- 1Department of Chemistry, South Kazakhstan Pedagogical University, Shymkent, Kazakhstan
- 2Department of Outpatient Therapy, Institute of Clinical Medicine, I.M. Sechenov First Moscow State Medical University, Moscow, Russia
Introduction: The metaverse is a promising platform for learning that all participants in the teaching and learning process may find appealing. This study aims to investigate the effectiveness of using metaverse-related visualization technologies, such as Virtual Reality (VR) and Augmented Reality (AR), in teaching chemistry.
Methods: The study has an experimental research design and uses the following tools to collect data: Dombrovskaya’s Motivation for Learning Scale, the SAMOAL Questionnaire, and the Self-management Questionnaire. The study sample includes 236 first-year undergraduate students studying chemistry at the Faculty of Natural Sciences.
Results: Results suggest that the integration of immersive VR/AR technologies in chemistry education signifies the beginning of a metaverse transition; the process, however, is in its early stages.
Discussion: The study concludes that immersive VR/AR technologies have an advantage over traditional programs in terms of motivation and competence development. Future research can investigate the impact of VR/AR technologies on students’ anxiety and build a model for predicting student achievements in the metaverse-based chemistry classroom.
Introduction
The concept of the metaverse has been increasingly penetrating all spheres of human life since its first use in 1992 (Damar, 2021). Some researchers consider the metaverse to be a promising tool to enhance education (Allcoat and von Mühlenen, 2018; Sahin and Yilmaz, 2020; Fernandes and Werner, 2022). The cyber-simulated environment of the metaverse allows many users to interact, learn, and work simultaneously in remote physical locations (Bicen and Adedoyin, 2022). Accordingly, metaverse technologies and applications are of interest to researchers in many areas of science and practice (Trunfio and Rossi, 2022). The idea of Augmented Reality (AR) is drawing both students and educators (Mundy et al., 2019). Immersive technologies (augmented, mixed and virtual reality) are becoming increasingly popular in education, expanding the possibilities of online learning through the creation of immersive scenarios and learning environments (Oktay and Yüzer, 2023). Various visualization methods are used to process big data in VR and AR; meantime, modelling and statistical analysis take place in the metaverse in real time (Siwach et al., 2022). For instance, in the study conducted by Allcoat and Von Mühlenen (2018), 99 participants were assigned to one of three learning conditions: traditional (textbook style), virtual reality (VR), and video (passive control). The educational materials utilized the same text and 3D model across all conditions. Participants in both the traditional and virtual reality conditions exhibited improved overall performance (i.e., learning, including knowledge acquisition and comprehension) compared to those in the video condition. Furthermore, participants in the VR mode demonstrated superior results in “recall” compared to participants in the traditional and video conditions. Additionally, participants in the VR mode reported a higher level of engagement than those in the other conditions. Overall, VR demonstrated an enhanced learning experience in contrast to traditional teaching methods and video-based instruction.
In the wave of the COVID-19 pandemic, many scholars re-imagined the potential of the metaverse as a virtual learning environment that brings together the physical and digital worlds as a leading trend of the future (Iqbal et al., 2022; Zhang et al., 2022). The Virtual (VR) and Augmented Reality (AR) technologies signify the Fourth Industrial Revolution and promote the development of human-machine interaction (Eswaran and Bahubalendruni, 2022) VR, AR and artificial intelligence technologies show promise for many sectors of the economy, including education (Sosnilo et al., 2021; Zarantonello and Schmitt, 2023).
As a tool that provides alternative learning experiences, Virtual Reality has the power to facilitate knowledge assimilation. This advantage over traditional learning is of great importance for teaching chemistry as a discipline that requires interactive visualization (Richard et al., 2006; Nyssanbek et al., 2022). Prompt this perspective, it seems important to explore the potentials of metaverse technologies, VR and AR, in teaching chemistry. In the study conducted by Sahin and Yilmaz (2020), the experimental group completed a module of a scientific course utilizing augmented reality technology, while the control group completed the same module using traditional methods and textbooks. The results revealed that students in the experimental group exhibited higher academic performance scores and a more favourable attitude towards the course in comparison to students in the control group.
In this particular context, it becomes imperative to explore the potential of metaverse technologies, including VR and AR, in the realm of chemistry education. By substantiating the potential applications of these technologies in chemical education, this research posits novel avenues for enhancing the teaching and learning of chemistry, thereby integrating the virtual and physical educational domains. The novelty of this study lies in the fact that it substantiates the potential applications of immersive metaverse technologies (VR and AR) in chemistry education. In the contemporary world, these technologies are becoming increasingly significant and are being integrated into various facets of our lives, including education. Recent research trends are associated with a reevaluation of the metaverse’s potential as a virtual learning environment that merges the physical and digital realms (Dahan et al., 2022). Researchers and educators are beginning to recognise the potential afforded by the metaverse for educational purposes: it can facilitate the creation of interactive educational scenarios wherein students can engage with information and tasks within a virtual environment, thereby enhancing their learning and comprehension of subject matter (Damar, 2021; Bicen and Adedoyin, 2022). Furthermore, this can bridge the gap between the physical and digital realms, offering novel and more engaging avenues for learning and experimentation. This is particularly pertinent in light of the COVID-19 pandemic, which placed constraints on traditional teaching methods. Virtual Reality (VR) and Augmented Reality (AR) technologies are pivotal tools of the Fourth Industrial Revolution, enhancing the level of interaction between humans and machines. Their integration into education significantly expands the possibilities of online learning and enriches educational scenarios. In one of the systematic reviews, it is emphasized that various types of emerging technologies are being utilized in chemical education, including learning analytics, robotics, virtual reality (VR), and augmented reality (AR) (Chiu, 2021). Chiu’s study (2021) reveals that AR and VR applications have been the most extensively examined among the identified technologies employed in chemical education. Meanwhile, the primary areas of focus have revolved around virtual chemical laboratories, visualization and interaction with chemical structures, as well as in-class practical activities. The evidence presented in the reviewed work also points to promising applications of artificial intelligence and learning analytics for the analysis of feedback and student behavior, the assessment of students’ understanding of chemical concepts, and the investigation of reasoning and cognitive processes of students when undertaking chemical tasks such as spectral interpretation. The present findings can be used to improve chemistry teaching and learning in higher educational institutions through the interaction of virtual and physical learning environments.
Literature review
VR/AR technologies and the metaverse
The metaverse brings the Internet to a new level (Kress, 2019; Yuniarti and Suciati, 2019; Sutopo, 2022) by creating an interconnected network of immersive worlds focused on multisensory interactions with virtual environments, digital objects and people such as VR and AR (Mystakidis, 2022). One of the highlights of the metaverse is that it helps to develop soft skills and communicative competence (Sabtu, 2023). This feature expands learning opportunities while also creating several social issues and risks (Jian et al., 2022).
It is difficult to ensure a consistent interaction between virtual and physical environments in the metaverse (Guan, 2022). This problem necessitates the adoption of clear criteria for its definition. Even though the Metaverse is at its early stage of development, it shows promise, especially for education (Dahan et al., 2022; Zhao et al., 2023).
Metaverse as an innovative paradigm in education
The digitalization of education is associated primarily with the metaverse, which brings together mixed reality audiences and remote VR platforms (Chien et al., 2020; Wang et al., 2022). Mixed reality and gamification in education enable maximum immersion into learning activities. Other benefits of these technologies include the ability to provide individual feedback, improve motivation, and instill 21st-century competencies in students (Tang et al., 2020; Korkut and Surer, 2023; Tiemann and Annaggar, 2023).
The COVID-19 pandemic accelerated the adoption and development of metaverse-related learning technologies in distance education (Sumardani and Sumardani, 2022). The future of education is linked to the future of the metaverse, an innovative learning space integrating artificial intelligence, gamification, big data, the Internet of Things, and blockchain (Lin et al., 2022; Tlili et al., 2022). VR and AR technologies are successfully used in STEM education to create custom learning environments, develop communication skills, and motivate learners (Papanastasiou et al., 2019; Estudante and Dietrich, 2020; Iqbal et al., 2022). The main advantage of VR and AR technologies is the ability to provide conducive 3D learning experiences and reduce cognitive load (Papanastasiou et al., 2019).
Potentials of the metaverse for chemistry teaching and learning
The VR technologies help students to understand abstract concepts better and gain spatial skills in the chemistry classroom (Edwards et al., 2019). One example of an immersive learning environment for studying chemistry is the VR Multisensory Classroom (VRMC), which makes use of haptics (Edwards et al., 2019). Learning in the VRMC environment has proven to be engaging and improves student motivation. Another example of a learning platform that uses haptic interaction is the multimodal VR platform for simulation and experimentation, also known as VE VIREPSE. It allows users to conduct chemical experiments and provides them with multi-modal (visual, haptic, auditory and olfactory) feedback (Richard et al., 2006; Buchner and Kerres, 2022).
The spherical video-based VR (SVVR) technologies help students learn the theory and acquire the competencies necessary to conduct chemical experiments (Yang et al., 2022). The media mix modelling platforms used to model complex chemical systems help students develop a conceptual and systemic understanding of chemistry (Saba et al., 2023). Individuals performing chemistry experiments in the immersive virtual world of Second Life get less distracted than those who work in a real-world laboratory; consequently, they show better performance (Winkelmann et al., 2020).
Web-based learning (WBL) is effective in teaching a competency-based chemistry curriculum (Iyamuremye et al., 2023). The AR-based quests are used to illustrate an open chemical process (Estudante and Dietrich, 2020). VR has also been used to teach chemistry in an inclusive learning environment (Nsabayezu et al., 2022).
The VR/AR software enables the visualization of 3D molecules. A web-based AR platform for chemistry moleculARweb1 is one such program, providing interactive content that users can access through a variety of mobile devices, including laptops, tablets, and smartphones (Rodríguez et al., 2022). There are also special programs that allow teachers and students to create and control biological macromolecules and other materials using devices and immersive VR headsets compatible with WebXR devices (Rodríguez et al., 2022).
Hence, the integration of virtual and augmented reality technologies into the educational process holds substantial significance for the advancement of teaching and research in the field of chemistry. The benefits encompass a deeper comprehension of abstract concepts, an engaging and motivating learning environment, and multimodal feedback for students. The utilization of immersive environments, such as multisensory virtual reality classrooms and multimodal modelling platforms, enriches learning and contributes to the conceptual understanding of complex chemical systems. Virtual and augmented reality technologies provide a broad spectrum of opportunities for transforming the educational process in chemistry, enhancing its efficacy, and stimulating interest in the discipline. These innovations can significantly enrich education, making it more captivating, interactive, and immersive.
Nonetheless, there are challenges associated with the integration of these innovations into education. This encompasses the necessity of ensuring the accessibility of appropriate hardware and technical infrastructure, as well as the training of educators for the effective utilization of these technologies in teaching. The management and support of such innovations also demand additional resources and time.
Problem statement
Industry 4.0 is built on advanced technologies, including the Internet of Things, Artificial Intelligence, VR/AR, and a cyber-physical system. These technologies find their way into universities to transform the education system in a way that fulfils the modern labor market demands. Amid these changes, the role of chemistry education seems to increase (Sari et al., 2021). This issue received insufficient attention in the literature, and the present study seeks to narrow the said knowledge gap. This study aims to investigate the effectiveness of using metaverse-related visualization technologies, such as VR and AR, in teaching chemistry. The objective of the study is to answer the following research questions:
Q1: How well are VR and AR technologies represented in chemistry education research from the metaverse perspective?
Q2: Do the current applications in visualization technology signify the rise of metaverse in chemistry education?
Q3: Do VR/AR technologies have any advantages for chemistry learning from the perspective of motivation and competency development when compared to traditional learning formats?
Materials and methods
Study design
The study has an experimental research design and uses analytical, psychodiagnostic, and statistical methods to collect and interpret data. The research framework is built on the concept of Metaverse – a fully immersive virtual world created with the help of advanced technologies, such as VR and AR technologies (Oktay and Yüzer, 2023).
Participants
The study lasting over 1 month took place at the Faculty of Natural Sciences in the South Kazakhstan State Pedagogical University. The study population comprised 236 first-year undergraduate students divided into two groups by simple randomization. The experimental group had 122 students and used immersive VR/AR technologies to teach chemistry. The control group had 114 students and followed the traditional learning program under real-world laboratory conditions. The groups were comparable in terms of chemistry proficiency, age, gender and ethnic composition.
The chemistry topics taught in the two groups were identical, encompassing various aspects of the chemical sciences, including atomic structure, chemical bonding, kinetics and thermodynamics of chemical reactions, fundamental laws, and theories of chemistry, such as “Atoms,” “Chemical Bonds,” “Chemical Reactions,” and so forth. The integration of these topics into the educational process enabled students to gain a more vivid understanding of intricate concepts and deepen their knowledge in the field of chemistry.
For the experimental group utilizing VR/AR technologies, interaction with the educational material occurred within virtual laboratories designed by the chemistry curriculum, three times a week, for two academic hours each session. In the experimental group, activities were conducted to engage students with virtual reality, simulations of chemical reactions, visualization of complex chemical structures, and similar endeavours. These activities facilitated a deeper comprehension of chemical concepts. VR/AR technologies allow students to interact with chemical processes, molecules, and reactions within a virtual environment. In the control group, which followed a traditional curriculum in real laboratory settings, students adhered to the curriculum, meeting three times a week for two academic hours per session. This curriculum comprised lectures, practical exercises, and laboratory sessions conducted in physical chemistry laboratories.
Instruments
All participants underwent preliminary assessment and psychodiagnostic examination before training to identify their levels of learning motivation and creativity. For this, the following methods were used: Dombrovskaya’s Motivation for Learning Scale (Dombrovskaya, 2018), the SAMOAL Questionnaire (Fetiskina, 2002), and the Self-management Questionnaire. The Dombrovskaya’s Motivation for Learning Scale measures two types of motivation for learning, cognitive and social, and helps to determine the level of engagement. The questionnaire has 30 statements and uses a 5-point Likert-type scale, ranging from Never (0) to Always (4). Motivation score < 2 and motivation score > 3 were defined as low and high, respectively. The average level of motivation corresponds to a motivation score of 2 to 3 points.
The SAMOAL Questionnaire (by A.V. Lazukin, M.F. Kalin’s adaptation) examines whether an individual feels the need for self-actualization. SAMOAL has eleven scales that measure some personal qualities of an individual: Orientation to time; Values; Perception of human nature; Need for cognition; Creativity; Autonomy; Spontaneity; Self-understanding; Auto-sympathy; Communicability; Flexibility in communication. The score for each scale ranges from 0 to 15 points. The self-management questionnaire aims to assess self-management skills. The focus is on time management and strategic and tactical planning.
Data analysis
Statistical data processing was carried out in Microsoft Excel. A Student’s t-test was used to determine whether the results were statistically significant and reliable. The Pearson R statistical test was applied to evaluate the relationship between scales; the results were interpreted using Chaddock tables. All calculations were done in Social Science Statistics.
Limitations
The research exhibits several constraints associated with a limited sample size and a restricted time frame. The focus was on first-year undergraduate students, for they had sufficient theoretical knowledge and practical experience in chemistry, while their educational competencies were at an early stage of development. Involving participants with such a profile made it possible to view the dynamics more clearly. The time factor was also important. Because control students did not use VR/AR technologies during their training, they could have been less motivated to study than their peers in the study group. Therefore, it seemed crucial to limit the time of training to prevent any imbalance in the curricula. It is also important to acknowledge the potential limitations and systematic bias associated with the implementation of this study. Systematic biases may arise due to variations in student preferences related to the use of VR/AR technologies. Some students may have been more inclined toward technological innovations, which could have influenced the research outcomes.
Ethical issues
The study strictly follows the principles of bioethics. The research protocol was approved by the Ethical Committee of the University. All participants gave their written informed consent to participate in the study. Confidentiality and anonymity were ensured. The principle of academic integrity was also respected.
Results
Immersive learning in the metaverse is conducive to high academic performance primarily because it enhances student engagement, motivation, and communicative competence. This study seeks to confirm or refute this statement by examining the effectiveness of metaverse technologies in teaching chemistry. Table 1 shows the influence of VR/AR technology on students’ motivation.
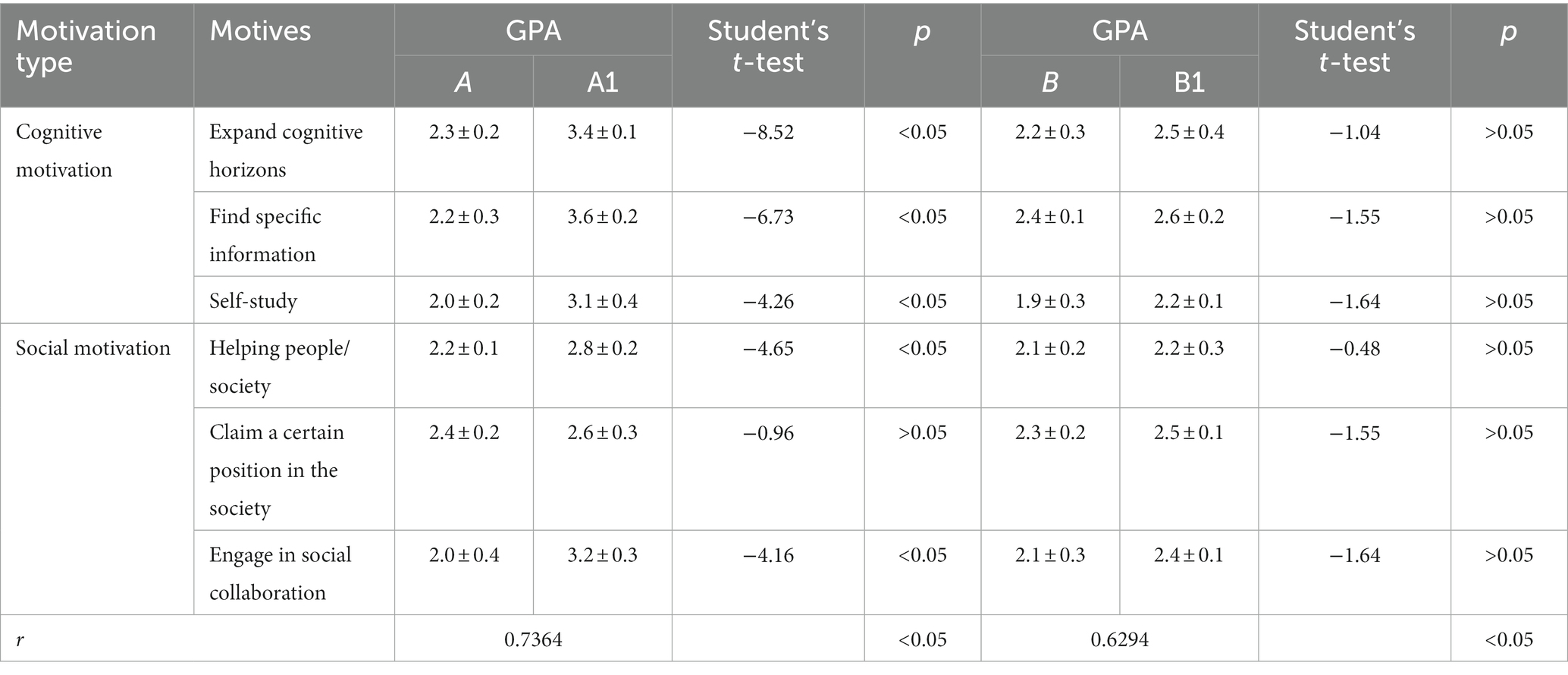
Table 1. Changes over time in motivations for learning across the VR/AR (A – baseline, A1 – post-training) and control groups (B – baseline; B1 – post-training).
As can be seen, the baseline levels of cognitive and social motivation were comparable between the two groups and fell within the range of average values. Students became more motivated after VR/AR-enhanced training in the metaverse. By contrast, the control group demonstrated no statistically significant differences. A statistically significant increase in motivation to expand cognitive horizons (p < 0.05) suggests that learners in the metaverse show higher interest in learning the theoretical principles of chemistry and are more eager to acquire new knowledge. Higher motivation to find specific information (p < 0.05) suggests that learners became more inclined to explore the scientific side of specific topics and gain skills necessary for independent learning.
Students immersed in the metaverse are more propelled to learn independently than those training with traditional means, as evidenced by a statistically significant increase in motivation to self-study (p < 0.05). A statistically significant increase was found in students’ motivation to help people and society and in their motivation to engage in social collaboration (p < 0.05). This finding suggests the formation of communicative competence among students learning chemistry with VR/AR. Meantime, their level of motivation to claim a certain position in society changed insignificantly (р > 0.05). A strong direct correlation was found between cognitive motivation and social motivation (r = 0.7364 in the VR/AR group and r = 0.6294 in the control group).
Table 2 presents a comparative summary of how teaching chemistry in the metaverse and a real-world laboratory affects students’ desire for self-actualization. As can be seen, the baseline scores for self-actualization were comparable between the two groups and fell within the range of average values. Both groups demonstrated an increase in self-actualization indicators after training, but the change was more significant in the VR/AR group.
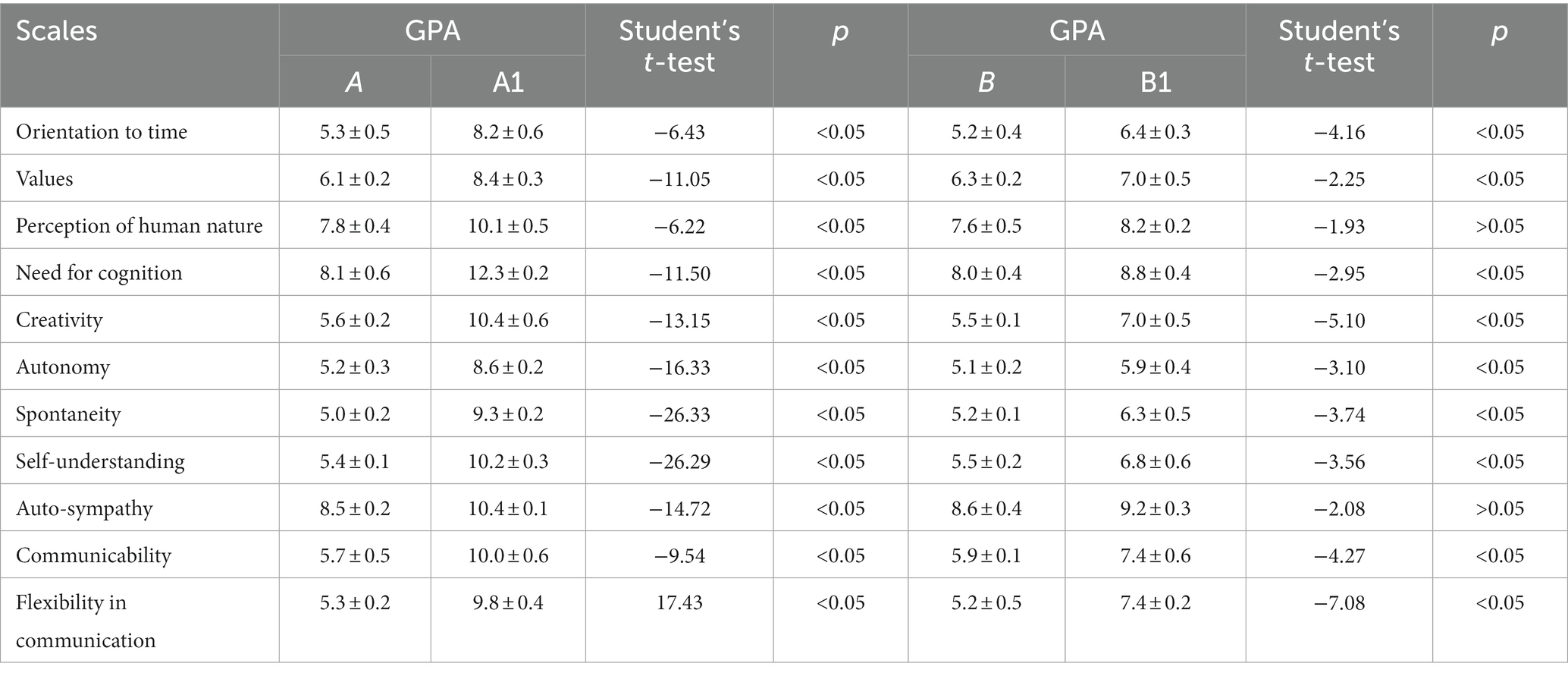
Table 2. Changes over time in self-actualization across the VR/AR (A – baseline, A1 – post-training) and control groups (B – baseline; B1 – post-training).
A higher score on the orientation to time scale suggests an increase in self-confidence, which was more pronounced in the study group (8.2 ± 0.6 vs. 6.4 ± 0.3 points, p < 0.05). A higher score on the values scale reflects harmonization of the interpersonal relationships, which again was more evident in the VR/AR group (8.4 ± 0.3 vs. 7.0 ± 0.5 points, p < 0.05). While the study group experienced a significant improvement in this regard (p > 0.05), no significant differences were seen in the control group (p > 0.05). It seems that students immersed in the metaverse started to feel a much stronger need for cognition than the ordinary controls (12.3 ± 0.2 vs. 8.8 ± 0.4 points, p < 0.05). Similarly, they became more creative, autonomous and spontaneous. An improvement was also seen in their sense of self-understanding, communicability and flexibility in communication which reflect personal growth and the development of communicative competence. Unlike the VR/AR group, students studying in an actual laboratory demonstrated no statistically significant changes in their level of auto-sympathy (p > 0.05). This finding suggests that teaching chemistry in the metaverse using immersive VR/AR technologies is more conducive to the formation of positive self-concept, critical awareness and self-confidence than traditional teaching.
Table 3 presents a comparative summary of how teaching chemistry in the metaverse and a real-life laboratory affects students’ ability to self-organize. As can be seen, the baseline scores for self-management were comparable between the two groups and fell within the range of average values. After training, VR/AR users exhibited a pronounced improvement on all scales of self-management (p < 0.05). This finding suggests that students in the VR/AR group became more engaged with the learning process. They likely became better at planning their cognitive activity. The control group, on the other hand, showed no significant changes in terms of self-management (р > 0.05). According to the results of the baseline assessment, the knowledge of the basics of chemistry in the study groups was of comparable depth; the average test score was 68.4 ± 2.1 points (of the 100 points available). The post-training assessment revealed an improvement in the basic knowledge levels, with the average score in the control group being 75.3 ± 2.8 (p < 0.05) and the average score in the experimental group being 86.1 ± 4.0 points (p < 0.05).
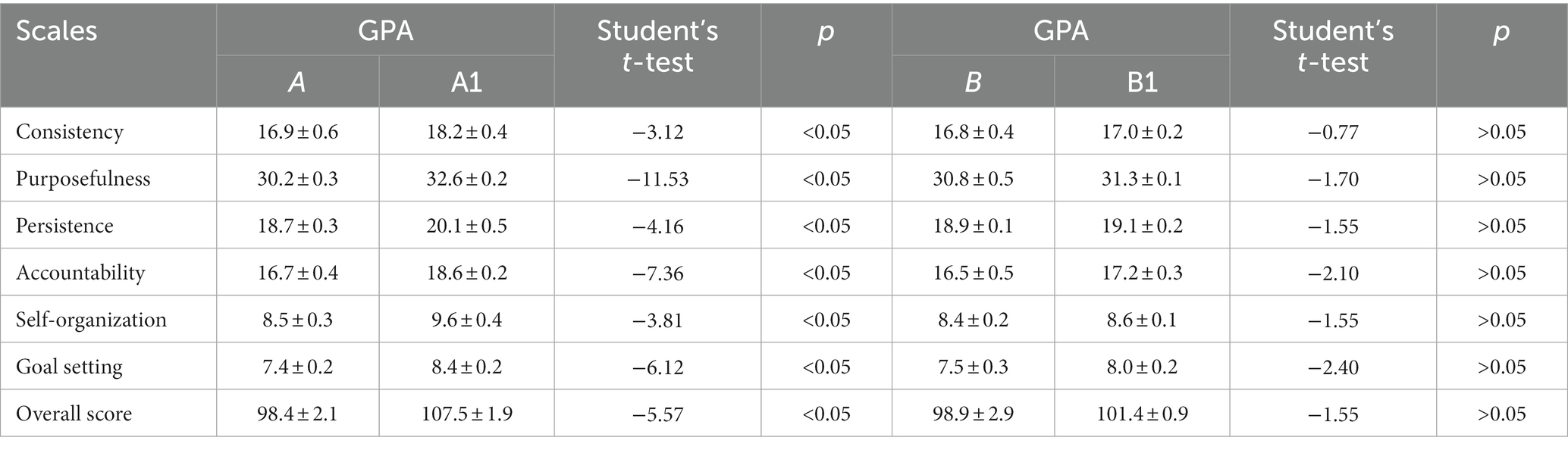
Table 3. Changes over time in self-management across the VR/AR (A – baseline, A1 – post-training) and control groups (B – baseline; B1 – post-training).
The present findings suggest that teaching chemistry in the metaverse with immersive VR/AR technologies is more engaging than traditional programs. The VR/AR-enhanced learning in the metaverse can also improve students’ self-actualization, self-management and communication skills, facilitating the assimilation of theoretical knowledge and the acquisition of practical skills. The result would be a more prominent improvement in academic performance when compared to traditional learning.
Discussion
The available indicates suggests that the integrated use of VR and AR technologies in the classroom makes them more effective (Park et al., 2019; Shen et al., 2019). Shen et al. (2019) presented students with a video clip showcasing the application of VR in education, followed by an online survey. The results demonstrated that all examined constructs exerted a positive and significant influence on students’ behavioural intention to use VR in their learning, with only the specific experience mode within Kolb’s learning styles exhibiting a positive and significant effect. The present study agrees with this statement, for according to the results of the assessment, the experimental group had more significant improvements than the control group. The post-training assessment identified an enhancement in basic knowledge levels, with the average score in the control group registering at 75.3 ± 2.8 (p < 0.05), while the average score in the experimental group reached 86.1 ± 4.0 (p < 0.05).
Some researchers argue that VR and AR technologies help learners promote the development of cognitive processes and communicative competencies (Horváth, 2021). Horváth (2021) presents a study involving 90 students. The research aims to investigate how the application of 3D VR platforms as personalized educational environments can enhance the effectiveness of VR learning. Within the scope of this study, the correlation between task effectiveness and Kolb’s learning style is examined. The research underscores the immense significance of selecting the optimal task type for each Kolb learning style and individualized learning environment. 3D spaces such as MaxWhere demonstrate a high potential for personalizing VR education. Unobtrusive guiding features in VR environments and educational content integrated into 3D VR spaces have proven highly successful, as learners were able to achieve test scores 20 per cent higher following VR-based instruction compared to the utilization of traditional educational tools. Note in our paper that immersive VR/AR technologies also had a positive effect on students’ social motivation, primarily in terms of social collaboration which is crucial for self-study and self-improvement. The statistically significant increase in motivation for expanding cognitive horizons (p < 0.05) indicates that students in the metaverse exhibit a heightened interest in delving into the theoretical foundations of chemistry and are more inclined towards acquiring new knowledge. A statistically significant improvement in the perception of human nature, communicability and flexibility in communication suggests a positive impact of innovative immersive learning on the ability to build benevolent, harmonious and effective relationships with others.
A significant advantage of VR/AR-enhanced learning is its capability to facilitate the formation of adequate self-esteem and self-concept, as evidenced by a statistically significant increase in the auto-sympathy score in the experimental group. In the control group, no such improvement was demonstrated, which suggests a possibly low self-confidence among students in that group. The use of VR/AR technologies could be the reason behind the improvement in auto-sympathy. An additional study is needed to verify this statement that would investigate the anxiety level of students using the Spielberger–Khanin method.
Chemistry is one of the important areas of education that stands behind scientific and technological innovations in the global market (Osman and Lay, 2022). Digitalization and gamification contribute to a better understanding of theory and help to develop practical skills; one such case involves the Malaysian Kimia (chemistry) Digital Game, known as MyKimDG (Osman and Lay, 2022). The VR and AR technologies enable a deep engagement with three-dimensional digital content and allow users to control mobile scenarios in the virtual world in the same way as in the real physical environment, which helps to improve their spatial perception (Zhang et al., 2021). The Internet of Things (IoT) plays a key role in achieving sustainable development goals (Cadeado et al., 2022). The ability to obtain analytical data in real-time allows students to use IoT technologies to solve practical environmental and educational problems in the field of analytical chemistry (Attwood, 2020; Cadeado et al., 2022). The present study also suggests that VR/AR technologies are more helpful for studying chemistry than traditional means. Advances in metaverse technology may lead to revolutionary transformations in education. However, it is too early to know its potential, for the integration of VR/AR experiences represents only the initial stage of future transformations. Nevertheless, the results of this study are convincing enough to believe that the technological transformation of the educational system shows promise from the perspective of effectiveness, engagement, and personal fulfilment.
Taking advantage of the Internet of Things (IoT) and Deep Learning (DL) technologies to build a personalized learning environment allows educators to expand their resources and predict student achievements (Wang and Lv, 2022). The findings of the study by Wang and Lv (2022) reveal that 96.67% of students express satisfaction with the educational effectiveness of the personalized learning model, while 100% of the students are content with the various forms of educational resources offered by this model. In this paper, the VR/AR-enhanced learning was more effective than the traditional chemistry program, primarily in improving students’ self-management and self-actualization skills. Following the training, users of VR/AR exhibited a pronounced improvement across all self-regulation scales (p < 0.05). Initial self-actualization scores were comparable between the two groups and fell within the range of moderate values. Both groups demonstrated an increase in self-actualization scores after the training, but the change was more significant in the VR/AR group. The present findings can be used in further research to build a predictive model for student achievements.
Conclusion
Even though the Metaverse was reported to show potential for chemistry teaching, the number of empirical studies in this field is insufficient. The current integration of immersive VR and AR technologies into the chemistry curriculum can be considered the beginning of the Metaverse transition process. The present study shows a statistically significant increase in students’ motivation to expand cognitive horizons (2.3 ± 0.2 points at baseline vs. 3.4 ± 0.1 points after immersive learning, p < 0.05) and find specific knowledge (2.2 ± 0.3 points at baseline vs. 3.6 ± 0.2 points after immersive learning, p < 0.05). This finding suggests that students immersed in the metaverse through VR and AR headsets are more inclined to learn the theoretical principles of chemistry, gain new knowledge, and develop skills necessary for independent learning. Learners using the VR/AR technology also appeared more motivated to help society (2.2 ± 0.1 points at baseline vs. 2.8 ± 0.2 points after immersive learning, p < 0.05) and engage in social collaboration (2.0 ± 0.4 points at baseline vs. 3.2 ± 0.3 points after immersive learning, p < 0.05) than their peers enrolled in a traditional curriculum. This finding suggests that the combined use of VR and AR technologies is conducive to the formation of communicative competency among students. The results also show that learning chemistry in the metaverse using VR/AR technologies has a positive effect on students’ self-actualization, self-concept, motivation, and ability to plan their learning.
Based on these findings, it can be concluded that immersive VR/AR technologies have an advantage over traditional programs in terms of motivation and competence development. Future research can investigate the impact of VR/AR technologies on students’ anxiety and build a model for predicting student achievements in the metaverse-based chemistry classroom.
Data availability statement
The raw data supporting the conclusions of this article will be made available by the authors, without undue reservation.
Author contributions
EA contributed to conception and design of the study. NS performed the statistical analysis and wrote the first draft of the manuscript. EM wrote sections of the manuscript. All authors contributed to manuscript revision, read, and approved the submitted version.
Conflict of interest
The authors declare that the research was conducted in the absence of any commercial or financial relationships that could be construed as a potential conflict of interest.
Publisher’s note
All claims expressed in this article are solely those of the authors and do not necessarily represent those of their affiliated organizations, or those of the publisher, the editors and the reviewers. Any product that may be evaluated in this article, or claim that may be made by its manufacturer, is not guaranteed or endorsed by the publisher.
Footnotes
References
Allcoat, D., and von Mühlenen, A. (2018). Learning in virtual reality: effects on performance, emotion and engagement. Res. Learn. Technol. 26, 1–13. doi: 10.25304/rlt.v26.2140
Attwood, A. I. (2020). Changing social learning theory through reliance on the internet of things and artificial intelligence. J. Sustainable Soc. Change 12:8. doi: 10.5590/JOSC.2020.12.1.08
Bicen, H., and Adedoyin, O. B. (2022). Bibliometric analysis of studies on Metaverse in education. In C. E. Cîrnu (Ed.), Proceedings of the International Conference on Virtual Learning 179–192. Bucharest: Near East University
Buchner, J., and Kerres, M. (2022). Media comparison studies dominate comparative research on augmented reality in education. Comput. Educ. 195:104711. doi: 10.1016/j.compedu.2022.104711
Cadeado, A. N., Machado, C., Oliveira, G. C., Silva, D. A., Muñoz, R. A., and Silva, S. G. (2022). Internet of things as a tool for sustainable analytical chemistry: a review. J. Braz. Chem. Soc. 33, 681–692. doi: 10.21577/0103-5053.20220048
Chien, S. Y., Hwang, G. J., and Jong, M. S. Y. (2020). Effects of peer assessment within the context of spherical video-based virtual reality on EFL students’ English-speaking performance and learning perceptions. Comput. Educ. 146:103751. doi: 10.1016/j.compedu.2019.103751
Chiu, W. K. (2021). Pedagogy of emerging technologies in chemical education during the era of digitalization and artificial intelligence: a systematic review. Educ. Sci. 11:709. doi: 10.3390/educsci11110709
Dahan, N. A., Al-Razgan, M., Al-Laith, A., Alsoufi, M. A., Al-Asaly, M. S., and Alfakih, T. (2022). Metaverse framework: a case study on E-learning environment (ELEM). Electronics 11:1616. doi: 10.3390/electronics11101616
Damar, M. (2021). Metaverse shape of your life for future: a bibliometric snapshot. J. Metaverse 1, 1–8.
Dombrovskaya, N. (2018). “Information technology as an essential part of language teaching” in Innovative approaches in the educational process of higher education: National and international aspects: Electronic collection of articles from international scientific and practical conference dedicated to the 50th anniversary of the Polotsk state university. eds. Y. P. Golubev and N. A. Boreyko (Novopolotsk: Polotsk State University), 202–204.
Edwards, B. I., Bielawski, K. S., Prada, R., and Cheok, A. D. (2019). Haptic virtual reality and immersive learning for enhanced organic chemistry instruction. Virtual Reality 23, 363–373. doi: 10.1007/s10055-018-0345-4
Estudante, A., and Dietrich, N. (2020). Using augmented reality to stimulate students and diffuse escape game activities to larger audiences. J. Chem. Educ. 97, 1368–1374. doi: 10.1021/acs.jchemed.9b00933
Eswaran, M., and Bahubalendruni, M. R. (2022). Challenges and opportunities on AR/VR technologies for manufacturing systems in the context of industry 4.0: a state of the art review. J. Manuf. Syst. 65, 260–278. doi: 10.1016/j.jmsy.2022.09.016
Fernandes, F., and Werner, C. (2022). “A systematic literature review of the metaverse for software engineering education: overview, challenges and opportunities” in Software engineering education in the Metaverse (Washington, WA, USA: PRESENCE), 1–37.
Fetiskina, N. P. (2002). Social’no-psihologicheskaya diagnostika razvitiya lichnosti i malyh grupp [socio-psychological diagnostics of personality and small development]. Moscow, Russia: Institute of Psychotherapy.
Guan, J. (2022). Extending the Metaverse: Exploring generative objects with extended reality environments and adaptive context awareness. Thesis. OCAD University.
Horváth, I. (2021). An analysis of personalized learning opportunities in 3D VR. Front. Comput. Sci. 3:673826. doi: 10.3389/fcomp.2021.673826
Iqbal, M. Z., Mangina, E., and Campbell, A. G. (2022). Current challenges and future research directions in augmented reality for education. Multimodal Technol. Interact. 6:75. doi: 10.3390/mti6090075
Iyamuremye, A., Mukiza, J., Nsengimana, T., Kampire, E., Sylvain, H., and Nsabayezu, E. (2023). Knowledge construction in chemistry through web-based learning strategy: a synthesis of literature. Educ. Inf. Technol. 28, 5585–5604. doi: 10.1007/s10639-022-11369-x
Jian, S., Chen, X., and Yan, J. (2022). “From online games to “Metaverse”: the expanding impact of virtual reality in daily life” in Culture and computing: 10th international conference, C&C 2022, held as part of the 24th HCI international conference, HCII 2022, virtual event, June 26–July 1, 2022, proceedings. ed. M. Rauterberg (Cham: Springer International Publishing), 34–43.
Korkut, E. H., and Surer, E. (2023). Visualization in virtual reality: a systematic review. Virtual Reality 27, 1447–1480. doi: 10.1007/s10055-023-00753-8
Kress, B. C. (2019). “Digital optical elements and technologies (EDO19): applications to AR/VR/MR” in Digital optical technologies 2019. eds. B. C. Kress and P. Schelkens, vol. 11062 (Munich: SPIE), 343–355.
Lin, H., Wan, S., Gan, W., Chen, J., and Chao, H. C. (2022). Metaverse in education: Vision, opportunities, and challenges. arXiv preprint arXiv:2211.14951
Mundy, M. A., Hernandez, J., and Green, M. (2019). Perceptions of the effects of augmented reality in the classroom. J. Instruct. Pedagog. 22, 1–15.
Nsabayezu, E., Iyamuremye, A., Urengejeho, V., Mukiza, J., Ukobizaba, F., Mbonyiryivuze, A., et al. (2022). Computer-based learning to enhance chemistry instruction in the inclusive classroom: teachers’ and students’ perceptions. Educ. Inf. Technol. 27, 11267–11284. doi: 10.1007/s10639-022-11082-9
Nyssanbek, M., Mukhametov, A., and Azimov, A. (2022). Isolation of microfibers in the processing of polyamide fabrics. Materials Technol. 56, 623–627. doi: 10.17222/mit.2022.621
Oktay, Ö. S., and Yüzer, T. V. (2023). “Immersive learning, immersive scenarios, and immersive technologies” in Shaping the future of online learning: Education in the Metaverse. eds. G. Durak and S. Cankaya (Hershey: IGI Global), 83–111.
Osman, K., and Lay, A. N. (2022). MyKimDG module: an interactive platform towards development of twenty-first century skills and improvement of students’ knowledge in chemistry. Interact. Learn. Environ. 30, 1461–1474. doi: 10.1080/10494820.2020.1729208
Papanastasiou, G., Drigas, A., Skianis, C., Lytras, M., and Papanastasiou, E. (2019). Virtual and augmented reality effects on K-12, higher and tertiary education students’ twenty-first century skills. Virtual Reality 23, 425–436. doi: 10.1007/s10055-018-0363-2
Park, M., Lee, S., Jeon, K. S., and Seol, H. (2019). A study on the development direction of education and training system based on AR/VR technology. J. Korea Institute of Military Sci. Technol. 22, 545–554. doi: 10.9766/KIMST.2019.22.4.545
Richard, E., Tijou, A., Richard, P., and Ferrier, J. L. (2006). Multi-modal virtual environments for education with haptic and olfactory feedback. Virtual Reality 10, 207–225. doi: 10.1007/s10055-006-0040-8
Rodríguez, F. C., Dal Peraro, M., and Abriata, L. A. (2022). Online tools to easily build virtual molecular models for display in augmented and virtual reality on the web. J. Mol. Graph. Model. 114:108164. doi: 10.1016/j.jmgm.2022.108164
Saba, J., Hel-Or, H., and Levy, S. T. (2023). Much. Matter. In. Motion: learning by modeling systems in chemistry and physics with a universal programing platform. Interact. Learn. Environ. 31, 3128–3147. doi: 10.1080/10494820.2021.1919905
Sabtu, M. A. S. (2023). “Metaverse and soft skills development through video games” in Metaverse applications for new business models and disruptive innovation. eds. M. Anshari, M. Syafrudin, and G. Alfian (Hershey: IGI Global), 120–132.
Sahin, D., and Yilmaz, R. M. (2020). The effect of augmented reality technology on middle school students' achievements and attitudes towards science education. Comput. Educ. 144:103710. doi: 10.1016/j.compedu.2019.103710
Sari, I., Sinaga, P., and Hernani, H. (2021). “The impact of industrial revolution 4.0 on basic chemistry learning” in The 2nd science and mathematics international conference (SMIC 2020): Transforming research and education of science and mathematics in the digital age. ed. M. Delina , vol. 2331 (Jakarta: AIP Publishing LLC)
Shen, C. W., Ho, J. T., Ly, P. T. M., and Kuo, T. C. (2019). Behavioural intentions of using virtual reality in learning: perspectives of acceptance of information technology and learning style. Virtual Reality 23, 313–324. doi: 10.1007/s10055-018-0348-1
Siwach, G., Haridas, A., and Bunch, D. (2022). “Inferencing big data with artificial intelligence & machine learning models in Metaverse” in 2022 international conference on smart applications, communications and networking (SmartNets). eds. J. M. Chuma and S. Masupe (Palapye: IEEE), 1–6.
Sosnilo, A. V., Kreer, M. Y., and Petrova, V. V. (2021). AR/VR technologies in management and education. Management 9, 114–124. doi: 10.26425/2309-3633-2021-9-2-114-124
Sumardani, D., and Sumardani, N. I. (2022). “How metaverse—virtual reality—can facilitate the emergency remote teaching” in Indonesia post-pandemic outlook series: social perspectives. eds. I. M. A. Hidayahtulloh, I. Jati, and D. Sumardani (Indonesia: BRIN Publishing), 289–305.
Tang, Y. M., Au, K. M., Lau, H. C., Ho, G. T., and Wu, C. H. (2020). Evaluating the effectiveness of learning design with mixed reality (MR) in higher education. Virtual Reality 24, 797–807. doi: 10.1007/s10055-020-00427-9
Tiemann, R., and Annaggar, A. (2023). A framework for the theory-driven design of digital learning environments (FDDLEs) using the example of problem-solving in chemistry education. Interact. Learn. Environ. 31, 1199–1212. doi: 10.1080/10494820.2020.1826981
Tlili, A., Huang, R., Shehata, B., Liu, D., Zhao, J., Metwally, A. H. S., et al. (2022). Is Metaverse in education a blessing or a curse: a combined content and bibliometric analysis. Smart Learn. Environ. 9:24. doi: 10.1186/s40561-022-00205-x
Trunfio, M., and Rossi, S. (2022). Advances in Metaverse investigation: streams of research and future agenda. Virtual Worlds 1, 103–129. doi: 10.3390/virtualworlds1020007
Wang, Y., Lee, L. H., Braud, T., and Hui, P. (2022). “Re-shaping post-COVID-19 teaching and learning: a blueprint of virtual-physical blended classrooms in the metaverse era” in 2022 IEEE 42nd international conference on distributed computing systems workshops (ICDCSW). ed. G. Pau (Bologna: IEEE), 241–247.
Wang, M., and Lv, Z. (2022). Construction of personalized learning and knowledge system of chemistry specialty via the internet of things and clustering algorithm. J. Supercomput. 78, 10997–11014. doi: 10.1007/s11227-022-04315-8
Winkelmann, K., Keeney-Kennicutt, W., Fowler, D., Lazo Macik, M., Perez Guarda, P., and Joan Ahlborn, C. (2020). Learning gains and attitudes of students performing chemistry experiments in an immersive virtual world. Interact. Learn. Environ. 28, 620–634. doi: 10.1080/10494820.2019.1696844
Yang, Q. F., Lin, H., Hwang, G. J., Su, P. Y., and Zhao, J. H. (2022). An exploration-based SVVR approach to promote students’ chemistry learning effectiveness. Interact. Learn. Environ., 1–25. doi: 10.1080/10494820.2022.2135106
Yuniarti, A., and Suciati, N. (2019). “A review of deep learning techniques for 3D reconstruction of 2D images” in 2019 12th international conference on Information & Communication Technology and system (ICTS). eds. M. Ashari, A. Z. Arifin, and D. Herumurti (Surabaya: IEEE), 327–331.
Zarantonello, L., and Schmitt, B. H. (2023). Experiential AR/VR: a consumer and service framework and research agenda. J. Serv. Manag. 34, 34–55. doi: 10.1108/JOSM-12-2021-0479
Zhang, X., Chen, Y., Hu, L., and Wang, Y. (2022). The metaverse in education: definition, framework, features, potential applications, challenges, and future research topics. Front. Psychol. 13:1016300. doi: 10.3389/fpsyg.2022.1016300
Zhang, L., He, W., Liu, Y., Zou, Q., Bai, H., and Billinghurst, M. (2021). “Arinvr: bringing mobile AR into VR” in SIGGRAPH Asia 2021 Posters. eds. S. J. Shiota, A. Kimura, and W.-C. A. Ma (Tokyo: ACM), 1–2.
Keywords: 3D reconstruction, artificial intelligence, chemistry education, internet of things, metaverse games
Citation: Amirbekova E, Shertayeva N and Mironova E (2024) Teaching chemistry in the metaverse: the effectiveness of using virtual and augmented reality for visualization. Front. Educ. 8:1184768. doi: 10.3389/feduc.2023.1184768
Edited by:
P. G. Schrader, University of Nevada, Las Vegas, United StatesReviewed by:
Antonio Luque, University of Almeria, SpainWang-Kin Chiu, The Hong Kong Polytechnic University, China
Copyright © 2024 Amirbekova, Shertayeva and Mironova. This is an open-access article distributed under the terms of the Creative Commons Attribution License (CC BY). The use, distribution or reproduction in other forums is permitted, provided the original author(s) and the copyright owner(s) are credited and that the original publication in this journal is cited, in accordance with accepted academic practice. No use, distribution or reproduction is permitted which does not comply with these terms.
*Correspondence: Elmira Amirbekova, eamirbekova7@rambler.ru