- 1Eindhoven School of Education, Eindhoven University of Technology, Eindhoven, Netherlands
- 2Department of Industrial Design, Eindhoven University of Technology, Eindhoven, Netherlands
Engineering education more and more adopts Challenge Based Learning (CBL). In CBL, students learn in groups by taking on open and challenging projects that draw on a broader STEM knowledge base. The challenges reflect and mimic STEM professional core practices (e.g., higher education) or authentic but pedagogically simplified versions (e.g., secondary education). Challenges also connect to societally relevant themes and issues. CBL thus seems well suited to helping students build a positive STEM identity (seeing oneself and being recognized by others as a STEM person c.q., STEM professional). However, CBL is a very new educational concept with little empirical research yet available. Therefore, this study explores the question of CBL's contribution to STEM identity development at a conceptual level. What, conceptually, can CBL be expected to contribute to STEM identity development? We first explore the concept of CBL as it is currently defined to find its strengths and weaknesses and improve it. We then look for empirical evidence on the effects of conceptually akin education (such as design-based learning and project-based learning) on STEM identities. When learning effects can be traced using educational theories to conceptual features they share with CBL, these can be building blocks for our modeling of CBL's contribution to the development of STEM identities. Current definitions of CBL were found to be purely enumerative and did not clearly distinguish CBL from related concepts such as problem-based learning (PBL) or Design-Based Learning (DBL). A new definition for CBL is proposed that is based on an underlying pedagogical vision. It is concluded that CBL and related forms of education can be expected to facilitate the construction of STEM identities, primarily through a combination of a motivation boost, a higher perception of competence and the enjoyable and experiential orientation to STEM that it provides students. However, CBL seems limited with respect to the development of STEM identities in vocational or higher education.
Introduction
STEM identity—seeing oneself and being recognized by others as a STEM person (Carlone and Johnson, 2007)—is known to play a crucial role in raising interest in STEM. Both pursuing STEM careers (or not) and the accessibility of STEM education for underrepresented minority students (URM students) depend on STEM identity formation (Taconis and Kessels, 2009; Hazari et al., 2010).
Open and authentic learning environments have recently been shown to promote the development of a STEM identity and help increase interest in STEM and STEM careers. A new form of such education is Challenge Based Learning (CBL hereafter). This educational concept emerged particularly in engineering education (e.g., Mitra, 2018). Various universities worldwide are changing their education to become more “challenge-based” (Graham, 2018). In CBL, students work on open real-life engineering challenges. These typically require expertise from various (engineering) disciplines or other professional fields. Challenges reflect nowadays professional practice. They also relate to the significant societal challenges of our time (van den Beemt et al., 2021). CBL aims to combine the learning of engineering knowledge and skills with the development of general skills and professional attitudes. Furthermore, it aims to let students gradually develop into increasingly self-steering and responsible professionals. Put differently: CBL aims to train engineers with broad and societally connected professional STEM (engineering) identities.
Although originating in engineering education, the concept of CBL is defined broadly. The concept appears not to be restricted to engineering education and can be applied to various forms of (non-)formal STEM education.1 Hence, it is vital to explore how CBL could help student in building STEM identities which is the central question of this study.
Because CBL is such a new concept, we cannot answer the question directly with empirical results on the effect of CBL on STEM identities. Hence, this study explores on a conceptual level what may be expected of CBL's contribution to the development of STEM identities. It aims to analyze the concept of CBL and, from there, explore how it can be further developed for its optimal use for STEM identity development. It also addresses some (theoretical) implications for the design (and implementation) of CBL education for that purpose.
We first examine current definitions of CBL to understand the concept better. Next, we explore the concept of STEM identity and its main components. This analysis already shows some strengths and weaknesses of the concept of CBL and its current definition. Therefore, we next propose a more sophisticated definition of CBL that avoids these weaknesses and builds explicitly on educational theory.
We then take a side step and look at empirical material on cases of CBL-like education, i.e., teaching based on educational concepts such as problem-based learning (PBL) that share some of their basic (defining) characteristics with CBL. For such CBL-like education, we look for indications of links between these basic features shared with CBL on the one hand and the development of STEM identities and their components on the other hand. Those that can be understood as a logical consequence of recognized educational theories provide the building blocks for a model-based view of the (potential) effect of CBL on the construction of STEM identity. As an example, we can focus on “open group work”, a basic (defining) feature of CBL that it shares with (most forms of) PBL and DBL. Suppose empirical evidence was to be found that “open group work” in (these forms of) PBL or DBL correlates with higher student motivation. We could then look for an established theory that supports the interpretation of this as a causal mechanism. In this case, self-determination theory suggests that “open group work” can increase student motivation because it increases student autonomy (Deci and Ryan, 2008). So, combining the above, we can assume that also in the case of CBL, “open group work” would contribute to increased student motivation.
All in all, this would imply that “open group work” would be an ingredient in CBL underlying increased student motivation. In this way, empirical research on PBL and the like can contribute to our theoretical expectation of CBL's contribution to the development of STEM identity. It is a theoretical expectation as the use of established educational theories is crucial in the above reasoning. We will be building a theoretical model of CBL's impact on identity development.
What is Challenge Based Learning?
Challenge Based Learning (CBL) is a relatively new educational format. Its purest form uses open-ended and meaningful challenges drawing on a broad STEM knowledge base. The challenges are open-ended and often “ill-defined” problems. Students work in groups and actively connect to stakeholders and other parties to explore the challenge and then specify their projects within the scope of the challenge.
The concept has emerged as part of a movement to renew engineering education by putting a stronger emphasis on Engineers' capacities to collaborate in interdisciplinary teams, connect to customers, and take into account societal issues (Graham, 2018; Mitra, 2018; TU/e, 2018; Loohuis and Bosch-Chapel, 2021). Its conceptualization draws on ideas and theories from various traditions such as social constructivism (Scardamalia and Bereiter, 2007), context-based STEM education (Taconis et al., 2016), situated learning (Herrington and Oliver, 2000), and Science Technology Society (Yager, 1996).
Various authors define CBL differently (Lin and Chen, 2017; Taconis, 2021b; van den Beemt et al., 2021). In addition, the term is used in a variety of educational contexts, in primary education, secondary education, higher engineering education, and (independently) by Apple company (Nichols and Cator, 2008), further adding to the diversity of definitions. Hence, the term seems best understood as indicating a “family” of educational approaches that share common characteristics and fundamental beliefs about what education should aim to achieve (e.g., knowledge, competence, and general skill) and how these learning outcomes can be achieved (Thomas, 2000; van den Beemt et al., 2022). This study explores CBL as it is currently used in engineering education. It is usually defined by listing defining characteristics. Several such lists of defining features exist, such as the list developed at the authors' home university (TU/e, 2018), or the list by Gallagher and Savage (2020). The latter comprises the following elements: global themes, real-world challenges, collaboration, technology, flexibility, multidisciplinarity (e.g., experts contributing from their disciplinary backgrounds), creativity and innovation, and the use of “open challenges” rather the predefined problems. It turned out that such lists of characteristics often overlap, and on that basis, an aggregate list was previously constructed (Taconis, 2021b; Taconis and Hobbelen, 2023). Table 1 gives an overview.
These characteristics apply to all CBL versions, but the exact mix and accents may differ from case to case and from situation to situation. For example, differences exist in terms of emphasizing the importance of “multiple stakeholder perspectives” (Kohn Rådberg et al., 2020), the use of ill-defined problems University Twente, 2022, the need to focus on sociotechnical issues (Loohuis and Bosch-Chapel, 2021), or the active communication of students with stakeholders (Taconis, 2021b; Taconis and Hobbelen, 2023). Table 1 generally typifies a broadly defined concept of CBL as used in various engineering universities worldwide (Graham, 2018; Mitra, 2018). As an aside, we note here that Table 1 includes only characteristics of learning and not of teaching (e.g., teacher roles/tasks, assessment) as one would expect in a teaching model.
From a classification perspective, the situation is, however, still confusing as these listed characteristics partly overlap with those used for defining other educational concepts such as problem-based learning (PBL) (Hung et al., 2008) and Design-Based Learning (e.g., Kolodner, 2002; Scheltenaar et al., 2015; Bekker et al., 2018). Moreover, some cases of PBL or DBL would also qualify as CBL—at least according to the characteristics in Table 1.
Comparison of features shows some gradual differences. First, CBL prioritizes real-world challenges that model professional practices and/or are socially relevant. This seems to differ from, for example, PBL, which usually focuses only on professional practices without emphasizing a societal connection. Second, and related to the first, CBL emphasizes that challenges must be “live problems”. These typically are complex, cross boundaries between different academic disciplines, include various communicative, ethical, and “soft” aspects, and often are ill-defined or “wicked problems”. Hence, CBL emphasizes multi- or trans-disciplinarity of teamwork, while most CBL-like educational concepts mentioned above focus on mono-disciplinary problems. Third, CBL is not limited to one particular type of problem, which differs from DBL, which deals specifically with “design problems”. Fourth, CBL emphasizes more strongly than DBL and PBL, the active communication of students with stakeholders and/or problem owners.
On the contrary, it must be said that defining CBL by enumeration implies that differences between CBL and other educational concepts may manifest themselves only in the form of specific characteristics that are emphasized more (or less) in CBL than in other educational concepts. The method of “definition by enumeration” suggests that the differences are “gradual” and may obscure possible underlying more fundamental differences.
A more fundamental definition of CBL
From a theoretical point of view, the above approach to defining CBL by listing characteristics is not very satisfying. On the one hand, enumerative may give the impression that the differences and similarities of CBL and, for example, PBL and DBL are merely a matter of more or less emphasis on one or more aspects. It may be tempting to interpret an overlap of concrete characteristics as an indication of fundamental similarity, but this seems incorrect. For example, when comparing whales to fish, there are several observable similarities, but underneath are fundamental differences. In fact, by listing concrete features, a definition cannot reveal similarities or differences at a more fundamental level. Hence, we need a more fundamental way of defining CBL based on an underlying purpose, mechanism, or principle (Goguen, 2005). From such a guiding principle, the various characteristics of CBL would emerge logically: not as stand-alone features but as interconnected building blocks that collectively realize the underlying principle in practice.
Such a fundamental definition may show, for example, that some characteristics are closer to the core and realization of the underlying principle than others, or it may indicate that specific features necessary on theoretical grounds are missing or underexposed in existing enumerative definitions. In short, a more fundamental definition would contribute to a better understanding of CBL as a teaching concept and provide guidelines for making design choices when designing CBL lessons, for example, by emphasizing the essential features at the expense of the more ancillary ones.
Looking for such a definition, we find “challenge”, the concept from which CBL takes its name, not only an obvious but also a good starting point. The term challenge can be defined as a task a person undertakes that is relatively difficult and typically recognized as valuable within a particular group. Sometimes, challenges can involve a struggle with “adversary forces”, and they may also require personal excellence, adding to the value of success. Therefore, when undertaken, challenges can mobilize energy, commitment, and dedication and—depending on the context and circumstances—are usually rewarded with a combination of achievement valued within the community and social recognition (Cambridge Online Dictionary, 2021).
In education, a challenge invites and encourages students to participate more and more extensively and productively. It can provide a mechanism that encourages the transition from peripheral to full participation (Lave and Wenger, 1991). When a challenge relates to “societal challenges”, it prompts awareness, learning about, developing a personal perspective, and connection to possible solutions at the level of citizenship and personal development and integration. When it (also) relates to real problems of STEM (professional) practice, it implies STEM learning, personal development, and integration within the person.
Various authors connect the concept of challenge to the concept of play as “an activity that is voluntary, intrinsically motivated, fun, incorporates free will/choices, offers escape, and is fundamentally exciting” (Sutton-Smith, 1997). Play is an authentic and essential driver in learning and human development. While play for young children is mainly connected to “fun”, this gradually gives way to an orientation toward challenge-oriented play during middle school age (Tisza, 2023). In line with this and for the context of inquiry-based learning, Ireland et al. (2014) suggest mainly focusing on STEM experiences for young kids (<K4). For older children, they recommend a mix of open experiences/explorations (without specific learning objectives) and “cookbook investigations” (K4–K7), gradually incorporating more and more “voids” that students can fill in themselves (K6–K8). They suggest using open-ended challenges only from K7 onward, where students can create and track their own plans (Ireland et al., 2014).
Although challenges can be potentially frustrating when over-demanding students, engaging in the challenges can result in a highly pleasurable altered state that Csikszentmihalyi labeled “flow” (Boyan and Sherry, 2011) and is associated with high intrinsic motivation (Deci and Ryan, 2008).
For example, Tulloch argues that many computer games employing challenges actually harvest on “being challenged” as a pedagogical mechanism (Tulloch, 2014). This pedagogic mechanism is a critical driving force that functions to signify, reward, and correct play practice (Tulloch, 2014, p. 325). On the one hand, it is about mastering the game and getting reward/recognition. On the other hand, as observed in role-play simulation games, it is about identification. Players typically play a role that is more mature than their current state (e.g., king and caretaker). The challenge of playing a more adult role thus seems to be an intrinsic part of the experience, corresponding to an implicit desire to explore adulthood, in this case, through challenges in play. Within the domain of STEM, students can discover and playfully explore “possible selves” connected to STEM (professional) roles, when allowed to experiment in an open and realistic environment (e.g., Schlegel et al., 2019). It is clear from the existing definitions that CBL seeks to transcend the level of education focused solely on STEM knowledge and skills, as well as contribute to the development of learners in general and to society and STEM practices. Therefore, it is good to also look at this from a fundamental perspective, for example, to consider what educational outcomes CBL could or should then focus on. Biesta (2015) argues that in modern technologically democratic societies, learning and education in a broad sense encompass three necessary components (Figure 1). The first is qualification, which refers to learning for citizenship and for a profession. It includes the acquisition of general and specific knowledge and skills. Some also link this to the acquisition of domain-specific values and a repertoire of acceptable and effective behaviors. For STEM, this includes the “STEM literacy” needed to understand and participate in our technological society and, if one chooses, to learn for a STEM profession. The second, socialization, is about becoming a functioning and participating member of society and relevant communities, e.g., professionally. Socialization clearly relies on developing communication skills and connecting to others. For engineers, it includes building competence to participate in professional communities and to operate as a professional, within the larger process of societal socialization. In both qualification and socialization, learning is primarily aimed at acquiring knowledge, beliefs, values and ways of doing things in society, or (professional communities) as they are today. The last component, subjectification, places the learner (subject) in a central position and goes in exactly the opposite direction by emphasizing his unique qualities, perspectives, and contributions.
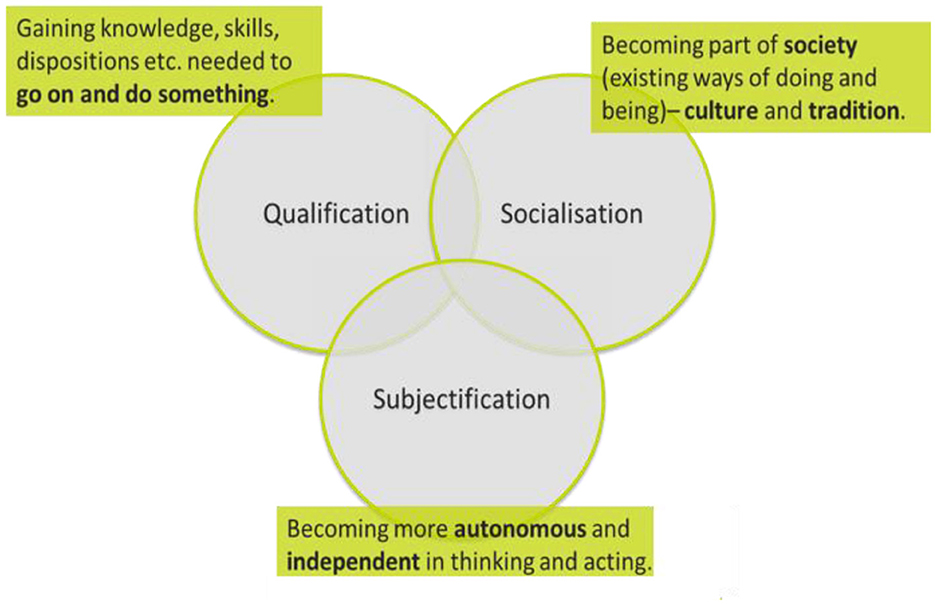
Figure 1. Biesta's (2015) three components of the pedagogical process.
Biesta (2009) describes it as the process of personal development and maturation in which students become independent and self-directed individuals who make decisions and act in a personally anchored, responsible and socially connected way. Subjectification fosters the ability to take informed and personally anchored independent position on issues. It requires qualification (e.g., knowledge) and socialization (e.g., skills) but transcends these. The process of subjectification requires the space to experience, experiment, explore, make decisions, experience consequences, and take responsibility. It can be helped by (encouraging) reflection and critical thinking.
For STEM, this includes critical thinking about existing technology solutions and their personal and societal implications, not as a “school skill” encapsulated in the processes of qualification and socialization, but as a way to articulate and complete oneself as an independent individual (Hasslöf and Malmberg, 2015). The “risk” that students may develop a “rebellious” voice should be gladly taken in democratic societies, as this rebelliousness creates openness and innovation (Biesta, 2015).
As a whole, Biesta's pedagogical framework comes close to identity development, mainly through the element of subjectification. It is hard to see how identity development, i.e., becoming an independent and autonomous individual, could take place without subjectification, especially concerning personal growth and developing agency. Subjectification operates on a general level of identity development, while STEM identity development would take place as more specific development embedded within that.
Subjectification is a comprehensive process associated with the development of one's self-identity. As such, it provides an embedding for the development of STEM identities as a more specific process within it.
Biesta argues that education always affects the subjectification of learners, but that it must be actively addressed, especially in our current and complex society (Biesta, 2015). First, critical and independent thinking and agency are needed to advance society. Second, subjectification allows students to become independent, agentic, and responsible citizens and professionals.
At the STEM level, Godwin et al. (2016) underscore that STEM education should strive to produce students who can see themselves as “powerful thinkers and doers in STEM who can contribute to society”. STEM education should empower students to participate in debates and decision-making in society, as well as in their possible future STEM professions. In his words, they must acquire “critical engineering agency (CEA)” (Godwin et al., 2016). Finally, Godwin argues that the development of a CEA is related to the choice of STEM careers and contributes to the empowerment of underrepresented minority students in particular (Godwin et al., 2016). CBL's emphasis on realistic problems, its connection to “the big societal challenges”, and its emphasis on direct contact of students with stakeholders align very well with these arguments.
Wrapping up, the challenge in CBL is not “just another learning task” but a compelling invitation to take on a role/activity that realistically puts the learner in a position that (ultimately) points toward maturity. In this, “challenging students” is an energizing pedagogical mechanism, so we propose it as the central principle underlying a new definition of CBL and its characteristics.
Theoretical implications of the new definition of CBL
The definition of CBL, provided above, as an “(open) assignment which challenges students to take on a task that reflects a more mature STEM-related role in society” has some implications.
CBL is defined on the level of both a (cognitive) task and situated activities
Being challenged refers to an activity, an effort, providing evidence of competence and getting recognized for that. Hence, challenges are performed within and for a community and involve interacting with several others, e.g., collaborators, stakeholders, beneficiaries, and others. The challenges in CBL typically include taking up (parts of) a professional role and enacting STEM practices in as real a situation as possible. Even though the existing enumerative definitions of CBL may be over-focusing the “enacting” on “teamwork” and “stakeholder contact”, for example, excluding the participation in a scientific community, CBL is more than just solving realistic “paper and pencil” problem or assignment. In terms of Gilbert (2006), who identifies four types of “realistic STEM education”, CBL goes beyond “narrative embedding” of STEM assignments by “tailoring the assignment to an authentic situation and packaging it in an authentic story” (type 3). CBL is an example of “situated activities” in which learners perform authentic roles and activities in a realistic situation (type 4). Hence, CBL is both a cognitive and a social game (Bekker, 2022).
CBL is intrinsically orientated toward recognition of stem achievements
By their nature, challenges involve tasks that are valuable to the community, and successes typically are socially rewarded. Thus, the concept of CBL has an intrinsic orientation to tasks/activities likely to produce recognition for successful students and their STEM achievements. This characteristic of challenges being “valuable” to communities and/or society seems downplayed in the existing enumerative definitions of CBL.
Part of the concept of challenge is that it is valued and recognized in the community.
CBL activities can evolve around any stem “core method”
CBL's underpinning by the underlying pedagogical principle of “being challenged” conceptually demarks CBL from concepts such as PBL and DBL as the latter two are defined by a specific activity, “problem-solving”, or “designing”, respectively. Since the basis of CBL is that students “are challenged” to perform (more) mature tasks/roles, the concrete task/activity can be any activity or activities, as long as the total reflects the core activities of the STEM domain(s) involved.
So, CBL could be interdisciplinary, as long as it (a) adequately mobilizes the pedagogical vehicle of “challenging” and (b) centers on (educational derivatives of) “core professional activities” from the relevant STEM domains. As long as it focuses on a realistic problem from STEM practice and connects to “big societal issues”, it could be mono-disciplinary and center on one or more of the “engineering methods” of that field (Taconis, 2021b; Taconis and Hobbelen, 2023). For physics, for example, this could be “problem-solving”, and CBL would resemble PBL but with a clear emphasis on “challenging” and “stakeholder involvement”. While for “industrial design”, it would seem to be DBL. For mathematics, CBL could center on “delivering proof” (Dahl, 2018; Pepin and Kock, 2020). Hence, CBL may be elaborated using various challenge types, but these need to be authentic for (key) practices of the domain or domains involved. These “modalities” of CBL would resemble, but not completely overlap with, concepts such as PBL or DBL (Figure 2).
CBL is authentic in three ways
Authentic learning can be understood in several ways. First, learning tasks can be authentic in the sense that they revolve around real problems or challenges with which learners engage (Bransford et al., 2004). In addition, authenticity can relate to learners' activities. These can take place in an authentic setting (physically authentic) and/or follow (or mimic) the activities of practitioners (Saeli et al., 2020). Finally, authentic learning can be understood as learning that connects to learners' natural developmental tendencies and ways of learning. For example, younger children learn through play and discovery.
CBL is authentic with respect to both the tasks and student activities, and it provides students with an opportunity to enact STEM and discover and explore “possible selves” in relation to STEM (professional) roles (e.g., Schlegel et al., 2019). It is also authentic in the third way because it is close to students' natural way of learning and development when they are actually challenged.
A prioritization of CBL aspects
The new definition highlights some aspects of Table 1 as crucial to achieving the underlying pedagogical goals of qualification, socialization, and subjectification. CBL challenges should always be challenging, attractive, meaningful, authentic, engaging, and multi-perspective. Other aspects now seem of secondary value, such as challenges being completely open-ended, ill-defined, or multidisciplinary. From our perspective on CBL, these aspects can be omitted or minimized if there are good reasons to do so, such as adapting to the students' age, developmental level, or interest.
On the contrary, the existing defining lists seem to fall short: first, with regard to emphasizing students' central need to be “challenged”, and second, concerning the pedagogical function of subjectification. Some elements in Table 1, such as reflection, self-direction, and welcoming contributions from different perspectives, contribute to this. However, other factors such as critical thinking, space (and encouragement) for (creative) exploration, and the availability of role models are missing in Table 1. In addition, the need for decision-making and the urge to build personal and informed views (commitment) are left implicit.
STEM identities and CBL
Generally, there are several definitions of identity, all concerning three common aspects. The first is a “mediating” character of the concept of identity. Identities typically “connect situated persons to situated behavior” (Schachter, 2005) and are “negotiated agreements” tying together one's self-view and how the outside world views a person. The second is that identities are a coherent combination of values, views, beliefs, and “ways of understanding” that underlay (preferred) behavior (Taconis, 2021a). Third, identities are “developmental”. Gradually developing over time, for example, as one slowly changes views, behavior, finds new (social) contexts and changes environments.
This nature of a “dynamic interface” is also reflected in the characterization of identity as an “agency|structure” dialectic (King, 2016). Dialectic indicates a continuous interplay between the “structure” (the total of the individual's surroundings comprising other interacting individuals, rules, institutions, physical and technological givens, etc.) and the acting individual, who is interacting with it, being influenced by it, and being a factor contributing to constituting the structure. Examples are being reflective and critical and possibly making deliberate attempts to change both the own behavior, self-definition, and surrounding structure. Identity development evolves by various mechanisms: verification, exploration, commitment, internal alignment, group processes, experiences of success or failure, reflection, etc. (Stets, 2020).
Overall, identities can be classified into more or less separate types, such as self-identities, role identities, and social identities (Burke and Stets, 2009). STEM identities are role identities derived from what STEM professionals do (Taconis, 2021a). They are derived from professional roles directly (higher education) or indirectly through the educational system (secondary education).
An example is playing the role of a scientist in role-playing games or, on a more basic level, symbolically wearing a white coat during innocent experiments. As role identities, their development principally emerges from “doing STEM” and being recognized for these achievements. Since the distinction between role identities and social identities is not absolute, social mechanisms can also contribute (Burke and Stets, 2009). Examples are identification with role models or being confronted with stereotyped images of STEM for example, which can reinforce or work against the construction of positive STEM identities. Ultimately, however, STEM achievements play a pivoting role in STEM identities and their formation (Bamberg, 2011).
However, STEM achievement is not the only factor in developing a STEM identity. Other factors include intrinsic STEM interest, student-perceived STEM competence (or confidence in STEM), and recognition of STEM achievement. Moreover, STEM achievements are a necessary—but unfortunately not always sufficient—condition for being recognized in, and thus acquiring, a STEM identity (Knez, 2016). Strong relationships are found between positive STEM identities, STEM achievement, and (feelings of) competence in STEM (e.g., Dou and Cian, 2022).
STEM identity research
A first branch of research involves efforts to stimulate interest in STEM and interest in STEM careers. It was found that developing positive STEM identities correlates highly with pursuing STEM careers and performance in STEM education (Taconis and Kessels, 2009; Hazari et al., 2010). The STEM identity framework principally applies to all students but is particularly relevant for promoting the participation in STEM of underrepresented minority (URM) students (Carlone and Johnson, 2007). These students, in particular, may struggle with a mismatch between their personal and/or cultural identities and the identity STEM or STEM subjects education may “require” (e.g., Taconis and Kessels, 2009; Holmegaard et al., 2014).
In addition, particularly these students may not always be recognized for their STEM achievements (Avraamidou, 2022). Unfortunately, STEM performance is not always automatically recognized (Carlone and Johnson, 2007). One reason could be that one may be reluctant to appropriate one's STEM successes, for example, students from a background where STEM and STEM careers are considered “not for us”. In addition, teachers may overlook or misinterpret exhibited STEM competence (Dee and Gershenson, 2017), in particular for students with cultural and/or ethnic minority backgrounds. Effects become more robust within the cross-section of minority characteristics (e.g., Afro-American women). Being deprived of fair recognition for achievements can have a cumulative negative effect on STEM development (Cohen et al., 2009).
A second area of STEM identity research focuses on secondary, vocational, or higher education students who are considering or in the process of “becoming” STEM professionals. In addition to the aspects mentioned above of interest, achievement, self-efficacy, and recognition, the content of STEM identities plays a prominent role here (Taconis, 2021a). The focus is on what kind of professional one becomes: “what professional skills one acquires and prefers,” “learning to communicate and behave professionally adequately,” and “developing personal yet professional values”. Learning concerns professional skills, the individual's critical confrontation with professional values, developing personal views, internalizing professional norms, and role adaptation (Johnson and Ulseth, 2016). On this level, STEM identities and their development cannot be understood separated from the development of professional competence (Schellings et al., 2018).
Aspects and elements of STEM identities
According to Carlone and Johnson (2007) a person with a STEM identity is “a person that sees him/herself and is seen by others as a STEM person”. This widely used definition directly reflects how identities dialectically mediate between individuals to their context. Contexts can be an informal learning situation, STEM classes in school or professional life.
Researchers found that STEM identities involve three interconnected components: (1) competence (understanding the subject as defined in a particular context), (2) performance (being able to perform the tasks and activities as defined in that context, both cognitively and socially), and (3) recognition (of STEM achievements or as a STEM person, by oneself and by others involved in that context).
Others have proposed additional components, such as interest and competence beliefs (Carlone and Johnson, 2007; Wang and Hazari, 2018). Within interest, a general aspect (e.g., recognizing STEM as interesting and generally relevant for society) and a career-related interest could be distinguished (e.g., having an appetite for a STEM career) (Fraser, 1981). The latter is particularly relevant for promoting STEM careers and preluding STEM professional identities. Chen and Wei (2022) proposed four factors within “science identity”: science learning competence, science (classroom) interest and performance, science career interest, and science recognition. Various studies have shown how performance, competence, STEM interest, and recognition contribute to STEM identity (e.g., Dou and Cian, 2022).
Researchers in the field of STEM professional development have further elaborated on the aspects of STEM career interest. For example, the Professional Identity Five-Factor Scale (PIFFS) questionnaire (Tan et al., 2016) comprises five subscales: (1) knowledge about professional practices, (2) having the professional as a role model, (3) experience with the profession, (4) preference for a particular profession, and (5) professional self-efficacy. Especially in the context of vocational or advanced STEM education (professional), STEM identities include metacognition (e.g., self-regulation and self-management skills) and professional skills (Johnson and Ulseth, 2016).
Figure 3 shows the elements and aspects of STEM identities considered in this study. Moreover, STEM identities require these elements to be interconnected and personally integrated, functioning as an active interface between the person and his/her environment.
Empirical indications for CBL'S contribution to STEM identities
Since CBL is such a new concept (Graham, 2018), there are currently too few studies completed on CBL practices to conduct a review study. Hence, this study takes a conceptual approach and focuses on how CBL can contribute to developing STEM identities at the conceptual level. Nevertheless, there is a way to support this conceptual study empirically, by looking at empirical studies on CBL-like education models that share some basic characteristics with CBL.
This empirical material provides statistical evidence for relationships of the characteristics shared with CBL on the one hand and the development of STEM identities and their components on the other hand. If such a relationship can be interpreted using established educational theories as a causal effect, it can be assumed that it also operates in the context of CBL education.
This approach yields a “low-resolution picture” only. First, due to the uncertainties, the extrapolation procedure introduces. Second, because our view of the learning effects of PBL, DBL, etc., is statistically blurred by the differences between these concepts, the variability within these concepts, and the considerable heterogeneity in implementations, educational contexts, and research designs used (Walker and Leary, 2009). Finally, this approach can only point toward the expected learning outcomes of CBL insofar as they derive from characteristics that CBL shares with these well-studied concepts, e.g., PBL, DBL, etc. For example, it cannot shed light on the specific contribution challenging learners can make to developing a “STEM identity”.
Much of this study builds on a previous study that followed this strategy by Taconis (2021b) and Taconis and Hobbelen (2023). It comprised over 250 empirical studies and the primary literature reviews available (e.g., Barrows, 1986; Roselli and Brophy, 2006; Hung et al., 2008; Strobel and van Barneveld, 2009; Lin and Chen, 2017; Altan et al., 2018). Approximately 10% of the studies focused on CBL, 40% on PBL, and 20% on DBL. 18% were concerned with other CBL-like forms of education, such as inquiry or discovery learning, context-based education, trans- or multidisciplinary engineering education, or addressing the learning of “student teams” or in “innovation spaces”. The vast majority focused on or included STEM or at least one STEM subject. The studies concerned a variety of educational contexts and employed various approaches and methodologies.
The abovementioned study focused on cognitive, metacognitive, social, communicative, and affective learning outcomes of CBL-like STEM education. Additional resources were used to explore other STEM-identity-related outcomes, such as STEM motivation (STEM confidence), achieved recognition, and students informed view of STEM. Together, these more or less cover the aspects of STEM identities listed in Figure 3.
STEM performance: cognitive outcomes
Cognitive outcomes are related to STEM performance and impact students' views on STEM in particular. They comprise knowledge and skills. Taconis (2021b) employ a distinction between three levels of knowledge and skills. It distinguishes between practical skills (domain-related but requiring little domain-specific knowledge), operational skills (practical but dependent on specific domain knowledge), operational knowledge (domain-related and defined on the basis of observable entities), and theoretical knowledge and skills (comprising concepts that are theoretically defined by their mutual formal or mathematical relationships, and not directly depending on observables). Theoretical knowledge constitutes a largely abstract and formalized structure of interrelated concepts that are external to operational reality but that “models” reality such that theoretical thinking can produce useful and viable predictions and guidelines.
Using this distinction into three levels, it appears that a strong point of CBL-like education lies in acquiring and integrating knowledge and skills on the practical and operational levels. The acquisition of practical and operational knowledge was concluded to be slightly superior on average in comparison with “classical education” (Gijbels et al., 2005; Hung et al., 2008; Albanese and Dast, 2014). Strobel and van Barneveld claim PBL is superior to “traditional instruction” with respect to training competent and skilled practitioners but leads to slightly inferior results on “standardized tests” which treat knowledge in a decontextualized way (Strobel and van Barneveld, 2009).
Regarding the more theoretical levels in, CBL-like instruction was found to be less effective for abstract domain knowledge acquisition and for theoretical learning in particular (Albanese, 2000; Perrenet et al., 2000; Hung et al., 2008). Even further to the theoretical side of the spectrum, regarding the type of complex formal hierarchically organized knowledge that typifies STEM domains (at the higher levels), almost no studies were found that confirmed (and/or measured) the acquisition of this type of knowledge in CBL-like learning.
The studies that explicitly reported on deep theoretical learning showed that learning outcomes were low and/or less than in classical education. One study found that only those concepts were acquired that played an absolutely essential role in completing the “challenge” (e.g., van Breukelen et al., 2017). Although the evidence is incomplete and partly “circumstantial”, all of these strongly suggest that CBL is generally less effective for theoretical learning—and increasingly less effective the further away the learning is from practical or operational knowledge.
Taconis et al. provide conceptual reasoning that could explain this from the defining characteristics of CBL-like education. First, CBL-like learning projects include, by their nature, two partially overlapping and partially competing processes of “completing the project” and “learning”. Savin-Baden (2004) shows how task completion deadlines make students prioritize “passing” over deep learning. Second, CBL-like learning concepts—at least in their standard form—do not explicitly emphasize “deep learning activities” that are widely seen as necessary for building a deep, connected, and coherent knowledge base (Koopman et al., 2011). Third, CBL-like learning constitutes a case of “complex learning”, which is known to quickly overload learners due to high cognitive loads resulting from navigating unfamiliar terrain, project management, and collaboration issues, which impedes or hinders deep learning (Kirschner et al., 2006). Finally, assessment procedures do not focus on knowledge and deep understanding (Savin-Baden, 2004).
STEM performance: higher order skills
Higher order skills such as collaboration and self-directed learning are essential to professional STEM identities in particular. Taconis (2021b) concluded that there is no conclusive empirical evidence for acquiring these skills in CBL-like education. If they were successfully taught, it was in cases where they received extra attention in separate courses or workshops. In addition, Johnson and Ulseth (2016) describe how CBL-like sessions functioned primarily as an arena to practice such skills rather than as the point of acquisition. It, therefore, seems plausible that these skills will only be acquired in a structured way when given dedicated attention within the CBL-like education and/or by supplementary training to students (Taconis, 2021b; Taconis and Hobbelen, 2023).
STEM competence: confidence in doing STEM
Another common effect of CBL-like teaching is that students become more confident in STEM (e.g., Chen et al., 2015; Tan et al., 2016; Talafian et al., 2019; Fidai et al., 2020). All of the studies we have included address this issue reporting this. Some of them—but not all—target URM students, and the effect is clearly not limited to this group. Building confidence in doing STEM is recognized as one of the most important factors in programs that effectively stimulate interest in STEM (van den Hurk et al., 2019).
Building STEM confidence seems to be related to a safe and open working atmosphere. Classroom climate is essential in raising interest in STEM and STEM careers (van den Hurk et al., 2019; Starr et al., 2020). Such an environment is, for example, typified as a “welcoming and inclusive atmosphere” (Chen et al., 2008). Its crucial role is most clearly demonstrated in studies on URM students. These students follow dedicated programs where URM students are amongst each other. Their need for a very safe, inclusive, and welcoming atmosphere is expressed more explicitly as “(unbiased) appreciation of performance” (Dee and Gershenson, 2017; Mulvey et al., 2022) or “physically and psychologically safe” (Hughes et al., 2021).
A safe and open working atmosphere is crucial, regardless of whether the teaching is of the CBL type. CBL-type education does not guarantee this. On the contrary, CBL-like teaching can help to create a starting point for fostering a welcoming and inclusive atmosphere. Using multi-perspective problems, for example, will help students from different backgrounds to make valuable contributions. Group work can also be a good starting point but only if groups maintain an open, safe, and rewarding atmosphere. For teachers, monitoring, promoting, and safeguarding are an additional responsibility in CBL-like teaching contexts (Ping et al., 2021). Teachers should avoid unfavorable student group compositions such as groups with URM students being “token members” or student groups with “fault lines” regarding gender, background, language, and beliefs (Ping et al., 2021).
STEM recognition
The third category from Figure 3 is recognition. Studies on CBL-like education often indicate recognition as contributing to STEM identity formation considerably (e.g., Hughes et al., 2021; Dou and Cian, 2022) and STEM career interest (Starr et al., 2020). Low recognition may lead to negative self-appraisal, having a strong negative impact, e.g., on STEM performance (Cohen et al., 2009).
It should be clear that CBL-like teaching as such cannot create recognition that ultimately happens in the classroom. Nevertheless, CBL-like education creates an environment making it easy to deploy for the fair and equal recognition of STEM achievements, e.g., by peers. However, STEM recognition can also come from teachers, teaching assistants, or parents (Starr et al., 2020). “Fair recognition” can be seen as a passive “factor” or creating it can be made into a deliberate educational strategy (e.g., Hughes et al., 2021). As a strategy, it can help positively change students' sense of recognition, facilitating the development of STEM identities (Wang and Hazari, 2018; Brown and Livstrom, 2020), especially for URM students.
STEM interest and motivation
Various studies report that CBL-like tasks had an overall positive and motivating effect on students (Doppelt et al., 2008; Leary et al., 2019; Taconis, 2021b; Taconis and Hobbelen, 2023). Increasing motivation and engagement, and promoting positive attitudes toward STEM are the most salient learning outcomes and strengths of CBL-style teaching (Doppelt et al., 2008).
This effect was powerful for all students and especially for URM students. The most commonly indicated factors contributing to this are the “open nature of the tasks” (e.g., Betz et al., 2021), and the multi-perceptivity of the tasks (welcoming a broad spectrum of contributions) (e.g., Chen et al., 2015). Being granted “some level of freedom in choosing” usually increases motivation in theories on “playful learning” (Bekker et al., 2014).
Some studies also state that the meaningfulness of the “challenges” contributes to motivation. However, meaning does not always come from a connection to professional practice or large-scale social issues. It often comes from the link of the “challenge” with issues that play a role in a local community (e.g., King, 2016; Taconis et al., 2018; Huang et al., 2022). Other aspects listed in Table 1, including those specific to CBL (e.g., active involvement of stakeholders), do not seem particularly relevant as factors contributing to increased motivation for STEM.
STEM career interest
Concerning the last category in Figure 3, various studies report that CBL-like education helps increase interest in STEM careers (Taconis, 2021b; Taconis and Hobbelen, 2023). Beier et al. showed that participating in a CBL-like course in higher education led to higher career aspiration in STEM, greater confidence in STEM skills, and higher ratings of the utility of STEM courses (Beier et al., 2019).
An increase in career interest can have several causes. First is the experience of being competent in STEM during CBL-like education, which boosts “confidence in STEM abilities” both in secondary education and with engineers in training (Chen et al., 2015). LaForce et al. found that CBL-like interventions boosted students' STEM attitudes and STEM career interest through both a direct statistical effect and indirect effects mediated by “intrinsic interest in STEM” and “STEM ability beliefs” (LaForce et al., 2017). Second, CBL-like education can lead to a better view of STEM and STEM career opportunities (Taconis, 2021b; Taconis and Hobbelen, 2023). CBL-like education is experienced as a pleasant and experiential introduction to STEM and STEM (professional) practice (Johnson and Ulseth, 2016). It offers an excellent opportunity for getting a realistic (pre)view of STEM and STEM careers. It may also constitute an environment where students can explore and experience “possible selves” in relation to STEM (professional) roles (e.g., Schlegel et al., 2019).
Impact on shaping coherent and agentive STEM identities
In addition to the various individual components in Figure 3, building a STEM identity requires that the various components come together in a coherent pattern that can guide interpretations, decision-making, and further development. This seems especially important at the higher levels of STEM education, where the content of STEM identities and self-direction is increasingly important. Studies typically report that CBL-like education contributed to acquiring several components of STEM professional identities. For example, Johnson and Ulseth (2016) found that students participating in PBL improved several professional skills but not self-directed competence. Tan et al. (2016) found that PBL education, in which practitioners were actively involved in strengthening PBL's connection with professional practice still further, had contributed to the promotion of four of the five professional STEM identity components they pursued. One of the acquired components was “professional self-efficacy”. However, none of these studies could confirm that students develop a STEM identity in the sense of seeing themselves more as “STEM professionals” than before education. Tan et al. conclude that “to promote professional identity development comprehensively, PBL must include [the actual] experience of the profession” (Tan et al., 2016, p. 54).
Discussion and conclusion
This study aimed to paint a picture of the impact that challenge-based learning could have on various elements contributing to STEM identity formation. We conducted a conceptual analysis, first proposing a more informed theoretical definition of CBL and then looking for empirical evidence pointing toward the impact of core features of CBL on the development of STEM identities.
An obvious limitation of this article is that there is currently too little empirical material for a fully empirical review, and we could only conduct a conceptual analysis. Therefore, our conclusions about the effect of the defining characteristics of CBL on the development of STEM identities are “theoretical expectations” as they rely in part on the use of general educational theories extrapolated from research on, for example, PBL or DBL.
Moreover, the concept of CBL is still young and evolving, so there is still room to sharpen it conceptually, which also points to the desirability of conceptual analysis. We choose to approach CBL as a pedagogical concept that leads to the recognition that it should contribute to three aspects of student learning: qualification, socialization, and subjectification. One could have taken other directions, but we hope that our approach contributes to further developing the concept of CBL. A first conclusion is that our definition of CBL supports a more nuanced view of how variations of CBL might be implemented and how it differs from, e.g., PBL and CBL, and also that existing enumerative definitions of CBL place little emphasis on CBL's unique and demarking characteristics, such as challenging students and providing them with an arena to enact STEM realistically and explore possible selves in connection to STEM.
Second, our pedagogical exploration of CBL reveals some shortcomings in the concept of CBL, particularly in terms of a lack of emphasis on critical thinking and creativity. These are essential skills/attitudes related to the pedagogical goal of subjectification at the societal level and for building the kind of “critical citizenship” society needs, especially given the significant environmental and other societal challenges associated with STEM. Enumerative CBL definitions also lack attention to identity exploration.
A third conclusion is that, based on its defining characteristics, CBL can be expected to provide a strongly motivating and inspiring context for STEM identity development, by being authentic, but especially by being open (letting students choose or come up with their own projects), by using multi-perspective challenges (for which contributions from multiple perspectives can be honored), and by connecting students with each other and with stakeholders.
Together, these three factors imply that students in CBL have a relatively high degree of autonomy (e.g., open tasks, activities that honor a broad spectrum of STEM contributions) and experience connectedness (e.g., group atmosphere, group work, and working in groups composed entirely of students from underrepresented minorities). In addition, students are also likely to perceive that they are competent in STEM (e.g., the various ways one can contribute to the task, the possibility of mutual support, and help from teachers). According to self-determination theory (Deci and Ryan, 2008), these factors would explain the high reported levels of motivation and increased confidence levels.
In addition to this motivational effect, we found three other factors that are expected to contribute to CBL's impact on STEM identity formation:
• The lesser emphasis of CBL puts on learning content, domain knowledge, or theory. This may contribute to strengthening student's sense of STEM competence—at least at the lower levels of STEM education. A positive perception of one's STEM competence per se is a strong factor supporting STEM identity.
• The positive conditions CBL creates for obtaining (fair) recognition for STEM achievements. In particular, the group work, the use of challenges that allow for multiple approaches, the less “monochromatic” emphasis on domain knowledge, and the connection to stakeholders whose position naturally implies their recognition of what will be achieved. It must be said, however, that fair recognition—as in all education—requires the active attention of teachers and students to actually happen in the classroom, even though the CBL provides a good starting point.
• CBL-like education is—at all educational levels—perceived as an enjoyable and experiential introduction to STEM and STEM (professional) practice. It provides n rich and authentic introduction to STEM and the STEM professional world.
It is also worth noting that although none of the above motivational effects is theoretically unique to CBL, and some are not even unique to CBL-like teaching, CBL and the like seem to be a very effective way of organizing motivational factors, context, and content in such a way that motivation and confidence soar.
The above probably applies to vocational and higher education as well as primary and secondary education. However, studies on higher and vocational education show that for this level, building STEM identities comprising specific (professional) elements seems much more complicated and dependent on several factors other than the above. Research typically indicates that CBL could contribute to acquiring some elements of STEM identities but struggles to promote others, such as “self-directedness” and “learners ownership” It is still unclear how and how effectively CBL could contribute to the acquisition of some of the other elements such as higher order competencies. Moreover, CBL seems to fall short in creating “integrated” professional identities, possibly because the “immersion” in professional reality by CBL may be too limited.
On the contrary, this may also partly be due to shortcomings in the concept of CBL as currently defined. Enumerative definitions focus on describing what CBL education should look like but are less explicit about the pedagogy for making that learning happen. Enumerative definitions of CBL also seem to have some gaps and/or yet unarticulated features. First, there is no mention of subjectification as a vital aspect of learning. In addition, some of the connected higher order thinking skills such as critical thinking and employing creativity lack. Some other higher order skills, however, are included in enumerative definitions (e.g., developing self-direction and self-responsibility). Concerning STEM identity development, CBL seems to provide a rich environment for identity exploration (e.g., of possible selves) but is not currently not part of its definition.
We believe that “challenging learners” could provide such a pedagogical mechanism and improve the concept coherence. Challenging students could also make an additional contribution to motivation and meaningfulness. First, the concept of “being challenged” is principally connected to “showing competence” and “getting recognition”. Second, teachers can use challenges as an agentic ingredient to foster self-directedness and foster ownership. All in all, we conclude that CBL (and related forms of education) can be expected to facilitate the construction of STEM identities but that its contribution to building professional STEM identities seems partial and limited. The main mechanisms would be a combination of a motivation boost, higher self-competency perception, and the enjoyable and experiential orientation to STEM that it provides. Emphasizing CBL's quality of “challenging students”, and emphasizing a (playful) exploration of possible selves and STEM identities, could further boost motivation and engagement.
Author contributions
All authors listed have made a substantial, direct, and intellectual contribution to the work and approved it for publication.
Conflict of interest
The authors declare that the research was conducted in the absence of any commercial or financial relationships that could be construed as a potential conflict of interest.
Publisher's note
All claims expressed in this article are solely those of the authors and do not necessarily represent those of their affiliated organizations, or those of the publisher, the editors and the reviewers. Any product that may be evaluated in this article, or claim that may be made by its manufacturer, is not guaranteed or endorsed by the publisher.
Footnotes
1. ^The acronym STEM is here used to indicate both the school subject of STEM (or S.T.E.M.) and the various underlying school subjects and disciplines such as science, the different science subjects, Technology education, Engineering education, and mathematics.
References
Albanese, M. A. (2000). Problem-based learning: why curricula are likely to show little effect on knowledge and clinical skills. Med. Educ. 34, 729–738. doi: 10.1046/j.1365-2923.2000.00753.x
Albanese, M. A., and Dast, L. (2014). Problem-based learning: outcomes evidence from the health professions. J. Excell. Coll. Teach. 25, 239–252.
Altan, E. B., Yamak, H., Kirikkaya, E. B., and Kavak, N. (2018). The use of design-based learning for STEM education and its effectiveness on decision making skills. Univ. J. Educ. Res. 6, 2888–2906. doi: 10.13189/ujer.2018.061224
Avraamidou, L. (2022). Identities in/out of physics and the politics of recognition. J. Res. Sci. Teach. 59, 58–94. doi: 10.1002/tea.21721
Bamberg, M. (2011). Who am I? Narration and its contribution to self and identity. Theory Psychol. 21, 3–24. doi: 10.1177/0959354309355852
Barrows, H. S. (1986). A taxonomy of problem-based learning methods. Med. Educ. 20, 481–486. doi: 10.1111/j.1365-2923.1986.tb01386.x
Beier, M. E., Kim, M. H., Saterbak, A., Leautaud, V., Bishnoi, S., and Gilberto, J. M. (2019). The effect of authentic project-based learning on attitudes and career aspirations in STEM. J. Res. Sci. Teach. 56, 3–23. doi: 10.1002/tea.21465
Bekker, T., De Valk, L., and Eggen, B. (2014). A toolkit for designing playful interactions: the four lenses of play. J. Ambient Intellig. Smart Environ. 6, 263–276. doi: 10.3233/AIS-140259
Bekker, T., Taconis, R., Bakker, S., and d'Anjou, B. (2018). “Developing an online authoring tool to support teachers in designing 21st century design based education in primary school,” in International Conference on Computer Supported Education CSEDU 2018: Computer Supported Education (Funchal, Madeira), 142–165.
Betz, A. R., King, B., Grauer, B., Montelone, B., Wiley, Z., and Thurston, L. (2021). Improving academic self-concept and stem identity through a research immersion: pathways to STEM summer program. Front. Educ. 6, e674817. doi: 10.3389/feduc.2021.674817
Biesta, G. (2009). Good education in an age of measurement: on the need to reconnect with the question of purpose in education. Educ. Assess. Eval. Account. 21, 33–46. doi: 10.1007/s11092-008-9064-9
Boyan, A., and Sherry, J. L. (2011). The challenge in creating games for education: aligning mental models with game models. Child Dev. Persp. 5, 82–87. doi: 10.1111/j.1750-8606.2011.00160.x
Bransford, J. D., Brown, A. L., and Cocking, R. R. (2004). How People Learn: Brain, Mind, Experience, and School: Expanded Edition. Washington, DC: National Academies Press.
Brown, J. C., and Livstrom, I. C. (2020). Secondary science teachers' pedagogical design capacities for multicultural curriculum design. J. Sci. Teach. Educ. 31, 821–840. doi: 10.1080/1046560X.2020.1756588
Cambridge Online Dictionary (2021). Challenge. In Cambridge Online Dictionary. Available online at: https://dictionary.cambridge.org/dictionary/english/challenge (accessed July, 9, 2021).
Carlone, H. B., and Johnson, A. (2007). Understanding the science experiences of successful women of color: science identity as an analytic lens. J. Res. Sci. Teach. 44, 1187–1218. doi: 10.1002/tea.20237
Chen, H. L., Lattuca, L. R., and Hamilton, E. R. (2008). Conceptualizing engagement: contributions of faculty to student engagement in engineering. J. Eng. Educ. 97, 339–353. doi: 10.1002/j.2168-9830.2008.tb00983.x
Chen, P., Hernandez, A., and Dong, J. (2015). Impact of collaborative project-based learning on self-efficacy of urban minority students in engineering. J. Urban Learn. Teach. Res. 11, 26–39.
Chen, S., and Wei, B. (2022). Development and validation of an instrument to measure high school students' science identity in science learning. Res. Sci. Educ. 52, 111–126. doi: 10.1007/s11165-020-09932-y
Cohen, G. L., Garcia, J., Purdie-Vaughns, V., Apfel, N., and Brzustoski, P. (2009). Recursive processes in self-affirmation: intervening to close the minority achievement gap. Science 324, 400–403. doi: 10.1126/science.1170769
Dahl, B. (2018). What is the problem in problem-based learning in higher education mathematics. Eur. J. Eng. Educ. 43, 112–125. doi: 10.1080/03043797.2017.1320354
Deci, E. L., and Ryan, R. M. (2008). Self-determination theory: a macrotheory of human motivation, development, and health. Can. Psychol. Psychol. Can. 49, 182. doi: 10.1037/a0012801
Dee, T., and Gershenson, S. (2017). Unconscious Bias in the Classroom: Evidence and Opportunities, 2017. Mountain View, CA: Stanford Center for Education Policy Analysis.
Doppelt, Y., Mehalik, M. M., Schunn, C. D., Silk, E., and Krysinski, D. (2008). Engagement and achievements: a case study of design-based learning in a science context. J. Technol. Educ. 19, 22–39.
Dou, R., and Cian, H. (2022). Constructing STEM identity: an expanded structural model for STEM identity research. J. Res. Sci. Teach. 59, 458–490. doi: 10.1002/tea.21734
Fidai, A., Barroso, L. R., Capraro, M. M., and Capraro, R. M. (2020). Effects of engineering design process on science and mathematics. 2020 IEEE Frontiers in Education Conference (FIE) 1–4. doi: 10.1109/FIE44824.2020.9274167
Fraser, B. J. (1981). Test of Science Related Attitudes (TOSRA). Melbourne, Vic: Australian Council for Educational Research.
Gallagher, S. E., and Savage, T. (2020). Challenge-based learning in higher education: an exploratory literature review. Teach. Higher Educ. 1–23. doi: 10.1080/13562517.2020.1863354
Gijbels, D., Dochy, F., Van den Bossche, P., and Segers, M. (2005). Effects of problem-based learning: a meta-analysis from the angle of assessment. Rev. Educ. Res. 75, 27–61. doi: 10.3102/00346543075001027
Gilbert, J. K. (2006). On the nature of “context” in chemical education. Int. J. Sci. Educ. 28, 957–976. doi: 10.1080/09500690600702470
Godwin, A., Potvin, G., Hazari, Z., and Lock, R. (2016). Identity, critical agency, and engineering: an affective model for predicting engineering as a career choice: identity, critical agency, and engineering careers. J. Eng. Educ. 105, 312–340. doi: 10.1002/jee.20118
Goguen, J. (2005). “What is a concept?” in Conceptual Structures: Common Semantics for Sharing Knowledge, eds F. Dau, M.-L. Mugnier, and G. Stumme (Berlin; Heidelberg: Springer), 52–77.
Graham, R. (2018). The Global State of the Art in Engineering Education. Cambridge, MA: Massachusetts Institute of Technology (MIT) Report.
Hasslöf, H., and Malmberg, C. (2015). Critical thinking as room for subjectification in education for sustainable development. Environ. Educ. Res. 21, 239–255. doi: 10.1080/13504622.2014.940854
Hazari, Z., Sonnert, G., Sadler, P. M., and Shanahan, M.-C. (2010). Connecting high school physics experiences, outcome expectations, physics identity, and physics career choice: a gender study. J. Res. Sci. Teach. 47, 978–1003. doi: 10.1002/tea.20363
Herrington, J., and Oliver, R. (2000). An instructional design framework for authentic learning environments. Educ. Technol. Res. Dev. 48, 23–48. doi: 10.1007/BF02319856
Holmegaard, H. T., Madsen, L. M., and Ulriksen, L. (2014). To choose or not to choose science: constructions of desirable identities among young people considering a STEM higher education programme. Int. J. Sci. Educ. 36, 186–215. doi: 10.1080/09500693.2012.749362
Huang, B., Jong, M. S.-Y., King, R. B., Chai, C.-S., and Jiang, M. Y.-C. (2022). Promoting secondary students' twenty-first century skills and STEM career interests through a crossover program of STEM and community service education. Front. Psychol. 13, 903252. doi: 10.3389/fpsyg.2022.903252
Hughes, R., Schellinger, J., and Roberts, K. (2021). The role of recognition in disciplinary identity for girls. J. Res. Sci. Teach. 58, 420–455. doi: 10.1002/tea.21665
Hung, W., Jonassen, D. H., and Liu, R. (2008). 38 problem-based learning. Handb. Res. Educ. Commun. Technol. 3, 485–506. doi: 10.7771/1541-5015.1604
Ireland, J., Watters, J. J., Lunn Brownlee, J., and Lupton, M. (2014). Approaches to inquiry teaching: elementary teacher's perspectives. Int. J. Sci. Educ. 36, 1733–1750. doi: 10.1080/09500693.2013.877618
Johnson, B., and Ulseth, R. (2016). Development of professional competency through professional identity formation in a PBL curriculum. 2016 IEEE Front. Educ. Conf. (FIE) 1–9. doi: 10.1109/FIE.2016.7757387
King, D. (2016). “Teaching and learning in context-based science classes,” in Teachers Creating Context-Based Learning Environments in Science, eds R. Taconis, P. J. den Brok, and A. Pilot (Rotterdam: SensePublishers), 71–85.
Kirschner, P. A., Sweller, J., and Clark, R. E. (2006). Why minimal guidance during instruction does not work: an analysis of the failure of constructivist, discovery, problem-based, experiential, and inquiry-based teaching. Educ. Psychol. 41, 75–86. doi: 10.1207/s15326985ep4102_1
Knez, I. (2016). Toward a model of work-related self: a narrative review. Front. Psychol. 7, e00331. doi: 10.3389/fpsyg.2016.00331
Kohn Rådberg, K., Lundqvist, U., Malmqvist, J., and Hagvall Svensson, O. (2020). From CDIO to challenge-based learning experiences – expanding student learning as well as societal impact? Eur. J. Eng. Educ. 45, 22–37. doi: 10.1080/03043797.2018.1441265
Kolodner, J. L. (2002). Learning by design™: iterations of design challenges for better learning of science skills. Cogn. Stud. Bull. Japan. Cogn. Sci. Soc. 9, 338–350. doi: 10.11225/jcss.9.338
Koopman, M., den Brok, P. J., Beijaard, D., and Teune, P. (2011). Learning processes of students in pre-vocational secondary education: relations between goal orientations, information processing strategies and development of conceptual knowledge. Learn. Indiv. Diff. 21, 426–431. doi: 10.1016/j.lindif.2011.01.004
LaForce, M., Noble, E., and Blackwell, C. (2017). Problem-based learning (PBL) and student interest in STEM careers: the roles of motivation and ability beliefs. Educ. Sci. 7, 4. doi: 10.3390/educsci7040092
Lave, J., and Wenger, E. (1991). Situated Learning. Legitimate Peripheral Participation. Cambridge, UK: Cambridge University Press.
Leary, H., Walker, A., Lefler, M., and Kuo, Y. -C. (2019). “Self-directed learning in problem-based learning,” in The Wiley Handbook of Problem-Based Learning, 1st Edn, eds M. Moallem, W. Hung, and N. Dabbagh (John Wiley and Sons, Ltd.), 181–198.
Lin, J., and Chen, C. (2017). “A study on the course types of challenge-based learning—Based on the relevant courses in Tsinghua University,” in 2017 7th World Engineering Education Forum (WEEF) (Kuala Lumpur), 166–172.
Loohuis, R., and Bosch-Chapel, L. (2021). Strategising with Challenge-Based Learning to Boost Student's Transferable Competence Development: A White Paper (Enschede), 10.
Mulvey, K. L. J., Mathews, C., Knox, J., Joy, A., and Cerda-Smith, J. (2022). The role of inclusion, discrimination, and belonging for adolescent Science, Technology, Engineering and Math engagement in and out of school. J. Res. Sci. Teach. 59, 1447–1464. doi: 10.1002/tea.21762
Nichols, M., and Cator, K. (2008). Challenge Based Learning: Take Action and Make a Difference. Challenge Based Learning: A White Paper. Apple co. Avaiable online: http://ali.apple.com/cbl/global/files/CBL_Paper.pdf.diakses (accessed July 17, 2016).
Pepin, B., and Kock, Z.-J. (2020). Challenge Based Education in/for Mathematics and Physics Education. Centre for Engineering Education. Available online at: https://www.4tu.nl/cee/innovation/project/13181/challenge-based-education-infor-mathematicsand-physics-education (accessed March 25, 2023).
Perrenet, J. C., Bouhuijs, P. A. J., and Smits, J. G. M. M. (2000). The suitability of problem-based learning for engineering education: theory and practice. Teach. High. Educ. 5, 345–358. doi: 10.1080/713699144
Ping, C., Kleingeld, P. A. M., Rispens, S., and Taconis, R. (2021). “Does nationality composition affect student groups' collaboration and performance? A cross-case analysis,” in Proceedings of the 49th Annual SEFI Conference: Blended Learning in Engineering Education: Challenging, Enlightening-and Lasting? (Berlin: Technische Universität Berlin), 1160–1170.
Roselli, R. J., and Brophy, S. P. (2006). Effectiveness of challenge-based instruction in biomechanics. J. Eng. Educ. 95, 311–324. doi: 10.1002/j.2168-9830.2006.tb00906.x
Saeli, M., Kock, Z.-J., Schüler-Meyer, A., and Pepin, B. (2020). “Towards mobile centered authentic, personalized and collaborative assignments in engineering education,” in SEFI 48th Annual Conference Engaging Engineering Education, Proceedings: Engaging Engineering Education (Enschede: Twente University), 1074–1082.
Savin-Baden, M. (2004). Understanding the impact of assessment on students in problem-based learning. Innov. Educ. Teach. Int. 41, 221–233. doi: 10.1080/1470329042000208729
Scardamalia, M., and Bereiter, C. (2007). “Fostering communities of learners and knowledge building: An interrupted dialogue,” in Children's Learning in the Laboratory and in the Classroom: Essays in Honor of Ann Brown, eds J. C. Campione, K. Metz, and A. Palincsar (Routledge), 197–212.
Schachter, E. P. (2005). Context and identity formation: a theoretical analysis and a case study. J. Adoles. Res. 20, 375–395. doi: 10.1177/0743558405275172
Schellings, G. L. M., Beijaard, D., Koopman, M., and Mommers, J. (2018). “Teacher's professional identity: Configurations of personal and contextual factors,” in Paper Presented at SIG Professional Learning and Development, September 11th-14th Geneva, Switzerland. 9th International Conference of the EARLI SIG 14. Available online at: https://research.tue.nl/en/publications/teachers-professional-identity-configurations-of-personal-and-con (accessed February 3, 2020).
Scheltenaar, K. J., van der Poel, J. E. C., and Bekker, M. M. (2015). “Design-based learning in classrooms using playful digital toolkits,” in Entertainment Computing—ICEC 2015, eds K. Chorianopoulos, M. Divitini, J. Baalsrud Hauge, L. Jaccheri, and R. Malaka, Vol. 9353 (Trondheim: Springer International Publishing), 126–139.
Schlegel, R. J., Chu, S. L., Chen, K., Deuermeyer, E., Christy, A. G., and Quek, F. (2019). Making in the classroom: longitudinal evidence of increases in self-efficacy and STEM possible selves over time. Comp. Educ. 142, 103637. doi: 10.1016/j.compedu.2019.103637
Starr, C. R., Hunter, L., Dunkin, R., Honig, S., Palomino, R., and Leaper, C. (2020). Engaging in science practices in classrooms predicts increases in undergraduates' STEM motivation, identity, and achievement: a short-term longitudinal study. J. Res. Sci. Teach. 57, 1093–1118. doi: 10.1002/tea.21623
Stets, J. E. (2020). “Chapter 4 identity theory,” in Contemporary Social Psychological Theories, eds. P. J. Burke (Stanford, CA: Stanford University Press), 81–111.
Strobel, J., and van Barneveld, A. (2009). When is PBL more effective? A meta-synthesis of meta-analyses comparing PBL to conventional classrooms. Interdiscip. J. Prob. Based Learn. 3, 1046. doi: 10.7771/1541-5015.1046
Taconis, R. (2021a). “Representing STEM identities as pragmatic configurations,” in Science Identities—Theory, Method and Research, eds. L. Archer and H. T. Holmegaard (Cham: Springer).
Taconis, R. (2021b). “Acquiring theoretical knowledge in challenge based learning,” in Proceeding of the 49th SEFI Annual Conference 2021 (Berlin, 13 – 16 September 2021).
Taconis, R., Bekker, T., Bakker, S., and Van Der Sande, A. (2018). “Developing the teach21 online authoring tool: supporting primary school teachers in designing 21st century design based education,” in Proceedings of the 10th International Conference on Computer Supported Education (Madeira), 91–98.
Taconis, R., den Brok, P. J., and Pilot, A. (2016). Teachers Creating Context-Based Learning Environments in Science. Rotterdam: Sense Publishers. doi: 10.1007/978-94-6300-684-2
Taconis, R., and Hobbelen, H. (2023). Challenge Based Tasks For Fundamental Knowledge. Centre for Engineering Education. Available online at: https://www.4tu.nl/cee/innovation/project/13179/challenge-based-tasks-for-fundamental-knowledge (accessed March 25, 2023).
Taconis, R., and Kessels, U. (2009). How choosing science depends on students' individual fit to ‘science culture.' Int. J. Sci. Educ. 31, 1115–1132. doi: 10.1080/09500690802050876
Talafian, H., Moy, M. K., Woodard, M. A., and Foster, A. N. (2019). STEM identity exploration through an immersive learning environment. J. STEM Educ. Res. 2, 105–127. doi: 10.1007/s41979-019-00018-7
Tan, C. P., Van der Molen, H. T., and Schmidt, H. G. (2016). To what extent does problem-based learning contribute to students' professional identity development? Teach. Teach. Educ. 54, 54–64. doi: 10.1016/j.tate.2015.11.009
Thomas, J. W. (2000). A Review of Research on Project-Based Learning. San Rafael, CA: TA Foundation.
TU/e (2018). TU/eStrategy 2030: Drivers of Change. Eindhoven: Eindhoven University of Technology. Available online at: https://assets.tue.nl/fileadmin/content/pers/2018/09_september/TUE_Strategie_2030-LR.pdf
Tulloch, R. (2014). Reconceptualising gamification: play and pedagogy. Digital Cult. Educ. 6, 317–333.
University Twente (2022). What is Challenge-Based Learning all about. Universiteit Twente. https://www.utwente.nl/en/cbl/what-is-cbl/
van Breukelen, D. H. J., de Vries, M. J., and Schure, F. A. (2017). Concept learning by direct current design challenges in secondary education. Int. J. Technol. Design Educ. 27, 407–430. doi: 10.1007/s10798-016-9357-0
van den Beemt, A. A. J., van de Watering, G., and Bots, M. (2021). Challenge-Based Learning in a Higher Education Context. Eindhoven: Eindhoven University of Technology.
van den Beemt, A. A. J., van de Watering, G., and Bots, M. (2022). Conceptualising variety in challenge-based learning in higher education: The CBL-compass. Eur. J. Eng. Educ. 48, 1–18. doi: 10.1080/03043797.2022.2078181
van den Hurk, A., Meelissen, M., and van Langen, A. (2019). Interventions in education to prevent STEM pipeline leakage. Int. J. Sci. Educ. 41, 150–164. doi: 10.1080/09500693.2018.1540897
Walker, A., and Leary, H. (2009). A problem based learning meta analysis: differences across problem types, implementation types, disciplines, and assessment levels. Interdiscipl. J. Problem Based Learn. 3, 1061. doi: 10.7771/1541-5015.1061
Wang, J., and Hazari, Z. (2018). Promoting high school students' physics identity through explicit and implicit recognition. Phys. Rev. Phys. Educ. Res. 14, 020111. doi: 10.1103/PhysRevPhysEducRes.14.020111
Keywords: identity, science education, STEM education, identity development, educational design
Citation: Taconis R and Bekker T (2023) Challenge Based Learning as authentic learning environment for STEM identity construction. Front. Educ. 8:1144702. doi: 10.3389/feduc.2023.1144702
Received: 14 January 2023; Accepted: 14 June 2023;
Published: 01 August 2023.
Edited by:
Anneke Steegh, University of Kiel, GermanyReviewed by:
Zhe Li, Osaka University, JapanAndrée Tiberghien, Centre National de la Recherche Scientifique (CNRS), France
Copyright © 2023 Taconis and Bekker. This is an open-access article distributed under the terms of the Creative Commons Attribution License (CC BY). The use, distribution or reproduction in other forums is permitted, provided the original author(s) and the copyright owner(s) are credited and that the original publication in this journal is cited, in accordance with accepted academic practice. No use, distribution or reproduction is permitted which does not comply with these terms.
*Correspondence: Ruurd Taconis, r.taconis@tue.nl