- Institute for School Pedagogy and Didactics, Karlsruhe Institute of Technology (KIT), Karlsruhe, Germany
The article addresses the challenges faced by teachers incorporating digital tools into chemistry education to prepare students for responsible participation in a digital society. Against the background of the Technology Acceptance Model (TAM), the study analyzes the value that chemistry teachers place on digital tools and examines specific factors that influence their implementation in teaching. For this purpose, we conducted and analyzed interviews with 10 secondary school chemistry teachers in Germany. The findings revealed that while subject-specific digital tools were highly valued by teachers, several barriers to their strategic integration exist, including time constraints, high workloads, failing infrastructure, lack of technical support, and a fear of change. The study concludes that subject-specific digital tools have the potential to enhance learning outcomes and recommends teacher training and further education as well as future research to focus on developing and supporting opportunities for teachers to implement subject-specific digital tools to create a more dynamic and engaging learning experiences for students.
1. Introduction
Education in the 21st century has the task to prepare students for a responsible participation in our increasingly digital society (Vuorikari et al., 2016). This necessarily incorporates the confrontation with and implementation of digital tools in classrooms and presents the education systems and stakeholders therein with new and dynamic challenges. With massive disruptions within the educational systems induced by the COVID-19 pandemic, educators worldwide were forced to re-think teaching and implement digital tools to enable education, leading to fast-track digital transformation processes in many classrooms worldwide.
In chemistry education (CE), as part of science education in schools, the mere presence of digital tools does not automatically add value to the lessons. Overall, STEM subjects (science, technology, engineering, and mathematics) are highly relevant to society, as they are often at the center of innovation processes (Kennedy and Odell, 2014; Centre for the New Economy and Society, 2018). These subjects often require a high degree of abstraction and visualization, and digital tools could potentially facilitate the fulfillment of these requirements (Hrynevych et al., 2021). Digital tools can also help improve students’ understanding through interactive, multimedia learning content (Hillmayr et al., 2020). In addition, STEM subjects often require high competence in handling digital technologies, as many scientific processes have been digitized and data is often processed using computers (Walkowiak and Nehring, 2016). This means that the use of digital technologies in STEM subjects can be understood to prepare young people for the requirements of tomorrow’s job market (Centre for the New Economy and Society, 2018).
To foster this digital transformation within classrooms, teachers have been noted as crucial gatekeepers (Bridwell-Mitchell, 2015; Lockton and Fargason, 2019; Wohlfart and Wagner, 2022). They play a complex and vital role in digitalizing education, both as driving forces behind the process and as victims of its rapid pace. The COVID-19 pandemic has compelled many teachers to adopt distance teaching/learning methods out of necessity, resulting in heightened expectations for the use of digital technologies in education (Wohlfart, 2021). In CE, digital tools cannot replace technical language and experiments but can have a supporting and complementary role if they are meaningfully linked to content and tasks (Reiners, 2017; Braun et al., 2022). Previous studies discuss the opportunities and challenges of using digital tools in CE and emphasize the need for effective integration to enhance student learning (Jones, 2013; Libman and Huang, 2013; Bellou et al., 2018; Bernholt et al., 2018). Overall, mainly simulations, interactive whiteboards, augmented reality, digital textbooks and online collaboration tools have previously been highlighted as helpful in CE (Frailich et al., 2009; Moore et al., 2014; Bellou et al., 2018; Mazzuco et al., 2022).
Yet, so far it remains unclear, what value (if any) chemistry teachers place on such digital tools and which factors influence the implementation as part of their CE. The successful implementation of digital tools by teachers depends on a variety of factors, including access to the tools and time to explore them (Tondeur et al., 2012). Previous studies highlight teachers’ willingness and ability to integrate technology to further be influenced by their attitudes or personal fears (Wilson et al., 2020; Njiku, 2022). Additionally, exposure to a student-centered constructivist pedagogical approach during teacher education has been shown to have a positive effect on digital literacy development and integration of digital tools in teaching practice (Chai et al., 2013). Despite this research, there remains significant room for improvement in the formal incorporation of digital tools in CE across the globe.
Our study is based on an extended version of the widely recognized and extensively applied technology acceptance model (TAM) put forth by Davis (1986). This theoretical framework offers a valualble lens through which to better understand the factors influencing the utilization of digital tools in teaching chemistry. The core of the model consists of the variables “perceived usefulness” (PU) and “perceived ease-of-use” (PEOU). In addition, “attitudes towards using” (ATU) the technology are described as a direct product of these two variables in explaining “user motivation” for usage. However, it has been acknowledged that these three core variables fail to fully explain actual use due to a range of external factors that play into user acceptance (Davis, 1989; Bresler, 2016; cf. Figure 1).
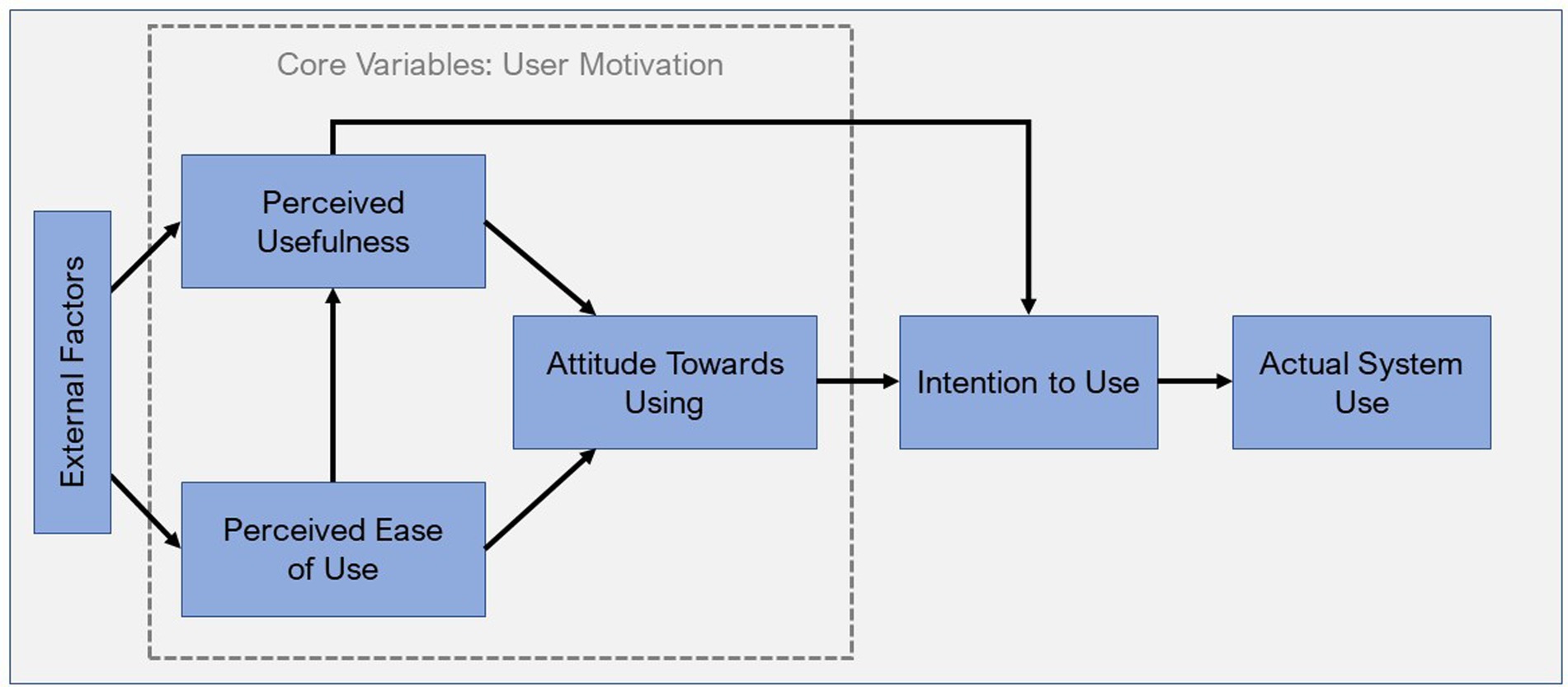
Figure 1. Technology acceptance model (own illustration based on Davis, 1989).
Previous studies have highlighted the effect of subjective standards (perception of how important others believe the use of technology is) or self-efficacy (one’s own ability to deal with technology) on acceptance and usage of digital tools in various educational contexts (Davis, 1989; Davis et al., 1989; Lee et al., 2003; Burton-Jones and Hubona, 2006; Bazelais et al., 2018). Though limited, the TAM has also been applied to investigate the use of digital tools in STEM subjects (Sarıtaş, 2015; Mayer and Girwidz, 2019): Mayer and Girwidz (2019) examined the link between the TAM and the widely known TPACK-Framework1 by Mishra and Koehler (2006) in physics teachers’ acceptance of multimedia applications. They found that TPACK is a key moderator variable that significantly influences the PEOU, PU for students, and personal job relevance assessment of multimedia applications for physics teaching. However, TPACK did not significantly impact the perceived personal usefulness of the technology in the examined sample. Sarıtaş (2015) used a mixed-methods approach to collect data from chemistry teacher candidates who participated in a molecular geometry course, focusing on the participants’ experiences and opinions about the use of virtual reality (VR) technology in the course. The study indicates that chemistry teacher candidates view the use of VR technology in teaching molecular geometry positively. However, cost and accessibility were highlighted as important factors to consider, and improvements are needed to make the technology more interactive and engaging for students. Overall, the literature lacks studies on the value of digital tools for CE.
Based on the TAM and current findings, we wanted to better understand the value which teachers place on and factors which influence subject-specific implementation of digital tools in CE, leading us to the following two research questions (RQ):
RQ 1: What value do teachers attribute to digital tools in chemistry education?
RQ 2: Which factors influence teachers’ acceptance and usage of digital tools in CE?
2. Method
To answer our research questions, we conducted an interview study in Germany, in the federal state of Baden-Wuerttemberg. This state’s chemistry education curriculum covers a diverse range of topics and activities spanning multiple grade levels (Ministerium für Kultus, Jugend und Sport [Ministry of Education, Youth and Sports] Baden-Württemberg, 2016). In secondary education schools (so-called “Gymnasien,” which are college-preparatory schools), chemistry is first introduced as a two-hour course in 8th grade, building upon the competencies acquired in BNT (biology, natural sciences, and technology) classes. In 11th grade, chemistry becomes a core subject with 3 h of instruction per week, and students may opt to take it as a 5-h advanced subject.
The federal state’s CE curriculum prioritizes an experimental culture, placing a strong emphasis on hands-on learning and experimentation. The use of digital media is also incorporated into the curriculum to aid teaching and learning, with measurement systems and simulation programs being used as tools. Furthermore, students are encouraged to leverage various media to showcase their work, thereby developing media competency skills. The curriculum recognizes the various functions of digital media in education, including its use as a learning tool, companion, experimental tool, or object of study (Voß, 2018).
2.1. Instrument
The findings of this study are based on a qualitative data analysis of semi-structured interviews. The semi-structured interview format allows for a detailed understanding of topics and social settings and, at the same time, provides a certain degree of flexibility in the interview process based on the background, experience, and status of the interviewees (Denzin and Lincoln, 2018). The interview guide consisted of seven main questions focusing the value, acceptance and usage of digital tools in chemistry class, with several in-depth and follow-up questions to guide the interviewer (Table 1 in Supplementary Material). In addition, we used a short questionnaire to obtain the socio-demographic information of the participants. The interviews were conducted by one of the authors in June and July, 2022 via videoconferences using the software Microsoft Teams. The interviews lasted between 35 and 71 min, were audio-recorded, and transcribed verbatim according to a specific transcription guideline based on the work of Dresing and Pehl (2019). Non-verbal signals were deliberately omitted to improve the readability of the transcripts. In addition, the transcripts were subjected to de facto anonymization to avoid tracing back individual statements to specific teachers. We replaced not only personal characteristics but also personal details with pseudo-information. We generated 142 pages of single-spaced transcribed text, and the total interview time was 434 min.
2.2. Participants
A purposeful sampling strategy, based on Patton's (2015) work, was employed in this study. We contacted teachers both individually as well as via an open call for participation distributed by the local school authority. With regards to the selection criteria for our research participants, we determined that teachers from Baden-Wuerttemberg who teach classes at secondary levels (with students aged 12 to 18 years old) would be included. Furthermore, we ensured that the teachers had different degrees of professional experience as well as workloads associated with extra-curricular activities at their respective schools such as departmental management or collaboration on a school media development plan commission. An overview of the 10 teachers interviewed is summarized below in Table 1; gender is represented by letter while number represents order of interviews conducted.
2.3. Data analysis
We performed a qualitative content analysis based on the study of Mayring (2022) for the 10 interview transcripts. As the advantages and possible barriers of implementing more general digital classroom tools have previously been presented and discussed to a high degree (cf. Bresler, 2016; Granić and Marangunić, 2019; Scherer et al., 2019; Wohlfart et al., 2021; Wohlfart and Wagner, 2022), our analysis focused subject-specific acceptance and usage of digital tools in CE. The authors read the transcripts repeatedly and coded specific segments using MAXQDA Analytics Pro 2022 as per deductive categories found through the literature review (e.g., perceived usefulness, tools applied, infrastructure, etc.) as well as inductive categories that emerged from the transcribed interview material (e.g., organization, difficulties, expectations, COVID-19 etc.). The qualitative data analysis resulted in 45 codes and 936 coded segments (divided into 13 main categories and 32 subcategories). To ensure trustworthiness and rigor in our qualitative analysis, we implemented several strategies to enhance the reliability of our coding scheme and maintain consistency across the dataset. Coders underwent comprehensive training sessions to familiarize themselves with the research objectives, theoretical framework, and coding guidelines. Regular discussions and consensus meetings were held to resolve coding discrepancies, while detailed documentation, including coding manuals and memos within the MAXQDA file, was maintained throughout the coding process. Table 2 in the Supplementary Material provides an exemplary overview of the main category “added value,” its accompanying subcategories as well as associated definitions and anchor examples.
We selected quotes from the interview transcripts to illustrate the findings and interpretations related to our research questions. All interviews were conducted in German, with subsequent translation of selected quotes into English. We then critically reflected on these translations to ensure that participants’ voices were maintained and possible misunderstandings avoided (Denzin and Lincoln, 2018).
3. Findings
In this section, we present the findings obtained through our interviews with the teachers regarding their experiences with using digital tools for teaching in CE. These have been divided into two sections: First, we present the teachers’ perceived value of digital tools (PEOU, PU, and ATU) in terms of how it affects technology acceptance based on the TAM (Davis, 1986; RQ 1). Second, we illustrate influential factors that affected the acceptance and usage of digital tools in our sample (RQ 2).
3.1. Attitude towards and value of digital tools in CE
While all teachers interviewed indicated implementing some form of digital tools in their teaching, the mode of implementation varied a great deal. Most often, the interviewed teachers made use of digital learning platforms (e.g., Ilias, Moodle, MS Teams etc.) and Apps (e.g., Padlet, Messenger, ZUMPad) to support the organization of teaching materials and (structured) communication with students before, during and after class. In addition, the teachers indicated benefits of utilizing various versions of digital white boards, laptops and tablets, visualizers and document cameras in their classes. Surprisingly, not all interview-partners made use of subject-specific digital tools. Four teachers elaborated on the use of digital data acquisition programs (M01, M05, M09, and M10) and molecule drawing programs such as ChemSketch (M09 and M10). In addition, several interviewees specified having previously integrated and/or produced videos or animations to support or facilitate content transfer (W03, M01, M05, and M09).
Overall, user motivation is explained and depicted along the three core variables of the TAM to depict user motivation: PU (3.1.1), PEOU (3.1.2), and ATU (3.1.3).
3.1.1. Perceived usefulness
The interviewees highlighted several advantages of digital tools for CE which we identified as PU. The biggest strength seems to lie in the potential of being able to (better) illustrate complex or abstract learning content. Simulations, animations and the visualization of complex molecules or reactions can make the content more “tangible” for students (W02 and M05). Within this context, the interview partners spoke about showing and integrating videos, learning apps and augmented reality (AR). Specifically, animations can depict the three-dimensional atoms and processes better than a “sheet of paper” (M07 and M08). In addition, these applications “open up new possibilities that were not possible before […]” (M05). One interview partner explained his perspective on AR as follows:
“[…] with AR you could at least show a little bit better the three-dimensionality of the atoms, molecules… why the water molecule is angled for example, even orbital models. […] Of course, you could also work with other models, but the models are somehow then again very theoretical to understand and […] AR is already existent to present these models directly in front of your eyes” (M08).
This illustrative usefulness of digital tools was further highlighted in the context specifically with experiments and associated protocols (M09). In addition, PU was identified from digital tools as an extension of analog media, as videos of experiments can be shown which would be dangerous in the classroom (M07) or too time-consuming to set up (M08). In addition, the chemical processes are better visualized in videos making the outcome more comprehensive and accessible (M07). Next to this illustration, videos enable a transfer of knowledge from technology and industrial processes such as “the blast furnace process” (M10) to mechanisms of everyday life. On the other hand, the interview partners did not find digital tools sensible as an extension to analog media in student experiments. From M08’s point of view, the reason is that the tools “cannot be used in such a meaningful way” (M08) or the technology is only compatible with computers and certain programs (M01).
A further, pragmatic benefit of tools identified was the associated flexibility and spontaneity: adding on or completing write-ups with digital tools such as document cameras (M01) or taking photos or videos of everyday objects or substances and their ingredients [e.g., salt (M04)] and incorporating these into the lessons. In addition, one teacher highlighted opportunities for self-regulated learning in the context of solving technical problems of new programs with program tutorials and learning to apply digital measurement systems early on in secondary education (M10). Finally, conserving resources and promoting sustainability was mentioned by several interview partners (M01, M04, and M10). As one teacher summarized:
“CE often incorporates learning circles which you print out, then laminate, then you realize you have changed something, then you print it out again, laminate it again, and produce garbage. Today, all these learning circles end up on Moodle, if necessary also with an explanatory video” (M10).
3.1.2. Perceived ease-of-use
Overall, the analysis of the interviews indicated moderate usage of subject-specific digital tools in CE. General tools such as integrating videos (e.g., via YouTube), learning apps (e.g., Kahoot, Learningsnacks), digital learning platforms (e.g., moodle) and digital boards (e.g., smart boards, Apple TV) were mentioned to be simple, quick and/or intuitive (M01, M05). Challenges in this context were perceived in association with group collaboration (M01). Subject-specific digital tools, meanwhile, were often associated with increased time expenditure (M01, M04, M05) and the lack of exchange and/or collaboration with colleagues (M04). As one teacher explained this distinction:
“But as soon as it becomes a bit more demanding, then I really need support. And we still have far too few people who maintain the equipment, who have the responsibility, and who also receive credit for this” (M05).
All in all, the teachers seldomly mentioned specific technological challenges (e.g., need to re-calibrate instruments – W03) in implementing subject-specific digital tools in CE. When asked about their PEOU, they often answered evasive and focused on the implementation of general digital tools for communication and didactics. For several interview partners, the beginning or introduction of digital tools was the biggest challenge (M01 and W06). Once acquainted with specific tools, the interview partners explained these to be quite simple and referred to external factors (e.g., lack of infrastructure such as WIFI or mobile devices) as reasons to not implement the same (M07 and M09). Analogously, functionality was highlighted to be more important than “perfect technical implementation” (W03).
3.1.3. Attitude towards usage
The interviewed teachers’ attitudes towards digital tools were very different individually. We identified positive attitudes as “it […] is actually also the trend of the times (M01)” and “digital tools […] are part of modern teaching” (M09). In addition, digital tools can “simplify [everyday teaching]” (M08), because “when you have familiarized yourself with them, you ultimately have less work” (M07). One teacher highlighted digital tools to make more work, but “it pays off” (M10). Time, on the other hand, is seen as a precious resource and often impedes the transition to teaching with digital tools (M05, M09, and W06). This is especially the case, the more complex the digital tools become. Furthermore, we identified an inhibiting factor concerning the ATU highlighting the need to conduct experiments live and “for real” (M08). In this sense, “Digitization should not substitute practical work” (M03) but support the same (M05 and W02). ATU of digital tools is expressed as being reliant on an “added value for the lessons and within a time-benefit relationship” (W02). Overall, the analysis indicated a pragmatic, functional and minimalistic attitude towards digital tools in CE among the sample, as illustrated by one teacher:
“…I’m not interested in the technology. Only to the extent that I must use it so that it works in didactics, in teaching. […] I do not feel like solving any technical problems. But we are pretty busy with that. Now, it’s basics like Apple TVs, which simply do not work” (M05).
3.2. Influential factors
We observed various external factors (RQ 2) that affected the acceptance and usage of subject-specific digital tools in CE, both directly and indirectly. We have structured our findings into three sections focusing on time and workload, infrastructure and support, as well as life-long-learning.
3.2.1. Time and workload
As mentioned above, time (or lack thereof more specifically) was repeatedly identified as a barrier towards acceptance and usage of digital tools in the analysis of the interviews (M04, M05, M09, and W06). This was often linked to an extremely high workload to (learn how to) integrate digital tools (in a meaningful way). One teacher claimed:
“Well, even for a 10-min test, you work for a few hours until you have everything together. So […] you just do not do that all the time, because that’s also a lot of work” (M01).
When students are not fully equipped with tablets, the option to work with tablet cases was referenced as unappealing and a further time expenditure of “booking tablets, collecting tablets, returning tablets […]” (W06). The integration of subject-specific, measuring equipment set-ups, meanwhile, was described to be “unrealizable” (M08) and demands an intense preparation which was considered “additional workload” (M10).
On the other hand, time was also mentioned in offering new opportunities in connection to digital tools: though time-consuming in the first creation, the generated products (i.e., worksheets, videos) can repeatedly and variably be reproduced in the following lessons and years of teaching (W03, M07, M08). The statement of another teacher contradicted this explicitly:
“Then all the work might have been for naught for next year, because who knows if I’ll teach the subject again in the same way” (W06).
On a more subject-specific level, digital tools such as videos or apps for visualization of molecules were highlighted to save valuable time if time constraints do not allow individual construction via molecule construction kits (W02).
3.2.2. Infrastructure and support
Technological infrastructure (WIFI, digital devices) was consistently demanded as a foundation for the acceptance and usage of any digital tool in CE among the interviewed teachers. Several interviewed teachers (M04, M08, and M10) highlighted the catalyzing effect of the COVID-19 pandemic and accompanying development of technological infrastructure of the schools. Resulting from this, new technical equipment was seen to enable new projects into lessons – such as measuring the conductivity or pH value with a Mobile-CASSY measurement device (M10). One limitation in connection to the equipment lies in the availability for teachers. One teacher explains:
“[…] the equipment I have at home I have to buy myself. I do not get it from the school. So it depends on how well or how poorly equipped I am” (M01).
In addition, the interviewed teachers often wished for more (technical) support in applying digital tools in their classes. This ranged from the wish for specific persons in charge at school (M05 and M07), better/functioning infrastructure and programs (M05), moral support (M07), to subject-specific platforms with digital teaching material (M09). A need was also identified in the availability of material adapted to classrooms, e.g., from publishers (W02, M04, and W06). Here, material on AR and VR was mentioned to be most promising (M08 and M09).
Next, to the above factors, the COVID-19 pandemic as well as current data protection requirements influence the acceptance and usage of digital tools within the interviewed sample. In the context of the COVID-19 pandemic, teachers in the sample were either unable to draw a comparison with the time before the pandemic because they had not yet taught regularly themselves (W02, M04, W06, and M08) or the necessary equipment such as robust WIFI or learning platforms was not available (M01, M09, and M10). Teachers, with more than 5 years of teaching experience, indicated that they use more digital tools than before the pandemic (M01, W03, M05, M07, and M09).
Data protection requirements, meanwhile, were declared as a limiting, restricting factor in implementing digital tools (M01) – though this was only mentioned by one teacher and in connection to distance learning in the course of the school closing during the COVID-19 pandemic.
3.2.3. Life-long-learning
A further influential factor we identified focused aspects of what we declared “life-long-learning,” including comments on continuing education, collaboration with colleagues, and learning from students.
The link to continuing education formats was quite individual in the interviewed sample, ranging from no known training opportunities (W02 and W06), across participation within internal or external training opportunities (general: M04, STEM-specific: M08, technology-specific (H5P): M01) to giving training (M07). In this sense, teachers commented being more apt to invest time in their training for specific digital tools for CE and complained of a lack of “subject-specific” training (M07 and M09) and the absence of “realistic scenarios” (M08).
Rather than partaking in specific trainings, some interviewees highlighted their willingness and ability for “autodidactics” (M09) and “learning-by-doing” (M10), finding support for this amongst colleagues. One teacher highlights this as follows:
“And my best source of continuing education is my colleagues as consultants, my seminar colleagues. So what they always contribute when we meet at the College, there are an incredible number of ideas. And, also the young trainee teachers. The assignments they prepare, the things they try out with their students, that’s always a source of ideas, too” (M09).
The exchange within the teaching staff takes place in particular between younger teachers (M04, M07), pre-service teachers (M09) or a “colleague who does all the media training at the seminar [pre-service teacher training institution]” (M10). Exchange occurs among teachers when sharing material, when assignments, classwork or worksheets are exchanged via the “school cloud” (M10, M07, and M01). They also talk about useful (subject-specific) apps (M04 and M08) and create or edit videos together during joint projects (M05 and M08). In contrast, four teachers at the school have no or hardly any exchange between teachers (W02, W06, and M08) or the exchange is “not really specifically promoted” (M05). One teacher further highlighted opportunities of an exchange with students when using digital tools, because they “support the teacher […] when stuck at some point “(M01).
4. Discussion of findings
In the previous sections, we summarized the findings from the analysis of our interviews along the variables of the TAM. In this section, we first integrate the individual findings to better understand the actual (desired) output – implementation of digital tools in CE (4.1). We discuss these findings in relation to previous studies and their respective outcomes. Based on this discussion, we then derive opportunities of subject-specific digital tools as an added value for CE (4.2). Finally, we present and discuss limitations of the study (4.3).
4.1. Acceptance vs. usage
Research has repeatedly shown and highlighted the need to consider the (partly conflicting) effects of internal and external factors as well as their correlation to motivational variables in understanding the difference between intent to integrate technology vs. actual integration (Lee et al., 2003; Scherer et al., 2019; Wohlfart, 2021). Based on our findings, this seems to be even more the case when analyzing perspectives on subject-specific digital tools for CE.
Overall, the interviewed teachers accept and implement general digital tools for communication and organization purposes in CE. This changes drastically when examining the implementation of subject-specific digital tools. Surprisingly, only four of the interviewed teachers reported to currently make use of digital data acquisition and molecular drawing programs in their CE. This is contrasted by current literature which theoretically derive the added value of more accurate measurement values and representation (Pietzner and Wagner, 2018; Kastaun and Meier, 2021; Wejner and Wilke, 2022). In addition, current research promotes the use of UV–VIS spectrometer, photometer, or thermal imaging camera, showcasing the numerous implementation possibilities in CE (Schrader and Schanze, 2021; Bohrmann-Linde et al., 2022; Jurinovich and Domenici, 2022).
While the PU of these digital tools was confirmed by most interview partners, specific barriers (motivational and external) seem to hinder the teachers in a strategic integration of the same. Previous studies have highlighted other factors influencing technology acceptance such as fear of technology (Lockton and Fargason, 2019; Bürger et al., 2021). Analogously, we mapped a fear of change in the context of digital tools replacing hands-on work in experiments. This aligns with skeptical ATU and results in avoidance behavior of these teachers towards the implementation of subject-specific digital tools. Rather than replacing experiments, however, we expect digital laboratories to facilitate and support real laboratories in the long run, emphasizing the need to highlight the PU of these subject-specific digital tools as an added value to teaching (Wörner et al., 2021). The accompanying need for better technical support in implementing digital tools in education has also been highlighted in previous studies and furthers the divide between acceptance of and actual implementation of subject-specific digital tools (Schmid et al., 2017; Dittmar and Eilks, 2019).
In addition, lack of systematic training hinders the implementation of subject-specific digital tools. Based on the value of these as stated by the interviewed teachers, we would expect a higher intrinsic motivation for professional development. With this in mind, we conclude the perceived value – as mapped via the core TAM variables – and thus resulting motivation to implement subject-specific tools to not be strong enough for a large part of the interviewed sample. Several specific factors influenced the intended and actual implementation of the same. For our sample, lack of time on top of the teaching load in classrooms seems to be the greatest barrier for a sustainable confrontation and accompanying implementation of subject-specific digital tools in CE. This finding is particularly problematic as it is the declared mission of general education to prepare students for a digital world (Vuorikari et al., 2016), exceeding the mere consumption of digital tools in education.
While our sample showed no connection between teaching experience and age as factors influencing acceptance and usage of ICT, previous research has painted an inconsistent picture of this connection. While Guo et al. (2008) also reported age to have no significant influence on the use of and attitude toward digital tools, Dittmar and Eilks (2019) highlighted younger teachers to have shown higher acceptance and more implementation ideas.
Overall, with the TAM as an explanatory model, systemized factors are increasingly influential. In our sample, the factors infrastructure, self-initiative for familiarization and exchange, support by colleagues, and continuing education were identified as crucial for the acceptance and usage of subject-specific digital tools in CE. Time constraints, posed the biggest hindering factor of implementation, causing a contradiction between acceptance, on the one hand, and usage of subject-specific digital tools, on the other hand. While some teachers in the sample highlighted the added value of subject-specific digital tools, others were more reserved towards these as a necessary part of “modern teaching” (M09). The findings highlight the need for stakeholders within didactics of chemistry to critically reflect and modernize both teacher training and continuing professional development to sustainably support pre-service and in-service teachers in getting acquainted with and realizing the added value of subject-specific digital tools for CE. Analogously, if we want teachers to be successful in preparing students for a digital world, we must necessarily transform the framework conditions of the teaching profession to adequately meet the needs and enable a transformation process of the same (Wohlfart and Wagner, 2022).
4.2. Added value for chemistry education and future research
Concluding, we want to take a first step towards this transformation and offer insights on opportunities of subject-specific digital tools as an added value in CE based on the TAM and our findings as well as previous research.
1. VR, AR and 360° Videos: More than just a modern gimmick, the use of VR and AR technologies in CE has the potential to transform how students perceive and interact with chemical concepts (Tauber et al., 2022). Future research should focus on developing immersive VR and AR tools that allow students to visualize and interact with chemical structures and processes in a more dynamic and engaging way. However, developing these realities on one’s own requires program expertise. In contrast, 360° videos offer the first low-threshold opportunities for immersive teaching and learning experiences in a video format that is easy to create (Rosendahl and Wagner, 2023). Additionally, 360° videos can be utilized to promote interest and visualization of laboratory safety and sustainability. By recording experiments and laboratory work in a realistic setting, students can investigate and analyze laboratory procedures and safety protocols. This approach can also contribute to a more sustainable use of resources by reducing the number of experiments performed in the laboratory (Tauber et al., 2022).
2. Simulations and Modeling: Simulations and modeling tools have long been an important part of CE. As highlighted by various teachers in the interviews, these have vast potential in visualization of complex processes and thereby supporting student learning outcomes (Hillmayr et al., 2020). Artificial intelligence and machine learning algorithms promise dynamic development in this area, most likely improving the accuracy and realism of chemical simulations in the near future.
3. Data Analysis and Visualization: The analysis and visualization of data is an important aspect of CE. Paired with the intuitive access of students today, subject-specific tools such as Mobile-CASSY (M10) can help students more effectively analyze and visualize chemical data (Walkowiak and Nehring, 2016; Hrynevych et al., 2021). For example, researchers could explore the use of interactive data visualization tools that allow students to explore chemical data sets in a more intuitive and engaging way (Hillmayr et al., 2020).
In conclusion, future research in CE should focus on developing and refining subject-specific digital tools that can enhance learning outcomes and facilitate deeper engagement with the fundamental principles of chemistry. By leveraging the power of digital technology, educators and researchers can create a more dynamic and engaging learning experience that prepares students for success in the rapidly evolving world (Kennedy and Odell, 2014).
4.3. Limitations
Despite our efforts in selecting a diverse group, we cannot dismiss the possibility of self-selection bias in our limited, regional sample of chemistry teachers. Consequently, there may be social inclination towards teachers who hold positive views on digital tools as opposed to those with negative attitudes since they might not have volunteered for this study that focuses on their acceptance and usage. Furthermore, it is important to acknowledge that our study carries the inherent risk of eliciting socially desired responses from participants (Lavidas et al., 2022). By assuring the confidentiality and anonymity of our participants, we aimed to create a sense of security and reduce concerns about potential social judgment or repercussions. Additionally, we employed a rigorous and comprehensive interview protocol that included open-ended questions and prompts designed to encourage participants to reflect deeply on their experiences. While we acknowledge that a larger sample size might have provided additional perspectives and potentially expanded the breadth of the findings, the aim of our study was not to achieve exhaustive coverage but rather to provide a detailed examination of the investigated issues. We understand our findings as a nuanced exploration that offers valuable insights, albeit within the context of our selected sample. In addition, as the goal of analyzing and increasing the usage of digital tools in education is to prepare students for the digital world, actual student performance and assessment, rather than teachers’ attitudes and assessments, should be analyzed and considered in a next step.
5. Conclusion
The purpose of this study was to gain a greater understanding of the value that chemistry teachers place on digital tools as part of their CE as well as examining the factors which influence subject-specific implementation of digital tools in their teaching. For this purpose, we conducted 10 semi-structured interviews with secondary school chemistry teachers and analyzed the transcripts based on the theoretical framework of the TAM.
We found drastic differences between the acceptance and the usage of general digital tools and subject-specific digital tools for CE. While our findings confirmed the PU of these subject-specific tools, specific barriers (motivational and external) seem to hinder the teachers in a strategic integration of the same. Meanwhile, the reported PEOU and positive ATU of most interviewees did not support the limited acceptance and usage of these tools. Rather, we found several influential factors which strongly affected the actual implementation. Mainly, the interviewed sample complained of time constraints and high workloads, failing infrastructure and lack of (technical) support as well as professional development opportunities for subject-specific digital tools in CE. A fear of change was also identified to impede the transformation of digital tools in CE.
In conclusion, the study suggests that subject-specific digital tools have the potential to enhance learning outcomes and facilitate deeper engagement with the principles of chemistry. Therefore, teacher preparation and further development as well as future research in CE should focus on developing and refining subject-specific digital tools to create a more dynamic and engaging learning experience for students. The study highlights the added value of digital tools in CE, rather than being seen as mere modern gimmicks.
Data availability statement
The raw data supporting the conclusions of this article will be made available by the authors, without undue reservation.
Ethics statement
Ethical review and approval was not required for the study on human participants in accordance with the local legislation and institutional requirements. The patients/participants provided their written informed consent to participate in this study.
Author contributions
OW, AW, and IW contributed to conception and design of the study. AW and OW organized the data and performed the data analysis. OW wrote the first draft of the manuscript. All authors contributed to the article and approved the submitted version.
Funding
The writing of this article and the underlying study were supported, in part, by the Vector Foundation as well as through the funding of the project “digiMINT,” which is a part of the Qualitätsoffensive Lehrerbildung, a joint initiative of the Federal Government and the Länder that aims to improve the quality of teacher training. The program is funded by the Federal Ministry of Education and Research. The authors are responsible for the content of this publication. We acknowledge support by the KITPublication Fund of the Karlsruhe Institute of Technology.
Conflict of interest
The authors declare that the research was conducted in the absence of any commercial or financial relationships that could be construed as a potential conflict of interest.
Publisher’s note
All claims expressed in this article are solely those of the authors and do not necessarily represent those of their affiliated organizations, or those of the publisher, the editors and the reviewers. Any product that may be evaluated in this article, or claim that may be made by its manufacturer, is not guaranteed or endorsed by the publisher.
Supplementary material
The Supplementary material for this article can be found online at: https://www.frontiersin.org/articles/10.3389/feduc.2023.1197296/full#supplementary-material
Supplementary Table 1 | Interview guide.
Supplementary Table 2 | Exemplary overview of the main category “added value” and accompanying subcategories of the interviews as well as associated definitions and anchor examples.
Footnotes
1. ^The Technological Pedagogical Content Knowledge (TPACK) framework, developed by Mishra and Koehler (2006), is a theoretical framework that explains the complex interplay between technology, pedagogy, and content knowledge in teaching and learning. The framework highlights the importance of teachers’ understanding and integration of these three domains in the design and delivery of effective instruction. TPACK emphasizes the need for teachers to possess knowledge and skills that enable them to select, use, and integrate technology appropriately and effectively in their teaching, while also considering the content being taught and the pedagogical strategies that best support student learning.
References
Bazelais, P., Doleck, T., and Lemay, D. J. (2018). Investigating the predictive power of TAM: a case study of CEGEP students’ intentions to use online learning technologies. Educ. Inf. Technol. 23, 93–111. doi: 10.1007/s10639-017-9587-0
Bellou, I., Papachristos, N. M., and Mikropoulos, T. A. (2018). “Digital learning Technologies in Chemistry Education: a review” in Digital technologies: Sustainable innovations for improving teaching and learning. eds. D. Sampson, D. Ifenthaler, J. M. Spector, and P. Isaías (Cham: Springer International Publishing), 57–80.
Bernholt, S., Broman, K., Siebert, S., and Parchmann, I. (2018). Digitising teaching and learning – additional perspectives for chemistry education. Isr. J. Chem. 59, 554–564. doi: 10.1002/ijch.201800090
Bohrmann-Linde, C., Meuter, N., Zeller, D., and Tausch, M. W. (2022). Teaching photochemistry: experimental approaches and digital media. ChemPhotoChem 6:e202100141. doi: 10.1002/cptc.202100141
Braun, I., Langner, A., and Graulich, N. (2022). Let’s draw molecules: students’ sequential drawing processes of resonance structures in organic chemistry. Front. Educ. 7:1055280. doi: 10.3389/feduc.2022.1055280
Bridwell-Mitchell, E. N. (2015). Theorizing teacher agency and reform. Sociol. Educ. 88, 140–159. doi: 10.1177/0038040715575559
Bürger, N., Haselmann, S., Baumgart, J., Prinz, G., Girnat, B., Meisert, A., et al. (2021). Jenseits von Professionswissen: Eine systematische Überblicksarbeit zu einstellungs- und motivationsbezogenen Einflussfaktoren auf die Nutzung digitaler Technologien im Unterricht [beyond professional knowledge: a systematic review of attitudinal and motivational factors influencing the use of digital technologies in teaching]. J. Educ. Sci. 24, 1087–1112. doi: 10.1007/s11618-021-01050-3
Burton-Jones, A., and Hubona, G. S. (2006). The mediation of external variables in the technology acceptance model. Inf. Manag. 43, 706–717. doi: 10.1016/j.im.2006.03.007
Centre for the New Economy and Society (2018). The future of jobs report 2018. Cologny/Geneva: World Economic Forum.
Chai, C. S., Koh, J. H., and Tai, C. C. (2013). A review of technological pedagogical content knowledge. Educ. Technol. Soc. 16, 31–51.
Davis, F. D. (1986). A technology acceptance model for empirically testing new end-user information systems: Theory and results. [PhD]. [Cambridge, Mass.]: Massachusetts Institute of Technology.
Davis, F. D. (1989). Perceived usefulness, perceived ease of use, and user acceptance of information technology. MIS Q. 13:319. doi: 10.2307/249008
Davis, F. D., Bagozzi, R. P., and Warshaw, P. R. (1989). User acceptance of computer technology: a comparison of two theoretical models. Manag. Sci. 35, 982–1003. doi: 10.1287/mnsc.35.8.982
Denzin, N. K., and Lincoln, Y. S., eds. (2018). The SAGE handbook of qualitative research. Los Angeles, London, New Delhi, Singapore, Washington DC, Melbourne: SAGE.
Dittmar, J., and Eilks, I. (2019). An interview study of German teachers’ views on the implementation of digital media education by focusing on internet forums in the science classroom. Int. J. Educ. Math. Sci. Technol. 7, 367–381.
Dresing, T., and Pehl, T. (2019). “Transkription [transcription]” in Handbuch qualitative Forschung in der Psychologie [handbook of qualitative research in psychology]. eds. G. Mey and K. Mruck (Wiesbaden: Springer Fachmedien Wiesbaden), 1–20.
Frailich, M., Kesner, M., and Hofstein, A. (2009). Enhancing students’ understanding of the concept of chemical bonding by using activities provided on an interactive website. J. Res. Sci. Teach. 46, 289–310. doi: 10.1002/tea.20278
Granić, A., and Marangunić, N. (2019). Technology acceptance model in educational context: a systematic literature review. Br. J. Educ. Technol. 50, 2572–2593. doi: 10.1111/bjet.12864
Guo, R. X., Dobson, T., and Petrina, S. (2008). Digital natives, digital immigrants: an analysis of age and Ict competency in teacher education. J. Educ. Comput. Res. 38, 235–254. doi: 10.2190/EC.38.3.a
Hillmayr, D., Ziernwald, L., Reinhold, F., Hofer, S. I., and Reiss, K. M. (2020). The potential of digital tools to enhance mathematics and science learning in secondary schools: a context-specific meta-analysis. Comput. Educ. 153:103897. doi: 10.1016/j.compedu.2020.103897
Hrynevych, L., Morze, N., Vember, V., and Boiko, M. (2021). Use of digital tools as a component of STEM education ecosystem. Educ. Technol. Q. 2021, 118–139. doi: 10.55056/etq.24
Jones, L. L. (2013). How multimedia-based learning and molecular visualization change the landscape of chemical education research. J. Chem. Educ. 90, 1571–1576. doi: 10.1021/ed4001206
Jurinovich, S., and Domenici, V. (2022). Digital tool for the analysis of UV–Vis spectra of olive oils and educational activities with high school and undergraduate students. J. Chem. Educ. 99, 787–798. doi: 10.1021/acs.jchemed.1c01015
Kastaun, M., and Meier, M. (2021). Digitale Messwerterfassung in der Umweltanalyse [Digital data acquisition in environmental analysis]. In J. Meßinger-Koppelt & J. Maxton-Küchenmeister (Eds.), Naturwissenschaften digital: Toolbox für den Unterricht [Natural sciences digital: Toolbox for the classroom]: Band 2 (1st ed.,). Joachim Herz Stiftung. 64–67.
Kennedy, T. J., and Odell, M. R. L. (2014). Engaging students in STEM education. Sci. Educ. Int. 25, 246–258.
Lavidas, K., Papadakis, S., Manesis, D., Grigoriadou, A., and Gialamas, V. (2022). The effect of social desirability on students’ self-reports in two social contexts: lectures vs. lectures and lab classes. Information 13:491. doi: 10.3390/info13100491
Lee, Y., Kozar, K. A., and Larsen, K. R. (2003). The technology acceptance model: past, present, and future. Commun. Assoc. Inf. Syst. 12, doi: 10.17705/1CAIS.01250
Libman, D., and Huang, L. (2013). Chemistry on the go: review of chemistry apps on smartphones. J. Chem. Educ. 90, 320–325. doi: 10.1021/ed300329e
Lockton, M., and Fargason, S. (2019). Disrupting the status quo: how teachers grapple with reforms that compete with long-standing educational views. J. Educ. Chang. 20, 469–494. doi: 10.1007/s10833-019-09351-5
Mayer, P., and Girwidz, R. (2019). Physics teachers’ acceptance of multimedia applications—adaptation of the technology acceptance model to investigate the influence of TPACK on physics teachers’ acceptance behavior of multimedia applications. Front. Educ. 4:73. doi: 10.3389/feduc.2019.00073
Mayring, P. (2022). Qualitative Inhaltsanalyse: Grundlagen und Techniken [qualitative content analysis: Fundamentals and techniques]. Weinheim, Basel: Beltz.
Mazzuco, A., Krassmann, A. L., Reategui, E., and Gomes, R. S. (2022). A systematic review of augmented reality in chemistry education. Rev. Educ. 10:e3325. doi: 10.1002/rev3.3325
Ministerium für Kultus, Jugend und Sport [Ministry of Education, Youth and Sports] Baden-Württemberg . (2016). Bildungsplan 2016 - Chemie [Education plan 2016 - Chemistry].
Mishra, P., and Koehler, M. J. (2006). Technological pedagogical content knowledge: a framework for teacher knowledge. Teach. Coll. Rec. 108, 1017–1054. doi: 10.1111/j.1467-9620.2006.00684.x
Moore, E. B., Chamberlain, J. M., Parson, R., and Perkins, K. K. (2014). PhET interactive simulations: transformative tools for teaching chemistry. J. Chem. Educ. 91, 1191–1197. doi: 10.1021/ed4005084
Njiku, J. (2022). Attitude and technological pedagogical and content knowledge: the reciprocal predictors? J. Res. Technol. Educ. 1–16, 1–16. doi: 10.1080/15391523.2022.2089409
Patton, M. Q. (2015). Qualitative research & evaluation methods: Integrating theory and practice. Los Angeles, London, New Delhi, Singapore, Washington DC: SAGE.
Pietzner, V., and Wagner, W. (2018). Klassische und digitale Medien im Chemieunterricht [Traditional and digital tools in the chemistry classroom]. In Unterricht Chemie [Teaching Chemistry]. Konkrete Fachdidaktik Chemie [Concrete didactics of chemistry]: Grundlagen für das Lernen und Lehren im Chemieunterricht [Fundamentals for learning and teaching in chemistry classes]. eds. K. A. Sommer, J. Wambach-Laicher, and P. Pfeifer (1st ed.,). (Seelze: Friedrich Aulis), 557–600.
Reiners, C. S. (2017). Chemie vermitteln [Teaching Chemistry]. Berlin, Heidelberg: Springer Berlin Heidelberg.
Rosendahl, P., and Wagner, I. (2023). 360° videos in education – a systematic literature review on application areas and future potentials. Educ. Inf. Technol. doi: 10.1007/s10639-022-11549-9
Sarıtaş, M. T. (2015). Chemistry teacher candidates acceptance and opinions about virtual reality technology for molecular geometry. Educ. Res. Rev. 10, 2745–2757. doi: 10.5897/ERR2015.2525
Scherer, R., Siddiq, F., and Tondeur, J. (2019). The technology acceptance model (TAM): a meta-analytic structural equation modeling approach to explaining teachers’ adoption of digital technology in education. Comput. Educ. 128, 13–35. doi: 10.1016/j.compedu.2018.09.009
Schmid, U., Goertz, L., Behrens, J., and Stiftung, B. (2017). Monitor Digitale Bildung: Die Schulen im digitalen Zeitalter [Digital education monitor: Schools in the digital age]. Gütersloh: BStift - Bertelsmann Stiftung.
Schrader, F., and Schanze, S. (2021). “Zielorientierter Einsatz der Wärmebildkamera im Chemieunterricht [target-oriented use of the thermal imaging camera in chemistry lessons]” in Naturwissenschaften digital: Toolbox für den Unterricht [natural sciences digital: Toolbox for the classroom]. eds. J. Meßinger-Koppelt and J. Maxton-Küchenmeister, vol. Band 2 (Hamburg: Joachim Herz Stiftung), 28–31.
Tauber, A. L., Levonis, S. M., and Schweiker, S. S. (2022). Gamified virtual laboratory experience for in-person and distance students. J. Chem. Educ. 99, 1183–1189. doi: 10.1021/acs.jchemed.1c00642
Tondeur, J., van Braak, J., Sang, G., Voogt, J., Fisser, P., and Ottenbreit-Leftwich, A. (2012). Preparing pre-service teachers to integrate technology in education: a synthesis of qualitative evidence. Comput. Educ. 59, 134–144. doi: 10.1016/j.compedu.2011.10.009
Voß, S. (2018). Im digitalen Zeitalter qualitätsorientiert lernen [quality-oriented learning in the digital age]: Chancen und Grenzen digitaler Medien: Eine Handreichung für Lehrkräfte Aller Fächer aus Allen Schularten, −stufen und -typen [opportunities and limitations of digital tools: A handout for teachers of all subjects from all types and levels of schools]. Stuttgart: Landesinstitut für Schulentwicklung [State Institute for School Development].
Vuorikari, R., Punie, Y., Carretero, S., van Brande, L., and den., (2016). DigComp 2.0: The digital competence framework for citizens. Luxembourg: Publications Office.
Walkowiak, M., and Nehring, A. (2016). Using ChemDuino, excel, and PowerPoint as tools for real-time measurement representation in class. J. Chem. Educ. 93, 778–780. doi: 10.1021/acs.jchemed.5b00923
Wejner, M., and Wilke, T. (2022). LabPi: A Digital Measuring Station for STEM Education 4.0. J. Chem. Edu. 99, 819–827. doi: 10.1021/acs.jchemed.1c01139
Wilson, M. L., Ritzhaupt, A. D., and Cheng, L. (2020). The impact of teacher education courses for technology integration on pre-service teacher knowledge: a meta-analysis study. Comput. Educ. 156:103941. doi: 10.1016/j.compedu.2020.103941
Wörner, S., Scheiter, K., and Kuhn, J. (2021). “Das Beste aus beiden Welten: Simulationsexperimente als Ergänzung zu Realexperimenten [the best of both worlds: simulation experiments as a complement to physical experiments]” in Naturwissenschaften digital: Toolbox für den Unterricht [natural sciences digital: Toolbox for the classroom]: Band 2. eds. J. Meßinger-Koppelt and J. Maxton-Küchenmeister (Hamburg: Joachim Herz Stiftung), 48–51.
Wohlfart, O., Trumler, T., and Wagner, I. (2021). The unique effects of Covid-19 - a qualitative study of the factors that influence teachers’ acceptance and usage of digital tools. Educ. Inf. Technol. 26, 7359–7379. doi: 10.1007/s10639-021-10574-4
Keywords: technology acceptance model, digitalization, chemistry didactics, subject-specific tools, teacher training
Citation: Wohlfart O, Wagner AL and Wagner I (2023) Digital tools in secondary chemistry education – added value or modern gimmicks? Front. Educ. 8:1197296. doi: 10.3389/feduc.2023.1197296
Edited by:
Anatoliy Markiv, King's College London, United KingdomReviewed by:
Milan Kubiatko, J. E. Purkyne University, CzechiaKonstantinos Lavidas, University of Patras, Greece
Copyright © 2023 Wohlfart, Wagner and Wagner. This is an open-access article distributed under the terms of the Creative Commons Attribution License (CC BY). The use, distribution or reproduction in other forums is permitted, provided the original author(s) and the copyright owner(s) are credited and that the original publication in this journal is cited, in accordance with accepted academic practice. No use, distribution or reproduction is permitted which does not comply with these terms.
*Correspondence: Olivia Wohlfart, Olivia.wohlfart@kit.edu