- 1Department of Biology, University of Richmond, Richmond, VA, United States
- 2Department of Chemistry, University of Richmond, Richmond, VA, United States
- 3Department of Mathematics, University of Richmond, Richmond, VA, United States
- 4Department of Computer Science, University of Richmond, Richmond, VA, United States
- 5Digital & Computational Studies Program, Bates College, Lewiston, ME, United States
- 6Department of Biology, Bates College, Lewiston, ME, United States
In the early 2000s, our primarily undergraduate, white institution (PUI/PWI), began recruiting and enrolling higher numbers of students of color and first-generation college students. However, like many of our peer institutions, our established pedagogies and mindsets did not provide these students an educational experience to enable them to persist and thrive in STEM. Realizing the need to systematically address our lack of inclusivity in science majors, in 2012 faculty from multiple disciplines developed the Science, Math, and Research Training (SMART) program. Here, we describe an educational innovation, originally funded by a grant from the Howard Hughes Medical Institute, designed to support and retain students of color, first generation college students, and other students with marginalized identities in the sciences through a cohort-based, integrated, and inclusive first-year experience focused on community and sense of belonging. The SMART program engages first-year students with semester-long themed courses around “real world” problems of antibiotic resistance and viral infections while integrating the fields of Biology, Chemistry, Mathematics, and an optional Computer Science component. In the decade since its inception, 97% of SMART students have graduated or are on track to graduate, with 80.9% of these students earning a major in a STEM discipline. Here, we present additional student outcomes since the initiation of this program, results of the student self-evaluative surveys SALG and CURE, and lessons we have learned from a decade of this educational experience.
Introduction
In 2011, Vision and Change: A Call to Action identified the important need “for undergraduates to understand not only the process of science, but also the interdisciplinary nature of the new biology and how science is closely integrated within society” and outlined a set of competencies to address this necessity (American Association for the Advancement of Science, 2011). These competencies included the ability to (1) apply the process of science, (2) use quantitative reasoning, (3) utilize modeling and simulation, (4) tap into the interdisciplinary nature of science, (5) communicate and collaborate with other disciplines, and (6) understand the relationship between science and society. To achieve these competencies, Vision and Change called for their integration throughout the scientific curriculum with a focus on student-centered learning. Additionally, in 2011, the American Association of Colleges and Universities and Project Kaleidoscope produced the report, What Works in Facilitating Interdisciplinary Learning in Science and Mathematics (Kezar and Elrod, 2012). This report provided strategies for integration and support of student learning across the science, technology, engineering, and mathematics (STEM) disciplines. These two national calls for reforming science education served as guides for the curricular innovation we present here.
At that time and in the decade since, the STEM community also recognized the importance of incorporating inclusive pedagogical practices into our courses, as well as encouraging ourselves and our students to adopt growth mindsets (Dweck, 2006). In their recent essay “Inclusive Teaching,” Dewsbury and Brame note that there are many good reasons for STEM faculty to make their teaching more inclusive and describe an online, evidence-based teaching guide intended to serve as “a resource for science faculty as they work to become more inclusive, particularly with regard to differences in race, ethnicity, and gender” (Dewsbury and Brame, 2019). They note the importance of having a supportive classroom climate, fostering a student’s sense of belonging, and promoting engagement and self-efficacy. Two of the pedagogical choices they cite as promoting engagement and self-efficacy are emphasizing the relevance of coursework to real life and fostering the ability of students to see themselves doing research. These principles of inclusive pedagogy were foundational to our project. While the work of Dewsbury and Brame (2019) was published after we began our curricular development efforts, we were aware of their important work in this space and were heavily influenced by it.
In this research article, we describe a curricular innovation at the University of Richmond (UR) in which we sought to address issues of interdisciplinary integration, STEM retention, inclusivity, and belonging in STEM through the development of our Science, Math, and Research Training (SMART) program. Our main goal aligned with the 2012 national report, Engage to Excel: Producing One Million Additional College Graduates with Degrees in Science, Technology, Engineering and Mathematics, produced by the President’s Council of Advisors on Science and Technology (PCAST). This report called for improved STEM student recruitment and retention in the first 2 years of postsecondary education and stressed the need to provide all students with the tools necessary to succeed and the nation’s need to diversify pathways to STEM degrees (Olson and Riordan, 2012).
With initial support from the Howard Hughes Medical Institute (HHMI), we responded to this national call by creating an integrated course structure grounded in evidence-based practices that would help recruit and retain students from backgrounds traditionally and contemporarily excluded from STEM. Our goal was to develop a curricular and co-curricular environment in which our students could persist and thrive in STEM disciplines, by providing high impact educational experiences early in their careers in an environment that would support their sense of belonging in STEM. The foundational values of the course included rejection of deficit-minded thinking (viewing historically marginalized demographic groups as having inadequacies that have led to under-representation) that was pervasive in our disciplines, embracing the individual and intersectional identities of our students with a focus on their assets, and a belief that each of our students could be supported in their self-empowerment to learn and succeed in STEM (Harper et al., 2009; Ayala et al., 2021; Stanton et al., 2022; Stoddard, 2022). This work required building of instructor, social, and positional self-awareness through reflection and ongoing professional development, as well as significant investment in student relationships and facilitation of supportive cohort communities. This form of “deep-teaching” that positions empathy at the center has been described by Dewsbury and Brame (2019) and we found that pursuit of this model not only brings about more inclusion for students, but also increases instructor engagement (Dewsbury and Brame, 2019).
In what follows, we detail our motivation for developing SMART in 2012, its evolution and expansion over 10 years, and describe student outcomes in the form of self-evaluations and STEM retention. Specific elements of inclusive pedagogy built into the SMART course include integration of scientific disciplines, concentration on critical thinking skills, and application of theory, building supportive community cohorts and relationships, providing authentic research experiences, and focusing on societal impact and social justice. Our philosophy for the course was influenced greatly by the “Persistence Framework” (Graham et al., 2013) that recognizes learning and professional identification as determinants of persistence where early research, active learning, and learning communities contribute to a cycle of increased student confidence and motivation to persist. Our inclusive pedagogy and faculty development efforts were grounded in equity-mindedness, as well as the kind of deeply empathetic, relationship building teaching described by Dewsbury and Brame (2019). We discuss how we built these elements into the program and report on outcomes and student perceptions over the past decade to provide a model for similar institutions looking to build programs with these goals.
Science, math, and research training curricular design
Science, Math, and Research Training intentionally incorporates components of deeply empathetic, relationship building pedagogy (Dewsbury and Brame, 2019), high course structure (Eddy and Hogan, 2014; Freeman et al., 2014), promotion of student metacognition (Tanner, 2012; McGuire, 2015), and liberal use of teaching strategies that promote student engagement and classroom equity (Tanner, 2013). The SMART program consists of four integrated and interdisciplinary courses for first-year students as well as opportunities to gain research experience both in the course and in the following summer. In the first semester, SMART students take two courses: a lab-based integrated biology and chemistry course along with a coordinated Calculus I course. In the second semester, students enroll in a second lab-based integrated biology/chemistry course and Calculus II. In all four courses, fundamental concepts are taught through a “big picture” thematic lens such as antibiotic resistance or infectious disease (see Supplementary Curricular Information). In each semester, the math course is connected to the integrated biology/chemistry course through shared data sets, practice problems, and approaches. The integrated biology-chemistry component of SMART is team-taught with both biology and chemistry instructors attending all classes and labs. Calculus I and II are tightly integrated with the scientific component of SMART via regular conversations among the math and science faculty, as well as having students use the data they generate in the experimental part of SMART for modeling exercises in SMART-calculus. At the end of their first year, students have obtained credit for Calculus I and II, the first semester course of biology, and the first semester course of chemistry (both introductory chemistry and biology at UR are one semester courses). We focused on biology, chemistry, and calculus during the first few iterations of SMART, as these were the most frequently taken first year STEM courses. In later iterations, a SMART computer science course was added. In addition, all SMART students receive funding for a summer research experience at the end of their first year. Additional details for each component of SMART, and the context in which SMART was created, are described in the Supplementary Curricular Information document. We encourage individuals interested in creating a similar program as SMART to peruse this document and reach out to the authors for additional materials.
To date, 238 UR students have completed the two semester SMART program (131 have graduated from UR while another 107 are still undergraduates), with 62% of these students beginning in an immersive summer program, the University of Richmond Integrated Science Experience (URISE, see Supplementary Curricular Information). Additionally, 76% of the URISE participants and 60% of the SMART students fit the National Science Foundation criteria for minority groups underrepresented in STEM. Finally, 46% of the URISE participants and 38% of our SMART students are first-generation college students.
Evaluation of SMART effectiveness
Elements critical to the success of the SMART program include integration of scientific disciplines, developing critical and higher order thinking skills, increasing student sense of belonging, increasing student confidence generally and in laboratory skills specifically, building community, and focusing on societal impact and social justice. Each year, we used two surveys for assessing student perceptions of the success or failure of these elements of the course: the Student Assessment of their Learning Gains (SALG) and the Classroom Undergraduate Research Experience (CURE). The SALG is a free, course-evaluation tool that invites students to reflect on their learning in a specific course and assesses the extent to which certain course aspects influenced their learning. The SALG has been assessed and validated across various disciplines (Seymour et al., 2000; Carroll, 2012) and we have collected data using both its Likert-style and free response questions. The CURE survey was created in 2005, designed to measure student experiences in research-like courses, and featured in numerous publications for course assessment purposes (Lopatto, 2009; Auchincloss et al., 2014). This post-course survey includes Likert-style questions from four areas: estimate of learning gains in the course elements (25 questions), estimates of learning benefits (21 questions), overall evaluation of the experience (four questions), and science attitude questions (22 questions). Until 2018, individual programs could submit their CURE survey results to a national database and receive a report comparing their program to national data sets. The academic year 2017–18 was the final year for the surveys to be offered for centralized data collection and reporting, but benchmark CURE statistics from 2015 to 2018 are available for general use. The SALG was distributed to each student at the end of the course, and here we report on anonymized data collected from 70.1% of the total students since the start of the program. For the CURE, we compare results from an early cohort (collected spring 2016) and combined results from the most recent two cohorts (2021 and 2022) to benchmark statistics from the 2015 to 2018 national database (Supplementary Figure S3 includes CURE results from other years). Finally, as the overarching goal of SMART is to support the persistence of our students in STEM, we present the available data for our students beyond the first-year SMART experience.
Our goal in developing the SMART program was to remove the barriers that impede the persistence, retention, and success of underrepresented students in STEM disciplines. In this work, we define and measure persistence using student enrollments in subsequent STEM courses, progression to graduation with a major and/or minor in a STEM discipline, as well as post-baccalaureate engagement in a STEM career. To achieve this goal, we developed pedagogies based on integrated, research-based topics taught in a supportive and empathic fashion, emphasizing a growth mindset approach. We describe the results of these efforts in the following section.
Results
With a decade of experience developing, revising, and teaching the SMART course, we sought to determine its overall effectiveness at achieving the desired outcomes. We mined the SALG and CURE student assessments and collected student data post-SMART. The responses to the SALG Likert-style questions were aggregated and reported in Supplementary Figure S1. In the following subsections, we report student perceptions and outcomes over the time period in which we have taught SMART (2013–2022).
Integration
A founding principle of SMART is building a course that integrates scientific disciplines with Mathematics (Kezar and Elrod, 2012). We hypothesized that exposing students to these subjects in an intentionally coordinated and integrated manner would increase their interest and persistence in science.
The SALG data revealed that 96% of students felt that the “instructional approach taken in class” helped their learning, giving a “good” or “great” Likert response. Similarly, 95% of students gave good or great Likert responses when asked whether the “class topics, activities, reading and assignments fit together” to help their learning and 92% of students self-assessed that they made good or great gains in integration through “connecting key class ideas with other knowledge” (Supplementary Figures S1E,F).
These data are supported by free response answers throughout the SALG. When asked how SMART changed attitudes toward the subject and what will be carried into future classes, students were overwhelmingly positive (>90%) in their responses and some specifically described the integrated nature of the course. For instance, one student wrote that “SMART has helped me realize that all the sciences are much more connected than I thought. It has helped me appreciate most branches of science. It has also helped me become more confident talking about science when it relates to labs and research.” Similarly, another student remarked that they learned that “the sciences and other subjects are all interdisciplinary, and that they all bounce off of each other and have numerous connections.” Finally, while students may have been aware that the course involved Biology and Chemistry, some remarked that the integration developed a more complete view of how science works, as indicated by a student who remarked: “I thought this class is just a combination of Biology and Chemistry, but what I learned from this class is way more than these. The research experience and group work helps me a lot to understand the real world of science.” Taken together, the SALG data show that students self-assess that they have gained a strong sense of subject integration through SMART, validating the initial goals of our program.
Similarly, in the CURE survey, we consistently see that SMART students on average reported large learning gains compared to the national benchmarks on overall assessment of the course (Figure 1; Supplementary Figure S2). In particular, two items related to learning showed particularly large gains (Table 1) as to how students regard the ability of the SMART course to learn the subject material and scientific research.
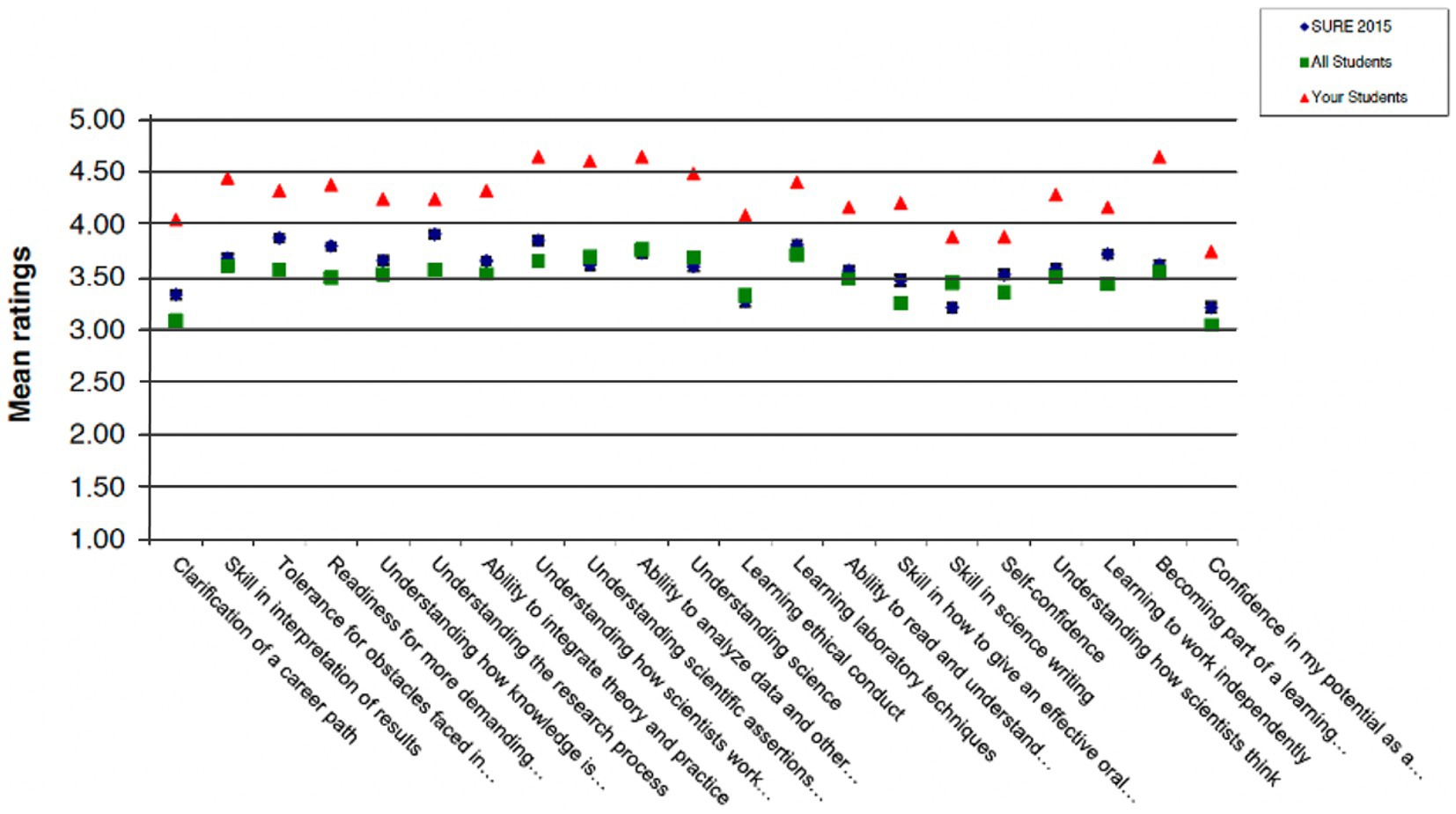
Figure 1. Course-based undergraduate research experience (CURE) self-reported learning gains. Representative CURE mean survey responses from 2016, comparing answers to Likert-style questions submitted by Science, Math, and Research Training (SMART) students (“Your Students,” red triangles) to all students who completed the CURE survey after the 2015/16 academic year (“All Students,” green squares). Also included for reference are responses of students who completed the Summer Undergraduate Research Experience (SURE) survey in 2015 (blue symbols). Additional CURE data are reported in Supplementary Figure S2.
Overall, the CURE data show that students found the SMART experience to be effective at supporting their learning gains across many measures, including those impacted by the integration of scientific disciplines through our course-based research experience. Indeed, in years where the CURE instrument data are also available for Summer Undergraduate Research Experience (SURE; Figure 1) students at other institutions, SMART students reported higher learning gains as compared to the mean ratings for students at other institutions in summer research experiences as well as those in course-based research.
Critical thinking and application
Through intentional course-design, students were guided in building their critical thinking skills and viewing science as an iterative, investigative process. Several Likert questions and free response prompts in the SALG asked students to self-assess what gains they made in these areas. A high percentage of SMART students rated their gains as “good” or “great” in how the course helped them integrate “applying what I learned in this class in other situations” (91%), “using a critical approach to analyzing data and arguments in my daily life” (92%), and “using systematic reasoning in my approach to problems” (90%; Supplementary Figure S1E). Similarly, over 90% of SMART students felt they made “good” or “great” gains in the following skills: identifying patterns in data, recognizing a sound argument and appropriate use of evidence, and developing a logical argument (Supplementary Figure S1C).
Given the opportunity to respond to the SALG free response questions about how the instructional approach helped their learning and what they will carry with them into future classes, multiple students (~18%) brought up critical thinking or problem solving. For instance, one student wrote: “I will carry the problem-solving skills that I had to use in this class. If an experiment went wrong we were not told why it could have gone wrong. We would have to figure out the reason why. I am now okay with not knowing everything because I can work through it.” Similarly, some students appreciated the focus away from memorization to more complex levels of Bloom’s Taxonomy. As one student put it, “The way our biology professor [planned the] curriculum was brilliant. Having us work [through] case [studies] in class to reinforce the subject matter really drove home the main points and forces us to think outside the box and move away [from] memorization to understanding.”
Through an integration of these subjects, students were encouraged to think critically about the role of science and how they could apply what they learned in SMART more broadly. Several CURE questions regarding benefits of the course delve into how students perceive their learning gains in areas related to critical thinking and application. SMART students self-reported higher gains than average in these areas, particularly those shown in Table 2. Based on these metrics, SMART students perceive that they are making more critical thinking gains than in other courses from the CURE dataset that contain a research component (see Figure 1; Supplementary Figure S2).
Building community
As discussed in the “Description and history of the SMART program development” section, discussions and focus groups with some of our students and alumni from backgrounds minoritized in STEM revealed that a lack of community might contribute to a retention deficit and an equity gap in the sciences. We developed SMART to directly address this experience by using a cohort model (e.g., after Meyerhoff Scholars, STEM Posse; Maton et al., 2016), using an on-campus summer immersion experience prior to fall orientation (URISE), and structuring the SMART class to foster community-building.
Responses on the CURE surveys were consistently in strong agreement with the statement that becoming part of a learning community was a benefit of the course (see Figure 1; Supplementary Figure S2). Similarly, the SALG data showed the importance in community-building for our course. Students consistently indicated that this aspect of the course made an impact on them, illustrated by these sampled comments to the SALG question, “Please comment on how the support you received from others helped your learning in this class”:
“I liked the class discussion and how it felt like a family in class. It did not feel like I was in a lecture hall where the teacher does not know my name.”
“The instructional approach is very inclusive and helped me become comfortable with being able to ask for help and not be scared to not understand something as quick as some of my classmates do. I really appreciate when the professors say ‘there is no dumb question,’ it makes me feel so much more comfortable to ask.”
“The professors were an excellent resource that I felt comfortable asking for help. They made themselves very available to all students. My peers formed very close knit groups early in the year, and this camaraderie led to frequent collaboration. Group projects, such as the posters, allowed us to consider other people’s perspectives on a common task.”
Community building was consistently emphasized by the instructors in the course, and students reported that working with peers outside of class (85% good or great) and inside of class (88% good or great) helped their learning. An aim of SMART was to create a community of student learners who would work with each other to develop their understanding of the material, and the SALG data indicate that this is the case (see Supplementary Figure S1J).
Connecting social issues to science
From the initiation of the course, SMART has included a social justice component with its theme of antibiotic resistance in the fall and human immunodeficiency virus (HIV) in the spring. Recently, we have increased this emphasis in both the biology/chemistry and mathematics portions to include modules on the ethical implications of antibiotic overuse and misuse, as well as a module amplifying health disparities and lack of access to treatments and medications in lower resourced and minoritized communities. We have developed social justice and bioethics-based case studies that are incorporated into the lecture and laboratory component of the course, and we have included social and ethically oriented questions on student assessment materials.
The SALG data reveal students appreciate the “real world” applicability and “relevance to the modern world” aspect of the course. A high percentage of SMART students rated their gains as “good” or “great” in how the course increased their “interest in discussing the subject area with friends or family” (96%) and “Applying what I learned in this class in other situations” (see Supplementary Figures S1D,E). The CURE survey includes questions about attitudes toward science. Regarding the statement “Science is not connected to non-science fields such as history, literature, economics, or art,” SMART classes from the beginning disagreed more strongly than the (national) comparison population (SMART 2016: 2.02; SMART 2021–22: 2.08; National Benchmark 2.20). Likewise, regarding the statement, “Students who do not major/concentrate in science should not have to take science courses,” SMART classes from the beginning disagreed more strongly than the (national) comparison population (SMART average 2.16; National Benchmark 2.45). As SMART has progressed from 2014 to the present, instructors have been intentional in adding inclusive pedagogical practices that connect science to social issues and non-science academic fields.
Student persistence in STEM
The implementation of SMART specifically sought to retain our student population who entered college with an excitement and interest in the sciences but did not find the necessary support and inclusive pedagogies to nurture this interest. SMART addresses these issues directly, from recruiting students who identify with groups who have been marginalized in STEM to directly presenting data that demonstrate that STEM systems and cultures are the problem, not them. Throughout the courses, instructors continually stress that students can succeed at these subjects, encouraging the use of meta-cognition, embracing a growth mindset, and seeking instructor and peer support (Dweck, 2006; Nottingham and Larsson, 2019; Richardson et al., 2020).
A strong majority of students report making good or great gains in their “willingness to seek help from others when working on academic problems” (89%) and “confidence that [they] can do this subject area” (93%). Some students found that the course cemented their interest in the subject, illustrated by the following student quote: “This class has made me love biology and chemistry even more, and led me to decide on majoring in BMB [Biochemistry and Molecular Biology]. The class was challenging, so I often had to advocate for myself and seek extra help. This impacted my attitude in that I am completely comfortable with going to my professors with questions.” Alternatively, some students found that they could succeed in a subject that piqued their interest but caused apprehension. In their SALG surveys, several students revealed that SMART changed how they thought about these subjects, giving them newfound confidence as they continue through science.
“Before I felt that I could never be good at bio, now I feel confident in my knowledge of the subject matter and am more willing [to] seek the help needed to succeed.”
“I came into this class feeling very uneasy about the biology portion. Now I feel like biology is one of my stronger areas.”
“I was not very confident in biology coming into college and now it’s my best subject.”
Emphasizing student persistence is an integral part of the SMART curriculum, and the data show that students internalize this message (see Supplementary Figure S1D).
Student outcomes beyond SMART
University of Richmond enrollment data from 2010 to 2013 showed 20–33% of the students in our first-year science and math courses were from minoritized backgrounds and this representation in science and math courses matched their representation in the overall class demographics. However, when we analyzed the percentage of students from minoritized groups who were graduating with a STEM degree, those numbers fell to between 4 and 9%. Closer examination revealed that most of those science- and math-interested first-year students graduated from UR by majoring in a non-STEM field.
As one measure of the effectiveness of the program, we tracked the 238 SMART students from the initial cohort in 2013 to the present. Of these students, 97% have graduated or are on track to graduate from UR. Of the 131 students who have graduated, 82% graduated with STEM majors (Figure 2). The next largest major was health care studies which is considered to be STEM adjacent (5%). Of those students who did not major in STEM, 26% of the health care studies and other non-STEM majors added STEM minors to their majors. Altogether, 87% of the students gained academic credentials in STEM.
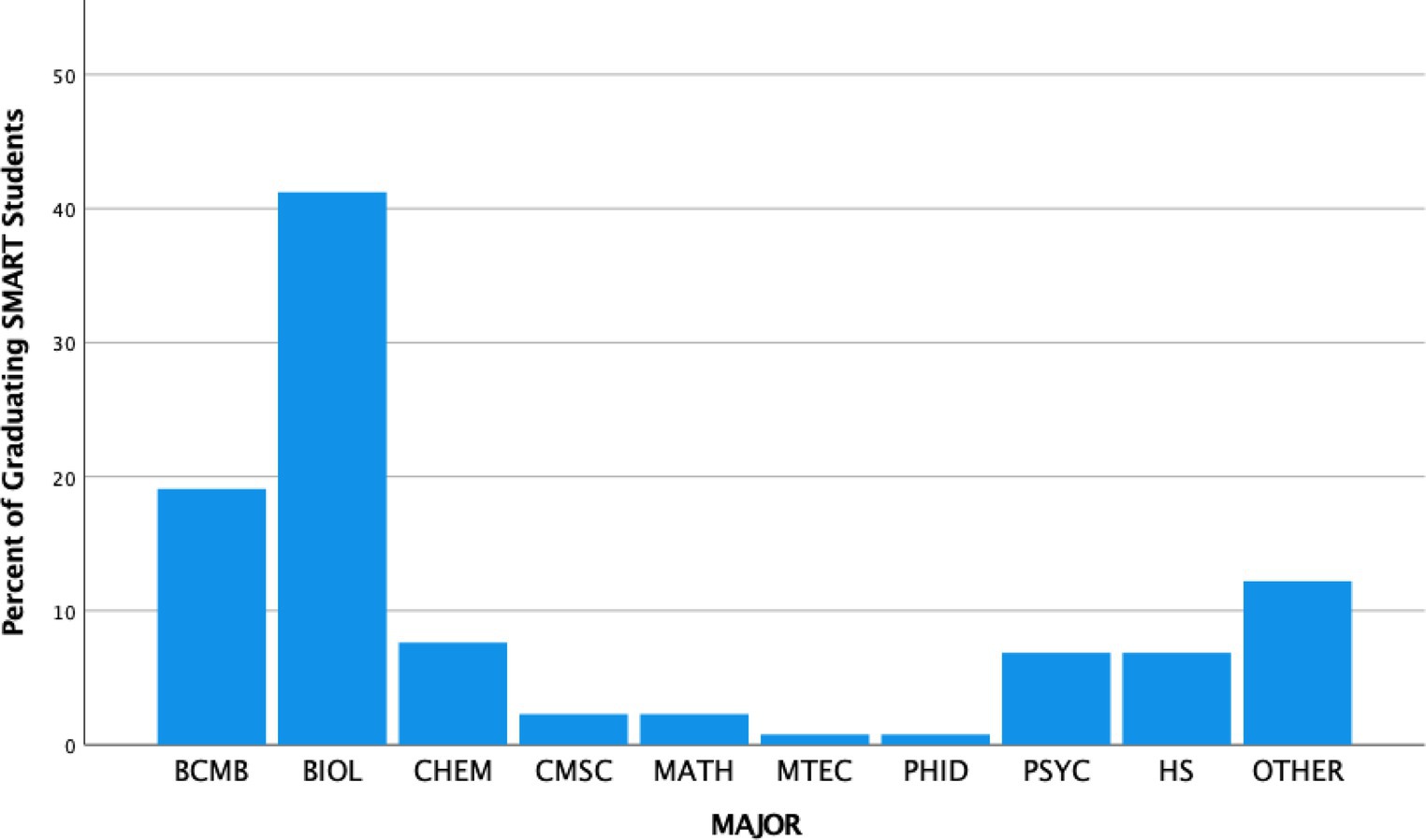
Figure 2. Primary major choices of SMART graduates. Students were tracked after graduation (n = 131). BCMB, Biochemistry & Molecular Biology; BIOL, Biology; CHEM, Chemistry; CMSC, Computer Science; MATH, Mathematics; MTEC, Mathematical Economics; PHID, Physics; PSYC, Psychology; and HS, Health Studies.
Science, Math, and Research Training students persisted in science and this persistence had a profound effect on the growth of first generation and under-represented minority students graduating from UR (Figure 3). The overall number of UR students graduating in STEM fields rose from 2012–2014 (12.3% average of overall class) to 2015–2022 (16.9% average). First generation (1st gen) and under-represented minority (URM) students majoring in STEM have risen dramatically during these 10 years, making up over half of the graduating seniors in 2022 (Figure 3A). We additionally show how the majors for these targeted groups have changed over time (Figure 3B); notably, we have seen an increase in Biology, Chemistry, and Biochemistry & Molecular Biology (BMB) majors since 2012–2014.
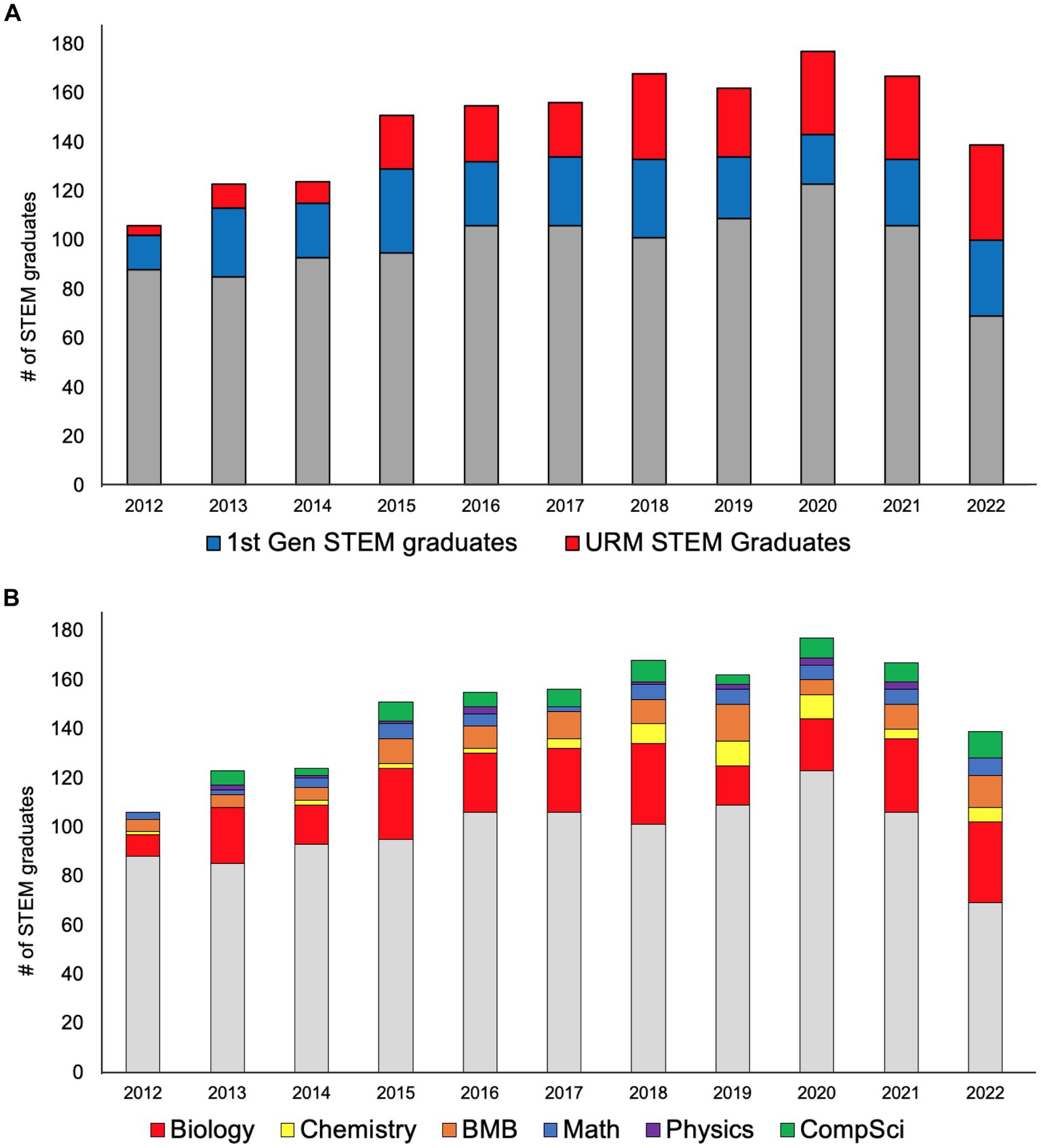
Figure 3. SMART target populations at UR majoring in STEM. (A) Demographics of STEM graduates at UR since the SMART program was established in 2012. First generation students (blue bars) and under-represented minority (URM) students (red bars) have shown an increase in choosing STEM majors over time. The bars represent total STEM majors, with students outside of first Gen and URM in gray. (B) URM + first Gen STEM graduates by major (color-coded) over time at UR since the SMART program was established in 2012. Gray bars represented students outside of the SMART targeted demographics.
An important component of SMART is the granting of a summer research fellowship, which students usually complete after their first year in SMART. Of the SMART graduates, 93% engaged in a summer research project for at least 8 weeks during at least one summer. The median number of summers SMART students who were STEM majors engaged in research was 2 (min 0 and max 4); the median for other majors was 1 (min 0 and max 3). All of the Health Studies majors conducted at least one summer of research.
Most of the students (85%) who completed SMART took the “next” course in biology (Integrated Biological Principles I). Of these students, 68% continued to the next course in biology (Integrated Biological Principles II). Likewise, 85% took the next chemistry course (Organic Chemistry I); of these 202, 72% continued to the next course in chemistry (Organic Chemistry II). In addition, 11% took the next course (either multivariate calculus, linear algebra, or both) in mathematics and 19% took the first course in computer science.
SMART student post-baccalaureate outcomes
Our goal in establishing the SMART program was to provide an engaging and inclusive curriculum supported by a cohort-based community so that all students interested in STEM could flourish. We attempted to build a program that focused on our students’ sense of belonging as well as their academic success. If students found their passion in a field outside of STEM we celebrated those victories, but our main goal was to ensure that students did not leave STEM because they felt that they were lacking in talent, that they were not supported, or that they could not see themselves in STEM. As such, another measure of our students’ success is their post-baccalaureate career choices and placements. Of the 131 students from the SMART program who have graduated in 2019 or earlier (85% with a STEM degree), 60% pursued graduate school in science, health or health-policy (MD, PhD, PharmD, MPH, nursing programs, etc.). If we include all students who have graduated (2022 or earlier), 39% have pursued graduate work in these fields. This is a remarkable retention and success rate; for calibration, approximately 11% of U.S. students who receive a Bachelor of Science degree go on to graduate or medical school (National Science Foundation, 2020; Association of American Medical Colleges, 2021). Of those SMART students who did not pursue additional education, 85% are gainfully employed, some in STEM related fields (Data Scientist, Analyst, Contact Tracer, Operations Specialist, Laboratory Manager, Science Journalist, Production Assistant, etc.).
Discussion
Science, Math, and Research Training was designed over a decade ago as a course to address a specific issue—namely, our institution, our STEM Departments, and curricula were all deficient in their support of students from groups that have been and still are underrepresented in the sciences, but who express an interest in these disciplines. We intentionally sought to create a course that combined biology, chemistry, and math, with clear goals of building community and sense of belonging through a supportive and inclusive classroom environment, components missing in typical courses. We have found that the integration of the biology, chemistry, and calculus curricula focused on applications of science, is an important aspect of the SMART course. Integrating such topics allows students to view science as inherently interdisciplinary and pertinent to solving important real-world problems—not as disparate fields with separate details, nomenclatures, and techniques that must be learned out of context. Learning in this way motivates our students, and they begin to develop the work-ethic, focus, and discipline necessary to become successful scientists.
We do not believe, however, that the integration of disciplines and the focus on real-world problems alone would have led to the student success that we observe in SMART. It is clear from our student data as well as our experiences, that the cohort-based model that focuses on community building, self-empowerment, sense of belonging, respect for identities, and a belief that all students can succeed in STEM is a key component of the course. Students learn that they can make a difference. The focus on providing students with resources, inclusive pedagogies, academic and social supports, and kindness from their instructors and their peers is the heart of SMART. Faculty who have taught in the program for multiple years find a higher proportion of SMART graduates to remain in close relationship with their SMART faculty mentors than graduates from other courses those faculty teach. Many program graduates report back that SMART was a defining experience in their time at UR and that the relationships they formed with their peers and professors helped them feel a sense of community and belonging that lasted beyond their time in the course.
Based on our initial program goals, SMART has been a success. Our SMART graduates have persisted in science, with 87% gaining credentials in STEM. Further, that 60% of all SMART graduates continue on to science careers is a remarkable statistic, indicative of these students recognizing their accomplishments and place in STEM with a sense of empowerment in a field in which many of our students have been under-represented. Our SALG and CURE data indicate that SMART students perceive that they have made important academic gains through their time in the program. While we recognize the caveat that the SALG and CURE methodologies report self-reported gains and might be viewed as subjective measures of student achievement, the use of these tools has been well-documented in the literature (Seymour et al., 2000; Auchincloss et al., 2014) and our results compare favorably to the available benchmark scores. These results reveal that our efforts to build community, connect with our students, and encourage peer support have played a role in the persistence of these students beyond SMART. SMART students particularly appreciate the ability to connect the science and mathematics that they were learning to the “real world,” highlighting the importance of creating STEM curricula that capture student interest and are not siloed in individual disciplines.
As we move our program forward, there are additional areas we would like to explore about the SMART program. While our inclusive and integrative design of SMART was a structured approach to building the components, we felt were necessary to achieve retention and self-efficacy in the sciences, we recognize that what the students continue to bring to the course, in terms of community and persistence, is instrumental in its success (Stanton et al., 2022). In SMART, we try to help our students leverage the bonds that they form with us and with each other to help them find their place in a PWI, which creates an environment of persistence and achievement and the recognition that their participation matters. The SMART program, however, is clearly not solely responsible for the success of its selected students and, at this time, does not have the capacity to support all students who might benefit from the program. While we intentionally seek out students who have been accepted into our institution from our target groups (see SMART Curricular Design), students do “self-select” and apply to be accepted into our program—there are students who are interested in science that we miss. In addition, our institution has added and developed programs that fit with the stated values of both “Inclusivity and Equity” and “Diversity and Educational Opportunity.” SMART clearly aligns with these stated values and the support of the University has been instrumental in allowing the program to thrive beyond the initial support from HHMI. In future studies, we hope to investigate the aspects of community cultural wealth that are used by our students once their time in SMART is over (DiMaggio, 1982; Ayala et al., 2021; Stanton et al., 2022).
While the SMART course has maintained similar topics and learning objectives over the years, we have been more intentional with discussing how science is interwoven in all aspects of society. As such, we have added components to expressly discuss scientific and environmental racism among other social justice issues. Anecdotally, we have found that students value these discussions and gravitate toward them. Moreover, the increase in disagreement to the CURE statement “Science is not connected to non-science fields such as history, literature, economics, or art” could be attributed to including more of these discussions in the course.
We hope that the design of the SMART program can be used by similar institutions seeking to provide support for first generation college students, students of color, and other students with marginalized identities who express an interest in STEM. Our materials are available via request, and we are eager to work with colleagues who hope to develop a program like SMART at their institution. While certainly facing challenges, our students have had a remarkable rate of success, and the rewards from the implementation of this program on the students and instructors have been profound.
Data availability statement
The original contributions presented in the study are included in the article/Supplementary material, further inquiries can be directed to the corresponding authors.
Author contributions
SJ, AB, LC-S, JC, EG, FG, CH, HH, SH, BK, PK, BL, ML, GL, EM, KN, MN, JS, AS, MT, JW, DW, AH, KH, CP, and BP contributed to the teaching of the course. SJ, EG, MN, MT, BL, AH, KH, CP, and BP organized the data and cowrote the initial draft of the manuscript. SJ, DW, MN, BL, GL, FG, AH, KH, CP, and BP revised and edited the manuscript for submission. All authors contributed to the article and approved the submitted version.
Acknowledgments
We would like to sincerely thank the SMART students for their dedication, perseverance, and camaraderie. This work was initially supported by funding from the HHMI Science Education program and has subsequently received significant support from the University of Richmond and its Integrated and Inclusive Science program.
Conflict of interest
The authors declare that the research was conducted in the absence of any commercial or financial relationships that could be construed as a potential conflict of interest.
Publisher’s note
All claims expressed in this article are solely those of the authors and do not necessarily represent those of their affiliated organizations, or those of the publisher, the editors and the reviewers. Any product that may be evaluated in this article, or claim that may be made by its manufacturer, is not guaranteed or endorsed by the publisher.
Supplementary material
The Supplementary material for this article can be found online at: https://www.frontiersin.org/articles/10.3389/feduc.2023.1152339/full#supplementary-material
References
American Association for the Advancement of Science (2011). Vision and change: A call to action, final report. Available at: https://visionandchange.org/
Association of American Medical Colleges (2021). Total Enrollment by U.S. MD-Granting Medical School and Sex, 2017–2018 through 2021–2022. Available at: https://www.aamc.org/media/6101/download?attachment
Auchincloss, L. C., Laursen, S. L., Branchaw, J. L., Eagan, K., Graham, M., Hanauer, D. I., et al. (2014). Assessment of course-based undergraduate research experiences: a meeting report. CBE Life Sci. Educ. 13, 29–40. doi: 10.1187/cbe.14-01-0004
Ayala, M. J., Carter, J. K., Fachon, A. S., Flaxman, S. M., Gil, M. A., Kenny, H. V., et al. (2021). Belonging in STEM: an interactive, iterative approach to create and maintain a diverse learning community. Trends Ecol. Evol. 36, 964–967. doi: 10.1016/j.tree.2021.08.004
Carroll, S. B. (2012). Science education and civic engagement: the next level. ACS Symp. Ser. 217–242. doi: 10.1021/bk-2012-1121.ch014
Dewsbury, B., and Brame, C. (2019). Evidence based teaching guide: inclusive teaching. CBE Life Sci. Educ. 18:fe2. doi: 10.1187/cbe.19-01-0021
DiMaggio, P. (1982). Cultural capital and school success: the impact of status culture participation on the grades of U.S. high school students. Am. Sociol. Rev. 47:189. doi: 10.2307/2094962
Eddy, S. L., and Hogan, K. A. (2014). Getting under the Hood: how and for whom does increasing course structure work? CBE Life Sci. Educ. 13, 453–468. doi: 10.1187/cbe.14-03-0050
Freeman, S., Eddy, S. L., McDonough, M., Smith, M. K., Okoroafor, N., Jordt, H., et al. (2014). Active learning increases student performance in science, engineering, and mathematics. Proc. Natl. Acad. Sci. 111, 8410–8415. doi: 10.1073/pnas.1319030111
Graham, M. J., Frederick, J., Byars-Winston, A., Hunter, A.-B., and Handelsman, J. (2013). Increasing persistence of college students in STEM. Science 341, 1455–1456. doi: 10.1126/science.1240487
Harper, S. R., Patton, L. D., and Wooden, O. S. (2009). Access and equity for African American students in higher education: a critical race historical analysis of policy efforts. J. High. Educ. 80, 389–414. doi: 10.1353/jhe.0.0052
Kezar, A., and Elrod, S. (2012). Facilitating interdisciplinary learning: lessons from project kaleidoscope. Change Magaz. High. Learn. 44, 16–25. doi: 10.1080/00091383.2012.635999
Lopatto, D. (2009). Science in Solution: The Impact of Undergraduate Research on Student Learning. Tucson, Arizona: Research Corporation for Science Advancement.
Maton, K. I., Beason, T. S., Godsay, S., Domingo, M. R. S., Bailey, T. C., Sun, S., et al. (2016). Outcomes and processes in the Meyerhoff scholars program: STEM PhD completion, sense of community, perceived program benefit, science identity, and research self-efficacy. CBE Life Sci. Educ. 15:ar48. doi: 10.1187/cbe.16-01-0062
McGuire, S. Y. (2015). Teach Students How to Learn: Strategies You Can Incorporate Into Any Course to Improve Student Metacognition, Study Skills, and Motivation. Sterling, Virginia: Stylus Publishing.
National Science Foundation (2020). Survey Graduate Students and Postdoctorates in Science and Engineering: Fall 2020. Available at: https://ncses.nsf.gov/pubs/nsf22319/
Nottingham, J., and Larsson, B. (2019). Challenging Mindset: Why a Growth Mindset Makes a Difference in Learning—and What to Do When It Doesn’t. Thousand Oaks, California: Corwin.
Olson, S., and Riordan, D. G. (2012). Report to the president, engage to excel: Producing one million additional college graduates with degrees in science, technology, engineering, and mathematics (President’s Council of Advisors on Science and Technology (U.S.) & United States, p. 130). Executive Office of the President.
Richardson, D. S., Bledsoe, R. S., and Cortez, Z. (2020). Mindset, motivation, and teaching practice: psychology applied to understanding teaching and learning in STEM disciplines. CBE Life Sci. Educ. 19:ar46. doi: 10.1187/cbe.19-11-0238
Seymour, E., Wise, D. J., Hunter, A.-B., and Daffinrud, S. M. (2000). “Creating a better mousetrap: On-line student assessment of their learning gains” in National Meetings of the American Chemical Society Symposium.
Stanton, J. D., Means, D. R., Babatola, O., Osondu, C., Oni, O., and Mekonnen, B. (2022). Drawing on internal strengths and creating spaces for growth: how black science majors navigate the racial climate at a predominantly white institution to succeed. CBE Life Sci. Educ. 21:ar3. doi: 10.1187/cbe.21-02-0049
Stoddard, S. V. (2022). The benefits of enlightenment: a strategic pedagogy for strengthening sense of belonging in chemistry classrooms. Educ. Sci. 12:498. doi: 10.3390/educsci12070498
Tanner, K. D. (2012). Promoting student metacognition. CBE Life Sci. Educ. 11, 113–120. doi: 10.1187/cbe.12-03-0033
Keywords: course-based undergraduate research experience (CURE), SALG, curricular innovation, HHMI, science technology engineering mathematics (STEM), minoritized students
Citation: Jones S, Blake A, Corado-Santiago L, Crenshaw J, Goldman E, Gomez F, Hall C, Hoke H, Holmes S, Kornegay B, Kwarteng P, Lawson B, Leber M, Leconte G, Modeste E, Nolin K, Norris M, Santinni Roma J, Swackhammer A, Torres M, Wares J, Williams DE, Hill A, Hoke K, Parish C and Pierce BD (2023) A SMART decade: outcomes of an integrated, inclusive, first-year college-level STEM curricular innovation. Front. Educ. 8:1152339. doi: 10.3389/feduc.2023.1152339
Edited by:
Desiree Forsythe, Chapman University, United StatesReviewed by:
Stanley M. Lo, University of California, San Diego, United StatesBryan Dewsbury, Florida International University, United States
Copyright © 2023 Jones, Blake, Corado-Santiago, Crenshaw, Goldman, Gomez, Hall, Hoke, Holmes, Kornegay, Kwarteng, Lawson, Leber, Leconte, Modeste, Nolin, Norris, Santinni Roma, Swackhammer, Torres, Wares, Williams, Hill, Hoke, Parish and Pierce. This is an open-access article distributed under the terms of the Creative Commons Attribution License (CC BY). The use, distribution or reproduction in other forums is permitted, provided the original author(s) and the copyright owner(s) are credited and that the original publication in this journal is cited, in accordance with accepted academic practice. No use, distribution or reproduction is permitted which does not comply with these terms.
*Correspondence: April Hill, ahill5@bates.edu; Kathy Hoke, khoke@richmond.edu; Carol Parish, cparish@richmond.edu; B. Daniel Pierce, bpierce@richmond.edu