- 1Science Office, Omaha Public Schools, Omaha, NE, United States
- 2Department of Educational Psychology, University of Nebraska-Lincoln, Lincoln, NE, United States
- 3Department of Biology, University of Nebraska at Omaha, Omaha, NE, United States
- 4STEM TRAIL Center, University of Nebraska at Omaha, Omaha, NE, United States
The Next Generation Science Standards (NGSS), amid recent shifts in science curriculum, call for students to learn science through the practices of scientists and engineers (science and engineering practices, or SEPs). SEPs, related to inquiry learning, are ways students learn science content by doing science. Students have varied experiences learning science and engineering practices, including exposure in the classroom, from media, and in science fairs. Using a qualitative, multiple case study design, we analyzed public school educators’ and middle and high school students’ (ages 12–18) interview transcripts about learning through the science and engineering practices. Findings demonstrate that students learn different aspects of science and engineering practices during both in school and out-of-school science learning. Several transcending themes emerged from our interview data leading to recommendations for educators. Specific science and engineering practices might be better leveraged to introduce students to scientific research, students saw themselves as scientists leading to development of science identity while learning through SEPs, the relevancy of their work drove student learning, and resiliency was important during many of their learning experiences.
Introduction
The Next Generation Science Standards (NGSS) are being implemented across educational institutions in the United States (National Research Council [NRC], 2012). Educators are continually challenged by pedagogical aspects of students “doing science” through the science and engineering practices (SEPs) (Duschl and Bybee, 2014; Table 1). While one of the strongest predictors for the pursuit of a career in STEM fields is the career of a student’s parents (Miller et al., 2018), many learners may not be exposed to the practices of scientists and/or engineers if they do not have a parent in a STEM field. In some instances, pop-culture media might be an initial or only opportunity for youth to see the process of “doing science” in action. As more and more states adopt NGSS style standards, those requirements warrant learning to take place through these experiences in our kindergarten through high school classrooms. Therefore, educators are tasked with quickly adapting to the question: how else might our young learners learn about doing science?
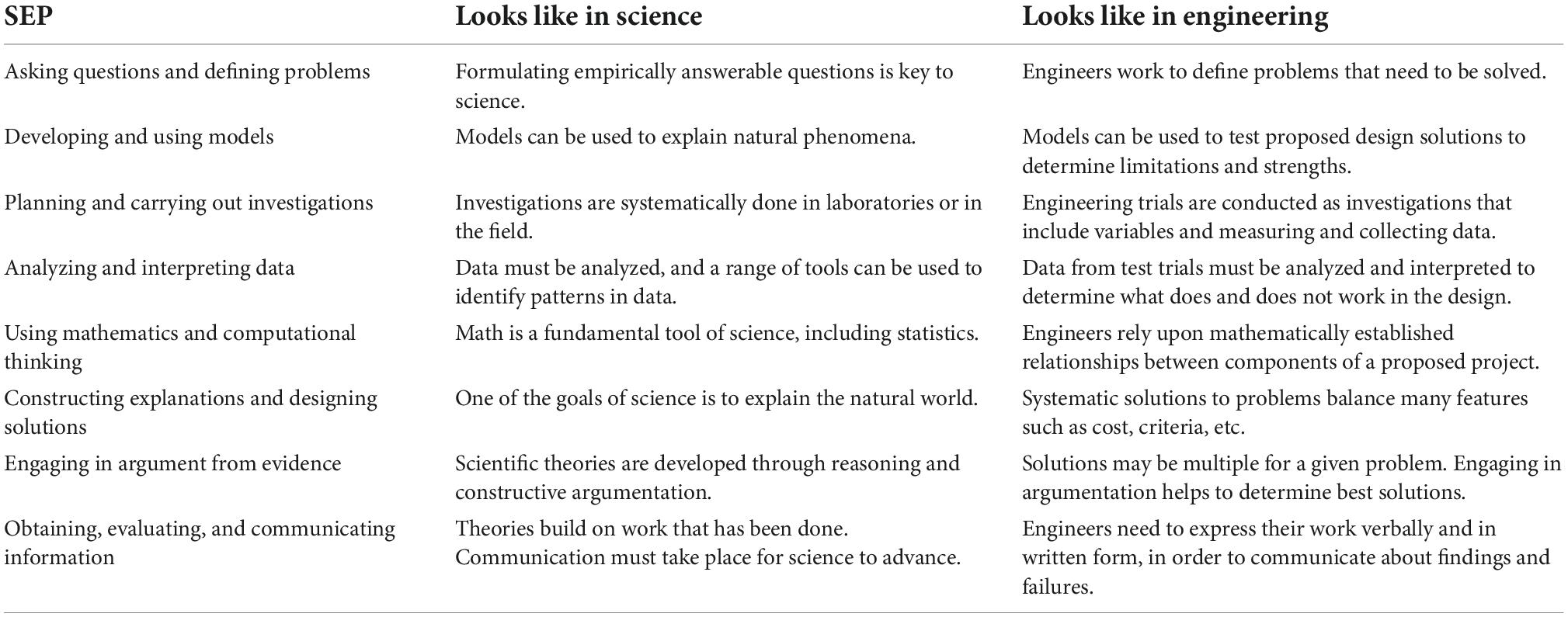
Table 1. The science and engineering practices (adapted from Figures 1 to 8, Bybee, 2011).
Updating science processes in the classroom: Adoption of Next Generation Science Standards
Recently, scientific inquiry shows a conflated relationship, and is oftentimes confused, with science and engineering practices. This conflation can be seen in publications from the American Association for the Advancement of Science [AAAS] (1993), as well as in work by Chinn and Malhotra (2002) who identify scientific inquiry as the practices of scientists. Crawford (2014) importantly adds that the methods of science differ among disciplines, but that inquiry might be more inclusive in its reliance on complex data analysis, and that it builds on scientific literature and principles. Bybee (2011) notes that changing from an inquiry model of science education to the more rigorous use of science and engineering practices is central to science education, which is further advocated for in the National Research Committee’s publication A Framework for K-12 Science Education: Practices, Crosscutting Concepts, Core Ideas “…students cannot fully understand scientific and engineering ideas without engaging in the practices of inquiry and the discourses by which such ideas are developed and refined” (National Research Council [NRC], 2012, p. 218).
The Framework emphasized the term “practices” to clarify and reshape what inquiry in science education looked like, with a focus on modeling and argumentation from evidence (Crawford, 2014). Students should be immersed in the practices to learn science concepts and to learn how to do the practices of scientists and engineers (Bybee and Van Scotter, 2006). The specific principles of the SEPs are captured in Table 1 (Bybee, 2011). The SEPs are expansive, and ever evolving given the nature of science and engineering, but the school day is still restricted to the same hours. Consequently, educators, administrators, and parents are seeking additional opportunities for youth to gain such skills in and out of the classroom.
To date, forty-four states have adopted NGSS or NGSS-like standards (National Science Teachers Assoication [NSTA], 2020), which include standards that are based on the three-dimensions of learning science: disciplinary core ideas, crosscutting concepts, and science and engineering practices. Guiding principles established by the National Research Council for SEPs include: student exposure to all eight practices during each predetermined grade band; practices are expectations for what students should experience while learning science concepts; all eight of the practices overlap with each other and build off of one another; aspects of each practice (not the entirety of the practice) are included in performance expectations; practices are based on progressing complexities; and success using these language-intensive SEPs relies on discourse in science (National Research Council [NRC], 2012). Considering the NRC’s guidelines of frequent student exposure to all the SEPs, it is important to understand the opportunities learners are afforded to engage with SEPs.
Teachers using science and engineering practices in the classroom
As noted, many states have adopted NGSS and NGSS-like standards, yet there are concerns about how three-dimensional learning, specifically SEPs, is enacted in schools. Teachers are likely purposeful in how they engage students in SEPs while delivering instruction based on NGSS and other three-dimensional standards. However, there are incongruencies between the perception of the teacher and the perception of classroom observers regarding how well SEP’s are being executed, and concerns about overall frequency of use. Malkawi and Rababah (2018) studied the frequency that Jordanian teachers of twelfth grade science asked learners to engage in each of the SEP’s. For this study teachers self-reported having used each SEP during instruction, although some were used more sparingly than others. Findings from this study indicated a need for more frequent student exposure to SEPs, particularly amid concerns of the Jordanian education system falling behind compared to science achievement measures of other countries. In a similar study, Kawasaki (2015) used ethnographic classroom notes, open-ended teacher interviews, and open-ended questionnaires from a situated perspective for seven teachers of middle and high school science in the United States. Teachers described carrying out more content instruction through SEPs than was deemed to have actually taken place in the classroom. Additionally, teachers conflated science and engineering practices with hands-on experiences such as traditional verification labs/experiments in the classroom. Even though teachers utilize SEPs via a variety of frameworks, and despite the inconsistencies in reporting the frequency of use and need for more SEPs in everyday science lessons, other opportunities exist for many youth to engage in SEPs.
Teachers using science and engineering practices in out-of-school-time science learning
Recent research has shown science fairs as effective ways for students to engage the SEPs (Koomen et al., 2018; DeLisi et al., 2021). Since Bybee (2011) states, “Science and engineering practices should be thought of as both learning outcomes and instructional strategies” (p. 39), we aimed to investigate some of the strategies that may further support students on their pathway to learning SEPs. Additionally, since 2014, states have been adopting the NGSS and while some states and districts have worked to be proactive with adoption, the preparation for teachers to adapt to yet another set of standards is less embedded.1 We sought to understand SEPs through the eyes of educators and of youth participating in research experiences within science.
Therefore, we aim to determine:
• What are students’ understandings about when and how they are engaging in science and engineering practices, both during science class and out-of-school time?
• How might students be learning content through the use of science and engineering practices?
Study context and theoretical framework
Study context
The data set utilized for this study was initially collected for a study on how middle and high school students (grades 6–12) experience science research for science fair in the United States (Andersson et al., 2021). Students from our sample might be considered “elite,” or at least very science-driven, as many of them performed at high levels both in science class and during their science fair experiences. During the analysis of that primary research question, we noticed an emergent pattern of SEPs, which led us back to the data set, to then use the SEPs as a lens through which to view the data set. This allowed us to analyze data in implicit ways (students were not specifically asked about SEPs) SEPs show up for students in these experiences, and how teachers and science fair sponsors view students’ research experiences.
Theoretical framework: Conceptual change theory
Inquiry approaches to science learning can be viewed through a combination of theories of learning: community of practice; sociocultural theory, and conceptual change theory (Crawford, 2014). Conceptual change theory notes that students have prior conceptual knowledge that is influenced by educational materials like curriculum, which can lead to alterations in conceptions (Strike and Posner, 1982). The sociocultural theory lens views student engagement with one another while using inquiry approaches in classroom contexts (Vygotsky, 1978). Finally, the community of practice framework acknowledges the dynamic interactions of students and teachers within and among overlapping communities such as social, school, and scientific (Lave and Wenger, 1991). For this study, the focus will be placed on the concept of community of practice, as we investigate if and where student interactions with science and engineering practices are taking place.
Research questions
Core research question
The central goal of this study is to understand how students experience science and engineering practices.
Research questions
The research questions for this study included:
1) How do students experience science and engineering practices both in school and in out-of-school time science learning?
2) How do educators (i.e., educators sponsoring the youth’s participation in a science fair) believe their students experience science and engineering practices in school and in out-of-school time learning?
Methodology
Qualitative research has been identified to have five traditional approaches: narrative, phenomenology, grounded theory, ethnography, or case study (Creswell and Poth, 2018). The approach of multiple-case study was selected for this work because it provides an in-depth understanding of the situation and contextual meaning for those involved (Saldaña, 2016; Yin, 2018). This study follows both youth (i.e., students with experiences in grades 6–12) and science educators (formal, classroom teachers) as they respond to questions about understanding in scientific processes and overlaying these responses to be in alignment (and to what degree) with the NGSS SEPs, see Figure 1. The researchers viewed the student participants as one case and the science educators as a second case.
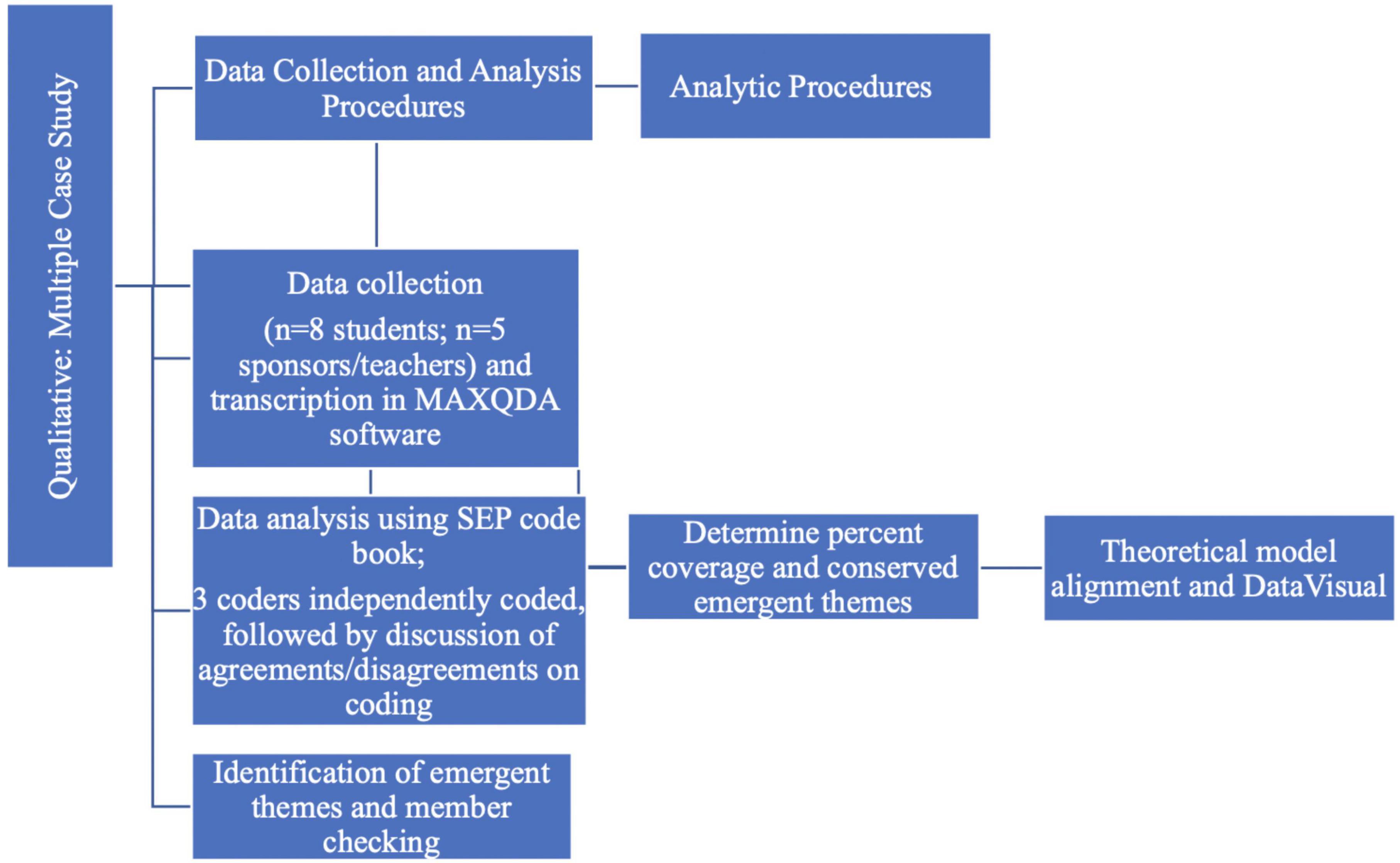
Figure 1. Procedures for methodology [adapted from Ivankova et al. (2006)].
Participants
The researchers employed criterion-based sampling (Creswell and Poth, 2018) as student participants all had experienced multiple years of science fair and phenomena-based, three-dimensional science instruction aligned to the Next Generation Science Standards. Further, all science fair sponsors (educators) also had multiple years of experience with leading students through science fairs, as well as their normal science teaching duties. The researchers gained access to participants through a regional science fair, with Institutional Review Board (IRB, University of Nebraska Lincoln) approval. Sponsors/science instructors were not necessarily responsible for the student participants who were interviewed for this study.
Participants were selected based upon their shared experiences of having at least two recent entrances (within three years) into an annual metropolitan area science and engineering fair that took place while they were in grades 6–12. Multiple entrances and recent engagement in science fair helped to ensure that study participants were close to their experiences and would have ample experiences from which to draw. Students with multiple experiences have an expanded worldview of conducting science research for science fair compared to participants who may have had a singular experience. Sampling methods included convenience sampling through partnership with a local science fair for the sampling pool, and maximum variation of the sample by selecting participants who varied across demographic markers. The science and engineering fair IRB approved our request for research participants and worked with us to contact participants. We obtained parental and participant informed consent per institutional IRB guidelines. Our final pool of student participants included: students who identified as male (2), and female (6); high school freshman (1), juniors (3), and college freshman (2) and sophomores (2); African American (4), Caucasian (2), Latinx (1), and Indian (1). Additionally, seven of the eight interviewees had participated at the state science fair level, five of whom presented at the American Junior Academy of Sciences in connection with AAAS. The participant pool also included five educators who had previously sponsored students to enter a science fair, or “sponsors.” More is included in the limitations section; however, this study is limited to the experiences of students who have been successful in science fair as an out of school time activity.
Data collection and analysis
Students and sponsors were interviewed about their science research experiences both in and out of school, but interview questions did not explicitly ask about science and engineering practices. We chose this data collection method to gain an implicit understanding of student experiences. Additionally, even though data was collected in a state that was in its third year of adoption of NGSS-like science standards, that include a focus on SEPs, it is unclear whether all participants have explicitly experienced these in the classroom, i.e., there are many challenges inherent to science-standards-implementation leading to dissimilarities in student experiences. So, our research aims are more exploratory in seeking to understand the contexts and conditions under which students may have had opportunities to use SEPs.
Data collection
Data was collected through semi-structured interviews, taking place via in-person meetings, telephone, and/or through Zoom. Thirteen total participants were each interviewed twice (eight students, five sponsors) for a total of 26 interviews. Each participant was interviewed by one of two researchers. First interviews focused on in-class science learning and second interviews focused on OST science learning and a comparison of both types, see Supplementary Appendix 1 for the Interview Guide. All recruitment, collection, and analysis were guided by local university IRB (# 20190619326EP-COLLA).
Data analysis
Interview audio was recorded, with permission from the participant, and then transcribed using automated transcription services such as Temi.com. Data was immediately de-identified and pseudonyms added, which are used throughout the results presented in this paper. Interview transcripts were checked for accuracy and then cleaned by the research team in MAXQDA.
The researchers developed a codebook based on an understanding of the SEPs (Schwarz et al., 2017) and used the Lexile search function of MAXQDA to identify initial segments of transcriptions aligned to each of the SEPs, see Table 2. Each transcript was then separately coded for each of the eight SEPs by two of the researchers, and then cross-checked across the research team for accuracy and for agreement. Discrepancies were discussed until an agreement was reached. For the final round of data analysis, and for congruency and to determine inter-rater reliability, three researchers completed side-by-side review with active discussions of codes and emerging themes through greater than 87% of interview transcripts. All SEPs were found across our study, being present in either or both student and sponsor coded transcriptions, Table 3 provides descriptive information about the numbers of coded segments.
Findings
The resulting data surrounding emergent themes utilizing the SEPs as a lens included subgroupings (1) SEP-specific emergent themes (i.e., by individual SEPs), see Table 4, and (2) Transcendent themes across SEPs, see Figure 2.
A key difference between in-class science learning vs. OST science learning, such as scientific research for science fair, is the depth of engagement in science as identified by the inquiry continuum (Biggers and Forbes, 2012). Classroom science learning, as prescribed by NGSS, likely gives students guided, more structured inquiry opportunities with learning focused on one or a few SEPs, without following through a complete cycle of scientific investigation. OST learning, as typified in research for science fair, includes engagement in an entire scientific research investigation, which may be more open inquiry than are classroom experiences. In other words, we may expect more thorough SEP experiences during OST science fair.
Science and engineering practice-specific emergent themes
All SEPs were represented in our entire dataset, albeit in varying amounts, see Table 3, with some SEPs (math and computational thinking, engaging in argument from evidence) being more thinly supported while others yielded very rich findings (asking questions and defining problems, planning, and carrying out investigations). Each SEP was not, however, found in each participant’s interview data, nor were all SEPs represented in both OST and in-school science learning. This led to some important nuances in the data and areas of future research focus, which will be further discussed. Sample quotes which support each theme are found in Table 4.
Asking questions and defining problems
Students had access to this SEP primarily through OST learning, but there was overlap with in-school experiences for some. Students and sponsors agree on the iterative nature of this SEP in practice, as the process of asking questions is more circular than it is linear. Relevancy was a theme across this SEP, as students who were able to develop their own questions were more engaged in the learning. In fact, some participants who developed research questions noted the broad implications of their research, how it fit into the research landscape, that is they “saw themselves among scientists.”
Sponsors noted that the current science curriculum can allow for students to explore open-ended questions. However, even though educators have license to provide learning opportunities for students to ask questions and define problems during in-school learning, students may not be exposed to this SEP early enough to develop student skills in asking investigable questions. Additionally, classroom contexts such as 30 students in a classroom for one 45-min segment create difficulties, as several sponsors identified. In this study, OST learning environments seem best positioned to allow students to access this SEP.
Due to the impact of this SEP on the learners interviewed for this study, we recommend that educators consider “Asking Questions and Defining Problems” as an access point for students to experience science learning through science research, both in-school and during OST. One method noted in our data suggests that more equitable outcomes might be achieved if students can discuss background content and work on question development in small groups, while focusing on what is relevant to the students and meaningful to the community.
Developing and using models
Students including Aliah, Sarah, and Damian, and sponsors such as Alissa, discussed the importance of using models toward learning gains. For example, Damian discussed the importance of developing models of scientific concepts to interpret phenomena, otherwise “you either get lost or you kinda just get bored with it” in your efforts to learn.
An important nuance to our interview data regarding Developing and Using Models is that the codes hailed almost entirely from in-class science learning. Our data suggest that students are most explicitly understanding this SEP when they are guided through aspects of it. There are implications here for the role of and need for mentors, whether they are classroom teachers or experts sponsoring students with their science-research endeavors.
Planning and carrying out investigations
Planning and carrying out investigations was implicated in both OST and in-school science learning. In the classroom, students largely noted the excitement for and effectiveness of learning when able to carry out investigations rather than learning from “reading from a textbook.” For one student participant, Sarah, learning how to plan and carry out experiments was key to how they developed confidence in doing science, which led to the student “seeing themselves among scientists.” Another student, Valarie, realized the iterative nature of this SEP, while “discovering new tools which changed the plan for data collection.” Relevance and autonomy were again important while students carried out their own investigations.
Mentoring was one of the key themes from our data analysis. Coded segments from several sponsor interviews identified the importance of mentoring with planning and carrying out investigations. From a mentor’s perspective, students benefit from guidance in understanding constraints of any given research, including variables and research methods. Student participants benefited from “dreaming big” about their ideal experiment, and then being supported in their exploration by sponsors, science coaches, and other mentors.
Findings from this SEP also brought out a negative case – Jared, a sponsor, saw school curriculum as the “dumbing down” of the process of science. OST requires students to develop this on their own, which is where many learners get “left behind.” For some student participants, the in-class science learning, while providing opportunity for student choice in how to set up an experiment, was mostly confirmatory science research – “what has already been established by other scientists and experts.” Perhaps some of the greatest potential for student gains in being able to effectively learn by doing science come across in access to this SEP in environments which are rich in mentoring, support, and opportunity for authentic investigation.
Analyzing and interpreting data
The majority of codes for Analyzing and Interpreting Data came through in sponsor interviews, and mostly during OST science learning. Sponsors noted the importance of mentoring, while students analyze and interpret data, which aligns with research findings from Chen et al. (2011). This can include students being guided through challenges associated with having null data. Further, sponsors noted that students get opportunities to collect and analyze data in both contexts (OST and in-school), but it is important for students to be able to analyze data they have collected, which is an access point for students to gain their science identity. Therefore, student ability to collect and analyze their own data might be a major contributor toward students “seeing themselves among scientists.”
Sarah discussed the interrelatedness of this SEP and Planning and Conducting Experiments, based on a lesson in Physics class, while another student noted the need for precision, and the time burden of data analysis. Although these findings are lightly represented in our data, they may nonetheless be important and require further investigation.
Using mathematics and computational thinking
Students likely have opportunities to use math and computational thinking when they’re conducting scientific research for OST science research but also during in-class learning. Yet, our findings yielded minor insights into this SEP. Both findings from sponsors showed students gained access to “doing science” and researching their interests came through math and computational thinking. For example, a student who enjoyed playing basketball became more interested in research when the sponsor and student started keeping track of shooting percentages from various points on a basketball court. To understand the implications of this SEP more fully, further research should explicitly investigate this SEP in both learning contexts, especially the potential of this SEP to be a gateway to doing science.
Constructing explanations and design solutions
Student participants experienced this SEP, both in-school and during OST science learning, according to sponsors and to student participants. Despite having relatively few coded segments compared to other SEPs such as Planning and Carrying Out Investigations, key findings came through in the interactive nature of constructing explanations such as adjusting research methods and data collection. Additionally, students expressed satisfaction in their ability to construct explanations from their data, as well as gaining confidence in explanations they were able to develop. Student feelings of success here may also play into them “seeing themselves among scientists.”
Engaging in argument from evidence
Our interview data yielded few coded segments for Engaging in Argument from Evidence, but once again this provided us with key insights. One sponsor, Doug, noted that students struggle to argue from their evidence when they gain inconclusive results, yet this may be critical for students to experience so they may more fully understand scientific research. Two student participants who experienced this SEP during OST learning, Marcus in an engineering project, and Andrea in a honeybee ecology project, discussed using not only the results of the experiment, but also the experimental design to effectively deliver their argument. While these two students found success with engaging in argument from evidence based on their experiences in science fair, there was a clear lack of responses from all students during science in school. This key finding is important as engaging in argument from evidence is central to the purpose of science (Bybee, 2011; Chen et al., 2011; National Research Council [NRC], 2012). A key implication here is for science educators to focus on this science practice more explicitly during instruction. Science education leaders should also look for opportunities to support teachers with appropriate curricula and professional learning experiences that include opportunities to practice engaging in argument from evidence.
Obtaining, evaluating, and communicating evidence
Nearly all our research participants experienced this SEP both in-school and during OST learning. The key theme was the potential for students to build confidence in their ability to effectively communicate science, both during in-class presentations of their work (OST or in-school), and, for example, presenting their research at science fairs. There was another key interplay between in-school and OST learning here, in that several students spoke to various methods of obtaining information they needed for class projects or for OST research. Students used sources such as internet searches, classroom lessons, reading texts and research articles, seeking professionals, and technology and science departments across the school to inform their coursework and their OST research. In other words, knowledge students gained from one context was not solely used in that context.
Transcending themes (themes found across science and engineering practices)
From the perspective of educational researchers who are also educators, one of the most rewarding aspects of this research was the emergence of themes that transcended SEPs. These themes not only support prescriptive uses of students doing scientific research, but also demonstrate the potential for powerful learning outcomes, both in and out of the classroom.
Introduction into scientific research
Findings suggest that some SEPs might be leveraged to introduce students to the processes of scientific investigation. Some of our participants did not embark on doing scientific research, rather they were drawn in by their exposure to one of the SEPs, typically during OST science learning. For example, a sponsor, Leslie, used a student’s interest in mathematics to get the student started on a science research project, through the mathematics and computational thinking SEP. Jared, also a sponsor, reported the benefits of jumping into learning sequences with more “guided inquiry,” using the SEP collecting and analyzing data for students to do their own collection and analysis, further giving the students an entry point into the SEPs and consequently into scientific research.
Our findings highlighted mathematics and computational thinking, analyzing and interpreting data, and especially asking questions and defining problems as entry points for the participants of this study. However, we postulate that any SEP which aligns with the interests and skills of a student might be used to introduce that student to scientific research. For example, a student who may enjoy being on the stage might be drawn in by obtaining, evaluating, and communicating information, or even engaging in argument from evidence. Sponsors we interviewed demonstrated skill in their ability to identify the strengths and interests of their students and then orient specific SEPs toward those strengths and interests, thereby opening doors for students which may not otherwise have been opened.
Finding yourself among scientists
Research participants experienced important breakthroughs during their OST science learning. These were points where students felt accomplished in the product of their learning, especially during the SEPs using mathematics and computational thinking, planning, and carrying out investigations, and analyzing and interpreting data. Students felt like they were “doing real science,” realizing they had completed processes which professional scientists and engineers do. Further, some students reported that they felt comfortable asking questions and discussing research with scientists. We believe this theme interplays with student science identity (Starr et al., 2020). This finding warrants further investigation to understand this relationship more fully.
Resilience
The research participants, both the students and mentors, reported learning about the role of failure in science and engineering. Both reported moving from a place of avoiding failure at all costs to embracing failure within their time of doing science and employing the science and engineering practices. These findings align with what is known about resilience and conceptual change, specifically related to anomalous data (Chinn and Brewer, 1993). This transcending theme is interconnected with other transcending themes, considering that students reported gaining confidence in their abilities which led to students finding themselves among scientists.
Relevancy of the work
Opportunities for students to explore problems that need to be solved, which are relevant to themselves, meaningful to the community are important hooks to gaining student involvement. This theme coincides with “introduction into scientific research” in that when students are able to ask their own questions, they typically ask questions which are meaningful to themselves. Relevancy of the work is what initially draws many students toward scientific investigation, so this is a catalyst for students to be exposed to any and hopefully all the SEPs.
While some sponsors, and likely many other educators, note that opportunities for students to investigate personally relevant topics are often confounded by the rigidity of standards and curricula, we feel it is still critical to highlight our findings here. When learning was relevant to students, they were more engaged in the learning activities. The OST science research experiences of our participants seemed to afford such opportunities, and in several cases student learning from their OST science activities enhanced their classroom learning.
Discussion
This study adds to the research on how students experience science and engineering practices. Specifically, we examined how students reported experiencing science and engineering practices during in-school science learning and OST science learning, i.e., science fair. The researchers also utilized interview data from educators who sponsored students engaged in science fairs. We realized that students often initially lack confidence in completing science research, and that this can be abated by having an educator, or other mentor, interact with the students as they progress through the practices. Increasing the students’ confidence leads to students seeing themselves among scientists. From the mentor’s data we also learned there may be a personal cost to students if their inquiry questions are not legitimized. So, a student’s questions, no matter how far-fetched, are a doorway to the student’s thinking. Further, if a mentor shuts down the question, doors to student thinking and doing science may be effectively closed, discouraging their participation in science.
The students also reported the importance of mentors. Although we had one student that reported seeking success to prove her science research abilities to her teacher/mentor, all the other students reported that the sponsors had a massive role for the students. Specifically, students found that what they learned while engaging in science and engineering practices translated to other learning experiences. The students who had already progressed into college reported their confidence to lead small groups with projects in college. They also reported feeling more prepared to enter the workforce even if they were not focused on science as a career. For example, they were able to find use in the lessons learned in talking with adults and presenting findings to judges.
Embedding science and engineering practices into student activities inside and outside the traditional classroom
Science and engineering practices, as part of the Next Generation Science Standards, have a history embedded in inquiry approaches to science learning. Research indicating the benefits of this approach to science learning is abundant (McGee-Brown et al., 2003; National Research Council [NRC], 2012; Marshall and Alston, 2014; Osborne, 2014). Additionally, and of essential importance are the implications that inquiry learning and learning through the use of SEPs can benefit the diverse learners that are present in our classrooms (Rahm and Moore, 2016). In other words, students should have access to this type of learning, in various settings.
Learners might engage with SEPs in their science classroom, or through more informal science-learning experiences such as OST. In the classroom, educators (pre-service and in-service) might not have the pedagogical or content background necessary to implement the necessary shifts, that is, students learning content through the use of SEP’s. Research has found that professional development can help provide more of these learning opportunities in the classroom for our science learners (Banilower et al., 2018). Curricular projects are available that can be scaled to district needs, including teacher preparation. Outside of the classroom, students may have access to SEP’s through activities such as science club and preparation for science competitions, which have been shown to provide excellent opportunities for those who can participate. An additional challenge to both in-school and OST science learning is understanding the epistemic and conceptual aspects of science and engineering practices, including the understanding of the nature of science. Finally, assessment systems might be a critical factor in the amount of resources that districts, schools, and teachers put into the vision of learning science content through the use of science and engineering practices.
There remains a gap in our understanding of how the science and engineering practices are being implemented in the classroom and potential inequities in this. There are also gaps in the student perspective of exposure to SEPs through informal, unstructured learning opportunities. Research on how students experience this most recent attempt at inquiry learning could yield great benefits toward many levels of education: lesson, curriculum, standards, and equity within science education.
Are some students more aligned to understanding and interpreting science and engineering practices, regardless of when or how presented?
During data analysis, it became clear that some students were more effective than others at articulating their scientific research experiences in the classroom or OST, be that visiting a laboratory of an independent scientist, or by working directly with their teacher. Consequently, we investigated any alignment by type of experience. For example, if a student articulated very specific and explicit acknowledgment and understanding of SEPs, did they cluster by a particular type of experience that could be modeled more in the future? Unfortunately, these data did not cluster to be by shared types of activities and were instead randomly dispersed.
Limitations
Classroom science learning experiences as described by our participants are just a snapshot of their entire learning experience, which may include learning progressions through all SEPs, and science disciplines as prescribed by NGSS. Our data do not yield an entire understanding of this learning, but just an indication of how some higher-level learners have experienced science and engineering practices in the classroom. Additionally, although student participants in this study hailed from various racial, ethnic, and gender identities, our sample was homogenous in that our participants typically excelled at school and sought out, or were sought out by educators for, involvement in OST science learning. Because we intentionally included students with multiple experiences in science fairs, our data set is limited to students who likely had “resource-rich” backgrounds (Bencze and Bowen, 2009). Finally, every research method has its limitations, as is the case with our qualitative approach here. While we gained deep insight toward our research questions, the findings will be difficult to generalize to broader/different populations than what we investigated here. However, we contend that our findings provide a rich description that contributes to our understanding of how middle and high school students may experience SEPs.
Data availability statement
The original contributions presented in this study are included in the article/Supplementary material, further inquiries can be directed to the corresponding author.
Ethics statement
The studies involving human participants were reviewed and approved by the University of Nebraska-Lincoln Institutional Review Board. Written informed consent to participate in this study was provided by the participants’ legal guardian/next of kin.
Author contributions
CS and JA designed the study and collected the data. CS, JA, and CC analyzed the data and contributed to the manuscript. All authors contributed to the article and approved the submitted version.
Funding
This work was supported by the grant from NSF NoyceSCIENCE #1659058.
Acknowledgments
We thank the study participants for the time they gave us in interviews and member-checks. The researchers also acknowledge Hayley Jurek for assisting with the graphics created. Finally, we also acknowledge Eric Buhs and Nealy Grandgenette for their assistance with the initial data collection and for their continued support.
Conflict of interest
The authors declare that the research was conducted in the absence of any commercial or financial relationships that could be construed as a potential conflict of interest.
Publisher’s note
All claims expressed in this article are solely those of the authors and do not necessarily represent those of their affiliated organizations, or those of the publisher, the editors and the reviewers. Any product that may be evaluated in this article, or claim that may be made by its manufacturer, is not guaranteed or endorsed by the publisher.
Supplementary material
The Supplementary Material for this article can be found online at: https://www.frontiersin.org/articles/10.3389/feduc.2022.960346/full#supplementary-material
Footnotes
References
American Association for the Advancement of Science [AAAS] (1993). Project 2061 Atlas of Scientific Literacy. Washington, DC: AAAS.
Andersson, J., Schaben, C., Buhs, E., and Grandgenett, N. (2021). Phenomenology of secondary students’ experiences in out-of-school time science research. Sci. Educ. 28, 30–39.
Banilower, E. R., Smith, P. S., Malzahn, K. A., Plumley, C. L., Gordon, E. M., and Hayes, M. L. (2018). Report of the 2018 NSSME+. Chapel Hill, NC: Horizon Research Inc.
Bencze, J. L., and Bowen, G. M. (2009). A national science fair: exhibiting support for the knowledge economy. Int. J. Sci. Educ. 31, 2459–2483. doi: 10.1080/09500690802398127
Biggers, M., and Forbes, C. T. (2012). Balancing teacher and student roles in elementary classrooms: preservice elementary teachers’ learning about the inquiry continuum. Int. J. Sci. Educ. 34, 2205–2229. doi: 10.1080/09500693.2012.694146
Bybee, R. W. (2011). Scientific and engineering practices in K-12 classrooms: understanding “a framework for K-12 science education”. Sci. Teach. 78, 4–40. doi: 10.1186/s41235-020-00222-9
Bybee, R. W., and Van Scotter, P. (2006). Reinventing the science curriculum. Educ. Leadership 64, 43–47.
Chen, J. J., Lin, H. S., Hsu, Y. S., and Lee, H. (2011). Data and claim: the refinement of science fair work through argumentation. Int. J. Sci. Educ. Part B 1, 147–164.
Chinn, C., and Malhotra, B. (2002). Epistemologically authentic inquiry in schools: a theoretical framework for evaluating inquiry tasks. Sci. Educ. 86, 175–218.
Chinn, C. A., and Brewer, W. F. (1993). The role of anomalous data in knowledge acquisition: a theoretical framework and implications for science education. Rev. Educ. Res. 63, 1–49. doi: 10.3102/00346543063001001
Crawford, B. (2014). “Scientific Practices and Inquiry in the Science Classroom,” in Handbook of Research on Science Education, eds N. G. Lederman and S. K. Abell (New York, NY: Routledge).
Creswell, J., and Poth, C. (2018). Qualitative Inquiry and Research Design: Choosing Among Five Approaches, 4th Edn. Thousand Oaks, CA: Sage.
DeLisi, J., Kook, J. F., Levy, A. J., Fields, E., and Winfiled, L. (2021). An examination of the features of science fairs that support students’ understanding of science and engineering practices. J. Res. Sci. Teach. 58, 491–519. doi: 10.1002/tea.21669
Duschl, R. A., and Bybee, R. W. (2014). Planning and carrying out investigations: an entry to learning and to teacher professional development around NGSS science and engineering practices. Int. J. STEM Educ. 1, 1–9. doi: 10.1186/s40594-014-0012-6
Ivankova, N. V., Creswell, J. W., and Stick, S. L. (2006). Using mixed-methods sequential explanatory design: from theory to practice. Field Methods 18, 3–20. doi: 10.1177/1525822X05282260
Kawasaki, J. N. (2015). Examining Teachers’ Goals and Classroom Instruction Around the Science and Engineering Practices in the Next Generation Science Standards. Los Angeles, CA: UCLA.
Koomen, M. H., Rodriquez, E., Hoffman, A., Petersen, C., and Oberhauser, K. (2018). Authentic science with citizen science and student-driven science fair projects. Sci. Educ. 102, 593–644. doi: 10.1002/sce.21335
Lave, J., and Wenger, E. (1991). Situated Learning: Legitimate Peripheral Participation. New York, NY: Cambridge University Press.
Malkawi, A. R., and Rababah, E. Q. (2018). Jordanian twelfth-grade science teachers’ self-reported usage of science and engineering practices in the next generation science standards. Int. J. Sci. Educ. 40, 961–976.
Marshall, J. C., and Alston, D. M. (2014). Effective, sustained inquiry-based instruction promotes higher science proficiency among all groups: a 5-Year analysis. J. Sci. Teach. Educ. 25, 807–821.
McGee-Brown, M., Martin, C., Monsaas, J., and Stombler, M. (2003). “What scientists do: science olympiad enhancing science inquiry through student collaboration, problem solving, and creativity,” in Proceedings of the Annual National Science Teachers Association meeting, (Philadelphia, PA).
Miller, K., Sonnert, G., and Sadler, P. (2018). The influence of students’ participation in STEM competitions on their interest in STEM careers. Int. J. Sci. Educ. Part B: Commun. Public Engagement 8, 95–114. doi: 10.1371/journal.pone.0108497
National Research Council [NRC] (2012). A Framework for K-12 Science Education: Practices, Crosscutting Concepts, and Core Ideas. Uttar Pradesh: The National Academy of the Sciences.
National Science Teachers Assoication [NSTA] (2020). Compare NGSS to existing state standards2. NGSS@NSTA. Available online at: https://ngss.nsta.org/compare-the-standards.aspx (accessed November 20, 2022).
Osborne, J. (2014). “Scientific practices and inquiry in the science classroom,” in Handbook of Research on Science Education, eds N. G. Lederman and S. K. Abell (New York, NY: Routledge).
Rahm, J., and Moore, J. C. (2016). A case study of long-term engagement and identity-in-practice: insights into the STEM pathways of four underrepresented youths. J. Res. Sci. Teach. 53, 768–801.
Saldaña, J. (2016). The Coding Manual for Qualitative Researchers. Los Angeles, CA: SAGE Publications.
Schwarz, C., Passmore, C., and Reiser, B. J. (eds) (2017). Helping Students Make Sense of the World Using Next Generation Science and Engineering Practices. Arlington, VA: NSTA Press.
Starr, C. R., Hunter, L., Dunkin, R., Honig, S., Palomino, R., and Leaper, C. (2020). Engaging in science practices in classrooms predicts increases in undergraduates’ STEM motivation, identity, and achievement: a short-term longitudinal study. J. Res. Sci. Teach. 57, 1093–1118.
Strike, K., and Posner, G. (1982). Conceptual change and science teaching. Eur. J. Sci. Educ. 4, 231–240. doi: 10.1080/0140528820040302
Vygotsky, L. (1978). Mind in Society: The Development of Higher Mental Processes. Cambridge, MA: Harvard University.
Keywords: out-of-school time, NGSS, science fair, science and engineering practices (SEPs), multiple case study, science fair mentor
Citation: Schaben C, Andersson J and Cutucache C (2022) A multiple case study to understand how students experience science and engineering practices. Front. Educ. 7:960346. doi: 10.3389/feduc.2022.960346
Received: 02 June 2022; Accepted: 11 November 2022;
Published: 01 December 2022.
Edited by:
Gudberg K. Jonsson, University of Iceland, IcelandReviewed by:
G. Michael Bowen, Mount Saint Vincent University, CanadaSimon Dalley, Southern Methodist University, United States
Copyright © 2022 Schaben, Andersson and Cutucache. This is an open-access article distributed under the terms of the Creative Commons Attribution License (CC BY). The use, distribution or reproduction in other forums is permitted, provided the original author(s) and the copyright owner(s) are credited and that the original publication in this journal is cited, in accordance with accepted academic practice. No use, distribution or reproduction is permitted which does not comply with these terms.
*Correspondence: Chris Schaben, Y2hyaXMuc2NoYWJlbkBvcHMub3Jn