- 1Centre de Recherches sur la Cognition et l’Apprentissage, Université de Poitiers, Université de Tours, CNRS, Poitiers, France
- 2Institut Universitaire de France, Paris, France
- 3PPRIME, Université de Poitiers, ISAE-ENSMA, CNRS, Poitiers, France
- 4Melioris, Centre de Médecine Physique et de Réadaptation Fonctionnelle Le Grand Feu, Niort, France
Several studies have shown that the acquisition of number meaning is related to finger motor representations. However, no study has tried to distinguish the respective roles of fingers representation and finger kinematics in this effect. Forty-three children (23 boys and 20 girls) enrolled in the middle class of kindergarten took part in the study. They were divided into two equivalent groups and compared on the acquisition of number meaning after a 2-week program designed to teach them a song about the numbers 1–9, scaffolded by visual supports. Each visual support included the symbolic representation of the number, the picture of an object mentioned in the song that rhymed with the number in French, and either a static image (static group) or an animated point-light display (animated group) of the finger representation. Results showed no difference between the two groups on the acquisition of numbers regarded as low (i.e., less than 5) but the animated group performed significantly better for numbers regarded as high (i.e., more than 5). Therefore, the present study shows the interest of using the observation of animated counting movement to teach number meaning in young children and suggests that finger kinematics may be more instrumental than static finger representation in the learning of number meaning. Future research is needed to confirm and specify this effect.
1 Introduction
Like reading and handwriting, arithmetic (Montagner, 2012) is one of the most important skills for humans to master. It relies on the acquisition of number meaning, which requires nonsymbolic representations of numbers (e.g., sets of dots) to be associated with symbolic representations (e.g., Arabic digits) (Brankaer et al., 2014; Li et al., 2018). Interestingly, the acquisition of number meaning can involve one of two different processes: either subitizing or counting. Subitizing refers to the cognitive ability to rapidly, automatically, and implicitly perceive small numbers (typically 1–4 items), whereas counting refers to a serial and effortful process that is typically used for numbers above 4 (Furman and Rubinsten, 2012). Subitizing is thought to be present from birth, whereas counting develops with age (Klein and Starkey, 1988). During the first years of their lives, children gradually develop their mathematical skills and, in particular, the ability to associate symbols (i.e., Arabic numbers and words) with semantic information about the meaning of the number. These skills would be based on the development of both non-symbolic (i.e., the ability to understand and manipulate numerical magnitudes) and symbolic (i.e., the ability to represent numbers verbally as strings of words and visually as strings of Arabic numerical symbols) representations (Dehaene and Cohen, 1995). In particular, number meaning acquisition would occur progressively between the ages of 2.5 and 6.5 years (Kolkman et al., 2013; Salminen et al., 2021), with the acquisition of number meanings from 1 to 3 between the ages of 2.5 and 4, and the acquisition of number meanings from 6 to 9 at around 6.5 years.
Interestingly, several studies assessing the role of finger-based strategies have shown that finger configuration is closely associated with numerical representations in both adults and children, suggesting that it plays a functional role in counting and arithmetic acquisition [see Moeller et al., 2012; Berteletti and Booth, 2016; Sixtus et al., 2023 for reviews]. For example, Crollen and Noel (2015) have shown that performance in counting and solving basic arithmetic problems is impaired in 5-year old children when they are asked to make interfering hand movements. Similarly, Costa et al. (2011) demonstrated that finger gnosia, i.e., “the ability to locate, name, and discriminate individual fingers” (p 2) is less developed in children with dyscalculia, suggesting that finger representations are directly related to arithmetic competencies (see Barrocas et al., 2020 for a review). Moreover, several electrophysiological, neuroimaging, and clinical studies confirmed that hand/finger representation and numerical processing share common neural processes (see Sixtus et al., 2023 for a review). For instance, a recent work showed that the left angular gyrus (BA 39) is active both when humans have to process digit numbers and fingers configurations, suggesting a strict neural association between these activities (Proverbio and Carminati, 2019). Altogether, the association between finger representation and acquisition of number meaning is well documented and most researchers agree that this association is important (even if not constitutive, see for examples Crollen et al., 2011; Lafay et al., 2013) for the early acquisition of symbolic number representations (Andres et al., 2007). Regarding the underlying mechanisms, the authors evoked an embodied view of cognition where numbers would be grounded in sensorimotor experiences (Sixtus et al., 2017). To bring some new arguments in favor of this theory, we decided to assess the role of biological kinematics. Indeed, biological kinematics refers to the natural way in which humans and nonhuman animals move (Johansson, 1973) and the literature tells that observing biological movements is equivalent to producing an action (Vaina et al., 2001). Consequently, assessing the role of kinematics in the link between fingers and numbers could bring some new insights into the role of embodiment in processing numbers even if no action is produced. Moreover, thanks to this paradigm, the unique contribution of finger movement in the link made between fingers and numbers can be isolated, since no visual representation of fingers is shown. To our knowledge, only one experiment in adults has directly tested the role of kinematics in number processing (Badets et al., 2015). In this study, the authors demonstrated that point-light displays (PLDs) primed numbers according to the number space association proposed by Dehaene (1992). Lower numbers (i.e., 1–5) were randomly generated after PLDs of movements directed to the left side, whereas higher numbers (i.e., 6–10) were randomly generated after PLDs of movements directed to the right side. However, this effect disappeared when PLD kinematics was modified to be nonbiological, suggesting that biological motion is crucial for number processing. Based on this finding, we sought to assess the role of finger kinematics in the acquisition of number meaning. More precisely, we aimed at assessing the respective roles of finger representation versus finger kinematics in the building of number processing. Distinguishing between these processes would be important at the theoretical level to bring some arguments in favor of the embodied theory of the link between fingers and numbers, and may lead to the development of new interventions for numbers learning and remediation. To this end, the present study compared the use of moving PLD versus static representations of fingers in the learning of number meaning in young children. As the acquisition of number meaning appeared gradually between 2.5 and 6.5 years, we proposed to assess children aged between 3 and 4 years old because they are still in progress concerning this competency. Actually, in accordance with the French program for the second year of kindergarten, children should have acquired the meaning of numbers up to 3 during the first year of kindergarten and of 1–9 numbers at the end of the third year (BOENJS, 2021). In line with previous research demonstrating that kinematics is essential for semantically representing concepts such as words (Beauprez and Bidet-Ildei, 2018) or numbers (Badets et al., 2015), we expected the PLDs to be more efficient than the static representations of fingers for children’s learning of number meaning. Moreover, given that subitizing can be used automatically to process quantities up to 4, we predicted that the difference between the PLD and static representations of fingers would be greater for numbers above 4 (i.e., when counting must be used).
2 Method
2.1 Participants
A total of 47 children (24 boys, 23 girls) in the middle class of kindergarten were invited to take part in the study. As this was the first study on the subject, we could not calculate the sample size beforehand, and the sample size was therefore based on previous studies of mathematics acquisition in children (see for example, Gibson et al., 2019). Children were enrolled in countryside villages localized near Poitiers (France) in one of the four public partner schools that had agreed to participate in the project. None of the children’s parents or legal guardians reported any uncorrected visual problems or specific disorder in the learning of mathematics. As four children did not complete the whole protocol, the results of only 43 children (23 boys, 20 girls) aged 3–4 years (mean = 3.89 ± 0.3) were included in the analysis. With this sample size, an a priori sensitivity analysis was made with Gpower (Faul et al., 2009) and for a normal distribution, a two-sided analysis, a probability of 0.05 and a power of 0.80, we should obtain an effect size with a Cohen’s d of 0.87.
2.2 Material
For the children’s song, we took the melody of a well-known nursery rhyme (“Frère Jacques”; https://www.youtube.com/watch?v=M1UIf6jh7EU) and adapted the lyrics to our experiment, by pairing each of the numbers 1 to 9 with an animal, person or object that rhymed with it in French (see Appendix 1 for all the pairs). These lyrics were scaffolded by visual supports featuring the written digit, a picture of the rhyming animal, person or object mentioned in the song, and a representation of the finger configuration (see Figure. 1). All the static images associated with the numbers are freely accessible online.1 The finger configurations were illustrated either with a photo of a hand representing the number or with an animated PLD representing the kinematics of the counting gesture (see Figure. 1). The static and moving images were both displayed for the same duration (about 53 s). The videos are freely accessible online (see footnote 1).
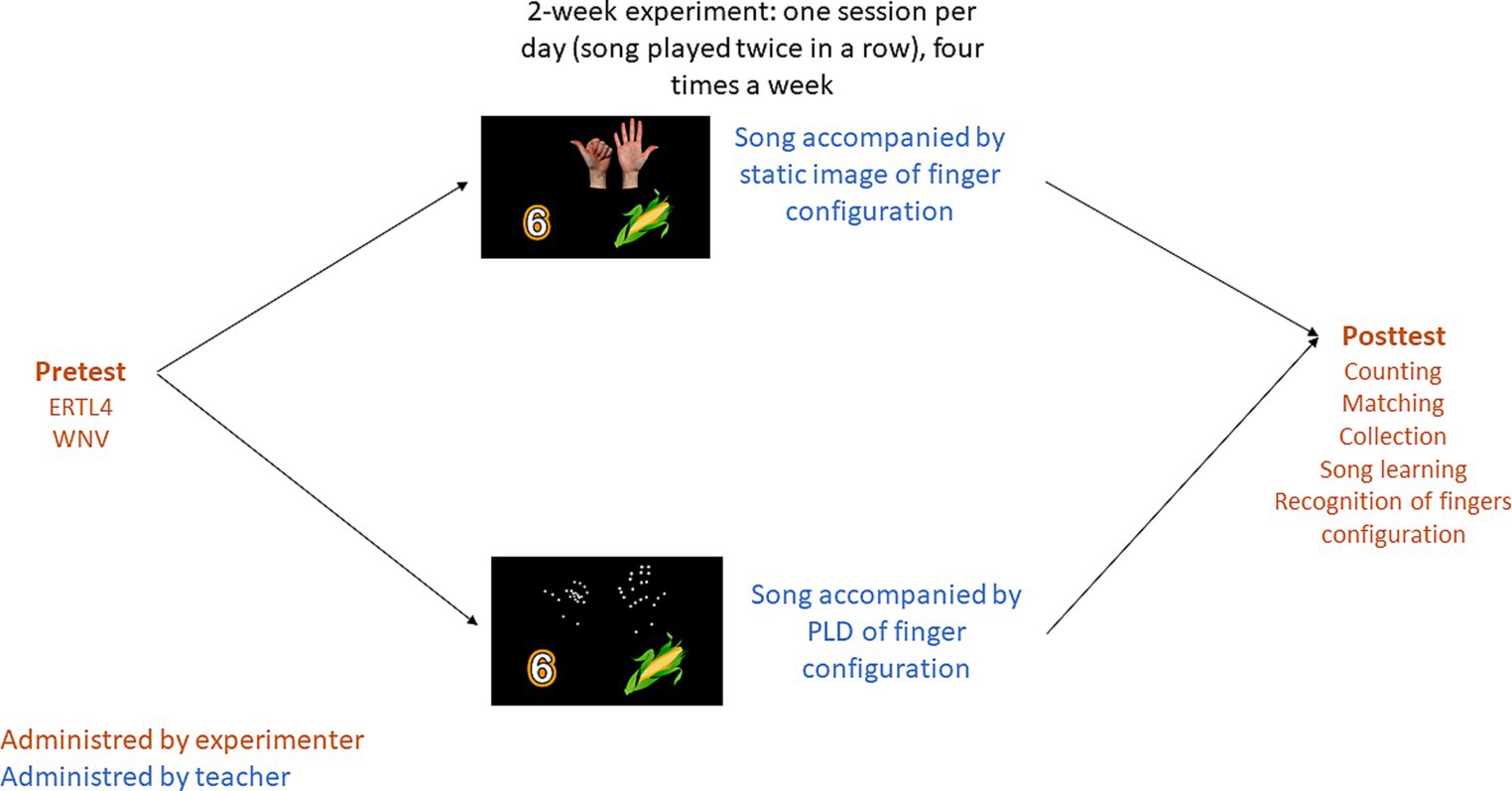
Figure 1. Study procedure. Each child underwent three phases in 2 weeks: pretest (Day 1) to assess the language and reasoning level, intervention phase (Days 2–14) where they had to learn a song with a video presenting the Arabic form of the numbers, a static image of an animal or object with a name which rhymed with each number and the corresponding static or animated finger configuration, and posttest (Day 15) with three tasks to assess the performance in the acquisition of number meaning and two control tasks.
To build the nine PLDs, a total of 42 light-reflecting markers were placed on the wrists and finger joints of an adult actor and filmed using a 3D motion capture system (Qualisys) comprising 20 infrared cameras (see Appendix 2 for positioning of the markers). The PLDs were then modified using PLAViMoP software (Decatoire et al., 2019), in order to present the same frontal hand view for each number (all PLDs available at https://osf.io/7xz8y/?view_only=df40a3b0b73e4331aea6e9744578d7ba).
2.3 Procedure
The children underwent the experiment at their school in October 2022 (i.e., beginning of the school year). The experiment was performed in three steps (see Figure 1).
2.3.1 Pretest
After inclusion, the experimenter came into each class and administered a pretest to each child. This pretest consisted of a language test and a global intelligence test adapted to young children aged 4 years upwards. These tests were chosen because language (e.g., Klein et al., 2013) and global intelligence (e.g., Passolunghi et al., 2008) are known to be involved in mathematic ability. The language test comprised the four subtests of the ERTL4 (word repetition, sentence repetition, comprehension, and image description), a French test that screens for language disorders in children (Alla et al., 1998). Children’s global intelligence was assessed with the Matrices and Recognition subtests of the short version of the Wechsler Nonverbal Scale of Ability [WNV; (Naglieri and Brunnert, 2009)]. After the pretest, children were divided in two equivalent groups matched on age, sex, teachers’ feedback about their mathematics ability and their pretest results. Both groups had to learn the same number song, accompanied by either the static images or the PLDs of the finger configurations.
2.3.2 Intervention
After the pretest, the experimenter gave each teacher the static and animated versions of the videos. The teachers then showed each group the corresponding version once a day, twice in a row. While one group was learning the song, the other group performed another activity. There were eight sessions in all, spread over 2 weeks (i.e., once a day four times a week, on Monday, Tuesday, Thursday and Friday). During each session, the teacher asked the children to try to learn the song and attend to the presentation, but did not mention learning the numbers.
2.3.3 Posttest
After the 2 weeks, the experimenter returned to each school and administered individual posttests. The posttest consisted of three tasks assessing the acquisition of number meaning, plus one task assessing the learning of the song, and two tasks assessing the recognition of either the photos or the PLDs of the finger configurations.
Concerning the acquisition of number meaning, children were asked to count with their fingers, select a number out of three possible options that corresponded to a set of cat pictures (see Appendix 3 for examples and https://osf.io/7xz8y/?view_only=df40a3b0b73e4331aea6e9744578d7ba for all the items), and count and arrange blocks corresponding to a given number. There were four trials for each task: two concerning low numbers (1–4), and two concerning large, high numbers (6–9). The low and high numbers used in each task were chosen randomly, but were the same for all the children (see Appendix 4 for all the combinations). At the end of the three number meaning acquisition tasks, each child had worked with the numbers 1, 2, 3, 4 and 6, 7, 8, 9.
The learning of the song was then assessed with a task where each child had to pair an image with a number learned in the song (see Appendix 5 for examples and https://osf.io/7xz8y/?view_only=df40a3b0b73e4331aea6e9744578d7ba for all items). Each number was displayed alongside three images: the image of the rhyming animal, person or object featured in the visual support, an image representing the same animal, person or object but in a different way, and an unrelated image. This task served to ensure that each child had learned the song and paid attention to the visual presentation.
Finally, children were shown a photo and a PLD, and had to say what each one represented (see Appendix 6). This task was used to determine whether our finger configurations were spontaneously associated with numbers. To congratulate them on their participation, each child was given a small diploma at the end of the experiment (see https://osf.io/7xz8y/?view_only=df40a3b0b73e4331aea6e9744578d7ba).
2.4 Data analysis
For all assessments, correct responses were scored 1 point, and incorrect responses were scored 0 points. For both the pretest (ERTL4, WNV) and posttest (acquisition of number meaning), the results of the subtests were summed, and the overall scores were then analysed. For the acquisition of number meaning, the scores for the low versus high numbers were also analysed. In all analyses, group (static vs. animated) was the between-participants factor. As our data violated the assumptions of sphericity and homogeneity tested with the Shapiro Wilk and Levene tests, we decided to conduct nonparametric Mann Whitney comparisons. Analyses were performed with JASP free software version 16.2. For all analyses, the level of significance was set at p < 0.05. The rank biserial correlation (rrb) indicated the effect size.
3 Results
3.1 Pretest
Table 1 shows the demographic data and pretest results for each group of children. The 43 children considered for analysis were all enrolled in the middle class of kindergarten in one of the four schools that agreed to take part in the project (see Appendix 7 for the characteristics of each child).
3.2 Posttest
3.2.1 Acquisition of number meaning
When we considered all numbers, there was no difference between the static and animated groups (W23,20 = 211, p = 0.65; see Figure. 2). Both groups performed well, with median scores of 7 (out of 12) (MAD = 2) for the static group, and 8 (MAD = 2.5) for the animated group. Interestingly, when we compared the groups on just the low or high numbers, no difference emerged between the groups (W23,20 = 298.5, p = 0.08) for the low numbers (Mdn = 6, MAD = 0 for static group and Mdn = 5, MAD = 1 for animated group), although the static group tended to perform better than the animated group. By contrast, the animated group (Mdn = 3, MAD = 1.5) performed significantly better than the static group (Mdn = 2, MAD = 1; W23,20 = 128, p < 0.05, rrb = −0.44) on the high numbers. Importantly, as the form of our distributions was not clearly identifiable, it was not possible to perform a post hoc analysis to assess the power of our effect. However, the effect size that we obtained (d = 0.983 instead of d = 0.88) was higher than the a priori effect size estimation (d = 0.88), suggesting that our effect achieved a power of at least 0.80.
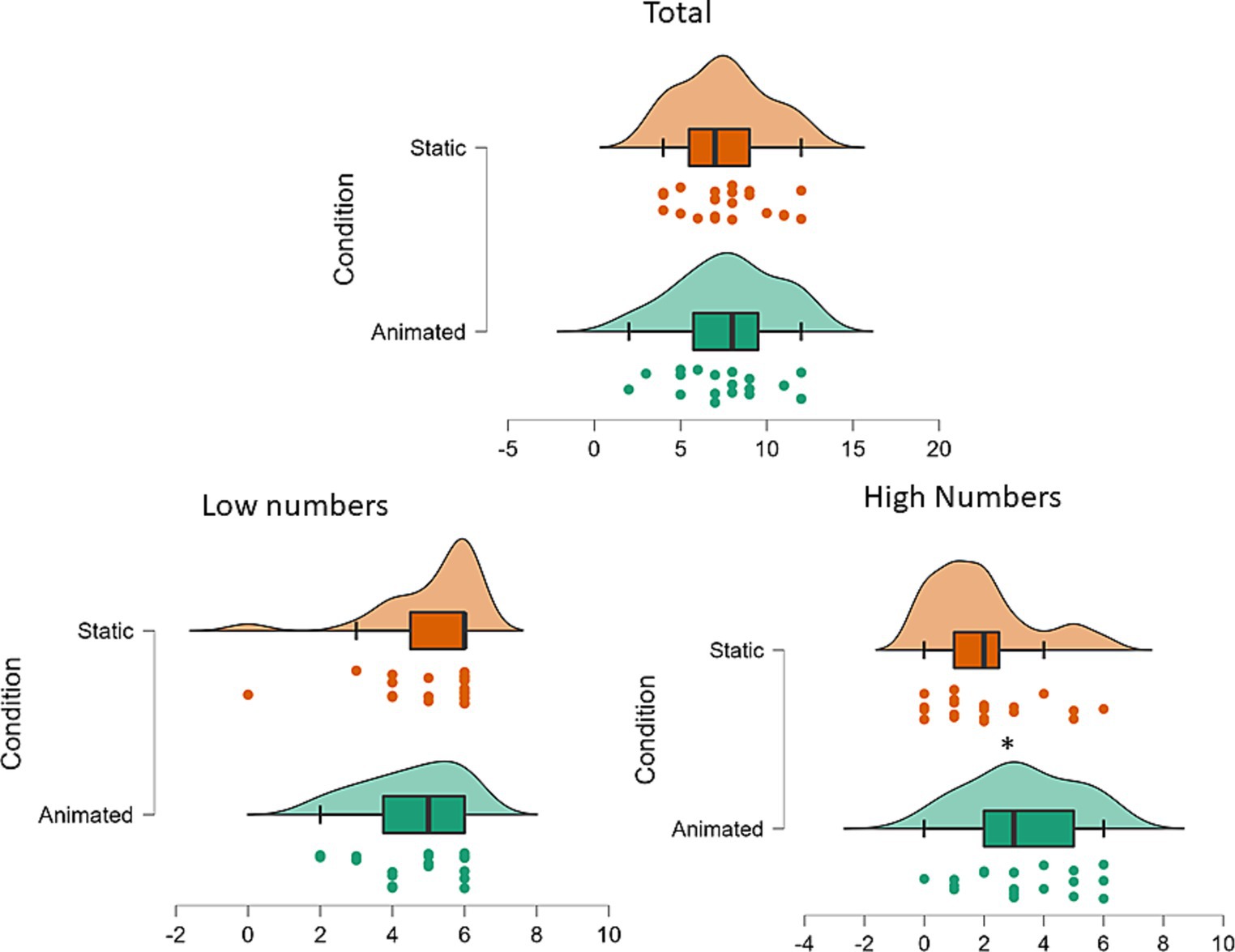
Figure 2. At the top: Raincloud plot representing the total performance obtained in the three tasks measuring the acquisition of number meaning in both groups. At the bottom: Raincloud plots representing the performance obtained in the three tasks measuring the acquisition of number meaning in both groups for low and high numbers, respectively. In each raincloud plot, dots represent the individual performances. The bold black line in the box plots shows the sample median, the hinges indicate the 25th and 75th quantile, and the whiskers point to 1.5 interquartile ranges beyond the hinges. There is also a representation of the density for each group using a Gaussian Kernel method. * p < 0.05.
3.2.2 Learning of the song
Analysis revealed no difference between groups (W23,20 = 268, p = 0.34), with both performing very well (Mdn = 8, MAD = 1 for static group and Mdn = 8, MAD = 1 for animated group).
3.2.3 Stimulus recognition
Analysis revealed no difference between groups on recognition of either the static images (W23,20 = 213, p = 0.50) or the PLDs (W23,20 = 221, p = 0.79). Interestingly, the static images were generally more closely associated with numbers than the PLDs (86% correctly recognized vs. 27% correctly recognized).
4 Discussion
Using the PLD paradigm, this study was designed to compare the respective roles of finger representation and finger kinematics in the acquisition of number meaning. Results indicated that PLDs representing moving fingers can improve the acquisition of number meaning in young children. These findings are in line with previous research showing that kinematics is involved in the processing of concepts such as words (Beauprez and Bidet-Ildei, 2018) and numbers (Badets et al., 2015), and demonstrate for the first time its role in the acquisition of number concepts. Moreover, our findings suggest that what is associated with number concepts are not the representation of fingers themselves, but rather the representations of finger movements (Di Luca and Pesenti, 2011). In the animated condition, the fingers themselves were not visible, and their presence was evoked solely by the motion. For us, this is the first evidence of the crucial role of finger movements in the link between numbers and fingers. Moreover, as biological motion observation implies motor system (Vaina et al., 2001), our result is consistent with the embodied view of cognition (Coello and Fischer, 2016) and with previous research showing that action production is more efficient than the viewing of static images (Sixtus et al., 2017).
Interestingly, the use of PLDs improved the children’s performance when the number processing was high (i.e., numbers above 5), but not when it was low (i.e., numbers below 5). These results are consistent with the idea that the acquisition of number meaning is based on two separate skills, i.e., subitizing and counting (Furman and Rubinsten, 2012), and suggest that finger kinematics could be more efficient to teach numbers to young children. The learning of quantities up to 5 (but not beyond) is in the curriculum for the middle class of kindergarten in France (BOENJS, 2021). This means that children had probably already worked with the numbers 1-4 in class. Moreover, the teaching of mathematics, and in particular the teaching of number meaning, is essentially based on the use of fingers, and static hand representations are usually associated with symbolic and nonsymbolic representations of numbers in class (BOENJS, 2021). As a consequence, the PLDs may not have affected performance on trials featuring low numbers because these numbers were already familiar to the children and usually associated with static representations of fingers. This hypothesis is supported by the fact that across the two groups, children had a success rate of about 80% for the acquisition of low number meanings.
One possible alternative explanation for the efficiency of PLDs for the learning of number meanings may be the sequentiality of the fingers’ appearance. In the static condition, the children saw all the fingers at the very outset, whereas in the animated condition, the fingers appeared one after the other. Therefore, independently of the finger movements, our effect may have been due to the sequential appearance of the dots (see Simon et al., 2021 for a review). The respective importance of biological kinematics versus sequentiality should be therefore studied in future research.
Finally, it seems that children had more difficulty spontaneously associating PLDs versus static images of finger configurations with the relevant numbers. This suggests that the advantage observed with PLD is implicit, and therefore confirms that finger representations are automatically evoked when children process the number concept (Andres et al., 2007). Moreover, the children may not have spontaneously associated the PLDs with the numbers because they had never before come across PLDs, whereas they were used to static representations of fingers being associated with numbers in class. This suggests that experience supports spontaneous associations between fingers and numbers (Lafay et al., 2013). However, the lack of association between PLDs and numbers could also be due simply to the question that was posed to children (“What is it?”) which may have prompted most of the children to respond by providing a visual description of what they had seen (e.g., “Some dots that were moving” or “Someone counting”) rather than the associated number. Future research should explore this point.
4.1 Limitations and future research
The present study had several limitations. First, even though our findings highlighted the potential role of biological kinematics in the learning of number meanings, our sample size was relatively small, and it would be useful to replicate these results with more participants.
Second, we cannot completely exclude that the impact of PLD presentation on the learning of numbers may be due to the fact that the PLD presentation was a video. Indeed, the video might have just captured children’s visual attention more than the static image of fingers.
Finally, the pretest did not include a direct evaluation of the mathematics competencies of children, in order not to draw the children’s attention to the mathematical aspect of the experiment and keep the learning of numbers implicit. Obviously, the use of teachers’ feedback to complement the results of the pretests introduced a subjective component in the definition of children’s groups that may have impacted the results.
Despite these limitations, the present study has shown that observing a point-light display could be efficient for the acquisition of number meaning, bringing new arguments for the embodied view of cognition. Furthermore, this paradigm is easy to use with young children and does not require time-consuming interventions. This suggests that observing a point-light sequence representing finger counting could be used to facilitate the acquisition of number meaning in typical children. This type of intervention has been tested here with children up to 3–4 years of age, but could be potentially of interest during all years of kindergarten where the aim is the acquisition of number meanings from 1 to 9. Moreover, this paradigm could also be proposed to children who have specific difficulties in acquiring mathematical skills. Indeed, some authors have proposed that mathematical disorders could be linked with poor acquisition of number meaning (e.g., Castaldi et al., 2020).
5 Conclusion
Taken together, our results suggest that PLD representing counting can foster the acquisition of number meaning in young children. In other words, kinematics may be crucial to the development of number processing (see Badets et al., 2015, for similar results in adults). However, more research is needed to confirm these findings, to assess their potential implications for educational practice, and to disentangle the various possible underlying processes.
Data availability statement
The datasets presented in this study can be found in online repositories. The names of the repository/repositories and accession number(s) can be found below: https://osf.io/7xz8y/?view_only=df40a3b0b73e4331aea6e9744578d7ba.
Ethics statement
The studies involving humans were approved by Comité d’éthique pour la recherche sur la personne des universités de Tours et de Poitiers (no. CER-TP 2022-01-01). The studies were conducted in accordance with the local legislation and institutional requirements and the ethical standards of the 1964 Declaration of Helsinki. Before the experiment, the children verbally assented to take part in the study, and their parents or legal guardians signed written informed consent forms.
Author contributions
CB-I, SF, VF, and CV contributed to conception and design of the study. CB-I, VF, and CV organized the database and performed the statistical analysis. CB-I and NV wrote the first draft of the manuscript. All authors contributed to the article and approved the submitted version.
Funding
The author(s) declare financial support was received for the research, authorship, and/or publication of this article. This research obtained the financial support of Institut Universitaire de France.
Acknowledgments
We thank all children and teachers who participated in the experiment and more particularly Laure Turi, national education inspector in Montmorillon for her help to contact the different schools. We also thank Yves Almecija for his help in the preparation of stimuli. The kindergarten schools of Montmorillon, Valdivienne, Tercé and Civaux participated in the project.
Conflict of interest
The authors declare that the research was conducted in the absence of any commercial or financial relationships that could be construed as a potential conflict of interest.
Publisher’s note
All claims expressed in this article are solely those of the authors and do not necessarily represent those of their affiliated organizations, or those of the publisher, the editors and the reviewers. Any product that may be evaluated in this article, or claim that may be made by its manufacturer, is not guaranteed or endorsed by the publisher.
Supplementary material
The Supplementary material for this article can be found online at: https://www.frontiersin.org/articles/10.3389/feduc.2023.1252731/full#supplementary-material
Footnotes
1. ^ https://osf.io/7xz8y/?view_only=df40a3b0b73e4331aea6e9744578d7ba
3. ^The d value was estimated with the website Psychometrica https://www.psychometrica.de/effect_size.html from the rank biserial correlation obtained with JASP.
References
Alla, F., Guillemin, F., Colombo, M. C., Roy, B., and Maeder, C. (1998). Valeur diagnostique de ERTL4: Un test de repérage des troubles du langage chez l’enfant de 4 ans. Arch. Pediatr. 5, 1082–1088. doi: 10.1016/S0929-693X(99)80004-9
Andres, M., Seron, X., and Olivier, E. (2007). Contribution of hand motor circuits to counting. J. Cogn. Neurosci. 19, 563–576. doi: 10.1162/jocn.2007.19.4.563
Badets, A., Bidet-Ildei, C., and Pesenti, M. (2015). Influence of biological kinematics on abstract concept processing. Quarterly Journal of Experimental Psychology (Hove) 68, 608–618. doi: 10.1080/17470218.2014.964737
Barrocas, R., Roesch, S., Gawrilow, C., and Moeller, K. (2020). Putting a finger on numerical development – reviewing the contributions of kindergarten finger gnosis and fine motor skills to numerical abilities. Front. Psychol. 11:1012. doi: 10.3389/fpsyg.2020.01012
Beauprez, S. A., and Bidet-Ildei, C. (2018). The kinematics, not the orientation, of an action influences language processing. J. Exp. Psychol. Hum. Percept. Perform. 44, 1712–1726. doi: 10.1037/xhp0000568
Berteletti, I., and Booth, J. R. (2016). “Chapter 5—finger representation and finger-based strategies in the Acquisition of Number Meaning and Arithmetic” in Development of mathematical cognition. eds. D. B. Berch, D. C. Geary, and K. M. Koepke (San Diego, USA: Academic Press), 109–139.
BOENJS (2021). “N° 25 École maternelle” in Ministère de l’Education Nationale et de la Jeunesse Available at:https://www.education.gouv.fr/bo/21/Hebdo25/MENE2116550A.htm
Brankaer, C., Ghesquière, P., and Smedt, B. D. (2014). Children’s mapping between non-symbolic and symbolic numerical magnitudes and its association with timed and untimed tests of mathematics achievement. PLoS One 9:e93565. doi: 10.1371/journal.pone.0093565
Castaldi, E., Piazza, M., and Iuculano, T. (2020). Learning disabilities: developmental dyscalculia. Handb. Clin. Neurol. 174, 61–75. doi: 10.1016/B978-0-444-64148-9.00005-3
Coello, Y., and Fischer, M. H. (2016). Foundations of embodied cognition: Perceptual and emotional embodiment. New York, NY, US: Routledge/Taylor & Francis Group.
Costa, A. J., Silva, J. B. L., Chagas, P. P., Krinzinger, H., Lonneman, J., Willmes, K., et al. (2011). A hand full of numbers: A role for offloading in arithmetics learning? Front. Psychol. 2:368. doi: 10.3389/fpsyg.2011.00368
Crollen, V., and Noël, M. -P. (2015). The role of fingers in the development of counting and arithmetic skills. Acta. Psychol. 156, 37–44. doi: 10.1016/j.actpsy.2015.01.007
Crollen, V., Seron, X., and Noël, M.-P. (2011). Is Finger-counting Necessary for the Development of Arithmetic Abilities? Front. Psychol. 2:242. doi: 10.3389/fpsyg.2011.00242
Decatoire, A., Beauprez, S. A., Pylouster, J., Lacouture, P., Blandin, Y., and Bidet-Ildei, C. (2019). PLAViMoP: how to standardize and simplify the use of point-light displays. Behav. Res. Methods 51, 2573–2596. doi: 10.3758/s13428-018-1112-x
Dehaene, S., and Cohen, L. (1995). Towards an anatomical and functional model of number processing. Math. Cogn. 1, 83–120.
Dehaene, S. (1992). Varieties of numerical abilities. Cognition 44, 1–42. doi: 10.1016/0010-0277(92)90049-N
Di Luca, S., and Pesenti, M. (2011). Finger numeral representations: more than just another symbolic code. Front. Psychol. 2:272. doi: 10.3389/fpsyg.2011.00272
Faul, F., Erdfelder, E., Buchner, A., and Lang, A. G. (2009). Statistical power analyses using G* power 3.1: tests for correlation and regression analyses. Behav. Res. Methods 41, 1149–1160. doi: 10.3758/BRM.41.4.1149
Furman, T., and Rubinsten, O. (2012). Symbolic and non symbolic numerical representation in adults with and without developmental dyscalculia. Behavioral and Brain Functions: BBF 8:55. doi: 10.1186/1744-9081-8-55
Gibson, D. J., Gunderson, E. A., Spaepen, E., Levine, S. C., and Goldin-Meadow, S. (2019). Number gestures predict learning of number words. Dev. Sci. 22:e12791. doi: 10.1111/desc.12791
Johansson, G. (1973). Visual perception of biological motion and a model for its analysis. Percept. Psychophys. 14, 201–211. doi: 10.3758/BF03212378
Klein, E., Bahnmuelller, J., Mann, A., Pixner, S., Kaufmann, L., Nuerk, H.-C., et al. (2013). Language influences on numerical development—inversion effects on multi-digit number processing. Front. Psychol. 4:480. doi: 10.3389/fpsyg.2013.00480
Klein, A., and Starkey, P. (1988). Universals in the development of early arithmetic cognition. New Dir. Child Dev. 1988, 5–26. doi: 10.1002/cd.23219884103
Kolkman, M. E., Kroesbergen, E. H., and Leseman, P. P. M. (2013). Early numerical development and the role of non-symbolic and symbolic skills. Learn. Instr. 25, 95–103. doi: 10.1016/j.learninstruc.2012.12.001
Lafay, A., Thevenot, C., Castel, C., and Fayol, M. (2013). The role of fingers in number processing in young children. Front. Psychol. 4:488. doi: 10.3389/fpsyg.2013.00488
Li, Y., Zhang, M., Chen, Y., Deng, Z., Zhu, X., and Yan, S. (2018). Children’s non-symbolic and symbolic numerical representations and their associations with mathematical ability. Front. Psychol. 9:1035. doi: 10.3389/fpsyg.2018.01035
Moeller, K., Fischer, U., Link, T., Wasner, M., Huber, S., Cress, U., et al. (2012). Learning and development of embodied numerosity. Cogn. Process. 13, 271–274. doi: 10.1007/s10339-012-0457-9
Montagner, H. (2012). Des enjeux majeurs pour les enfants et l’école. (Première partie). J du droit des jeunes 319, 36–42. doi: 10.3917/jdj.319.0036
Naglieri, J. A., and Brunnert, K. (2009). Wechsler nonverbal scale of ability (WNV) Practitioner’s guide to assessing intelligence and achievement. Hoboken, NJ, US: John Wiley & Sons Inc., 315–338.
Passolunghi, M. C., Mammarella, I. C., and Altoè, G. (2008). Cognitive abilities as precursors of the early Acquisition of Mathematical Skills during First through Second Grades. Dev. Neuropsychol. 33, 229–250. doi: 10.1080/87565640801982320
Proverbio, A. M., and Carminati, M. (2019). Finger-counting observation interferes with number processing. Neuropsychologia, 131, 275–284. doi: 10.1016/j.neuropsychologia.2019.06.001
Salminen, J., Khanolainen, D., Koponen, T., Torppa, M., and Lerkkanen, M.-K. (2021). Development of numeracy and literacy skills in early childhood—a longitudinal study on the roles of home environment and familial risk for Reading and math difficulties. Front Educ 6:725337. doi: 10.3389/feduc.2021.725337
Simon, M. A., Della Volpe, D., and Velamur, A. (2021). Construction of the cardinality principle through counting: critique and conjecture. Math. Think. Learn. 25, 465–480. doi: 10.1080/10986065.2021.2012737
Sixtus, E., Fischer, M. H., and Lindemann, O. (2017). Finger posing primes number comprehension. Cogn. Process. 18, 237–248. doi: 10.1007/s10339-017-0804-y
Sixtus, E., Krause, F., Lindemann, O., and Fischer, M. H. (2023). A sensorimotor perspective on numerical cognition. Trends Cogn. Sci. 27, 367–378. doi: 10.1016/j.tics.2023.01.002
Keywords: number meaning, fingers, action observation, point-light sequence, kinematics
Citation: Bidet-Ildei C, Vilain C, Fevin S, Francisco V and Vibert N (2024) The role of finger kinematics in the acquisition of number meaning in kindergarten: a pilot study. Front. Educ. 8:1252731. doi: 10.3389/feduc.2023.1252731
Edited by:
Marco Fabbri, University of Campania Luigi Vanvitelli, ItalyReviewed by:
Katarzyna Anna Bobrowicz, University of Luxembourg, LuxembourgGennaro Ruggiero, University of Campania Luigi Vanvitelli, Italy
Copyright © 2024 Bidet-Ildei, Vilain, Fevin, Francisco and Vibert. This is an open-access article distributed under the terms of the Creative Commons Attribution License (CC BY). The use, distribution or reproduction in other forums is permitted, provided the original author(s) and the copyright owner(s) are credited and that the original publication in this journal is cited, in accordance with accepted academic practice. No use, distribution or reproduction is permitted which does not comply with these terms.
*Correspondence: Christel Bidet-Ildei, christel.bidet@univ-poitiers.fr