- 1Complex Systems in Sport Research Group, Institut Nacional d’Educació Física de Catalunya (INEFC), Universitat de Barcelona (UB), Barcelona, Spain
- 2Complex Systems in Sport Research Group, Departament d’Ensenyament, Generalitat de Catalunya, Barcelona, Spain
- 3Complex Systems in Sport Research Group, Faculty of Physical Education, Sport and Health, Ss. Cyril and Methodius University, Skopje, North Macedonia
Introduction: Connecting academic disciplines and integrating knowledge is gaining popularity in elementary school. The relevant question is, how the targeted integration could be achieved? This research aimed to (a) evaluate the potential of teaching and learning Dynamic Systems Theory (DST) and Statistical Physics (ST) general concepts through embodied experiences in elementary school and, based on it (b) enable a far transfer analogical reasoning within and between different academic subjects.
Methods: Forty-eight elementary school students, aged 10.2 ± 0.82 y.o., followed an 8-week, 2-h/week intervention. The learning protocol contained a concreteness fading sequence of phases and consisted of four steps: (a) embodied experience, (b) reflective observation, (c) abstract conceptualization of DST/ST concepts, and (d) transfer of the DST/SP concepts to physical, sociological, biological and ecological phenomena. A validated questionnaire and an interview evaluated students’ knowledge and analogical reasoning.
Results: The Wilcoxon Signed Ranks Test showed a general positive effect of the intervention on the understanding of DSP/SP concepts and on the far transfer competencies of students (Z = −5.98; p < 0.0001). There was no association between the previous and newly acquired competencies (Spearman’s ρ = 0.112; p = 0.441).
Discusion: The qualitative results showed that, in general, the suggested embodied learning protocol supports the acquisition of DST/SP general concepts and the far transfer competencies. However, students of this age are possibly highly sensitive to the balance of the focus between the embodied phases and the conceptualization/transfer phases of the learning protocol. The study points to the potential of learning the general DST/SP for elementary school students’ integrative and far transfer competencies. The DST/SP concept-based transdisciplinary embodied education may offer a truly integrative approach to STEAM teaching.
1. Introduction
Linking different disciplines and phenomena is crucial for developing reasoning and critical thinking (Anderson and Krathwohl, 2001; Cañal et al., 2013; Adams, 2015; Clapp and Jiménez, 2016; Bautista et al., 2018). However, contemporary education, traditionally based on a fragmented structure of subjects, limits students’ integrating and knowledge transfer competencies (Goldstone, 2006; Jacobson and Wilensky, 2006; Hristovski, 2013).
Science education stands at the forefront of this endeavor by taking the active role of a pathfinder of connections in the complex landscape of academic disciplines. Indeed, integrating apparently disparate disciplines may be a crucial contribution to advancing science (Sengupta, 2019). In this line, there are exciting initiatives under the framework of STEAM (Science, Technology, Engineering, Arts, and Mathematics). However, the educational possibilities of integrating and transferring knowledge still need to be deeply explored.
STEAM education is a relatively new educational framework that aims to break down boundaries between disciplines taught in isolation and address actual-world problems (Dakers, 2006; Yakman, 2008; Baek et al., 2011). Typically, in STEAM, authors propose interdisciplinary models, integrating at least the contents of two of the acronyms (Stock and Burton, 2011; Yakman and Lee, 2012; Zamorano et al., 2018), and transdisciplinary models. Seeking to impact all educational fields, moving beyond specific disciplines and involving the space between disciplines, the transdisciplinary teaching of STEAM searches to produce new perspectives (Gibbs, 2015). Some examples include critical numeracy (Das and Adams, 2019), critical ecological sustainability (Kim et al., 2019; Lam-Herrera et al., 2019), and macroethics (Gupta et al., 2019).
Many countries are investing in STEAM learning at K-12 levels, for example, China (Li and Chiang, 2019), Australia (Sharma and Yarlagadda, 2018), England (Wong et al., 2016), and Canada (e.g., Science Technology and Innovation Council, 2015; Shanahan et al., 2016). Even South Korea has made STEAM the core of its education system (Yakman and Lee, 2012; Hong, 2016). However, there is still limited research on STEAM’s validity and effectiveness in the classroom. In addition, STEAM’s conceptualization is considered insufficient because the proposals and resources from specific disciplines do not provide acknowledgement of the commonalities between them (Zamorano et al., 2018; García-Carmona, 2020).
Although K-12 and high schools are adopting STEAM teaching methodologies, there is still limited empirical evidence about their benefits on students’ abilities to integrate and transfer knowledge. The lack of a well-founded integrative framework of the education curricula and the lack of teachers’ preparation to connect STEAM disciplines can explain such limitations (Radloff and Guzey, 2016). As suggested here, professionals from the STEAM disciplines work together on interdisciplinary projects but are rarely familiarized with accurate transdisciplinary approaches. Their academic training is usually fragmented into various specialties. The vast amount of different undergraduate and graduate degrees in science and engineering that universities offer each year proves it. The most common practice in a STEAM project is that each professional contributes to it through his/her area of knowledge (García-Carmona, 2020). This reveals STEAM primarily as a multidisciplinary approach, a non-integrative mixture of disciplines with only sporadic and superficial links among the subjects.
1.1. Early ideas and educational initiatives on understanding of complex systems
Intuitions about the educational benefits of using the commonalities between different phenomena and levels of organization have been expressed previously (e.g., Haken, 1977; Forrester, 1994). Early education initiatives have successfully used some concepts coming from the dynamical systems theory (DST) and statistical physics (SP) (from now on -DST/SP) concepts to understand complex systems’ levels formation (e.g., Resnick and Wilensky, 1998; Wilensky and Resnick, 1999). Hence, the research on explaining complex systems phenomena was mainly focused on important concepts such as emergence and self-organization. For instance, some software packages allow students to manipulate computer-simulated environmental constraints to study the dynamic behavior of molecules, cells, etc. (Wilensky and Reisman, 2006) and integrate micro- and macroscopic chemical organizational levels (Stieff and Wilensky, 2003). The authors studied students’ learning responses to computer agent-based-modeling relations between micro and macro–level phenomena on chemical, physical and social levels of substance organization. This line of research resulted in seminal reviews, position and prospect papers (Jacobson and Wilensky, 2006; Goldstone and Wilensky, 2008; Wilensky and Jacobson, 2015).
However, focusing solely on concepts and principles that are common for complex systems will still leave a fragmentation between systems that are defined as simple (e.g., pendulums, springs, elementary fields/particles, projectiles, mechanical tools, motion of artificial satellites, etc.), and truly complex systems that consist of a vast number of interacting components (e.g., fluids, cells, organism, societies etc.). Hence, the most crucial problem of fragmented education promised to be solved (Goldstone, 2006) would remain unsolved. This is especially relevant for STEM education since in the areas of technology and engineering, many sub-systems belong to the class of simple or, as a whole belongs to the class of complicated1 systems. Hence, the set of concepts and principles that would satisfy the constraint of unifying simple, complicated and complex systems under the same umbrella was a new and challenging problem.
The second challenge to this goal is that the number of concepts/principles should not be too large and should not be level- or phenomenon-dependent. We explain in more detail this idea below.
1.2. The SUMA-transdisciplinary embodied learning framework: conceptual approach
To solve the two main challenges discussed above and to achieve a conceptual integration of understanding among academic disciplines covering the whole spectrum of phenomena (from elementary fields/particles to sociology and art), including simple and complicated systems, there is a need for a “common conceptual currency” that can act as a glue for the integration. The Synthetic understanding through movement analogies (SUMA) learning framework (for the scientific basis of the framework, see Hristovski, 2013; Hristovski et al., 2014, 2019, 2020; Almarcha et al., 2022) approached this problem by putting forward a set of interconnected general concepts and principles coming from DST/SP which can play the role of a ‘common conceptual currency.2 However, extracting and verifying the general DST/SP principles/concepts from the above science fields, in which myriads of them vastly differ in generality, is an extraordinarily challenging problem. As said above, under the term ‘truly general concepts’, we mean concepts/principles instrumental in explaining dynamic phenomena of simple, complicated and complex systems in all levels of substance organization. More technically, truly general concepts represent level-independent ‘invariants’ (Hristovski, 2013; Nathan and Alibali, 2021) encompassing all levels of substance organization. These truly general concepts/principles are necessary for achieving the far-transfer (Goldstone, 2006) goals of the SUMA educational framework. Although these concepts/principles are necessary, they cannot explain many phenomena comprehensively. Hence, in SUMA, the interplay between the truly general (the skeleton) and level dependent (the meat) concepts/principles forms the educational apparatus for achieving the far-transfer goals.
The empirical research with aim to extract and verify concepts of the broadest possible generality showed that 14 DST/SP concepts are explanatorily relevant for the whole set of academic disciplines ranging from physics of elementary fields/particles to sociology and art (Hristovski, 2013; Hristovski et al., 2019) (see footnote 2). These concepts and principles, such as stability and instability, gradients, mass-energy, information entropy, phase transitions, constraints, state variables, symmetry-symmetry rearrangement, etc., form the transdisciplinary embodied approach within the SUMA educational framework. They create the skeleton of the academic disciplines, playing the role of discipline-free concepts that are explanatorily involved in dynamic phenomena of all academic disciplines. On the other hand, each one of the disciplines also contains a large (in fact, much larger) set of discipline-dependent concepts. Discipline-free and discipline-dependent concepts interact to provide explanations for each set of phenomena.
We offer some examples to contrast the SUMA educational framework with similar approaches. For instance, concepts such as: adaptation, evolution, fitness, altruism and selfishness (Wilensky and Centola, 2007) or homeostasis (Jacobson, 2001) are certainly relevant for all biological and social systems but not non-biological ones. Thus, in order to enable a unified account of biological and non-biological systems, by using far transfer analogies, SUMA emphasizes more general concepts and principles, such as ‘variability’ (i.e., ‘fluctuations’ and ‘perturbations’), ‘constraints’, ‘interactions’, gradient flow, and ‘stability-instability’, which are crucial in explaining adaptation, evolution, fitness and homeostasis as special instantiations of biological phenomena. Within the SUMA framework, concepts like altruism and selfishness are treated as biologically relevant constraints but not as general concepts. Because only stable entities survive (from elementary particles, planetary orbits to biological species), stability is the general precondition for natural selectivity. Hence, general concepts like ‘stability-instability’ are also crucial for explaining non-biological selectivity phenomena. The conceptual pair stability-instability plays a crucial role also in control theory which is fundamental to technology and engineering. Another concept, such as ‘energy minimization’ (e.g., Goldstone and Wilensky, 2008) is certainly of high generality, and in systems in thermodynamic equilibrium generates the system’s stable behavior. However, in non-equilibrium (e.g., biochemical enzyme kinetics) systems, the principle of ‘entropy production maximization’ is often responsible for the stability of the system’s patterns of behavior (e.g., Dobovišek et al., 2018). Hence, ‘stability - instability’ would appear as a more general concept/principle which may be generated either by energy minimization or entropy production maximization, depending on the concrete phenomenon. In this situation of far-transfer between equilibrium and non-equilibrium phenomena, this concept would have a role of deep analogy among the initial and target phenomena. Yet, another example are the concepts such as: ‘fractal’, ‘power law and ‘graph’ (Lage-Gómez and Ros, 2021). In contrast, the DST/SP concept-based transdisciplinary embodied educational framework - SUMA uses concepts of a high level of generality to achieve these ends. For example, the concepts of fractals, power laws and graphs can be subsumed into the more general DST/SP concepts of ‘symmetry - symmetry breaking’ and ‘interactions’, respectively, because fractals and power laws are a special case of the concept of ‘symmetry’ (i.e., scale symmetry), and graphs are a unique representation of the concept of ‘interaction’.
Hence, although SUMA transdisciplinary approach shares some similarities with other approaches (particularly those focused solely on complex systems education), it represents a distinct and genuine approach in important aspects. Hence, it offers powerful means for intersecting systems thinking, e.g., in biology with other STEM disciplines (Trujillo and Long, 2018; Momsen et al., 2022). First, it unifies (solves the fragmentation problem) among simple, complicated and complex systems; Second, but not less significantly, its reliance on truly general concepts/principles reduces their number and renders some concepts of narrower generality as level-dependent concepts/phenomena.
The extant interdisciplinary and transdisciplinary learning (e.g., Cone et al., 2009; Stock and Burton, 2011; Yakman and Lee, 2012; Zamorano et al., 2018; Lage-Gómez and Ros, 2021) is a phenomenon (problem)-based. In other words, the problem or phenomenon is the integrating factor that engages the cooperation of different academic disciplines. For instance, an integrated perspective of a virus infection problem can be approached from social, biological, psychological sciences, etc. On the other hand, in SUMA, the DST/SP general concepts are the integrating factor. The same DST/SP concept or principle (or one set of interdependent concepts and principles) integrates many phenomena (or problems). These concepts provide the ‘integration substrate’ needed to integrate processes and phenomena of different levels of organization (Martínez et al., 2017). For instance, the principle of ‘tendency toward ‘stability’ in semantic relation with other general and phenomenon-dependent concepts can integrate many simple and complicated technological/engineering systems, as well as truly complex social and biological phenomena, such as damped oscillations of a spring, system of springs in vehicles, social stability, and path dependence of athlete’s response to training stimuli, respectively. These two approaches have been explained in more detail for sports and exercise sciences (Hristovski et al., 2017). While the first has been called the “many (academic disciplines) to one (problem/phenomenon)” approach, the second is called the “one (concept/principle) to many (academic disciplines)” approach (see Figure 1).
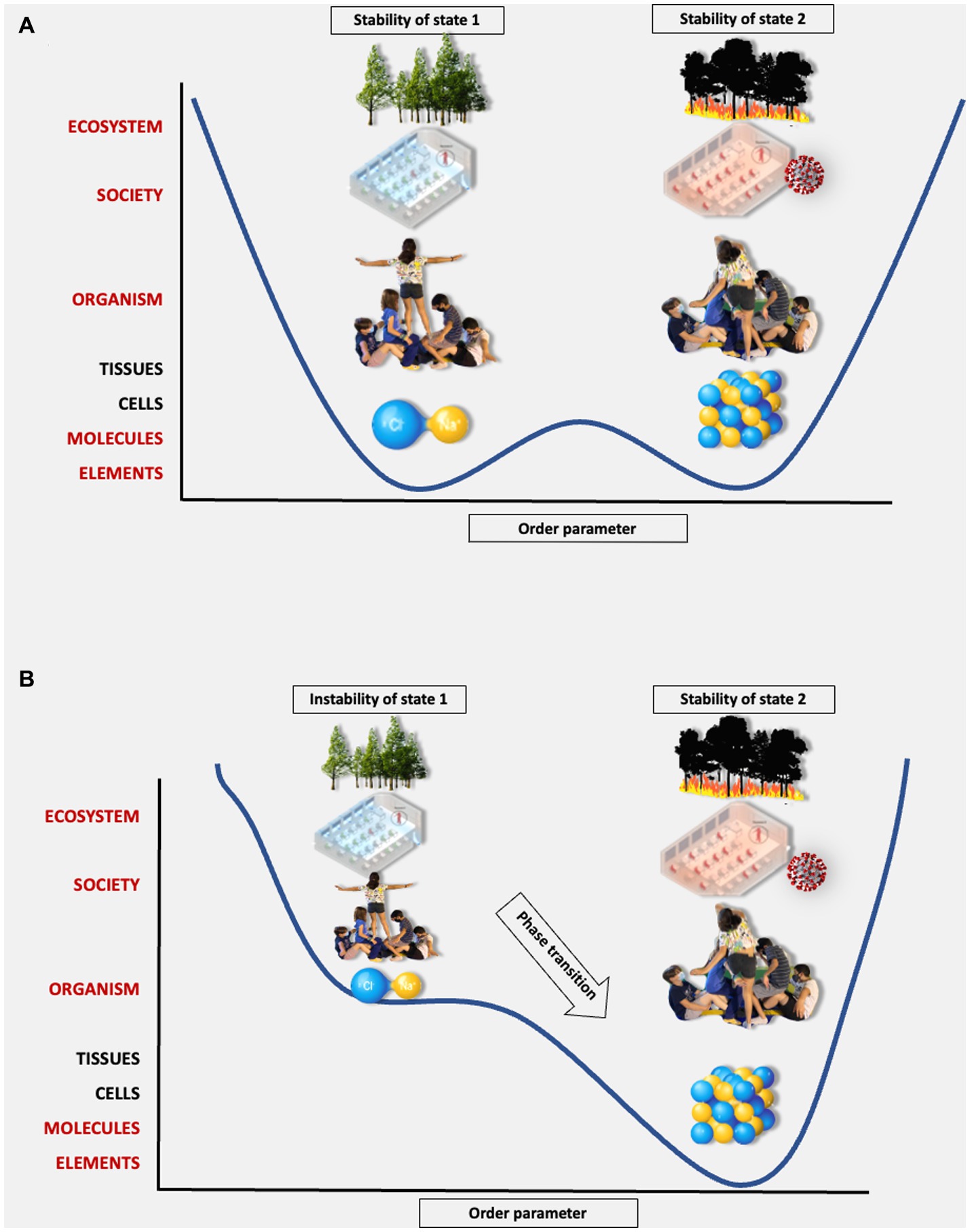
Figure 1. Stable states are represented as minima (bottom of the wells). Unstable states are represented as local maxima or inflection points. The order parameter (horizontal axis) represents the state of the system. (A) Representation of two stable states of an order parameter in phenomena of different organization levels. At the ecosystem level, it is represented by a green and burned forest; at the society level, a healthy and infected classroom; at the group level, a stable and collapsed acrosport figure; at the molecular level, the water is in the liquid and solid state. (B) Representation of the phase transition between the attractor states of the order parameter due to constraints (distance among trees, the distance among students, number of supports and temperature, respectively).
1.2.1. The embodied part of the SUMA educational framework
The SUMA educational framework also pays serious attention to the importance of embodied learning principles (Niedenthal, 2007; Stolz, 2015; Skulmowski and Rey, 2018). The embodied approaches to learning emerging in recent decades reinvented Poincare’s ideas of the central role movements have for cognizing the space and its geometry (Hristovski et al., 2014). Body movement experiences have already been used to study concepts in separate subjects such as mathematics, physics, biology, music, or culture (e.g., interdisciplinary physical education, Cone et al., 2009) and have gained some popularity in elementary and secondary school education due to their cognitive effectiveness and knowledge improvement (Clary and Wandersee, 2007; Schwartz-Bloom et al., 2011; Spintzyk et al., 2016). Recently, enactive and embodied approaches to STEM were successfully advocated on theoretical grounds (Hutto et al., 2015). The close link between movements and cognition has been innovatively elaborated (Abrahamson and Mechsner, 2022) with concrete applications in mathematics. On the other hand, preliminary research results have shown that DST/SP abstract general concepts can be mediated successfully using movement analogies (Almarcha et al., 2022). The embodied learning may show itself as an effective way of application of the educational principle of ‘concreteness fading’ (Terrace, 1963; Bruner, 1966; Goldstone and Son, 2005; Fyfe et al., 2014), in which the concreteness of the experience of learners gradually transits toward more abstract, and hence, context-free patterns of understanding, which are more transferable to new experiences (i.e., phenomena).
In order to apply the protocol of ‘concreteness fading’ Hristovski et al. (2014) proposed the following four phases of embodied learning of DST/SP concepts and their use in far or near transfer, based on Kolb (1984):
1. Embodied experience (a physical activity: perception-action or/and introspection).
2. Reflective observation of the embodied experience (paying attention to key perceived phenomena and their phases).
3. Abstract conceptualization (see Table 1) is based upon the previous reflective observation phase but proceeds to conceptualization, estimation and plotting of relations of perceived phenomena.
4. Experimenting with the newly acquired concepts and applying them to different fields and phenomena studied in the academic curriculum (i.e., transfer phase).
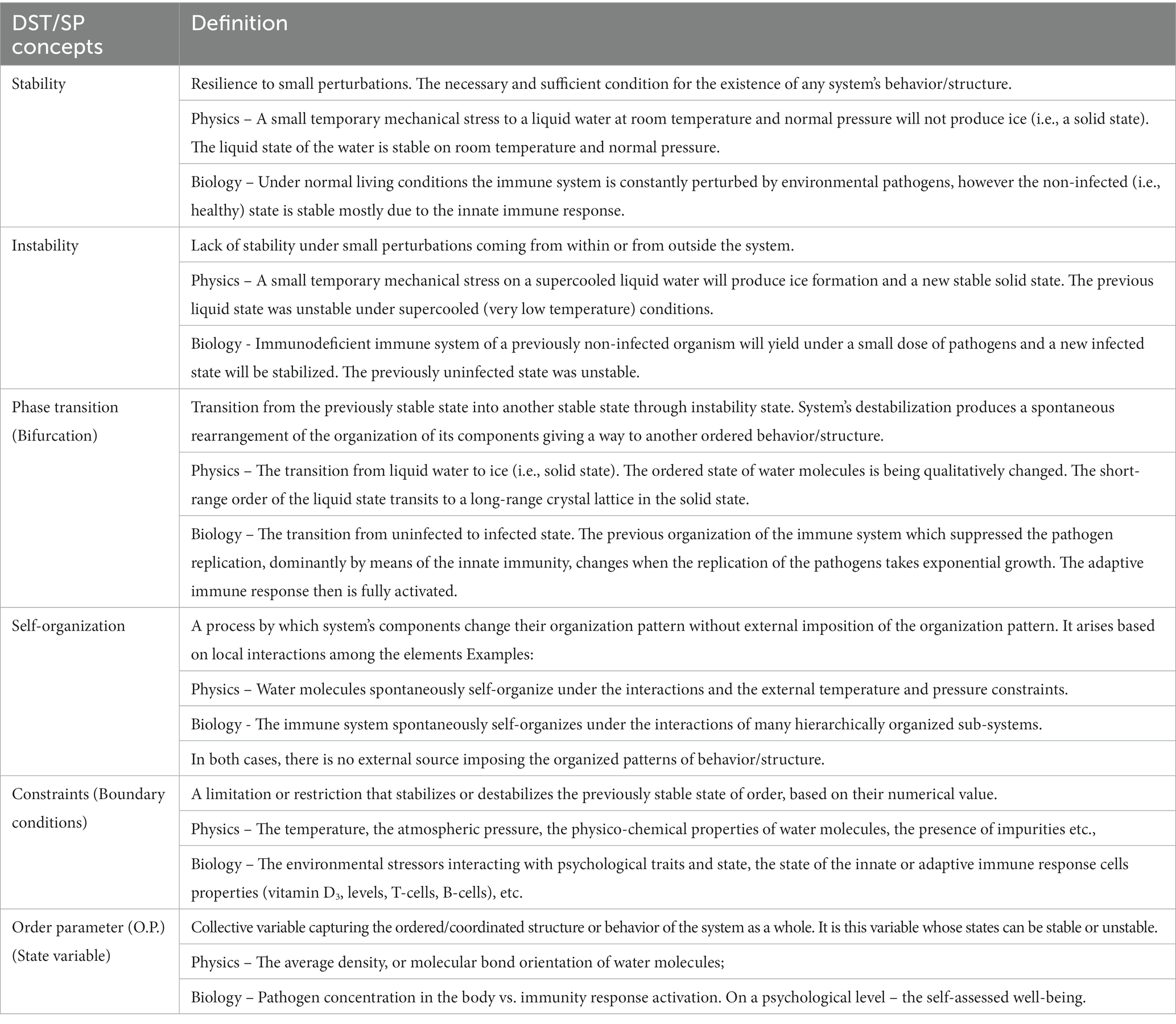
Table 1. Definition and examples in physics and biology of the concepts explained and worked during the intervention.
Note that, in the case of this protocol, the first two phases do not use the standard external representations (e.g., Bruner, 1966), but a first-person perspective concrete experience based either on perception-action cycles and/or on introspective judgments.
According to what has been discussed in the previous sections, we hypothesize that the embodied intervention will have a generally positive effect in acquiring general DST/SP concepts and their far transfer role among phenomena studied in different academic subjects of 5th grade elementary school. Particularly, we hypothesized that the four learning phases of the inbuilt concreteness fading protocol would (a) lead to the acquisition of the abstract meaning of the targeted general DST/SP concepts, and (b) enable a far transfer ability for connecting different phenomena.
2. Materials and methods
2.1. Participants
Forty-eight elementary school students of 5th grade (26 boys, 22 girls; 10.2 ± 0.82 y.o.) from two different class groups participated in the study. Ten (5 boys and 5 girls from each class) were randomly selected and interviewed individually about the learnt DST/SP concepts. Students did not have prior knowledge or experience with the contents and procedure of the intervention. After explaining the procedures, the legal educator signed informed consent. The local ethic committee approved the study according to the Helsinki declaration.
2.2. Design
A quasi-experimental design comparing pre- and post-intervention results was applied. As DST/SP concepts are not included in the elementary education curriculum, a control group was considered unnecessary in this research.
2.3. Procedure
The study was conducted in a physical education facility. The intervention lasted eight weeks and had a frequency of two lessons/week of 1-h duration. It was developed during regular physical education lessons and was led by a PE teacher and researcher with more than ten years of experience, respectively, applying DST/SP concepts. One experimenter supervised the intervention in situ. The learning phases proposed by Hristovski et al. (2014) were the following:
1. Students performed the proposed physical activities focusing attention on relevant aspects of the body experiences for the association with the DST/SP concepts (see Table 2).
2. Reflection of previous body movement experiences.
3. Association of movement experiences with general DST/SP concepts (see Table 1) through videos and photographs.
4. Transfer of the DST/SP concepts to molecular, ecological and sociological phenomena (see Table 2; Figure 1).
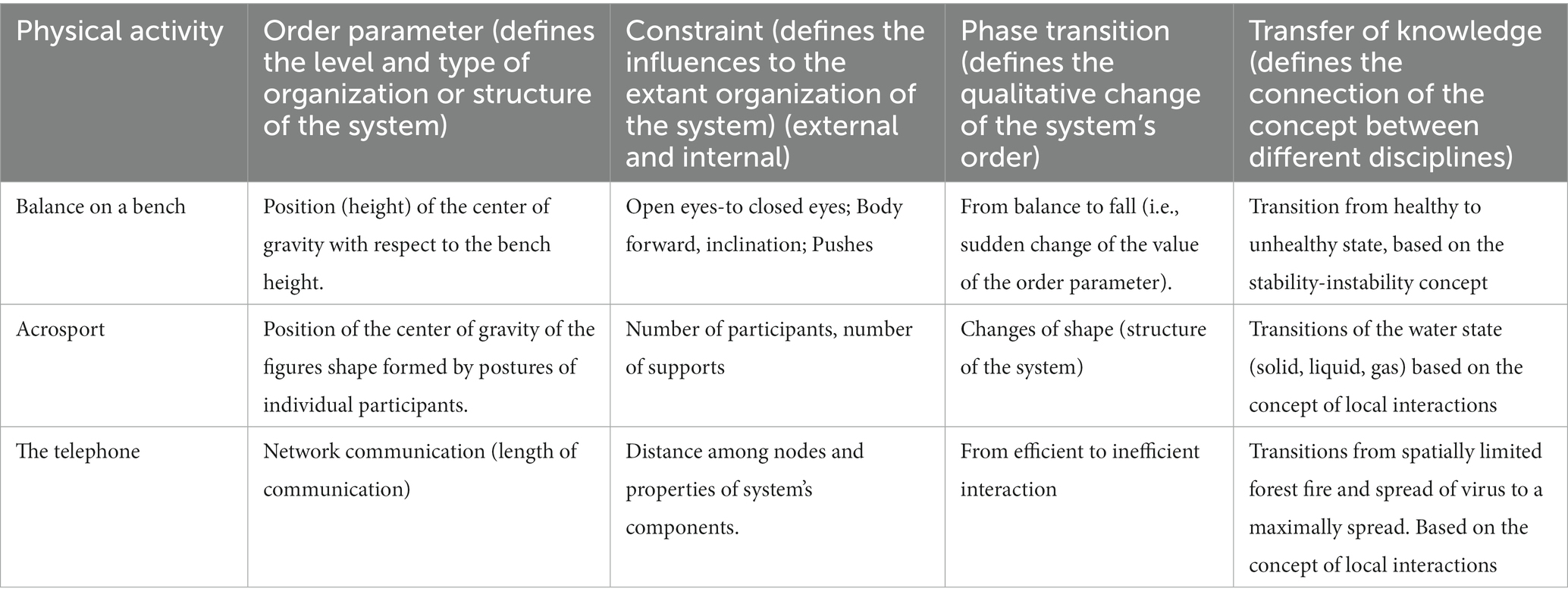
Table 2. Examples of applied physical activities, their relation with DST/SP concepts and their transfer to molecular, ecological and sociological phenomena.
Table 2 displays three examples of physical activities proposed to the students for learning DST/SP concepts of Table 1 and transferring them to ecological, molecular and biological phenomena. The transfer of knowledge was discussed with the students after each activity through audiovisual material projected in class. In the first activity, students had to maintain balance on a bench with open and closed eyes while a partner “pushed” them with different intensities. The order parameter (O.P.) was the body’s center of gravity position with respect to the bench height. This O.P. kept stable under open eyes (released constraint) but destabilized with closed eyes enhanced constraint. The perturbations (pushes) affected the O.P. differently under stable and unstable conditions: the same push intensity that could be adequately compensated to keep the balance produced a fall under unstable conditions. The transfer to our healthy or unhealthy physiological state was done as follows: when our immune system is adapted to the environmental constraints, our organism is in a stable4 healthy and uninfected state, and can control a pathogen attack. However, when it is constrained (e.g., by stress), the perturbation produced by a pathogen attack may destabilize the uninfected state and produce severe health consequences, i.e., a new stable state (disease, e.g., infection) (see, e.g., Krishnapriya and Pitchaimani, 2017; Shi et al., 2020; Su et al., 2022). The following reflective observations were addressed to students: “What did you experience while maintaining the balance?,” “What did you experience close to the fall? and when falling?”
In the acrosport activity, students had to build a stable acrosport figure in groups. Through instructions that manipulated some figure constraints (e.g., number of participants, number of supports and supporting surface), the figure destabilization was provoked and a change of its initial shape occurred; finally, additional constraints led to the figure collapse. The changes in the shape of the figure can be transferred to the changes of the structure of water (solid, liquid, gas) under slow unidirectional temperature variation (i.e., constraint changes).
In the telephone game, students sat in a circle touching their partners and transmitting messages with their hands. The initial stable communication was destabilized when the distance (acting as a role of constraint) among students increased until transmission was impossible. The experience was transferred to fire and virus infection spreading. In a forest, a fire spreads when the distance between trees is reduced, and in human societies, a virus infection spreads when the distance between unprotected (e.g., low D3 vitamin concentration) persons is reduced.
2.4. Instruments
The understanding of the selected DST/SP concepts was assessed through a questionnaire administered through a Plicker’s application.5 It consisted of five closed questions with four answer options and only one correct answer. Surveys, case studies, interviews, and students’ self-reporting questionnaires are among the most frequently chosen methods to investigate the effectiveness of transdisciplinary educational interventions (Takeuchi et al., 2020; Lage-Gómez and Ros., 2021). In particular, considering that knowledge transfer is difficult to measure objectively, close-structured interviews and questionnaires seem to be the most appropriate for children in elementary school.
2.4.1. Plickers questionnaire
Students responded to the Plickers questionnaire one day before and one day after the intervention. They were requested to answer honestly, emphasizing that their answers would be confidential and not affect their academic marks. They did not perform any additional learning activities (e.g., homework or additional lessons) during the intervention period. The content validity of the questionnaire was determined by two researchers with thirty years of experience in using CD concepts. Also, the students assessed face validity (clarity of the questions and the level of understanding). The internal consistency of the questionnaire was measured by Cronbach’s alpha. The questions of the Plickers Questionnaire were the following:
1. When do we say that a system is stable?
2. When do we say that a system is unstable?
3. Does a burned forest represent a stable state?
4. Do a forest fire, and a virus infection spread in the same way?
5. Which of the following statements about self-organization are correct?
In order to enable the detection of the ‘concreteness fading’ effect (Goldstone and Son, 2005) for questions 1, 2, and 5, the answers contained incorrect, superficially correct and correct answers (please see Table 3). Although correct, the content of superficially correct answers was oriented toward the actual bodily experience and not toward the more abstract content of the correct answers. This allowed us to check whether students have acquired the deeper analogy enabling the far transfer or stayed stuck merely at the correct interpretation of the bodily experience.
2.4.2. Interview
To avoid bias, students conducted a semi-structured interview by an external researcher. The reliability of the interviews was guaranteed by the same researcher, the same design scheme of the questions, the same length of interrogation and the same time for all participants. The interviews were audio recorded and transcribed verbatim. Two education researchers validated the interview questions before the study. It consisted of the following questions:
1. 1.1. For you, what is a stable and an unstable state/1.2 Can you describe a situation in which you feel stable and unstable?
2. Do you know any phase transitions? What is the initial stable state before water crystals are formed and before a fire or a virus infection spreads?
3. What does self-organization mean to you?
4. What natural processes, such as the spread of a fire and the formation of water crystals, have in common with social processes, such as the spread of COVID-19?
2.5. Data analysis
2.5.1. Plickers questionnaire
The percentages of correct and incorrect answers for the five questions in pre- and post-intervention were calculated.
2.5.2. Interviews
The interviews were transcribed, and two researchers scored each response according to the rubric presented in Table 4. The data matrix was organized in 2 (pre- and post-intervention) columns × 50 (10 students × 5 scores) rows. Cohen’s kappa analysis was performed to test the inter-observer agreement. The Kolmogorov–Smirnov test of normality tested the null-hypothesis of pre- and post-intervention scores’ normal distribution. The Wilcoxon signed-rank test was used to compare pre-and post-intervention responses. To check for a possible association between students’ pre- and post-intervention scores, we performed Spearman’s ρ correlation. All quantitative data were analyzed using SPSS software (Version 28.0.1). The significance level for rejecting the null hypothesis claiming that the pseudomedian of the differences between pre-and post-intervention scores of students is located at zero was set on p = 0.05.
3. Results
3.1. Plickers questionnaire
Experts confirmed that all items were relevant to the target population and the intended purpose of the questionnaire. All students answered that the questions were clear and understandable. The assessed inter-item reliability of the questionnaire was α = 0.72.
The detailed results from the Plickers questionnaire are given in Table 3. Before the intervention, the percentages of correct responses were 26, 35, 19, 45, 30, and 31% in each question, respectively. After the intervention, results improved, with question 1.1 having the lowest percentage of correct answers (41.5%) and questions 1.2 and 3 having the highest percentage of correct answers with 77 and 75%, respectively. Question 2 had 46% of correct responses.
3.2. Interviews
The Kappa analysis showed a high concordance of the interobserver scores (k = 0.81). Table 5 shows the student’s results of the interview questions scored by researchers before and after the intervention. The two-tailed Kolmogorov–Smirnov test revealed a significant difference in the scores’ data from the normal distribution at a significance level of p < 0.0001. The two-tailed Wilcoxon signed rank test revealed a clear effect of the intervention on students’ knowledge and transfer abilities (pre-intervention mean rank = 1.28; mode = 1; vs. post-intervention mean rank = 4.06; mode = 4); Z = −5.98; p < 0.0001. The Spearman’s ρ correlation showed no association between the pre- and post-intervention scores; ρ = 0.112; p = 0.441.
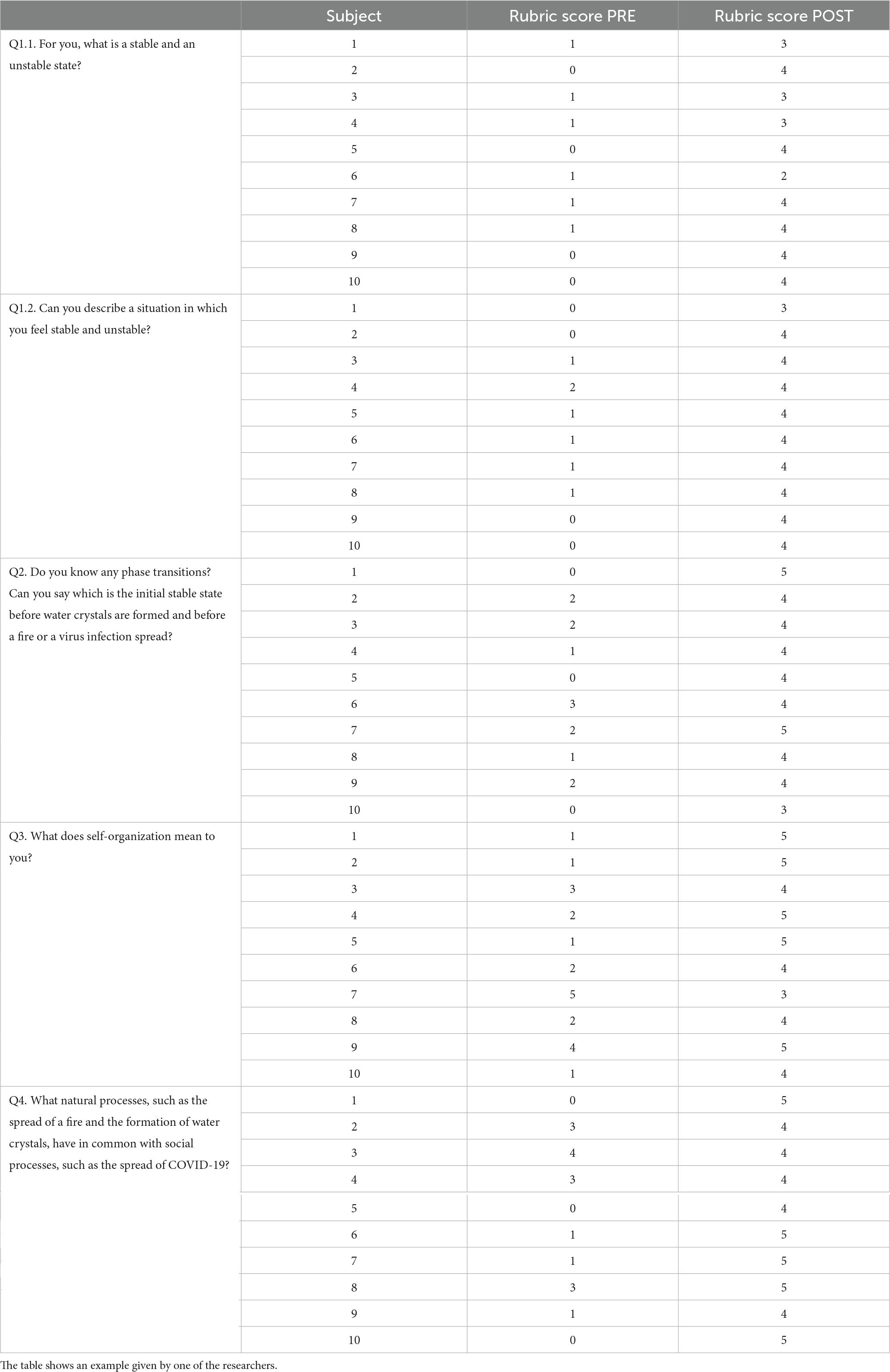
Table 5. Comparison of the pre- and post-intervention rubrics results obtained during the interview for each participant.
4. Discussion
This research aimed to evaluate if the SUMA embodied learning protocol with inbuilt concreteness fading structure: (a) will lead to the acquisition of the abstract meaning of the targeted general DST/SP concepts, and (b) will enable a far transfer ability for connecting different phenomena. The results showed that embodied transdisciplinary learning in 5th-graders can positively integrate topics of different STEM subjects, with some caveats. These findings are relevant because the learners age corresponds to the concrete operational stage in Piagetian classification of a child’s cognitive development.
4.1. Plickers questionnaire
The questionnaire results showed that, in general, the applied DST/SP concepts are learnable and transferable among phenomena taught in different academic subjects in elementary school 5th graders. However, the first question from the questionnaire, concerning the meaning of the concept of ‘stability’, showed interesting and, to a degree, unexpected results. As said in the methods section, of the three possible answers, the first one was false, but the next two were true, one of which was the correct abstract definition. In contrast, the other one was a more superficial definition with a more immediate relation to the embodied experience.
In class A, the majority of students in the pre-intervention phase chose the more superficial definition as a correct answer migrated to the correct, more abstract definition in the post-intervention phase. This class showed a typical effect of ‘concreteness fading’ (Goldstone and Son, 2005). The contrary happened in class B. Although the percent of the correct abstract definition increased, the percent of students who chose the more superficial definition increased, too. This apparent paradox of ‘concreteness enhancing’ may be possibly responsible for the slightly different emphasis on the ‘embodied experience’ and the ‘reflection on the experience’ phases instead of the ‘association-conceptualization’ and ‘transfer phases’ of the learning. Also, during the embodied and reflection phases, an emphasis to the ‘balance’ (present in the bench balancing) and the ‘structure’ (present in the acrosport experience) concepts may be possibly needed in order to make the ‘stability-balance-structure-state’ link more achievable for students. This is highly likely needed to emphasize the knowledge that what is ‘stable’ is the ‘structured/organized’ ‘state’ of the system itself.
Contrary to the concept of ‘stability’, the acquisition of the meaning of the concept of ‘instability’ did not show the paradox mentioned above. In both classes, the percent of incorrect and superficially correct answers from the pre-intervention phase substantially decreased and consequently, the number of correct answers increased. In this case, akin to class A for the concept of stability, the effect of ‘concreteness fading’ (Fyfe et al., 2014) was detected, as most students in classes A and B migrated from the incorrect and superficially correct answers to the correct generalizable answer.
The pre-intervention answers to question 3, “Does a burned forest represent a stable state?” which primes the link of the general concept of ‘stability’ to the phenomenon dependent ‘state of burned forest’, showed a strong bias toward the ‘No’ answer. This could be a consequence of the (metaphorical?) association between the concepts of ‘stability’ and ‘life’, in only living entities are stable. The post-intervention results showed that students generalized the concept of ‘stability’ to refer also to non-living states, such as ‘burned forest’. Similarly, the far transfer question 4: Do forest fires and virus infections spread in the same way?; revealed a migration of students from the dominantly ‘No’ answer to the affirmative answer, showing that they have acquired the analogical link between the phenomena of pathogen spread and forest fire as depending on the constraints such as physical distance/interactions and the properties of the components (people and trees) of the system. Post-intervention results showed that relations between different phenomena were acquired based on the general concepts.
The acquisition of the concept of ‘self-organization’ also showed the same pattern of migration of students from the superficially correct and incorrect answers to the correct, more abstract statement. The process of self-organization was experienced through the acrosport activity in which students created macrostructures with their bodies through local interactions (support surfaces). Hence, the abstract insight of ‘spontaneous organization’ was immediately helped by the fact that neither teachers nor any other external agent imposed the shape of the macrostructure they succeeded in building. Instead, students built organized structures by finding local firm support surfaces on classmates’ bodies. In this case, the ‘concreteness fading’ was much more closely related to the embodied experience than in the case of the concept of ‘stability of a state’ since the concept of ‘state’ has a more abstract meaning than the visually immediate concept of ‘organization’ or ‘structure’. Among other things, this line of thought would support the claims expressed by Jaakkola and Veermans (2018), that there is no universal number of steps (phases) in concreteness fading protocols. It may well be possible that each pair of ‘student’s age - concept to be learned’ has its own optimal number of phases for concreteness fading.
4.2. Interview
In order to more deeply analyze the level of understanding of the general concepts and abilities for far transfer in students, we interviewed 10 randomly assigned students in pre- and post-intervention conditions. The mean ranks and the mode differences of pre-and post-intervention scores showed that the intervention helped the students to learn the DST/SP concepts and identify them in molecular, ecological and sociological processes. The most expected (i.e., mode) answer characteristic in the pre-intervention phase was impossibility of, or vagueness of, explaining the concept and/or offering wrong examples. After acquainting them to DST/SP general concepts, on average, they formed a deeper understanding of phenomena acquired through body movement experiences. In the post-intervention phase, the most expected (i.e., mode) answers were characterized by students being able to explain the concept and giving examples of phenomena in which that concept plays essential role. In addition, students increased the capacity to show enhanced abilities of far transfer (i.e., to integrate) diverse phenomena studied in different academic disciplines (e.g., virus infection and forest fire transmission in infectology and ecology, respectively). The Spearman’s correlation showed that students’ previous state of knowledge did not significantly impact the post-intervention results. This is understandable since students’ curricula did not contain any of the concepts or transfer contents that were applied during the intervention. Hence, students either gave random answers or used a kind of ‘common sense’ answers.
Although most students already used the far analogies as part of the intervention, only a few could provide new far transfer examples (not used or mentioned in class). For example, one student used the concept of phase transition to explain the phenomenon of mitosis: “When a cell is reproduced, it is a phase transition from one to two cells with the same genetic information.” Another one connected the same general concept with the formation of the sun: “the collapse of a cloud of gas and the mass accumulated over time resulted in a phase transition from gas to the formation of the sun.” These two students connected the previously acquired knowledge of these phenomena with the property of phase transitions to induce sudden and qualitative changes. The mitosis and star formation are phenomena of sudden qualitative change of the system’s previous state. These phenomena in cell biology and astrophysics have already been modeled as such (e.g., Jeans, 1902; Spencer et al., 2013, respectively). The success of these two students to extrapolate the general concepts knowledge to phenomena that were not explicitly learnt during the intervention might be a consequence of a successful combination of embodied experiences of a sudden change, iconic thinking (e.g., Herlofsky, 2011) about the disruptive properties present both in mitosis and star formation, and the previously acquired knowledge in the school (e.g., the Kant-Laplace hypothesis of sun formation).
In general, before the intervention, the students had an inaccurate meaning of some concepts. For instance, the concepts of stability and instability as reflected in the scores of interview questions 1.1 and 1.2. Also, four students could link questions 3, and 4 to prior (experiential or otherwise) knowledge and respond logically based on the implicit intuition of how local interactions may produce global outcomes. For example, a student recalled similarities between different phenomena (question 4): “In the case of the spread of a virus, if you reduce the distance from someone infected sufficiently, you can become infected too. In the case of forest fire propagation, if the distance among trees is reduced, the fire spreads burns; and in the case of water, by raising the temperature, an ice cube melts.” However, in most cases, answers did not reflect a clear understanding of concepts. For example, (question 3) about the concept of self-organization, a representative explanation was the following one: “Self-organization is when you organize yourself.” This is, of course superficially true but lacks a valid, i.e., non-circular explanation.
However, post-intervention, most students were able to: (a) explain DST/SP concepts, and (b) provide concrete examples and link them to body movement experiences. For example: “A healthy person is in a physiologically stable state, but this state can be destabilized under stressing factors (e.g., getting mad at friends, parent’s divorce) and increase the susceptibility to disease, as another stable state.” The majority of students connected the concepts of phase transition, stability, and instability: “Phase transition is when you go from one stable state to another stable state: from a green forest to a burned forest, from solid water to liquid water.” In case of the concept of self-organization: “Nobody tells atoms what to do; they organize themselves,” showing somewhat important understanding that for something to be called self-organized, there should be no external agent imposing the pattern of organization on the system’s structure or behavior.
Also, for the phenomena of infection spread and forest fire, some students connected the previous knowledge of how other additional interaction constraints may change the ‘local interaction’, and consequently, the state of the system as a whole (human population or forest). For example, for infection spread, aside of the interpersonal distance, exposure to the sun and the levels of vitamin D may change the infection susceptibility. For the forest fires, aside from the tree-to-tree distance, some students mentioned the trees’ moisture level, which may reduce the inflammability of the tree. These are pertinent cases in which one testifies how the interplay of phenomenon (i.e., level)-dependent concepts, such as: ‘interpersonal physical distance’, ‘vitamin D’, ‘exposure to the sun’, or ‘inter-tree distance’ and ‘moisture’ in semantic relation with the general (i.e., level-independent) concepts such as ‘local interaction constraint’, ‘phase transition, may produce far transfer, analogy based, integration of seemingly unconnected phenomena.
5. General discussion
An eight weeks intervention teaching DST/SP concepts through embodied experiences enabled most 5th-grade students to (a) learn DST/SP concepts, (b) identify the general principles among physical, biological and sociological processes, and (c) enable far transfer between these academic subjects. Although most students developed an understanding of these basic concepts and showed abilities of far transfer, we need to be sure about the stability and depth of their understanding. Since students had no additional learning activities of DST concepts (e.g., homework) during the intervention period, all results were simply a product of their learning in-situ. Thus, it is likely that their understanding of the acquired concepts and analogies still needs to be improved, especially if we consider their age.
Embodied (transdisciplinary) learning may have potential advantages and disadvantages. A small difference in the emphasis of the concrete (i.e., embodied) experience vs. the association-conceptualization or the transfer phase may hamper the targeted concreteness fading (Fyfe et al., 2014) and result in its opposite effect – concreteness enhancement, as was the case with class B when learning the concept of ‘stability’. On the other hand, concreteness fading success may vary from subject to subject or phenomenon to phenomenon (Kokkonen et al., 2022) and/or vary due to age (Jaakkola and Veermans, 2018).
Concerning this, the findings that two dimensions of embodied learning settings are highly relevant for its success: (1) the degree of task integration; and (2) the degree of bodily engagement (Skulmowski and Rey, 2018). The task integration level increases continually, from incidental cue-based bodily effects to fully integrated bodily activities into the learning task. On the other hand, the level of bodily engagement increases as bodily actions continually transit from seated activities to the performance of whole-body movements and locomotion.
Our learning protocol’s characteristics (Hristovski et al., 2014) were: high bodily involvement and high task integration. By applying it, Almarcha et al. (2022) demonstrated the learnability of the general DST/SP concepts through embodied experiences in high school students aged 12–13 years. The findings of that and of the current study are in accordance with experimental results of other researchers which demonstrated that learning performance may be successfully molded by higher bodily involvement (e.g., Skulmowski and Rey, 2017), and by a higher level of task integration (Mavilidi et al., 2015). On the other hand, some authors have found that low bodily engagement and mere incidental cue-based bodily effects may not affect some learning performance measures (e.g., Alban and Kelley, 2013). However, Brucker et al. (2015) have found that low bodily involvement (e.g., sitting activities) may bring about high-performance gains for some tasks (e.g., in mathematics education: Abrahamson and Sanchez-García, 2016; Abrahamson et al., 2021; Shvarts and van Helden, 2021). On the other hand, although some authors (e.g., Johnson-Glenberg et al., 2016; Lindgren et al., 2016) have shown that a high bodily engagement may lead to higher learning performance, the possibility of a cognitive overload may minimize its effectiveness (Song et al., 2014; Ruiter et al., 2015). In general, our results of the intervention have revealed some subtleties of embodied transdisciplinary learning strategies, which point to the need of their further detailed investigation and careful implementation.
On a more prospective note, implementing the intervention proposed in this research is challenging for students and teachers, but its benefits may be tremendous for the entire school community. The school ceases to be where students go to learn, instead, it becomes an entire learning experience for establishing real-life connections. However, aside from the methodological issues discussed above, some challenges should be alleviated to implement the embodied transdisciplinary (SUMA) educational framework successfully. On the side of teachers, it may be challenging: (a) to promote the value of synthetic understanding of natural and societal phenomena under the same umbrella framework and hence, to motivate their accurate understanding of DST/SP general concepts, as well as the interconnectedness among them, and (b) to stimulate their imagination of creating embodied experiences for students that show as critical (Alberto et al., 2022). On the side of students, their personal constraints, such as age, cultural-value background and others, may play a crucial role. More important details that can enhance or hamper the ‘learnability’ of the DST/SP general concepts and the knowledge transfer among the academic disciplines must be researched before this educational framework can reveal its teaching potential. Future studies to compare the effectiveness of the teaching/learning strategies used in this study are warranted to use randomized controlled experimental designs, and a follow-up to verify the longer-term effects of the intervention. A particularly important line of research that directly stems from this research should be the age dependent ‘depth of learning’ of the general concepts as defined by the understanding of their interconnectedness and interdependence.
6. Conclusion
The study points to the potential of teaching and learning general DST/SP concepts through body movement experiences in elementary school, enhancing students’ abilities to integrate and transfer knowledge among phenomena studied in natural and social sciences. The proposed transdisciplinary embodied education may offer a truly integrative approach to STEAM teaching. Future work is needed to distillate the full potential of the approach and its possible shortcomings. In this intervention, based on the transdisciplinary embodied learning framework- SUMA, students of 5th grade in elementary school were able to learn the targeted DST/SP concepts and use them for far transfer among different phenomena thought in separate academic subjects. The concreteness fading was shown to be an effective embodied learning strategy for the intervention. However, a part of the results showed that the strategy, at this age, may be fragile and somewhat sensitive to the differences in the degree of abstractness of the learned concepts, as well as to the differences in emphasizing the content of the embodied experience phases (phase 1 and 2) vs. the content of those phases focused on the abstract conceptualization and transfer (phase 3 and 4). Considering the findings of this study, our previous research, and the above-mentioned caveats, we conclude that the embodied transdisciplinary learning framework – SUMA has a substantial integrative educational potential in the area of the STEAM framework.
Data availability statement
The original contributions presented in the study are included in the article/supplementary material, further inquiries can be directed to the corresponding author.
Ethics statement
The studies involving human participants were reviewed and approved by 07/2015/CEICEGC. Written informed consent to participate in this study was provided by the participants’ legal guardian/next of kin. The local ethic committee approved the study according to the Helsinki declaration.
Author contributions
MA and PV conducted the intervention, collected the data, and organized the database. PV performed the statistical analysis. All authors wrote the first draft of the manuscript, contributed to the conception and design of the study, manuscript revision, read, and approved the submitted version.
Funding
This work was supported by the National Institute of Physical Education of Catalonia (INEFC), Generalitat de Catalunya. MA was supported by the project “Towards an embodied and transdisciplinary education” granted by the Spanish government Ministerio de Educación y Formación Profesional (FPU19/0569).
Acknowledgments
The authors thank the students and teachers who have made this educational experience possible and Pedro Serra for the technical support.
Conflict of interest
The authors declare that the research was conducted in the absence of any commercial or financial relationships that could be construed as a potential conflict of interest.
Publisher’s note
All claims expressed in this article are solely those of the authors and do not necessarily represent those of their affiliated organizations, or those of the publisher, the editors and the reviewers. Any product that may be evaluated in this article, or claim that may be made by its manufacturer, is not guaranteed or endorsed by the publisher.
Footnotes
1. ^Complicated systems are defined as systems of simple components, each of which has a well-understood law of behavior and interaction with other elements (Grabowski and Strzalka, 2008).
2. ^https://suma.edu.mk/general-concepts/
4. ^In dynamical systems approach the state of health is always a continuous variable. It is never a predefined categorical variable (Figure 1). This continuum, however, may have stable and unstable regions. It is the instability region that naturally induces category-like stable states (health vs. illness or uninfected vs. infected, states). Stable states do not mean the system is in one set-point. It may deterministically oscillate or stochastically fluctuate widely around some average value, however due to the negative feedback of the healthy organism, it dwells inside a wide, but nonetheless limited region. What is crucial to understand, though, is that this stable state can become unstable due to a slowly changing set of constraints (e.g., accumulated stress, weakened immune system), and eventually for a small perturbation (increased pathogen presence), it may spontaneously (by a positive feedback) switch to another stable (diseased, e.g., infected) region. For example, in: Shi et al., 2020; or Su et al., 2022, it is the ratio between virus elimination capacity of the immune system and the received pathogen load that defines the stability- instability of the healthy (uninfected) state. The set of interacting constraints acting on the immune system response, though, can be very intricate as in Antonovsky’s model of salutogenesis (Antonovsky, 1979), or complex adaptive systems approach to health (Sturmberg et al., 2019).
References
Abrahamson, D., and Mechsner, F. (2022). Toward synergizing educational research and movement sciences: a dialogue on learning as developing perception for action. Educ. Psychol. Rev. 34, 1813–1842. doi: 10.1007/s10648-022-09668-3
Abrahamson, D., and Sanchez-García, R. (2016). Learning is moving in new ways: the ecological dynamics of mathematics education. J. Learn. Sci. 25, 203–239. doi: 10.1080/10508406.2016.1143370
Abrahamson, D., Tancredi, S., Chen, R., Flood, V., and Dutton, E. (2021). “Embodied design of digital resources for mathematics education: theory, methodology, and framework of a pedagogical research program” in Handbook of digital (curriculum) resources in mathematics education. eds. B. Pepin, G. Gueude, and J. Choppin (Berlin: Springer)
Adams, N. E. (2015). Bloom’s taxonomy of cognitive learning objectives. JMLA 103, 152–153. doi: 10.3163/1536-5050.103.3.010
Alban, M. W., and Kelley, C. M. (2013). Embodiment meets metamemory: weight as a cue for metacognitive judgments. J. Exp. Psychol. Learn. Mem. Cogn. 39, 1628–1634. doi: 10.1037/a0032420
Alberto, R., van Helden, G., and Bakker, A. (2022). Action-based embodied design for proportions: from the laboratory to the classroom. Implement. Replicat. Stud. Math. Educ. 2, 174–207. doi: 10.1016/j.ijcci.2021.100419
Almarcha, M. C., Martínez, P., Balagué, N., and Hristovski, R. (2022). Embodied transfer of knowledge using dynamic systems concepts in high school: a preliminary study. Hum. Mov. Sci. 84:102974. doi: 10.1016/j.humov.2022.102974
Anderson, L. W., and Krathwohl, D. R. (2001). A taxonomy for learning, teaching, and assessing: A revision of Bloom’s taxonomy of educational objectives. New York, NY: Longmans.
Baek, Y., Park, H., Kim, Y., Noh, S., Park, J. Y., Lee, J., et al. (2011). Steam education in Korea. J. Learn. Center. High. Educ. 11, 149–171.
Bautista, A., Toh, G. Z., Mancenido, Z., and Wong, J. (2018). Student-centered pedagogies in the Singapore music classroom: a case study on collaborative composition. Aust. J. Teach. Educ. 43, 1–25. doi: 10.14221/ajte.2018v43n11.1
Brucker, B., Ehlis, A. C., Häußinger, F. B., Fallgatter, A. J., and Gerjets, P. (2015). Watching corresponding gestures facilitates learning with animations by activating human mirror-neurons: an fNIRS study. Learn. Instr. 36, 27–37. doi: 10.1016/j.learninstruc.2014.11.003
Cañal, P., Criado, A. M., García-Carmona, A., and Muñoz, G. (2013). La enseñanza relativa al medio en las aulas españolas de Educación Infantil y Primaria: Concepciones didácticas y práctica docente. [the teaching related to the environment in the Spanish classrooms of early childhood and primary education: didactic conceptions and teaching practice]. Investigación en la Escuela 81, 21–42. doi: 10.12795/IE.2013.i81.02
Clapp, E. P., and Jiménez, R. L. (2016). Implementando STEAM en el aprendizaje centrado en el fabricante. [Implementing STEAM in manufacturer-centered learning]. Psicología de la Estética, la Creatividad y las Artes 10, 481–491. doi: 10.1037/aca0000066
Clary, R. M., and Wandersee, J. H. (2007). A mixed methods analysis of the effects of an integrative geobiological study of petrified wood in introductory college geology classrooms. J. Res. Sci. Teach. 44, 1011–1035. doi: 10.1002/tea.20178
Cone, T. P., Werner, H., and Cone, S. L. (2009). Interdisciplinary elementary physical education. Champaign, IL: Human Kinetics.
Dakers, J. R. (2006). Towards a philosophy for technology education. Defining technological literacy: Toward an epistemological framework. New York: Palgrave Macmillan.
Das, A., and Adams, J. (2019). “Critical transdisciplinary STEM: a critical numeracy approach to STEM praxis by urban environments and education research coven” in Critical, transdisciplinary and embodied approaches in STEM education. eds. P. Sengupta, M.-C. Shanahan, and B. Kim (Cambridge, MA: Springer), 291–306.
Dobovišek, A., Markovič, R., Brumen, M., and Fajmut, A. (2018). The maximum entropy production and maximum Shannon information entropy in enzyme kinetics. Physica A Stat. Mech. Appl. 496, 220–232. doi: 10.1016/j.physa.2017.12.111
Forrester, J. W. (1994). Learning through system dynamics as preparation for the 21st century. In Keynote Address for Systems Thinking and Dynamic Modelling Conference for K-12 Education.
Fyfe, E. R., McNeil, N. M., Son, J. Y., and Goldstone, R. L. (2014). Concreteness fading in mathematics and science instruction: a systematic review. Educ. Psychol. Rev. 26, 9–25. doi: 10.1007/s10648-014-9249-3
García-Carmona, A. (2020). STEAM, ¿una nueva distracción para la enseñanza de la ciencia? [STEAM, a new distraction for science education?] Ápice. Revista de Educación Científica 4, 35–50. doi: 10.17979/arec.2020.4.2.6533
Gibbs, P. (2015). “Transdisciplinarity as epistemology, ontology or principles of practical judgment” in Transdisciplinary professional learning and practice. ed. P. Gibbs (New York: Springer International Publishing), 151–164.
Goldstone, R. L. (2006). The complex systems see-change in education. J. Learn. Sci. 15, 35–43. doi: 10.1207/s15327809jls1501_5
Goldstone, R. L., and Son, J. Y. (2005). The transfer of scientific principles using concrete and idealized simulations. J. Learn. Sci. 14, 69–110. doi: 10.1207/s15327809jls1401_4
Goldstone, R. L., and Wilensky, U. (2008). Promoting transfer by grounding complex systems principles. J. Learn. Sci. 17, 465–516. doi: 10.1080/10508400802394898
Grabowski, F., and Strzalka, D. (2008). “Simple, complicated and complex systems—the brief introduction” in 2008 Conference on Human System Interactions (Krakow, Poland: IEEE), 570–573.
Gupta, A., Turpen, C., Philip, T., and Elby, A. (2019). “Narrative co-construction of stances towards engineers' work in socio-technical contexts” in Critical, transdisciplinary and embodied approaches in STEM education. eds. P. Sengupta, M.-C. Shanahan, and B. Kim (New York, NY: Springer), 251–272.
Herlofsky, W. J. (2011). Iconic thinking and the contact-induced transfer of linguistic material. Semblance Signification 10:19. doi: 10.1075/ill.10.02her
Hong, O. (2016). STEAM education in Korea: current policies and future directions. Asian Res. Policy 8, 92–102.
Hristovski, R. (2013). Synthetic thinking in (sports) science: the self-organization of the scientific language. Res. Phys. Educ. Sport Health 2, 27–34.
Hristovski, R., Aceski, A., Balague, N., Seifert, L., Tufekcievski, A., and Aguirre, C. (2017). Structure and dynamics of European sports science textual contents: analysis of ECSS abstracts (1996–2014). Eur. J. Sport Sci. 17, 19–29. doi: 10.1080/17461391.2016.1207709
Hristovski, R., Balagué, N., Almarcha, M. C., and Martínez, P. (2020). SUMA educational framework: the way to embodied knowledge transfer and disciplinary mobility. Res. Phys. Educ. Sport Health 9, 3–7. doi: 10.46733/PESH2092003h
Hristovski, R., Balagué, N., and Vázquez, P. (2014). “Experiential learning of unifying principles of science through physical activities” in Systems theory: Perspectives, applications and developments. ed. F. Miranda (New York: Nova Science), 37–48.
Hristovski, R., Balagué, N., and Vázquez, P. (2019). “Science as a social self-organizing extended cognitive system. Coherence and flexibility of scientific explanatory patterns” in Complexity applications in language and communication sciences. eds. À. Massip-Bonet, G. Bel-Enguix, and A. Bastardas-Boada, vol. 11. 1st ed (Cham: Springer), 17–30.
Hutto, D. D., Kirchhoff, M. D., and Abrahamson, D. (2015). The enactive roots of STEM: rethinking educational design in mathematics. Educ. Psychol. Rev. 27, 371–389. doi: 10.1007/s10648-015-9326-2
Jaakkola, T., and Veermans, K. (2018). Exploring the effects of concreteness fading across grades in elementary school science education. Instr. Sci. 46, 185–207. doi: 10.1007/s11251-017-9428-y
Jacobson, M. J. (2001). Problem solving, cognition, and complex systems: differences between experts and novices. Complexity 6, 41–49. doi: 10.1002/cplx.1027
Jacobson, M. J., and Wilensky, U. (2006). Complex systems in education: scientific and educational importance and implications for the learning sciences. J. Learn. Sci. 15, 11–34. doi: 10.1207/s15327809jls1501_4
Johnson-Glenberg, M. C., Megowan-Romanowicz, C., Birchfield, D. A., and Savio-Ramos, C. (2016). Effects of embodied learning and digital platform on the retention of physics content: centripetal force. Front. Psychol. 7:1819. doi: 10.3389/fpsyg.2016.01819
Kim, B., Rasporich, S., and Gupta, D. (2019). “Imagining the sustainable future through the construction of fantasy worlds” in Critical, transdisciplinary and embodied approaches in STEM education. eds. P. Sengupta, M.-C. Shanahan, and B. Kim (New York, NY: Springer), 61–82.
Kokkonen, T., Lichtenberger, A., and Schalk, L. (2022). Concreteness fading in learning secondary school physics concepts. Learn. Instr. 77:101524. doi: 10.1016/j.learninstruc.2021.101524
Kolb, D. A. (1984). Experiential learning: Experience as the source of learning and development. Hoboken, NJ: Prentice-Hall.
Krishnapriya, P., and Pitchaimani, M. (2017). Modeling and bifurcation analysis of a viral infection with time delay and immune impairment. Jpn. J. Ind. Appl. Math. 34, 99–139. doi: 10.1007/s13160-017-0240-5
Lage-Gómez, C., and Ros, G. (2021). Transdisciplinary integration and its implementation in primary education through two STEAM projects (La integración transdisciplinar y su aplicación en Educación Primaria a través de dos proyectos STEAM). J. Educ. Dev. 44, 801–837. doi: 10.1080/02103702.2021.1925474
Lam-Herrera, M., Ajkem Council, and Sengupta, P. (2019). “Decolonizing complexity education: a Mayan perspective” in Critical, transdisciplinary and embodied approaches in STEM education. eds. P. Sengupta, M.-C. Shanahan, and B. Kim (New York, NY: Springer), 329–350.
Li, W., and Chiang, F. K. (2019). “Preservice teachers' perceptions of STEAM education and attitudes toward STEAM disciplines and careers in China” in Critical, transdisciplinary and embodied approaches in STEM education. eds. P. Sengupta, M. C. Shanahan, and B. Kim (New York, NY: Springer), 83–100.
Lindgren, R., Tscholl, M., Wang, S., and Johnson, E. (2016). Enhancing learning and engagement through embodied interaction within a mixed reality simulation. Comput. Educ. 95, 174–187. doi: 10.1016/j.compedu.2016.01.001
Martínez, P., Hristovski, R., Vázquez, P., and Balagué, N. (2017). “Chasing in biological systems. A pedagogical example for learning general dynamical systems concepts” in Complex Systems in Sport International Congress. Linking theory and practice. eds. C. Torrents, P. Passos, and F. Cos (Lausanne: Frontiers Media), 130–133.
Mavilidi, M. F., Okely, A. D., Chandler, P., Cliff, D. P., and Paas, F. (2015). Effects of integrated physical exercises and gestures on preschool children’s foreign language vocabulary learning. Educ. Psychol. Rev. 27, 413–426. doi: 10.1007/s10648-015-9337-z
Momsen, J., Speth, E. B., Wyse, S., and Long, T. (2022). Using systems and systems thinking to unify biology education. CBE—Life Sci. Educ. 21:es3. doi: 10.1187/cbe.21-05-0118
Nathan, M. J., and Alibali, M. W. (2021). “An embodied theory of transfer of mathematical learning” in Transfer of learning: Progressive perspectives for mathematics education and related fields. eds. C. Hohensee and J. Lobato, 27–58. doi: 10.1007/978-3-030-65632-4_2
Radloff, J., and Guzey, S. (2016). Investigating preservice STEM teacher conceptions of STEM education. J. Sci. Educ. Technol. 25, 759–774. doi: 10.1007/s10956-016-9633-5
Resnick, M., and Wilensky, U. (1998). Diving into complexity: developing probabilistic decentralized thinking through role-playing activities. J. Learn. Sci. 7, 153–172. doi: 10.1207/s15327809jls0702_1
Ruiter, M., Loyens, S., and Paas, F. (2015). Watch your step children! Learning two-digit numbers through mirror-based observation of self-initiated body movements. Educ. Psychol. Rev. 27, 457–474. doi: 10.1007/s10648-015-9324-4
Schwartz-Bloom, R. D., Halpin, M. L., and Reiter, J. P. (2011). Teaching high school chemistry in the context of pharmacology helps both teachers and students learn. J. Chem. Educ. 88, 744–750. doi: 10.1021/ed100097y
Science Technology and Innovation Council. (2015). State of the nation 2014: Canada's science, technology and innovation system. Ottawa, Canada: Government of Canada (Science, Technology and Innovation Council).
Sengupta, P., M. C. Shanahan, & Kim, B. (2019). "Reimagining STEM education: critical, transdisciplinary, and embodied approaches. In: P. Sengupta, M. C. Shanahan, and B. V. Kim (eds) Critical, transdisciplinary and embodied approaches in STEM education. Advances in STEM education. Springer, Cham.
Shanahan, M. C., Carol-Ann Burke, L. E., and Francis, K. (2016). Using a boundary object perspective to reconsider the meaning of STEM in a Canadian context. Can. J. Sci. Math. Technol. Educ. 16, 129–139. doi: 10.1080/14926156.2016.1166296
Sharma, J., and Yarlagadda, P. K. (2018). Perspectives of `STEM education and policies' for the development of a skilled workforce in Australia and India. Int. J. Sci. Educ. 40, 1999–2022. doi: 10.1080/09500693.2018.1517239
Shi, S., Huang, J., Wen, J., and Ruan, S. (2020). Bifurcation analysis of a dynamical model for the innate immune response to initial pulmonary infections. Int. J. Bifurc. Chaos 30:2050252. doi: 10.1142/S0218127420502521
Shvarts, A., and van Helden, G.. (2021). Embodied design versus dynamic visualization: benefits for a far transfer problem solving in trigonometry. In Proceedings of the 15th International Conference of the Learning Sciences-ICLS 2021. International Society of the Learning Sciences.
Skulmowski, A., and Rey, G. D. (2017). Measuring cognitive load in embodied learning settings. Front. Psychol. 8:1191. doi: 10.3389/fpsyg.2017.01191
Skulmowski, A., and Rey, G. D. (2018). Embodied learning: introducing a taxonomy based on bodily engagement and task integration. Cogn. Res. 3, 1–10. doi: 10.1186/s41235-018-0092-9
Song, H. S., Pusic, M., Nick, M. W., Sarpel, U., Plass, J. L., and Kalet, A. L. (2014). The cognitive impact of interactive design features for learning complex materials in medical education. Comput. Educ. 71, 198–205. doi: 10.1016/j.compedu.2013.09.017
Spencer, S. L., Cappell, S. D., Tsai, F. C., Overton, K. W., Wang, C. L., and Meyer, T. (2013). The proliferation-quiescence decision is controlled by a bifurcation in CDK2 activity at mitotic exit. Cells 155, 369–383. doi: 10.1016/j.cell.2013.08.062
Spintzyk, K., Strehlke, F., Ohlberger, S., Gröben, B., and Wegner, C. (2016). An empirical study investigating interdisciplinary teaching of biology and physical education. Sci. Educ. 25, 35–42.
Stieff, M., and Wilensky, U. (2003). Connected chemistry—incorporating interactive simulations into the chemistry classroom. J. Sci. Educ. Technol. 12, 285–302. doi: 10.1023/A:1025085023936
Stock, P., and Burton, R. J. (2011). Defining terms for integrated (multi-intertrans-disciplinary) sustainability research. Sustainability 3, 1090–1113. doi: 10.3390/su3081090
Stolz, S. (2015). Embodied learning. Educ. Philos. Theory 47, 474–487. doi: 10.1080/00131857.2013.879694
Sturmberg, J. P., Picard, M., Aron, D. C., Bennett, J. M., Bircher, J., DeHaven, M. J., et al. (2019). Health and disease—emergent states resulting from adaptive social and biological network interactions. Front. Med. 6:59. doi: 10.3389/fmed.2019.00059
Su, J., Lu, M., and Huang, J. (2022). Bifurcations in a dynamical model of the innate immune system response to initial pulmonary infection. Qual. Theory Dyn. Syst. 21:41. doi: 10.1007/s12346-022-00573-2
Takeuchi, M. A., Sengupta, P., Shanahan, M.-C., Adams, J. D., and Hachem, M. (2020). Trans disciplinarity in STEM education: a critical review. Stud. Sci. Educ. 56, 213–253. doi: 10.1080/03057267.2020.1755802
Terrace, H. S. (1963). Errorless transfer of a discrimination across two continua. J. Exp. Anal. Behav. 6, 223–232. doi: 10.1901/jeab.1963.6-223
Trujillo, C. M., and Long, T. M. (2018). Document co-citation analysis to enhance transdisciplinary research. Sci. Adv. 4:e1701130. doi: 10.1126/sciadv.1701130
Wilensky, U., and Centola, D. (2007). Simulated evolution: facilitating students’ understanding of the multiple levels of fitness through multi-agent modeling. In Evolution challenges conference, Phoenix, AZ.
Wilensky, U., and Jacobson, M. J. (2015). “Complex systems and the learning sciences” in Cambridge handbook of the learning sciences. ed. K. Sawyer (New York, NY: Cambridge University Press), 319–338.
Wilensky, U., and Reisman, K. (2006). Thinking like a wolf, a sheep, or a firefly: learning biology through constructing and testing computational theories—an embodied modelling approach. Cogn. Instr. 24, 171–209. doi: 10.1207/s1532690xci2402_1
Wilensky, U., and Resnick, M. (1999). Thinking in levels: a dynamic systems approach to making sense of the world. J. Sci. Educ. Technol. 8, 3–19. doi: 10.1023/A:1009421303064
Wong, V., Dillon, J., and King, H. (2016). STEM in England: meanings and motivations in the policy arena. Int. J. Sci. Edu. 38, 2346–2366. doi: 10.1080/09500693.2016.1242818
Yakman, G. (2008). STΣ@M education: an overview of creating a model of integrative education. Pupils Attitudes Towards Technology 2008 Annual Proceedings. Netherlands.
Yakman, G., and Lee, H. (2012). Exploring the exemplary STEAM education in the US as a practical educational framework for Korea. J. Korean Elem. Sci. Educ. 32, 1072–1086. doi: 10.14697/jkase.2012.32.6.1072
Zamorano, T., García, Y., and Reyes, D. (2018). Educación Para el sujeto del siglo XXI: Principales características del enfoque STEAM desde la mirada educacional. [education for the subject of the 21st century: Main characteristics of the STEAM approach from an educational perspective]. Contextos Estudios de Humanidades y Ciencias Sociales 41.
Keywords: dynamic systems, concreteness fading, transfer of knowledge, analogy, knowledge integration, trans-disciplinarity, embodied learning, complex systems
Citation: Almarcha M, Vázquez P, Hristovski R and Balagué N (2023) Transdisciplinary embodied education in elementary school: a real integrative approach for the science, technology, engineering, arts, and mathematics teaching. Front. Educ. 8:1134823. doi: 10.3389/feduc.2023.1134823
Edited by:
André Bresges, University of Cologne, GermanyReviewed by:
Benedikt Heuckmann, University of Münster, GermanySebastian Becker, University of Cologne, Germany
Copyright © 2023 Almarcha, Vázquez, Hristovski and Balagué. This is an open-access article distributed under the terms of the Creative Commons Attribution License (CC BY). The use, distribution or reproduction in other forums is permitted, provided the original author(s) and the copyright owner(s) are credited and that the original publication in this journal is cited, in accordance with accepted academic practice. No use, distribution or reproduction is permitted which does not comply with these terms.
*Correspondence: Pablo Vázquez, cHZhenF1ZTZAeHRlYy5jYXQ=; Robert Hristovski, cm9iZXJ0X2hyaXN0b3Zza2lAeWFob28uY29t