability (Fitting et al., 2023). Usability is summarized by the European Committee for Standardization (DIN EN ISO 9241-110:2020–10, 2020) as the extent to which a system can be used to achieve goals with effectiveness and satisfaction in a specific context of use. Accordingly, usability can be defined as a predictor of acceptance in terms of media and didactic design (Bürg, 2005). In addition to usability, acceptance is also affected by the characteristics of the individual. Personality traits are based on Fishbein and Ajzen’s (2010) Theory of Planned Behavior, which holds that attitudes or self-efficacy can influence intention and thus change a person’s behavior. Graham et al. (2009) conducted a pilot study to measure STEM teachers’ self-efficacy concerning the TPACK model. The results indicate a relationship between usage behavior and self-efficacy regarding digital media (cf. Graham et al., 2009). Accordingly, it can be assumed that chemistry teachers’ assessment of competence can influence the likelihood of a desired behavior (Abd Majid and Mohd Shamsudin, 2019; Álvarez-Marín et al., 2021), such as the subject-specific reflective use of AR (Bürg, 2005; Vogelsang et al., 2019). Therefore, teachers should have subject-specific and media-didactic knowledge in order to be able to evaluate innovative technologies such as AR and their potential for teaching and learning in chemistry.
Thus, this study investigates how chemistry teachers evaluate AR learning environments and accept them as teaching and learning tools. Attitudes and self-efficacy regarding digital media and AR will provide insights into open-mindedness and media-related competencies. Taking into account the T(D)PACK model, the study examines the following questions:
RQ1: How do chemistry teachers evaluate the use of digital media and AR in chemistry classes, and how do they assess their skills and abilities in using them?
RQ2: How do chemistry teachers evaluate AR learning environments’ characteristics and usability, and to what extent do they accept AR learning environments?
RQ3: How do the characteristics of the individual and the learning environments relate to the acceptance of AR learning environments?
2 Methods
2.1 Participants and data collection
Teachers from the STEM field, primarily with chemistry as a subject, participate in the data collection. One hundred fifty-seven teachers are digitally surveyed to assess their self-efficacy and attitudes (digital media/AR). Subsequently, the chemistry teachers test the learning environments. Experimental group 1 consists of 46 subjects working with an AR learning environment on a tablet. Comparison group 2 (N = 41) works on the HMD-AR learning environment with the same content on the AR glasses, and the control group (N = 35) uses a simulation-based learning environment on the tablet with equivalent content. The treatment is followed by a usability and acceptance test to evaluate the learning environment (N = 122). Figure 1 describes the procedure of the entire data collection, although it was integrated into a more extensive main study to understand the matter-particle concept. This paper focuses on the pre-and post-surveys to capture the characteristics of the individual and the learning environments and omits the test tasks (cf. grayed out in Figure 1).
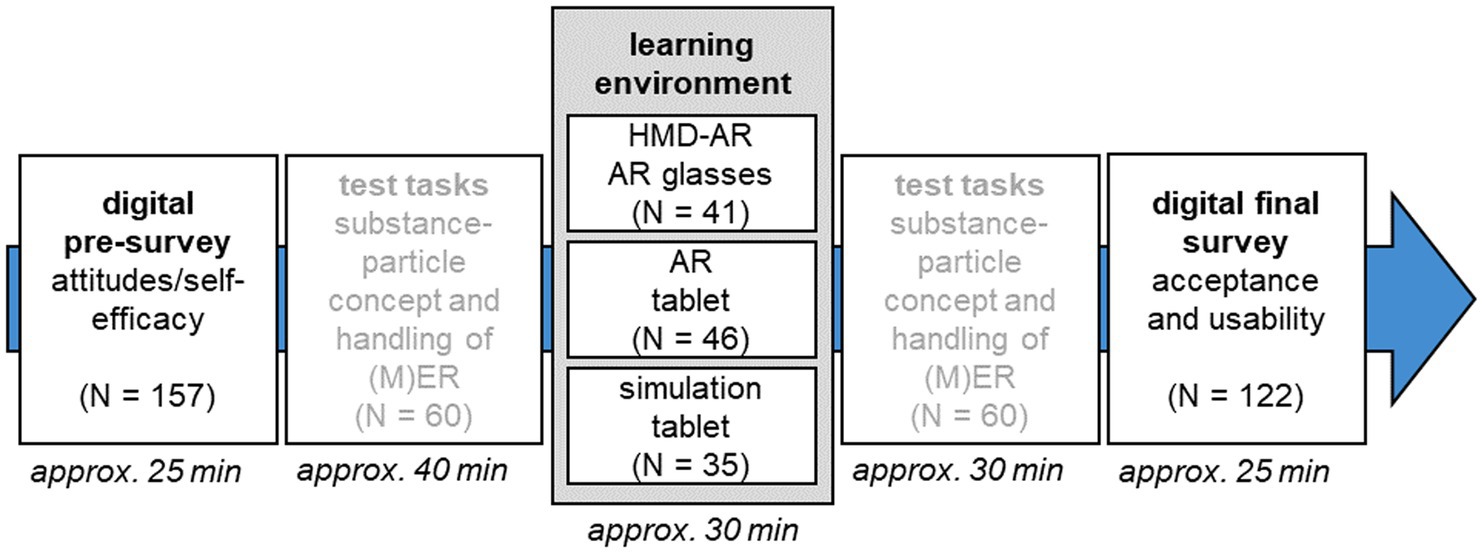
Figure 1. Implementation and procedure of data collection with a focus on the described sub-study: recording of person characteristics and recording of acceptance and usability, as well as processing of one of the three learning environments.
2.2 Design of the AR - learning environment
Three content-identical (non-) AR learning environments focusing on particle modeling were developed to determine the usability and acceptance of innovations such as AR. The AR learning environment on redox reactions consists of a real experimental setup for the electrolysis of zinc iodide. When the subjects point a tablet with the app at the electrolysis cell, the virtual learning environment appears in the foreground. The function menu allows interactive control, in which the display format is virtually projected onto the real experiment. The AR setting includes four learning paths elaborated before and after the DC voltage source is switched on. The user can distinguish between the presentation forms text, symbol, and image in every learning path.
A transfer to the non-AR variant (tablet) and HMD-AR variant (AR glasses) occurred based on the tablet-based AR setting. Figure 1 shows that the user can view the chemical reactions pictorially and simultaneously project the particle-level processes next to or into the real experiment (non-AR vs. AR vs. HMD-AR). The particle-level processes in the AR learning environments are always oriented to the real experiment sequence at the substance level (see Figure 2).
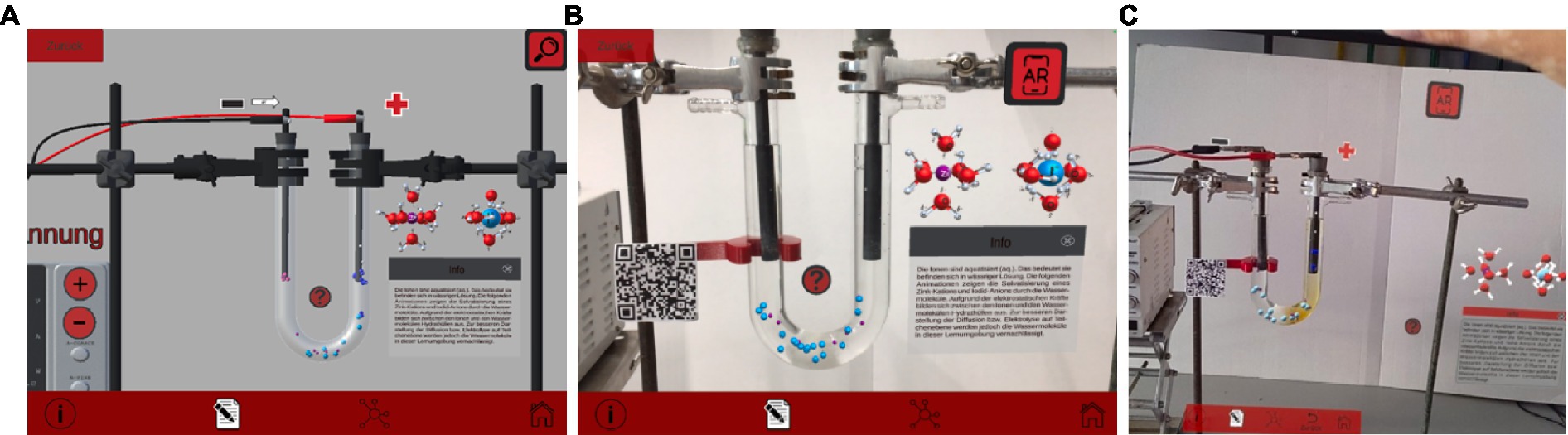
Figure 2. Comparison of the (A) non-AR, (B) AR, and (C) HMD-AR learning environments (from left to right) without and with the real experimental setup and the virtual particle processes on the tablet and AR glasses, respectively.
2.3 Survey instruments and research method
Based on Naumann et al. (2001) and Vogelsang et al. (2019), one questionnaire was developed to assess the characteristics of the persons. The items were intended to operationalize the use of digital media and innovations such as AR against the background of chemistry teaching. According to Graham et al. (2009), the items were differentiated for the TPACK model. Figure 3 demonstrates four scales on attitudes and self-efficacy regarding digital media and innovations such as AR. In addition, each of these main scales was subdivided into two to three subscales (see also N = number of items in Figure 3), so that the levels of technical pedagogical content knowledge (TPCK), technical pedagogical knowledge (TPK), technical knowledge (TK), and superordinate knowledge without a specific view on teaching (General) were focused on, correspondingly. Furthermore, exemplary sample items are given in Figure 3. Previous experience with digital media and augmented reality was also recorded as part of the preliminary survey.
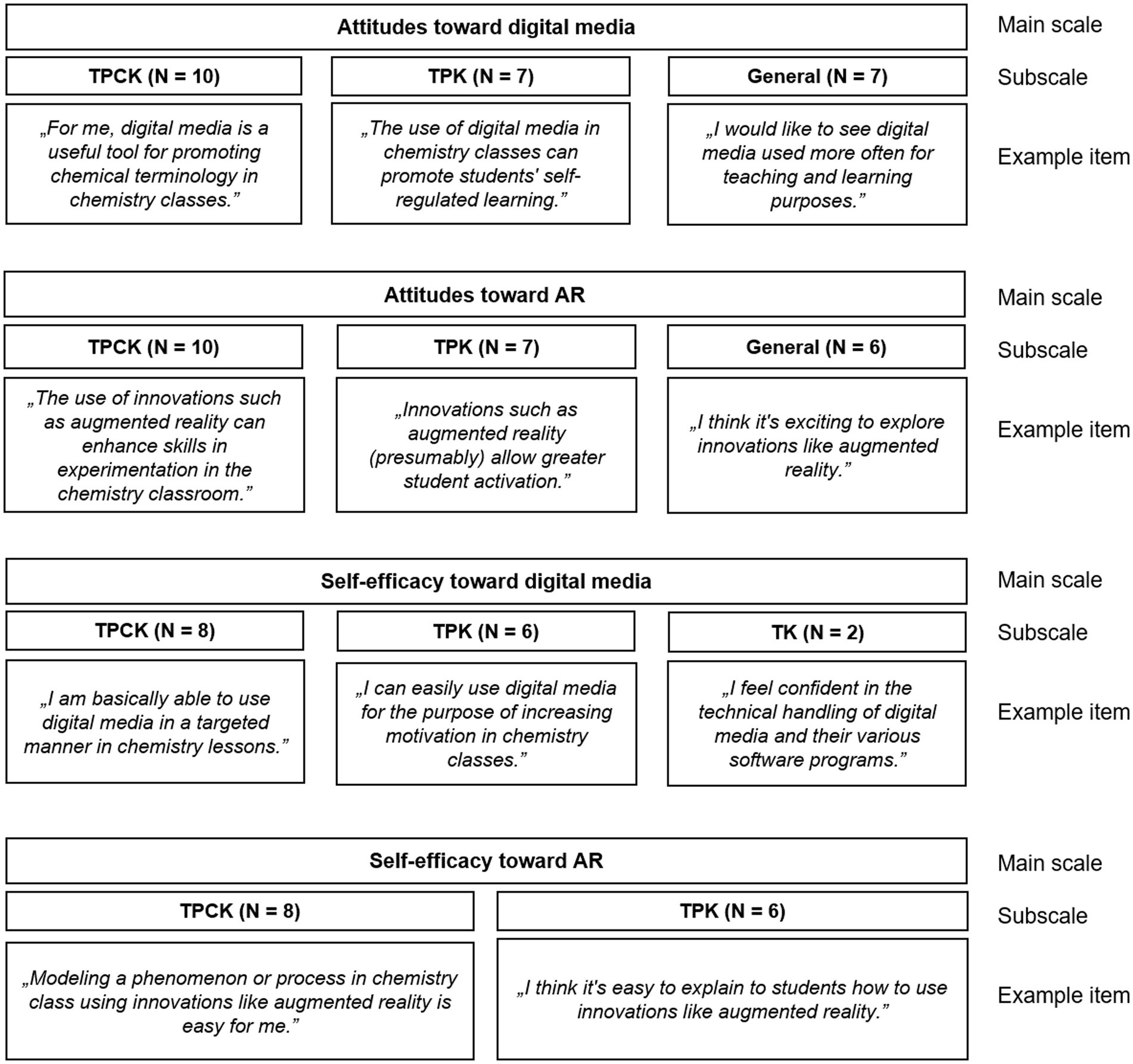
Figure 3. Overview of the main scales and subscales with exemplary sample items of the questionnaire for capturing personal characteristics; N = number of items.
In addition, following Bürg (2005), Kopp et al. (2003), and Simon (2001), another questionnaire was developed to assess the acceptance and usability of the particular learning environment. Accordingly, an acceptance scale and eight usability scales were constructed (see Figure 4). In order to be able to comprehensively survey the teachers’ assessments of the usability of the learning environments, a differentiation is made between didactic, media-didactic, and technical design criteria, as well as aspects of school transfer during implementation in the classroom.
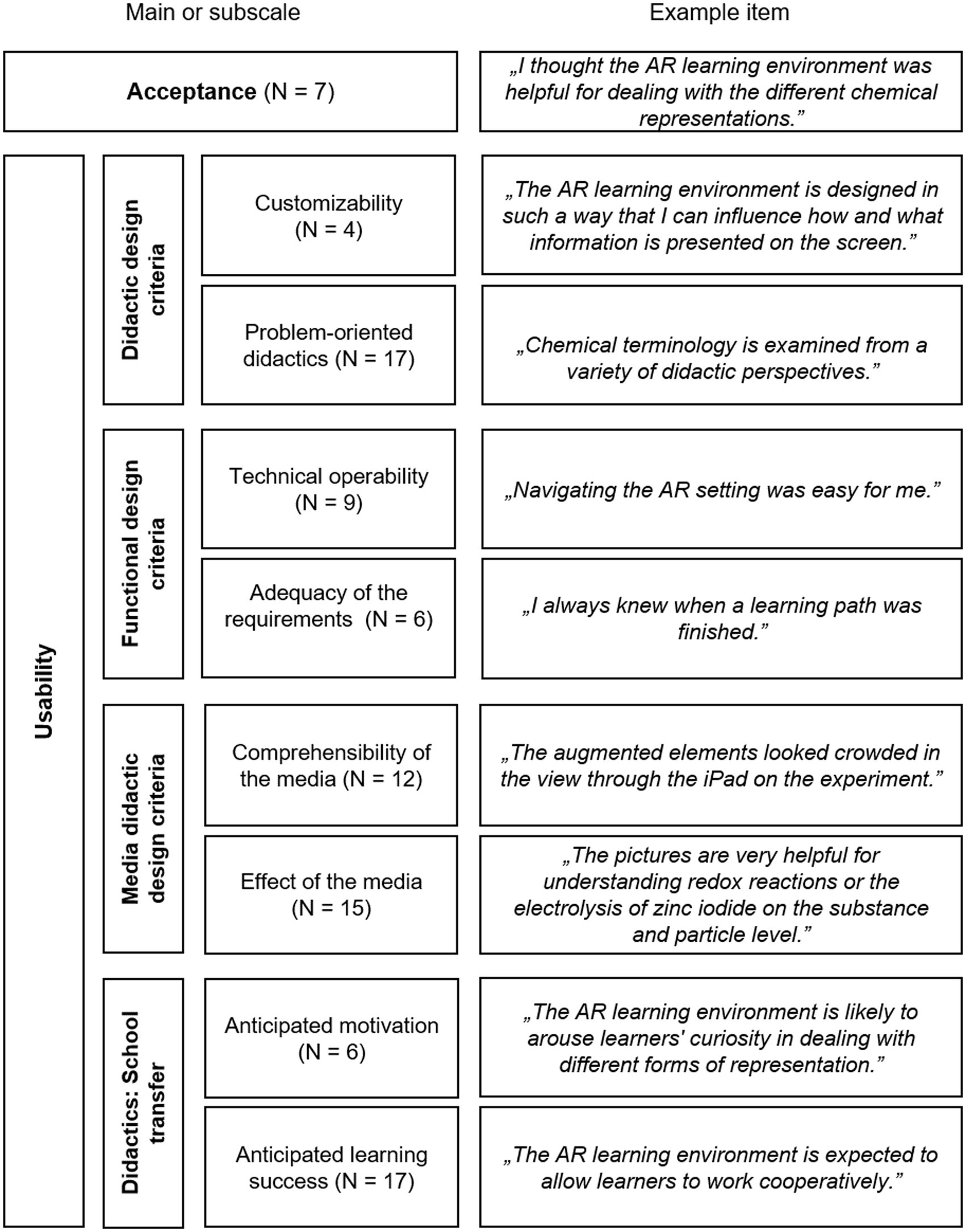
Figure 4. Overview of the main scales and subscales with exemplary sample items of the questionnaire for capturing acceptance and usability; N = number of items.
The data are analyzed descriptively and with reliability and correlation analyses based on classical test theory. A comparison of the three groups provides deeper insights into the acceptance of digital media and AR for chemistry teaching. The impact of the learning environment features on chemistry teachers is examined.
3 Results
All of the 157 participants who answered the test on personal characteristics stated that they used digital media privately, for example, for communication or entertainment purposes (“social media” or “YouTube videos”), and that they also regularly integrated them into their lessons. Traditional digital learning applications such as videos or presentations were mentioned more frequently. Five participants said they use AR privately (e.g., “PokemonGo”). Furthermore, 14 teachers stated that they have already used AR in their subject teaching. More than 60% of teachers (N = 98) declared that they regularly engage in digital professional development (e.g., measurement). This subset includes 16.3% who have attended at least one AR training (e.g., using the AR app “Blippar”) (N = 16).
The reliability analyses for the individual characteristics (attitudes and self-efficacy, see Table 1) resulted in excellent Cronbach’s α-values between α = 0.87 and α = 0.97. With mean scores above the mean scale level of 1.5, the usefulness of digital media and AR was rated highly. As expected, digital media self-efficacy is higher than AR below the mean scale level (see Table 1). Chemistry teachers rated their skills in using traditional digital applications as good (M ≥ 1.92). Values between 0.45 and 0.7 can describe the associated scatter. If self-efficacy is focused on AR, the mean values are consistently around 0.7. The increased standard deviation in the scale to AR (SD = 0.72) indicates a high dispersion. The subscales regarding TPACK support the results of the main scales. Strikingly, the TK and General subscales consistently showed the highest mean values, whereas the TPCK scales were the lowest, except for attitudes toward AR.
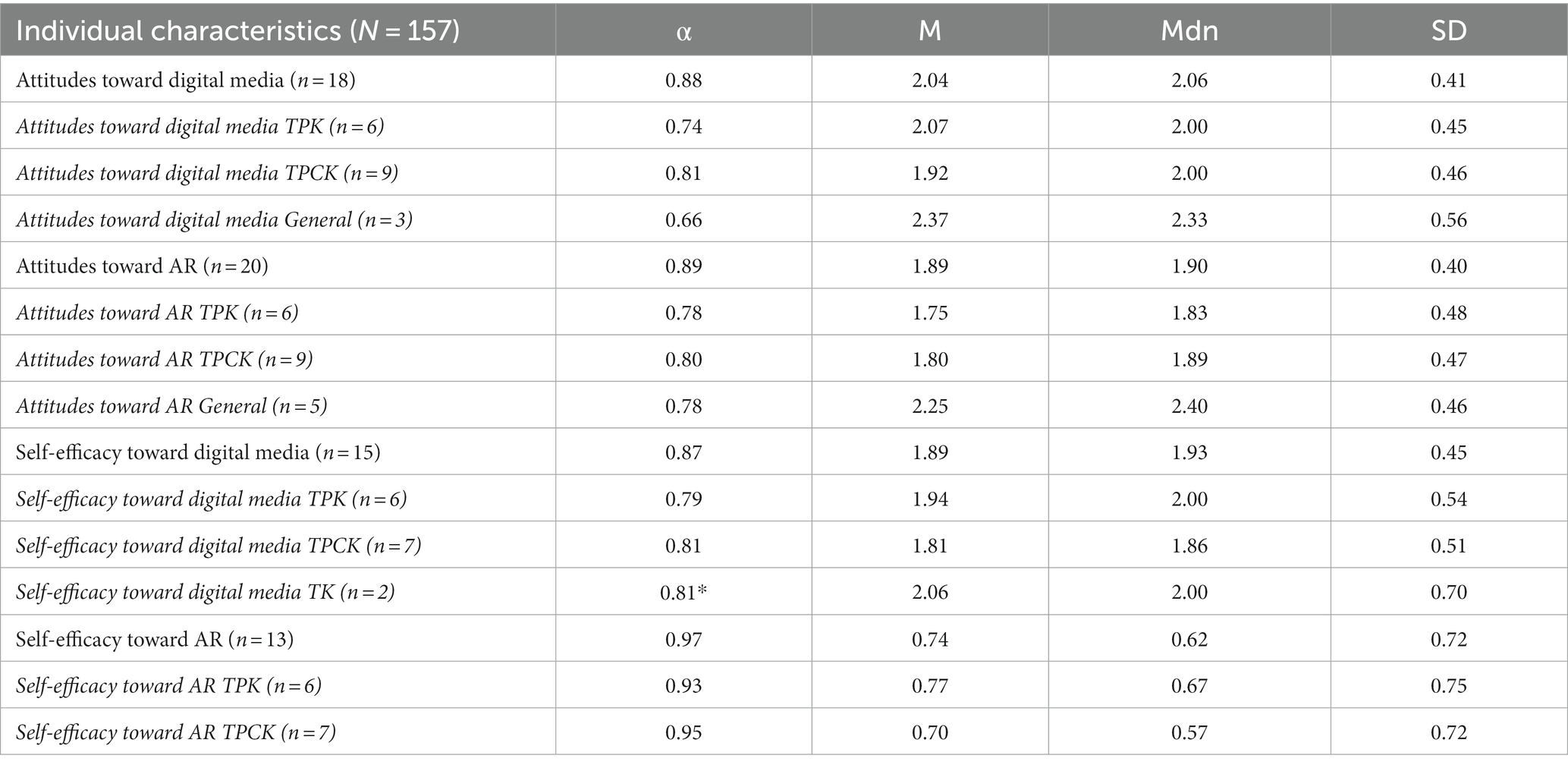
Table 1. Scale documentation of the test instrument on person characteristics (attitudes and self-efficacy) with main and their TPACK subscales, four-point Likert scale (0: “strongly disagree” to 3: “strongly agree”), indicating number of subjects (N), number of items per scale (n), Cronbach’s alpha of the scale (α), mean (M), median (Mdn), and standard deviation (SD).
The usability and acceptance scales had acceptable to excellent internal consistency values (cf. Table 2). Except for the scales adequacy of requirements, customizability, and comprehensibility of the media with alpha values between 0.69 and 0.78, these scales have high reliabilities (all Cronbach’s α > 0.79). Thereby, an excellent value of internal consistency (α = 0.90) is shown in the scale effect of the media (cf. Table 2). Overall, chemistry teachers rated the digital learning environments as consistently positive, with mean scores ranging from 2.05 to 2.47. In particular, subjects seem to embrace the concept of learning applications. This is evident from the high mean value in the acceptance scale with its low dispersion (M = 2.38, SD = 0.45). The customizability was estimated with M = 2.05 at the lowest. In this context, this scale’s increased standard deviation (SD = 0.56) stands out from the other scales and indicates a high degree of dispersion (Table 3).
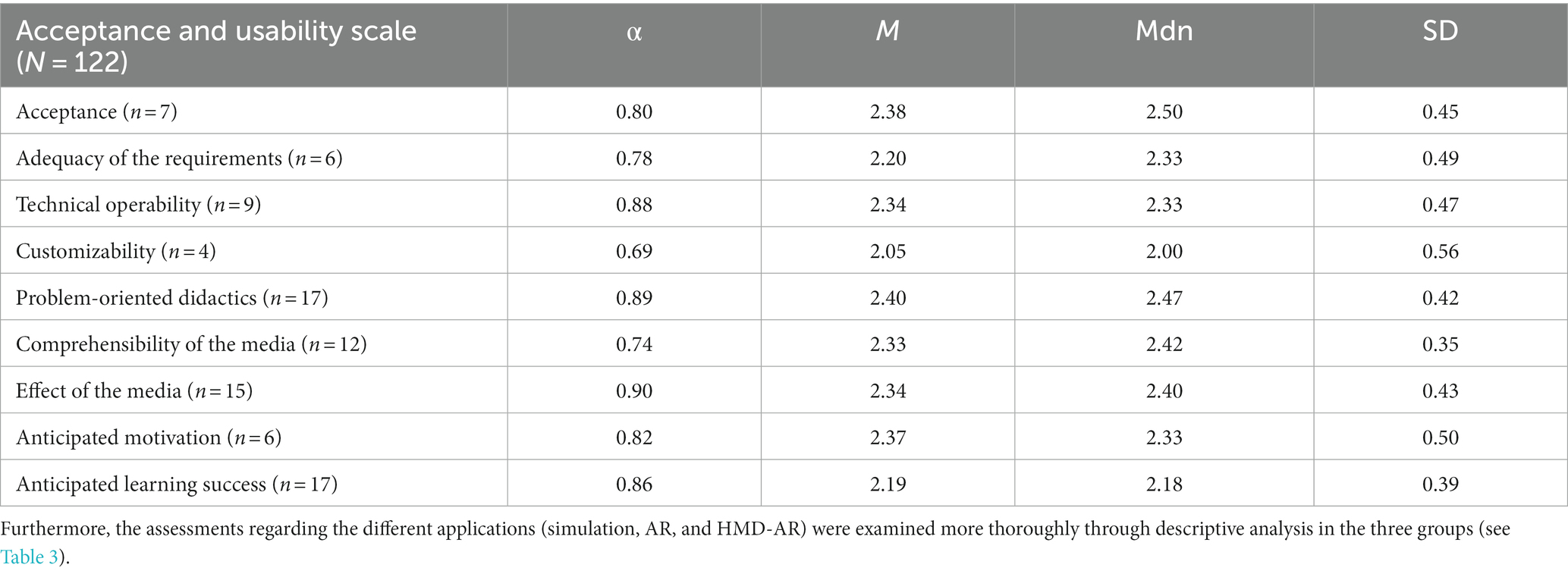
Table 2. Scale documentation of the Acceptance and Usability Test with a four-point Likert scale (0: “strongly disagree” to 3: “strongly agree”) in Main Study 1 indicating the number of subjects (N), number of items per scale (n), Cronbach’s alpha of the scale (α), mean (M), median (Mdn), and standard deviation (SD).
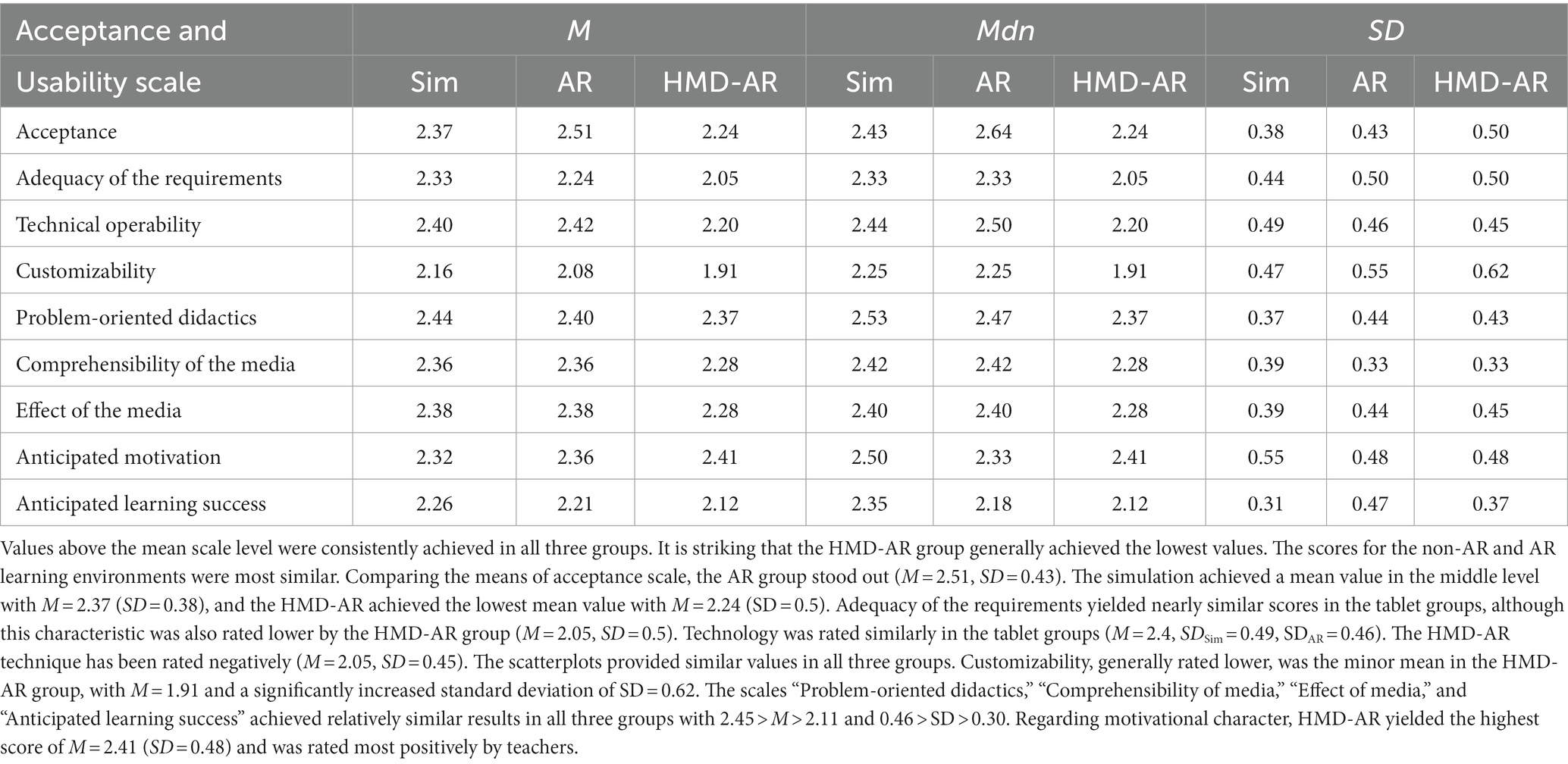
Table 3. Descriptive statistics of the acceptance and usability test with a four-point Likert scale (0: “strongly disagree” to 3: “strongly agree”) comparing groups with simulation (N = 35), AR- (N = 46), and HMD-AR (N = 41), indicating the mean (M), median (Mdn), and standard deviation (SD).
The results demonstrated significant correlations between acceptance and attitudes toward digital media and AR with rs values of at least 0.25 at the 0.01 significance level (cf. Table 4). There is almost a medium correlation (rs = 0.30) concerning the attitudes toward AR. Overall, there are highly marginal correlations between the scales on self-efficacy about digital media or AR and acceptance. According to this, self-efficacy seems to be largely independent of acceptance. The correlation matrix shows that a very weak correlation prevails between the self-efficacy regarding digital media and acceptance (rs = 0.10). The functional design criteria were less strongly associated with acceptance appropriateness of requirements: rs = 0.24 and technical usability (rs = 0.25). The customizability and comprehensibility of the media provided medium correlations with rs > 0.35. All other usability scales correlated highly with acceptance (rs > 0.50).
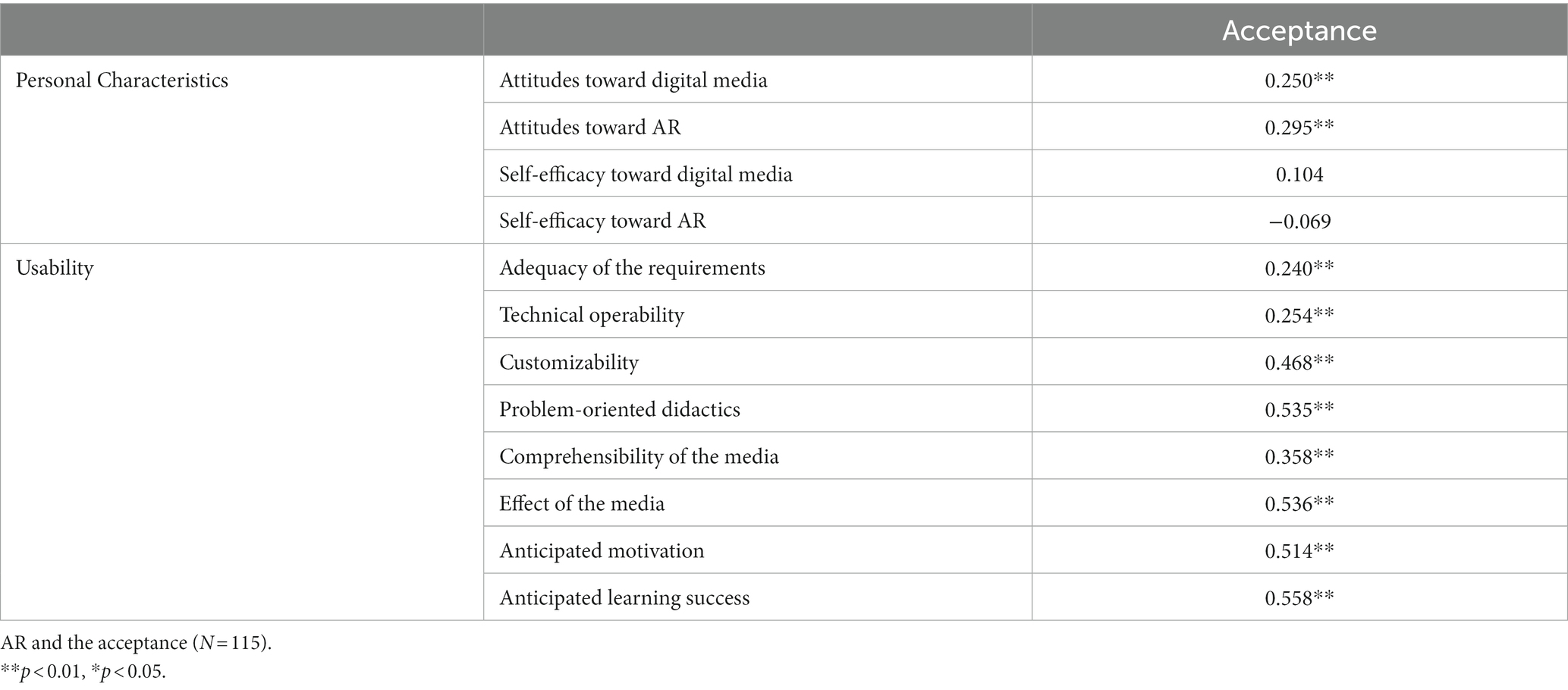
Table 4. Bivariate correlations between the scales on usability and person characteristics regarding digital media resp.
4 Discussion
The chemistry teachers saw opportunities and benefits in using digital media and AR (cf. RQ1). It is assumed that prior knowledge about digital media for school purposes was very pronounced and positively related to attitudes (Dinse de Salas, 2019). However, since the pandemic entailed numerous restrictions at the beginning of the data collection phase (e.g., homeschooling), it is conceivable that some subjects were critical of the technological innovations (Borukhovich-Weis et al., 2021). In addition to the restrictions such as contact bans, distance learning, and sick leave taking over substitute lessons, Corona revealed an acceleration effect concerning technologies, which certainly challenged the teachers mentally. Data collection on the scale described in Chapter 3.1 could have been too demanding for the participants and could have harmed the study results. While the usefulness of innovations such as AR was viewed positively overall, the slightly lower scores suggest that teachers were less receptive to AR than digital media. Like Vogelsang et al. (2019), the participants reported little touch points regarding the use of AR in chemistry classes. Accordingly, learning-related prior experience with AR and knowledge concerning T(D)PACK are low (cf. Graham et al., 2009). Teachers rated their own competencies in dealing with conventional digital applications as consistently good (cf. RQ1). Since subject teaching was confronted with many digital innovations during the survey period and teachers were repeatedly presented with new, mostly unknown possibilities of digital lesson design (cf. Tappe, 2019), media-related competencies seem to have been assessed lower. Accordingly, professional knowledge might be based more on generally manifested technical skills (cf. Self-efficacy toward AR and digital media with a focus on TK in Chapter 4) that are less targeted at instructional practice-related changes (cf. DigiCompEdu and T(D)PACK model). STEM teachers’ expertise in AR was weaker (see Sumadio and Rambli, 2010; Vogelsang et al., 2019), but still more robust than expected. Furthermore, a significant scattering is shown. Since teachers assigned innovations such as AR a moderate to slightly elevated potential for practical use in schools in the course of the survey, it becomes apparent that they have so far almost hardly used them in the classroom and consequently could not possess any experience with them (cf. Sumadio and Rambli, 2010). Accordingly, these teachers appear to be less competent concerning innovative technologies (cf. Scheiter, 2021). Although the self-efficacy tests did not test knowledge, self-efficacy and acceptance should tend to correlate (Dinse de Salas, 2019). Accordingly, it could be explained that the high degree of novelty, as well as a lack of media pedagogical knowledge regarding AR (cf. Sumadio and Rambli, 2010; Knüsel Schäfer, 2020), influenced the assessment of self-efficacy (Graham et al., 2009; Krause and Eilks, 2015). However, interest in technology or openness to new things might have positively affected self-efficacy and explained the re-expected elevated scores (Knüsel Schäfer, 2020). Teachers may also overestimate themselves (Knüdel Schäfer Becker et al., 2020). Therefore, knowledge tests regarding the use of AR should follow. They can give a deeper insight into the competencies related to the T(D)PACK model.
The teachers consistently rated the three digital learning environments positively (cf. RQ2). High values of the assessed features of the learning environment indicate that the didactic media design criteria of the learning environments were implemented very well (Bürg, 2005). The conceptual preparation of the learning application seems to have been considered promising (Kopp et al., 2003), which speaks for an exemplary implementation of the learning environment’s media, functional, methodological, and didactic design. The simulation-based and AR-based learning environments were assessed very similarly. Given the technical challenges associated with using HMD-AR technology (Scheerer, 2021), it is not surprising that the high-end technology scored the lowest with the highest dispersion. The scope of action to work self-directed with AR glasses was visibly rated lower than with the other applications. Finally, this finding should be explained with the help of the novelty level of the high-end technology. The novelty of AR glasses concerning their functions and their associated difficulties in using them have significantly impacted the rating (Buchner et al., 2021). Only the motivational strength seems to come to the fore with the HMD-AR technique (Bacca et al., 2014; Radu and Schneider, 2019; Buchner and Zumbach, 2020). The data material indicates the trend that the usability of the non-AR variant was rated slightly better than the AR learning environment on the tablet. The challenges in handling the HMD-AR technology were mentioned more often, calling for a visible need for optimization (Scheerer, 2021). Because the AR learning environment on the tablet tended to inspire greater familiarity with the technology, the technical operation should present fewer difficulties (Wu et al., 2013). Accordingly, the non-AR learning environment was rated most efficient, and the HMD-AR learning environment was least conducive to learning. The results of the acceptance scale also clearly show that STEM teachers accept the concept of the learning environment very well (cf. RQ2, Kopp et al., 2003). The AR learning environment experienced the highest adoption among chemistry teachers, whereas the simulation-based learning environment was ranked slightly lower, and the HMD-AR setting was the least accepted (Bürg, 2005). Despite its strongly motivational character, the AR glasses apparently could not consistently meet the teachers’ subject didactic requirements. This was justified by the extremely high degree of unfamiliarity and the low level of media-related knowledge regarding AR, which often led to technical difficulties (Scheerer, 2021). Furthermore, it cannot be excluded that the technical operation of the AR glasses led to a cognitive overload and thus negatively influenced the evaluation (cf. Wu et al., 2013; Ibáñez and Delgado-Kloos, 2018). Following recent findings attributing a high extrinsic load (cf. Chandler and Sweller, 1991) to AR glasses (cf. Buchner et al., 2021), teachers’ cognitive overload may have led to lower satisfaction with the technology. The study results demonstrate the need to promote STEM teachers’ media-related skills and abilities in addition to technical ones.
It is clear that the questionnaire from the preliminary survey, similar to Graham et al. (2009) and Vogelsang et al. (2019), is very well suited to assess the person characteristics against the background of the TPACK model. Furthermore, the study revealed an assessment tool’s importance in operationalizing usability and acceptance (cf. Kopp et al., 2003). Such evaluation instruments can help teachers manage the evaluation process and gather important information for the prospective use of the teaching and learning tool in the classroom (cf. Silius et al., 2003). Concerning the DigComEdu competency framework, innovations such as AR, in particular, should be integrated more firmly into the framework so that teachers become more confident in handling them and better evaluate their potential for use in the classroom. However, it is noted that aspects of digitality should have been considered in the survey instruments of the present study. Tools for augmenting chemistry experiments that focus on socially relevant, real-world problems and include ethical components (e.g., greenhouse effect/global warming or alcohol) put digitality in the foreground (cf. Stalder, 2018; Huwer et al., 2019a). For this case, the questionnaires should urgently be expanded and, in addition to the teachers’ digital competence (questionnaire of the preliminary study), also depict items on usability concerning so-called socio-scientific issues in the final survey questionnaire (Rahayu, 2019).
The results demonstrated significant correlations between attitudes and acceptance (cf. RQ3). If digital media and AR are assessed as beneficial for STEM teaching, their adoption as teaching and learning material increases (Stark et al., 2001; Schallehn, 2004). Concerning the attitudes toward AR, an almost medium and thus higher correlation is shown than in the attitudes regarding digital media. Accordingly, the more significant effect on attitudes toward AR suggests that, in particular, a high open-mindedness toward innovative technologies is associated with a high adoption of AR. The innovation specification should explain the significance of the attitudes regarding AR (Bandura, 2001; Bürg, 2005). According to Karapanos et al. (2018), experienced and skilled individuals tend to be more likely to perceive interaction principles, make analogies, and recognize the effectiveness and efficiency of the learning material when dealing with technology. The data material provided only a weak correlation between self-efficacy regarding digital media and acceptance. This result is astonishing since self-efficacy as an indicator of media-related prior knowledge (Fishbein and Ajzen, 2010) should have a decisive influence on adopting innovations (Burkhardt and Brass, 1990). Per se, the teachers were inexperienced in AR, which should require much of the available elaboration time to learn how to use the app (Karapanos et al., 2018). Ultimately, descriptive analysis provided that subjects rated their self-efficacy for AR mostly higher than expected and sometimes contrary to their identified prior experiences. While positive experiences may increase self-directed use of their initiative and thus influence self-efficacy (Knüsel Schäfer, 2020), the surprising result is that only about 9% of the teachers reported prior AR experience. According to Scheiter (2021), more conservative assessments of one’s ability for AR would suggest highly competent teachers because participants would recognize the wide range of AR. After the acceptance scale yielded very high scores, the results are difficult to interpret and should be analyzed in further research. Particularly when it comes to teachers’ digital literacy (cf. Stalder, 2018; Huwer et al., 2019a), future research should examine relationships not only between attitudes and self-efficacy regarding TPACK, but also DPACK, and acceptance. Ultimately, the novelty of AR seems to be responsible for the mixed picture. Significant values were diagnosed in all usability scales, whereas the functional design criteria were rather weakly associated with acceptance. Chemistry teachers seem to accept the learning environment when they find learning with it effective, efficient, and satisfying (Figl, 2010). As expected, a positive evaluation of usability, especially concerning media didactic design, perspective learning processes among students, and media effects, seems to be associated with high satisfaction. Therefore, future teacher training concepts should focus primarily on the didactic potential of innovative techniques (e.g., artificial intelligence) and, with a view to the significance of DPACK, also increasingly address the digitality of innovations (Huwer et al., 2019a).
Data availability statement
The raw data supporting the conclusions of this article will be made available by the authors, without undue reservation.
Ethics statement
The studies involving human participants were reviewed by an independent ethics committee and approved by the Bavarian State Ministry of Education and Cultural Affairs (Az. Ref.: IV.7-BO4106.2020/50/8 of 01.03.2021). The studies were conducted in accordance with the local legislation and institutional requirements. The participants provided their written informed consent to participate in this study.
Author contributions
MR: Writing – original draft, Writing – review & editing. CN: Writing – original draft, Writing – review & editing.
Funding
The author(s) declare that no financial support was received for the research, authorship, and/or publication of this article.
Conflict of interest
The authors declare that the research was conducted in the absence of any commercial or financial relationships that could be construed as a potential conflict of interest.
Publisher’s note
All claims expressed in this article are solely those of the authors and do not necessarily represent those of their affiliated organizations, or those of the publisher, the editors and the reviewers. Any product that may be evaluated in this article, or claim that may be made by its manufacturer, is not guaranteed or endorsed by the publisher.
References
Abd Majid, F., and Mohd Shamsudin, N. (2019). Identifying factors affecting acceptance of virtual reality in classrooms based on technology acceptance model (TAM). Asian J. Univ. Educ. 15, 51–10. doi: 10.24191/ajue.v15i2.7556
Akçayır, M., and Akçayır, G. (2017). Advantages and challenges associated with augmented reality for education: a systematic review of the literature. Educ. Res. Rev. 20, 1–11. doi: 10.1016/j.edurev.2016.11.002
Álvarez-Marín, A., Velázquez-Iturbide, J., Castillo-Vergara, M., and Castillo-Vergara, M. (2021). Technology acceptance of an interactive augmented reality app on resistive circuits for engineering students. Electronics 10:1286. doi: 10.3390/electronics10111286
Ayres, P., and Sweller, J. (2021). “The Split-attention principle in multimedia learning” in The Cambridge handbook of multimedia learning. 3rd ed. eds. R. E. Mayer and L. Fiorella (Cambridge: Cambridge University Press).
Azuma, R. T. (1997). A survey of augmented reality. Presence Teleop. Virt. 6, 355–385. doi: 10.1162/pres.1997.6.4.355
Bacca, J., Baldiris, S., Fabregat, R., and Graf, S. (2014). Augmented reality trends in education: A systematic review of research and applications. J. Educ. Technol. Soc. 17, 133–149.
Bandura, A. (2001). Social cognitive theory: an agentic perspective. Annu. Rev. Psychol. 52, 1–26. doi: 10.1146/annurev.psych.52.1.1
Becker, S., Bruckermann, T., Finger, A., Huwer, J., Kremser, E., Meier, M., et al. (2020). “Orientierungsrahmen Digitale Kompetenzen für das Lehramt in den Naturwissenschaften – DiKoLAN” in Digitale Basiskompetenzen–Orientierungshilfe und Praxisbeispiele für die universitäre Lehr-amtsausbildung in den Naturwissenschaften. eds. C. Tyssen, S. Becker, and J. Meßinger-Koppelt (Hrsg.) (Hamburg: Joachim Herz Stiftung), 14–43.
Borukhovich-Weis, S., Grey, J., Łączkowska, E., and Gryl, I. (2021). Distanzlehre und die Einstellungen zukünftiger Lehrer*innen zu Digitalisierung. In: A. Kienle, A. Harre, and J. M. Haake und A. Lingnau (Hg.): GI-edition proceedings band 316 DELFI 2021. Bonn: Gesellschaft für Informatik e.V. (GI-Edition. Proceedings, 316). 307–318.
Buchner, J., Buntins, K., and Kerres, M. (2021). A systematic map of research characteristics in studies on augmented reality and cognitive load. Comp. Educ. Open 2, S1–S8.
Buchner, J., and Freisleben-Teutscher, C. (2020). Die Erweiterung der Realität als Bildungschance: Fallbeispiele für immersives Lernen in Schule und Hochschule. A. Beinsteiner, L. Blasch, T. Hug, P. Missomelius, and M. Rizzoli (Hg.): Augmentierte und virtuelle Wirklichkeiten. 1. Auflage. Innsbruck Medien - Wissen - Bildung: Innsbruck University Press.
Buchner, J., and Zumbach, J. (2020). Augmented reality in teacher education: a framework to support teachers’ technological pedagogical content knowledge. Ital. J. Educ. Technol. 28, 106–120.
Bürg, O. (2005). “Akzeptanz von E-Learning in Unternehmen” in Die Bedeutung von institutionellen Rahmenbedingungen, Merkmalen des Individuums und Merkmalen der Lernumgebung für die Akzeptanz von E-Learning (Berlin: Logos)
Burkhardt, M. E., and Brass, D. J. (1990). Changing patterns or patterns of change. The effect of a change in technology on social network structure and power. Adm. Sci. Q. 1, 104–127.
Chandler, P., and Sweller, J. (1991). Cognitive load theory and the format of instruction. Cogn. Instr. 8, 293–332. doi: 10.1207/s1532690xci0804_2
Dalim, C. S., Kolivand, H., Kadhim, H., Sunar, M. S., and Billinghurst, M. (2017). Factors influencing the acceptance of augmented reality in education: a review of the literature. J. Comput. Sci. 13, 581–589. doi: 10.3844/jcssp.2017.581.589
Dalle, J., Raisinghani, M. S., Putra, A., Suriansyah, A., Hadi, S., and Sahara, B. (2021). A technology acceptance case of Indonesian senior school teachers. Int. J. Online Pedagog. Course Des. 11, 45–60. doi: 10.4018/IJOPCD.2021100104
DIN EN ISO 9241-110:2020–10. Ergonomie der Mensch-System-Interaktion_- Teil_110: Interaktionsprinzipien (ISO_9241–110:2020). Deutsche Fassung EN_ISO_9241–110:2020 (2020).
Dinse de Salas, S. (2019). Digitale Medien im Unterricht - Entwicklung professionellen Wis-sens und professionsbezogener Einstellungen durch Coaching [Erlangung des Grades einer Doktorin der Philosophie (Dr. phil.)]. Heidelberg: Pädagogischen Hochschule Heidelberg.
Dunleavy, M., and Dede, C. (2014). Augmented reality teaching and learning. In: M. J. Spector, D. M. Merrill, and J. Elen und M. J. Bishop (Hg.): Handbook of research on educational communications and technology. New York, NY: Springer New York.
Figl, K. (2010). Deutschsprachige Fragebögen zur Usability-Evaluation im Vergleich. Zeit-schrift für Arbeitswissenschaft 4, 321–337.
Fiorella, L., and Mayer, R. E. (2021). Principles for reducing extraneous processing in multimedia learning. In: R. E. Mayer und L. Fiorella (Hg.): The Cambridge handbook of multimedia learning. Cambridge: Cambridge University Press. 185–198.
Fischer, F., Wecker, C., and Stegmann, K. (2015). Auswirkungen digitaler Medien auf den Wissens- und Kompetenzerwerb in der Schule. Kurzbericht. Ludwig Maximilans Universität München, München.
Fishbein, M., and Ajzen, I. (2010). “Predicting and changing Behavior,” in The reasoned action approach. Psychology Press.
Fitting, N., Ulber, R., Czubatinski, L., and Hornung, G. (2023). Evaluation digitaler Arbeitsblätter im Chemieunterricht in Hinblick auf Usability und Interesse. In: Roth, J., Baum, M., Eilerts, K., Hornung, G., and Trefzger, T. (Hg.): Die Zukunft des MINT-Lernens – Band 2. Digitale Tools und Methoden für das Lehren und Lernen. Berlin, Heidelberg: Springer Berlin Heidelberg, 93–108.
Graham, C. R., Burgoyne, N., Cantrell, P., Smith, L., St Clair, L., and Harris, R. (2009). TPACK development in science teaching: measuring the TPACK confidence of in-service science teachers. TechTrends 53, 70–79.
Gu, X., Zhu, Y., and Guo, X. (2013). Meeting the "digital natives". Understanding the acceptance of technology in classrooms. J. Educ. Technol. Soc. 16, 392–402.
Hellriegel, J., and Čubela, D. (2018). Das Potenzial von Virtual Reality für den schulischen Unterricht - Eine konstruktivistische Sicht. MedienPädagogik, (Occasional Papers), 58–80.
Holden, H., and Rada, R. (2011). Understanding the influence of perceived usability and technology self-efficacy on Teachers' Technology acceptance. J. Res. Technol. Educ. 43, 343–367. doi: 10.1080/15391523.2011.10782576
Huwer, J., and Banerji, A. (2020). Corona sei Dank?! – Digitalisierung im Chemieunterricht. CHEMKON 27, 105–106. doi: 10.1002/ckon.202000037
Huwer, J., Irion, T., Kuntze, S., Schaal, S., and Thyssen, C. (2019a). Von TPaCK zu DPaCK – Digitalisierung im Unterricht erfordert mehr als technisches Wissen. MNU J.
Huwer, J., Lauer, L., Dörrenbächer-Ulrich, L., Perels, F., and Thyssen, C. (2019b). Chemie neu erleben mit Augmented Reality. Neue Möglichkeiten der individuellen Förderung. MNU J. 420–427.
Ibáñez, M. B., and Delgado-Kloos, C. (2018). Augmented reality for STEM learning: A systematic review. Comput. Educ. 123, 109–123.
Karapanos, M., Becker, C., and Christophel, E. (2018). Die Bedeutung der Usability für das Lernen mit digitalen Medien. MedienPädagogik: Zeitschrift für Theorie und Praxis der Medienbildung, 36–57.
Keller, S., and Habig, S. (2022). “Supporting spatial thinking in organic chemistry through augmented reality —an explorative interview study,” in Student reasoning in organic chemistry. eds. N. Graulich and G. Shultz (Hg.) (The Royal Society of Chemistry), 19–35.
Kiraz, E., and Ozdemir, D. (2006). The relationship between educational ideologies and technology acceptance in pre-service teachers. J. Educ. Technol. Soc. 9, 152–165.
Knüsel Schäfer, D. (2020). Überzeugungen von Lehrpersonen zu digitalen Medien: eine qualitative Untersuchung zu Entstehung, Bedingungsfaktoren und typenspezifischen Entwicklungsverläufen. Verlag Julius Klinkhardt, p. 288.
Köhler, M. J., and Mishra, P. (2009). What is technological pedagogical content knowledge? Contemp. Issues Technol. Teach. Educ. 9, 60–70.
Kopp, B., Dvorak, S., and Mandl, H. (2003). Evaluation des Einsatzes von Neuen Medien im Projekt "Geoinformation – Neue Medien für die Einführung eines neuen Querschnittfachs" (Forschungsbericht Nr. 161). Ludwig Maximilans Universität München, München. Department Psychologie Institut für Pädagogische Psychologie.
Kotzebue, L. V., Meier, M., Finger, A., Kremser, E., Huwer, J., Thoms, L. J., et al. (2021). The framework DiKoLAN (Digital competencies for teaching in science education) as basis for the self-assessment tool DiKoLAN-Grid. Educ. Sci. 11:775.
Krause, M., and Eilks, I. (2015). Lernen über digitale Medien in der Chemielehrerausbildung. CHEMKON 22, 173–178. doi: 10.1002/ckon.201410259
Kultusministerkonferenz (2017). Strategie der Kultusministerkonferenz. Bildung in der digitalen Welt.
Lachner, A., Scheiter, K., and Stürmer, K. (2020). Digitalisierung und Lernen mit digitalen Medien als Gegenstand der Lehrerinnen- und Lehrerbildung. C. Cramer, J. König, and M. Rothland und S. Blömeke (Hg.): Handbuch Lehrerinnen- und Lehrerbildung. Bad Heilbrunn: Klinkhardt.
Lauer, L., and Peschel, M. (2023). ‹pedagogical usability› von augmented reality zum Thema Elektrik. MedienPädagogik 51, 25–64. doi: 10.21240/mpaed/51/2023.01.11.X
Milgram, P., and Kishino, F. (1994). A taxonomy of mixed reality visual displays. IEICE Trans. Info. Syst. 77, 1321–1329.
Naumann, J., Richter, T., and Groeben, N. (2001). Validierung des INCOBI anhand eines Vergleichs von Anwendungsexperten und Anwendungsnovizen. Zeitschrift für Pädagogische Psychologie 15, 219–232. doi: 10.1024//1010-0652.15.34.219
Nechypurenko, P. P., Starova, T. V., Selivanova, T. V., Tomilina, A. O., and Uchitel, A. D.. (2018). “Use of augmented reality in chemistry education,” In Proceedings of the 1st international workshop on augmented reality in education. Kryvyi Rih, Ukraine: CEUR Workshop Proceedings, 2257, 15–23.
Nerdel, C., and von Kotzebue, L. (2020). Digitale Medien im naturwissenschaftlichen Unterricht. Aufgaben für die Lehrerbildung. Zeitschrift für Pädagogik 66, 159–173.
Radu, I., and Schneider, B. (2019). What can we learn from augmented reality (AR)? In: S. Brewster, G. Fitzpatrick, A. Cox, and V. Kostakos (Hg.): Proceedings of the 2019 CHI Conference on Human Factors in Computing Systems. Glasgow Scotland UK, New York, NY, USA: ACM, 04 05 2019 09 05 2019.
Rahayu, S. (2019). Socio-scientific issues (SSI) in chemistry education: enhancing both Students' chemical literacy & transferable skills. J. Phys. Conf. Ser. 1227:012008. doi: 10.1088/1742-6596/1227/1/012008
Redecker, C., and Punie, Y. (2017). “Digital competence of educators: DigCompEdu,” in Publications office of the European Union. Available at: http://bit.ly/39yohbE
Sailer, M., Murböck, J., and Fischer, F. (2017). Digitale Bildung an bayerischen Schulen-Infrastruktur, Konzepte, Lehrerbildung und Unterricht [Digital education in Bavarian schools - infrastructure, constructs, teacher education, and teaching]. vbw.
Schallehn, A. (2004). Akzeptanz und Motivation beim selbstregulierten Lernen unter Einsatz von neuen Medien in der berufsbegleitenden Weiterbildung [Inaugural-Disseration zur Erlangung des akademischen Grades des Doktorgrades (Dr. rer. pol.)]. Universität Kassel.
Scheerer, F. (2021). Interaktion mit der MBP IoT-Plattform mittels der Microsoft HoloLens (Master’s thesis).
Scheiter, K. (2021). Lernen und Lehren mit digitalen Medien: Eine Standortbestimmung. Zeitschrift fur Erziehungswissenschaft: ZfE 24, 1039–1060. doi: 10.1007/s11618-021-01047-y
Silius, K., Tervakari, A. M., and Pohjolainen, S. (2003). A multidisciplinary tool for the evaluation of usability, pedagogical usability, accessibility and informational quality of web-based courses. The Eleventh International PEG Conference: Powerful ICT for Teaching and Learning 28, 1–10.
Simon, B. (2001). E-Learning an Hochschulen. Gestaltungsräume und Erfolgsfaktoren von Wissensmedien. Köln: Josef Eul Verlag.
Stalder, F. (2018). “Herausforderungen der Digitalität jenseits der Technologie. Synergie,” Fachmagazin für Digitalisierung in der Lehre, 5, 8–5. Available at: https://www.synergie.uni-hamburg.de/de/media/ausgabe05/synergie05-beitrag01-stalder.pdf.
Stark, R., Hinkofer, L., and Mandl, H. (2001). Beispielbasiertes Lernen im Bereich Buchführung: Einfluss instruktionaler Erklärungen und multipler Perspektiven auf Lernverhalten und Lernerfolg (Forschungsbericht Nr. 134 ). München: Ludwig-Maximilians-Universität, Lehrstuhl für Empirische Pädagogik und Pädagogische Psychologie.
Sumadio, D. D., and Rambli, D. R. A. (2010). “Preliminary evaluation on user acceptance of the augmented reality use for education,” in 2010 2nd international conference on computer engineering and applications. ICCEA 2010, 2, 461–465.
Tappe, E.-H. (2019). Prädiktoren der Intention zum didaktischen Einsatz von digitalen Medien im Unterricht - Überführung der Unified Theory of Acceptance and Use of Technology (UTAUT) in ein schulisches Untersuchungssetting. T. Knaus (Hg.): Forschungswerkstatt Medienpädagogik. Profekt - Theorie - Methode. München: kopaed. 999–1027.
Venkatesh, V., and Davis, F. D. (2000). A theoretical extension of the technology acceptance model: four longitudinal field studies. Manag. Sci. 46, 186–204. doi: 10.1287/mnsc.46.2.186.11926
Vogelsang, C., Finger, A., Laumann, D., and Thyssen, C. (2019). Vorerfahrungen, Einstellungen und motivationale Orientierungen als mögliche Einflussfaktoren auf den Einsatz digita-ler Werkzeuge im naturwissenschaftlichen Unterricht. Zeitschrift für Didaktik der Na-turwissenschaften 25, 115–129.
Keywords: augmented reality - learning environment, substance-particle concept understanding, chemical terminology, teacher education and training, attitudes, self-efficacy, acceptance, usability
Citation: Ripsam M and Nerdel C (2024) Teachers’ attitudes and self-efficacy toward augmented reality in chemistry education. Front. Educ. 8:1293571. doi: 10.3389/feduc.2023.1293571
Edited by:
Johannes Huwer, University of Konstanz, GermanyReviewed by:
Sarah Malone, Saarland University, GermanyHolger Weitzel, University of Education Weingarten, Germany
Copyright © 2024 Ripsam and Nerdel. This is an open-access article distributed under the terms of the Creative Commons Attribution License (CC BY). The use, distribution or reproduction in other forums is permitted, provided the original author(s) and the copyright owner(s) are credited and that the original publication in this journal is cited, in accordance with accepted academic practice. No use, distribution or reproduction is permitted which does not comply with these terms.
*Correspondence: Melanie Ripsam, bWVsYW5pZS5yaXBzYW1AdHVtLmRl