- Faculty of Education, Kagoshima University, Kagoshima, Japan
Visuospatial thinking in science education is an important form of thinking that involves the purposeful use of the human eyes to develop an internal representation. This study examined the visuospatial thinking skills of primary school students with two aims (1) identifying students’ cognitive levels of these skills in domain-general learning, and (2) discovering how primary school students respond to visuospatial tasks that require interpretation of a diagrammatic representation. The study also investigated whether there are differences in how male and female students answer visuospatial thinking tasks. The participants included 93 fourth-grade students (8–9 years old), including 51 male and 42 female students, from a public primary school in Japan. The participants completed two types of paper-pencil tests. The first test required participants to complete the Wide-range Assessment of Vision-related Essential Skills (WAVES), a domain-general test that measures visual perception and eye-hand coordination skills. In the second test, students answered questions about the relationship between the movement of the sun and the behaviors of solar cells located in different places by interpreting a diagrammatic representation. Female students outperformed male students in one of the four WAVES index scores; otherwise, no other statistically significant differences were found. A small number of students had low visuospatial perception scores. When students were asked to explain their reasoning regarding how the solar cells worked based on their interpterion of the diagram, only a few answered correctly using perspective-taking and/or visualizing. Other students struggled to provide their reasoning, even if they had factual knowledge. Some students held an alternative conception of sunlight intensity and the sun’s path in the sky. They worked through the problem from their alternative conceptions without reference to visuospatial information or taking different perspectives from the diagram. No statistically significant differences were found in the relationship between achievement in the domain-general test and the number of correct answers in the domain-specific test. The study’s findings imply that students should be encouraged to practice visuospatial thinking to overcome previously held alternative conceptions. Furthermore, science education should emphasize the concept of space and teach conventional knowledge on different representation types. Further research on students’ learning progress in visuospatial thinking that includes alternative conceptions such as the students’ domain-specific knowledge is recommended.
Introduction
From a sociocultural perspective, science is seen as a practice of constructing different representation types (Latour, 1987; Roth and Tobin, 1997; Roth and McGinn, 1998). These types include external representations, which are constructed outside a person through language, diagrams, equations, materials, and so on, and internal representations, which are constructed inside a person through mental operations (Pande and Chandrasekharan, 2017). External representations have mobility, meaning they are easily transferred by their creators and users. In addition, they have immutability; that is, their properties do not change when they are transferred. They also have scalability because they can be easily re-scaled without changing their internal relationships. Moreover, they have reproducibility and can be reproduced at lower cognitive, temporal, and economic costs. Finally, external representations are characterized by combinability, as they can be easily combined with other representations (Roth and McGinn, 1998). Internal representations, in contrast, are formed through the interpretation and communication of external representations in mental processes. Thus, the two types of representation are interrelated.
This idea that multiple representation forms are used in learning is most commonly associated with science and science education. Research shows that enhancing students’ representational competence through science learning is becoming increasingly important (diSessa, 2004; Gilbert, 2008; Pande and Chandrasekharan, 2017; Daniel, 2018). Representational competence in science education is defined as the students’ ability to combine procedural and epistemic knowledge with content knowledge through representational practices (diSessa, 2004; Novick, 2006; Tippett, 2016; Daniel, 2018). In representational practices, students are required to move from one level of representation to another, such as transitioning from the macro-level to the microscopic level, or from some dimension of representation to another. For example, students can imagine a chemical structure, which is three-dimensional (3D), by interpreting two-dimensional (2D) drawings (Gilbert, 2008).
The cognitive process of transformation from 2D to 3D representation, or from external representation to internal representation, is a key function of visuospatial thinking. Visuospatial thinking or visuospatial reasoning is a form of thinking that involves the purposeful use of human eyes to develop an internal representation. It is characterized by both logical and creative processing of internal representations to solve problems, create new ideas, and improve skills (Mathewson, 1999; Shah and Miyake, 2005; Tversky, 2005; National Research Council, 2006; Ramadas, 2009; McCormack, 2017). Visuospatial thinking is based on a constructive amalgam of three elements: concepts of space, tools of representation, and processes of reasoning (National Research Council, 2006). Concepts of space include ideas related to dimensionality, continuity, proximity, and separation. Tools of representation consist of a variety of modes and media to describe, explain, and communicate the structure, operation, and function of objects and their relationships. Processes of reasoning are different thinking skills that use representation tools. Visuospatial thinking is a common cognitive process of humans, pervading everything from our everyday lives to expert practices in science, art, and other professional fields. However, each field has distinct visuospatial thinking practices (Shah and Miyake, 2005; Tversky, 2005; National Research Council, 2006).
In science education, McCormack’s taxonomy of visuospatial thinking skills is well known (McCormack, 2017). This taxonomy has four components: visual-spatial perception, visual-spatial memory, logical visual-spatial thinking, and creative visual-spatial thinking. This taxonomy has a pyramid structure that is layered from visuospatial perception to creative visuospatial thinking. Visual-spatial perception includes the elementary physiological ability to observe objects and construct internal representations as mental images. Differentiating between figure and ground in complex drawings or photographs is an operation of visuospatial perception. Mathewson (1999) refers to this skill as a vision–defined as the ability to use one’s eyes to identify, locate, and think about objects and orient oneself in the world. Visual-spatial memory comprises the ability to mentally store representations and retrieve them later, and the ability to communicate descriptions of images through drawings and language. This category includes different types of skills, such as perspective-taking, which is the ability to visualize objects as observed from different points of view, and mental rotations, which is the ability to rotate 2D or 3D objects mentally. Logical visual-spatial thinking consists of operations involving internal representations, where the operations are based on a set of rules as well as analytical and convergent thinking. Most of these operations involve logical reasoning processes. Creative visual-spatial thinking involves the production of rare, unique, or original internal representations. These can include representations produced in the realms of fantasy, invention, design, aesthetics, humor, and metaphor.
Some researchers have argued that visuospatial thinking has been overlooked by science educators (Mathewson, 1999) and that the nature of visuospatial thinking is misunderstood or has not been clarified (Newcombe and Stieff, 2012). Other researchers have reported that the development of visuospatial thinking skills impacts the ability to learn Science, Technology, Engineering, and Mathematics (STEM) subjects (Wai et al., 2009; Uttal and Cohen, 2012; Khine, 2017; Wai and Kell, 2017; Sorby et al., 2018). Wai et al. (2009) suggested that first, visuospatial thinking ability is a salient psychological characteristic among students who subsequently go on to achieve advanced educational and occupational credentials in STEM. Second, visuospatial thinking ability plays a critical role in structuring educational and occupational outcomes in the general population as well as among intellectually talented individuals. Third, measurement of visuospatial thinking should be available as a selection criterion for identifying intellectually talented students. Gagnier et al. (2022) reported that primary school teachers in the United States tended to believe that spatial thinking is more important for older students, though there were differences based on years of teaching experience. Experienced teachers were more likely to believe that spatial thinking is important at all ages, particularly in the younger grades. Moreover, students who have developmental or social problems related to visuospatial thinking may face challenges in science learning. It is well known that children with disabilities related to visuospatial perception have learning disabilities in reading, spelling, arithmetic, and other subjects (Groffman, 2006). In science learning, for example, chemistry students with lower-level visuospatial abilities are unable to perform as well as their peers with higher visuospatial abilities in solving both spatial and non-spatial chemistry problems, and they have more difficulty moving and transforming from one representation to other representations (Wu and Shah, 2004). Overall, male students tend to outperform female students on visuospatial tasks (Uttal et al., 2013; McCormack, 2017).
In a broader context, gender differences were reviewed in a variety of tests on visuospatial abilities through standardized paper-and-pencil or computerized tasks and in different fields (Halpern and Collaer, 2005). Gender differences in visuospatial abilities have been argued from three perspectives: the evolutionary perspective, the biological perspective, and the learning and experience perspective. From the evolutionary perspective, gender differences in visuospatial abilities are explained as reflections on the development of neuroarchitecture through the division of labor in hunter-gatherer societies where men traveled long distances to hunt animals and women gathered food closer to their home base. From the biological perspective, gender differences related to visuospatial thinking skills were explored by focusing on the “what” (ventral) and “where” (dorsal) visual pathways in the human brain, hemispheric lateralization, and exposure to gender steroid hormones. However, these differences are not fixed or immutable, but rather may be influenced by environmental and educational factors. From the learning and experience perspective, how gender differences can be developed and improved were investigated depending on the quality and quantity of training and experience in visuospatial thinking skills. Researchers have reported that visuospatial thinking skills are highly malleable; visuospatial thinking skills development and training is effective, durable, and transferable. This is one of the many promising avenues for increasing student success in STEM fields (Uttal et al., 2013; Stieff and Uttal, 2015; Cheng, 2017). Different trainings for each visuospatial thinking skill have been developed and conducted (Lane, 2005; Cheng, 2017; Williams, 2020).
There have been many studies on students’ conceptual understanding of natural phenomena related to space, movement, matter, and so on (Driver et al., 1985; Barke et al., 2009; Allen, 2010). For a natural phenomenon that students can experience in daily life, students have different ideas than scientists about astronomical phenomena such as the shape of the earth, the disappearance of the sun during the night, the disappearance of stars during the day, the apparent movement of the moon, and the cycle of day and night (Vosniadou and Brewer, 1992, 1994). The nature of alternative conceptions by students is debated from the perspective of the consistency of cognitive structures (Vosniadou, 2013). On the one hand, some researchers insist that individual cognitive structures have a kind of consistency that was strengthened or accumulated through daily life. On the other hand, other researchers argue that students’ understandings are not very coherent, rather they are situational or fragmented. However, both researchers agree that students should be supported to progress and acquire better conceptions that are shared with scientists (von Aufschnaiter and Rogge, 2015).
In addition to studies on students’ alternative conceptions that focus on specific contents and cognitive structures, scholars have also researched students’ use of cognitive skills, including visuospatial thinking skills, when learning scientific content and solving problems. Åberg-Bengtsson et al. (2017) reported how third- to sixth-grade students (aged 9–12 years) from a primary school in Sweden make sense of an illustration frequently used to explain the cause of lunar phases. In this study, a majority of students made sense of the important features of the illustration, but few students spontaneously understood the cause of the lunar phases in the intended meaning-making way. Taking different perspectives, such as the standing-on-Earth and above-the-ecliptic perspectives, which is necessary for understanding the phenomenon, was a stumbling block for the students. Another study found that preservice teachers who joined a primary education degree course created different models of day and night and the sun’s path, depending on their different perspectives (Heywood et al., 2013). These studies show that operating visuospatial thinking skills, such as perspective-taking while learning science content is a challenging task not only for younger children but also for older students and preservice teachers.
Stieff (2007) examined how undergraduate students and experts processed mental rotation and a learned heuristic strategy when they solved chemistry tasks involving spatial information. While more than half of the students used the visuospatial thinking skill of mental rotation on all tasks, other students applied the analytical strategy that was taught in organic chemistry instruction. However, the experts applied analytic strategies to the targeted tasks as a first step before using mental rotation. The results implied that analytical strategies may become dominant as expertise grows, thereby decreasing reliance on mental rotation or other forms of visuospatial thinking skills. In other studies, Stieff (2011) reported that undergraduate students rarely used imagistic strategies, that is, visuospatial thinking skills on tasks that did not specifically require representation translations. Rather, students engaged in diagrammatic strategies that refer specifically to the application of heuristics or algorithms to domain-specific diagrams without processing complex spatial transformations. Stieff et al. (2010) pointed out that there are distinct and interactive roles for imagistic, diagrammatic, and analytic strategies for problem-solving in the science classroom. It was also shown that some high school students prefer to rely on imagistic and diagrammatic reasoning, even after instruction on how to reason analytically (Kiernan et al., 2021). These studies revealed that older students do not always apply visuospatial thinking skills to visuospatial tasks. However, whether younger students behave like older students in visuospatial tasks has not yet been clarified, except for the studies on conceptual understanding in primary astronomy (Yanagimoto and Ohtaka, 2008; Okada and Matsuura, 2014). Specifically, when younger students do not seem to process visuospatial thinking skills, there are few studies investigating whether these students engage in tasks without visual operation or have difficulties developing basic visuospatial thinking skills. Moreover, our understanding of how they recall background knowledge of natural phenomena and apply it to problem-solving in a different context is limited. Additionally, the appearance of gender differences in visuospatial abilities could vary depending on the participant’s age or the task (Halpern and Collaer, 2005). To help address this, gap, this study posed the following research questions:
• What cognitive level do primary school students have in visuospatial thinking skills in domain-general learning?
• How do primary school students respond to visuospatial tasks that require the interpretation of a diagrammatic representation?
• Are there any differences between male and female students in visuospatial thinking tasks?
Materials and methods
Participants
The participants comprised 93 fourth-grade students (9–10 years old), including 51 male students (average = 10.2 years) and 42 female students (average = 10.1 years), from a public primary school in Japan. The students were taught by the same teacher. All fourth-grade students who attended the school participated in the study, except for the absentees. The Course of Study (CoS), which is the national science curriculum in Japan, was developed by the Ministry of Education, Culture, Sports, Science, and Technology (MEXT) and is usually revised every 10 years. The participating fourth-grade students were taught according to the 2008 revised CoS (Ministry of Education, Culture, Sports, Science, and Technology, 2008), which focuses on nurturing the ability to think, decide, and express. These are emphasized at all school levels from kindergarten to upper secondary school (Matsubara, 2018), and according to the CoS, the sciences include physics, chemistry, biology, and earth and space science. The curriculum for fourth-grade students includes various topics, such as simple electric circuits, changes in the state of matter, the human body, seasons, and the moon and stars. The topics in this study were how the sun moves through the sky, which is taught in third grade, and how solar cells work, which is taught in fourth grade. The students had already learned this content, and no special visuospatial training was offered before this study.
Data collection and analysis
Participants completed two types of paper-pencil tests written in Japanese. The first test required participants to answer the Wide-range Assessment of Vision-related Essential Skills (WAVES) test, which measures visual perception and eye-hand coordination skills (Okumurara and Miura, 2014). The WAVES test was validated using Japanese normative data and by comparing it with the Developmental Test of Visual Perception–Third Edition (DTVP-3) (Okumura et al., 2020). The WAVES test comprises ten subtests and measures visuospatial thinking skills (Table 1). WAVES mainly measures the visuospatial perception and visual memory components of McCormack’s taxonomy of visuospatial thinking skills.
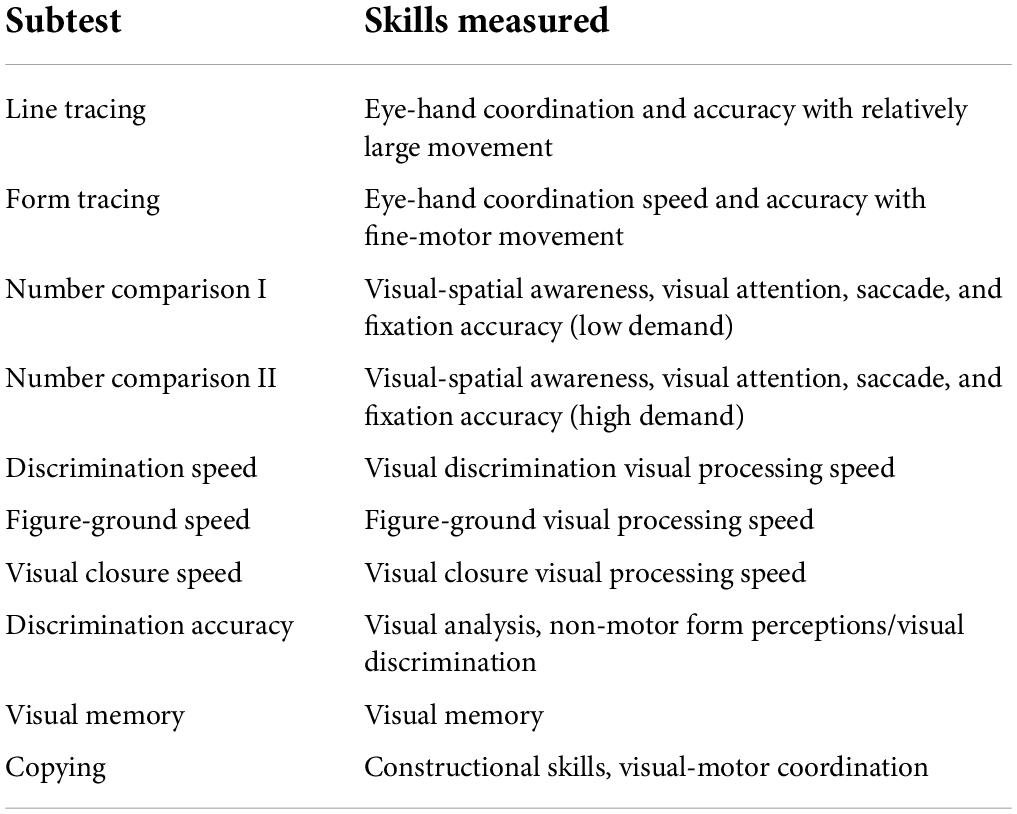
Table 1. Skills measured by the WAVES subtests (Okumura et al., 2020).
Following test instructions, the four index scores for the WAVES were calculated: Eye-hand Coordination Accuracy Index (ECAI), Eye-hand Coordination General Index (ECGI), Visual Perception and Eye-hand Coordination Index (VPECI), and Visual Perception Index (VPI). Developmental differences in visuospatial thinking skills among the students were then evaluated by comparing each index score to the standardized sample scores provided by the WAVES Guidebook (Okumurara and Miura, 2014).
The second test required students to answer questions about the relationship between the movement of the sun and the behaviors of solar cells placed in different places by interpreting a diagrammatic representation (Figure 1).
The students were asked to answer six questions regarding solar cells. The first two were factual knowledge questions. The first asked where the sun moves in the sky during the daytime, and the other asked how the motor works when sunlight shines on the solar cells. Students were then given three visuospatial questions. They were asked to identify the best places to move the cells to make the motor rotate faster at 9 AM, noon, and 3 PM, respectively. The building size (length, width, and height) was presented as visuospatial key information. The last question was visuospatial. This question asked what time of day the motor would move fastest at Place 6. In these last two parts, students’ logical-visuospatial thinking was explored. The situation posited was 1 day in November in southern Japan, where the students participated in the test. For context, it is important to note that Japan is in the north latitude of 20–46° and an east longitude of 122–154°.
All participants completed two paper-pencil tests without instructional scaffolding, except for simple clarifications. Subsequently, structured interviews were conducted with nine students. These students were selected according to the following steps: In the first step, students were categorized into two groups: one group performed above the average for the two paper-pencil tests, and the other was not. In the second step, ten students were listed from each group. In the final step, the candidates were selected based on their science teachers’ suggestions and considering gender groups. The teachers provided the personal information of students who could participate in the interview with the researcher without special support. The researchers then interviewed five male and four female students. The structured interviews, which lasted from 5 to 10 min, were intended to further probe how students engaged in the solar cell problems, especially focusing on the final question. The interview protocol was transcribed into text data. The scores from the WAVES test, answers from the solar cell problems, and verbal protocols of the interviews were analyzed. In addition, gender differences in the index scores and responses were scrutinized using the chi-square test and t-test, and spearman rank-order correlation. Students’ responses were analyzed from the learning and experience perspective including students’ alternative conceptions related to an astronomical phenomenon.
Results and discussion
Students’ visuospatial thinking skills in domain-general learning
The visuospatial thinking skills in domain-general learning by fourth-grade students from a primary school were examined. Students’ responses to each skill subtest were graded, and their index scores were calculated. Subsequently, by comparing the students’ index scores to the standardized scores for WAVES, which show the expected scores that children can receive depending on their age, the students’ cognitive levels in terms of visuospatial thinking skills were estimated. A summary of the students’ four index scores is shown in Table 2. The distribution of students’ four index scores is shown in Figure 2.
If a student has an index score of 100, their cognitive level in terms of visuospatial thinking skills is equal to the average level expected for students of the same age. A score above 110 indicates that the student’s cognitive level is within the third quartile. A score of less than 90 means the cognitive level is within the first quartile for their age (Okumurara and Miura, 2014). In this study, the former was seen as high achievers, while the latter was seen as low achievers.
For ECAI, which is composed of the ratio scores for line tracing and form tracing, the mean index score for both male and female students was above 100 (male students: Mean = 108.4, SD = 13.8; female students: Mean = 114.1, SD = 7.4), and the scores for many students were higher than the average level. There was a statistically significant difference in the mean ECAI scores for male and female students, which was found through a t-test (t = −2.162, df = 92, p < 0.05). Female students outperformed male students in terms of their ability to write and draw using their eyes and hands in coordinated ways as quickly and accurately as possible.
For the ECGI, composed of the sum of the scores for line tracing and form tracing, the mean index score for male and female students was also above 100 (male students: Mean = 106.4, SD = 17.1; female students: Mean = 110.6, SD = 11.4). For the VPECI, which is composed of the sum scores for line tracing and form tracing, ratio scores for line tracing and form tracing, number comparison (type 1) scores, discrimination speed, discrimination accuracy, visual memory, and copying, the mean index scores for male and female students were also above 100 (male students: Mean = 103.3, SD = 19.6; female students: Mean = 108.9, SD = 12.5). In addition, 40–60% of students had higher scores on these skills measures than the average.
However, for the VPI, the mean index scores for male and female students were under 100 (male students: Mean = 96.8, SD = 17.4; female students: Mean = 98.2, SD = 12.7). The cognitive level of visuospatial perception by the students in this study was as high as the expected ability level for the Japanese student in their age group. Overall, nine male students and one female student were considered low achievers for both the VPECI and VPI scores. Additionally, only one male student was a low achiever for all index scores. The results indicated that most low-scoring students did not require immediate additional educational interventions. However, further observation of a few students might be needed to determine whether they need better visuospatial training. The t-test showed that no statistically significant differences between male and female students exist in the ECGI, VPECI, and VPI scores (ECGI: t = −1.534, df = 92, p = 0.128; VPECI: t = −1.586, df = 92, p = 0.116; VPI: t = −0.515, df = 92, p = 0.608). Although researchers have reported that male students tend to be superior to female students in visuospatial thinking skills (Uttal et al., 2013; McCormack, 2017), this kind of gender difference did not seem to apply to the students in this study.
Students’ use of visuospatial thinking skills and interpretation of the diagram
In the first part of the solar cell problems, 42 (82.4%) male students and 38 (88.4%) female students answered that the sun moved from east to south to west in the sky during the daytime. Studies have found that both young students and older students find it difficult to understand the sun’s path when students are required to use visuospatial thinking skills such as mental rotation and perspective-taking (Allen, 2010; Heywood et al., 2013). However, acquiring factual knowledge about the sun’s path was a lower-demand task for fourth-grade students in this study. In addition, 48 (94.1%) male students and 42 (97.7%) female students correctly answered that the stronger the sunlight on the solar cell, the faster the motor rotates. The students had already learned the causal relationship between the intensity of sunlight, the current flow of the solar cells, and motor movement before this test. This is typical content for the fourth-grade science curriculum. It was seen from the responses to the two factual questions that most of the students had the factual knowledge essential to answering the later questions.
In the second part, the students answered three questions on the best places for making the motor rotate depending on the time at 9 AM, 12 noon, or 3 PM. In answering the questions, students were able to choose any options that they believed were suitable. The students’ response rates to the questions are shown in Figure 3. From the building size information presented, we can reason that there could be places with more or less sunlight, depending on the time. In the grade four assessment rubric for students’ responses on this part, students that chose suitable places at all three times, without adding unacceptable places, received the highest marks. For example, Places 1, 2, and 4, where sunlight comes from the east, were considered suitable places at nine o’clock in the morning, whereas Place 5, where sunlight was blocked by the Gym, was unsuitable. Students who chose some of the acceptable locations without choosing any unacceptable ones were marked as the second level. Third-level students chose a mix of both suitable places and unacceptable places. Finally, students who chose only unacceptable places were marked as the lowest level.
The students’ scores for the suitable place questions are shown in Table 3. Only a few students answered each question perfectly, but 40–65% of students found suitable places properly. It seemed easy for these fourth-grade students to determine a suitable place for the solar cell at noon because almost all of them had factual knowledge that the sun crosses the southern sky during the daytime. However, to answer the other two questions–9 AM and 3 PM–correctly, they had to guess the position of the sun and reason the path of the sunlight in the morning and afternoon. Although Place 5 was unsuitable because sunlight coming from the southeast at 9 AM would be blocked by the Gym, 19 (37.3%) male students and 19 (44.2%) female students thought it was a candidate. In addition, 15 (29.4%) male students and 12 (27.9%) female students answered that Place 2 was a suitable place at 3 PM. The combination of the places that students answered as suitable was also different for each time, as shown in Figure 3. A small number of students drew lines on the paper for cognitive help that might represent the sun’s movement or sunlight. However, there were no representations, such as purposeful drawings, on the majority of papers. From these observations, it might be guessed that the students in this study had challenges in changing the position of the sun in the sky through the mental operation of imagining the direction of sunlight and then identifying which places were suitable. Chi-square test indicated that no statistically significant differences were found in the responses to the suitable place questions between male and female students (9 AM: χ2 = 2.798, df = 3, p = 0.424; 12 No: χ2 = 3.914, df = 3, p = 0.271; 3 PM: χ2 = 1.776, df = 3, p = 0.620).
In the last part, the students answered one question that asked them to identify the best time for making the motor rotate at Place 6. In answering the question, students chose one place as suitable and explained it. In the grade four assessment rubric for students’ responses to this part, students were marked at the highest level when they chose just one suitable time correctly and constructed an explanation that included the relationship between the position of the sun at that time and the path of the sunlight to Place 6. In addition, to the sun being in the east at 9 AM, the students were required to mention that sunlight can reach this location without being shaded or blocked by different things. In descending order, the students who answered only the sun’s position at 9 AM correctly were marked as the second level, the students who chose 9 AM but did not make a reasonable explanation were marked as the third level, and the students who could not answer anything correctly were marked as the lowest level.
Students’ responses to the suitable time question are shown in Table 4.
Although 18 (35.3%) male students and 12 (27.9%) female students could find a suitable time, only five (9.8%) male students and three (7.0%) female students provided both the correct time and explanation. For example, the complete explanation made by one male student was that “(The sun) is in the east direction at 9 AM and (the sunlight can) reach there without any interruption.” A sufficient explanation made by one female student was that “Place 6 is in the east, the sun rises in the east.” This type of explanation mentioned the direction of Place 6 and the sun rising but did not explain why the other times were not suitable. In the interview, some of the students who were marked Excellent or Good mentioned their reasoning process, including the use of visuospatial thinking skills. Student 1 (female) who was marked as Good mentioned that she reasoned the formation of a shadow by the different buildings and its effect on the solar cell workings. She explained that she also used the attached table, which indicated the building size, for her reasoning in later parts of the interview. In addition, she explained her visuospatial thinking as moving her body to Place 6 and looking up at the sun from this position. Her visuospatial thinking skills were visualizing and perspective-taking.
Interviewer: Then, I think that shadows will be formed because the sun moves, but were you aware of how shadows can be formed (in this question)?
Student 1: Yes.
Interviewer: How did you do it?
Student 1: Because a shadow can be made on the opposite side of the sun, there is a shadow on the athletic field and the school buildings. However, for the Gym, the sunlight was shining on it. So (I answered) Place 6.
(a few seconds later)
Interviewer: How did you do it?
Student 1: At Place 6, I move my body to the position. (And looking up at the sun) I was aware of how the sun was shining.
Of the nine students interviewed, two students mentioned that they formed a shadow for a specific time and mapped it onto the diagram by going along with the sun’s movement during the daytime. The students visualized the shadows of the buildings to answer this question. They monitored their cognitive process and viewed it from a third person’s perspective. Following their manifestation, they demonstrated metacognition of their visuospatial thinking. The other two students referred to their awareness of a shadow. They did not explain the details.
Some students had a typical alternative conception of sunlight intensity in which they believe the differences in temperature between the seasons are caused by differences in the distance from the sun to the earth (Allen, 2010). That is, students believe that summer is hotter than winter because the sun is closer to the earth in summer. In this study, some students demonstrated that Place 6 is a suitable place for solar cell activation because Place 6 is near the sun in the east. They seemed to explain the differences in the intensity of sunlight hitting the earth’s surface based on the height of the sun in the sky. For example, student 2 (male), who was marked as Excellent, explained his reasoning as follows.
Student 2: Oh, here. At noon, it (the motor) rotates because (the sunlight) reaches (it) from the south, and from above, but it does not rotate so fast. For the west, it (the sunlight) comes from here (the west), but the sunlight is blocked by the Gym. For the east, it (the sun) is on this side, because the height of the sun on this side (the direction of the east) is low, the sunlight is near, then (I answered) 9 AM.
At least four students were suspected of having similar alternative conceptions following their responses to the paper-pencil tests. In addition, there were students with similar ideas in the group of students who chose 3 PM. In their visuospatial thinking, although they considered the direction of the sun in the morning, they missed that the sunlight reached Place 6 not from a vertical direction but in a diagonal direction. To better explain this, it is also necessary for them to learn concepts of space, such as surface area and angle, as one of the three elements of visuospatial thinking (National Research Council, 2006).
However, 19 (37.3%) male students and 18 (41.9%) female students answered that noon was the best time for solar cells to work. For example, the incorrect explanation made by one male student was that “Because the sun comes to the highest place at noon, the sunlight reaches easily.” Of course, this is the correct explanation for a general situation. However, this does not apply to this situation in which the students must consider buildings that block sunlight as visuospatial information. For example, student 3 (male), who was marked as Poor, answered honestly as follows.
Interviewer: Place 6. This time, in this experiment Place 6 is very important. Did you look at this well? Didn’t you look at it?
Student 3: No, I didn’t look at it.
Interviewer: You didn’t look at it. Didn’t you find it?
Student 3: Yes, I found it.
Interviewer: You found it?
Student 3: But I didn’t care about it.
In this case, he neglected the part of the question that asked him to guess the motor workings in a specific place. Regardless of whether the students intentionally ignored the part of the question referring to the location of Place 6, the students who answered noon as a suitable time did not use visuospatial information as a cue to answer it. Rather, they reasoned it based on alternative conceptions, such as “The temperature at noon is the highest in a day” and “The sun comes just above us at noon.” As one student wrote, they examined how the solar cell’s working changed by shedding light from different angles. However, the statement “The sun comes just above us at noon” was not true for the students in this study, who lived at north latitude 32°. They also learned about the temperature change 1 day before this study. If the students have any alternative conceptions on the topic or have learned it before, they might prefer to use their available knowledge to solve the problem first, especially in the case of novel problems or cognitively loaded tasks. The diagram given was drawn from a vertical viewpoint, the students did not question whether they should change this, so they had the same point of view. That is, they continued to maintain one perspective without taking different perspectives into account. The current problem was not just a matter of visuospatial thinking skills but also a matter of whether or not students had the appropriate background knowledge for this context. As reported, the students’ alternative conceptions seemed to interrupt their scientific and visuospatial thinking if they had no instructional scaffolding. Students’ cognitive processes in the context that included a physical experiment in an outdoor environment are in line with the nature of understanding of observational astronomy by the different students as explored by Vosniadou and Brewer (1992, 1994); Kyriakopoulou and Vosniadou (2014, 2020). Behind students’ cognitive behaviors in this study is the fact that they use an alternative conception induced from ordinary and perceptional experiences, therefore, they may not distinguish the scientific representation from perceptional-based representations. Although learning an epistemological perspective that can contribute to cognitive flexibility to consider different possible representations of the same situation or phenomenon is recommended (Kyriakopoulou and Vosniadou, 2014), we assume the fourth-grade students in this study had no chance to use or learn this concept until this study. The chi-square test indicated there were no statistically significant differences in the responses to the suitable time question between male and female students (χ2 = 1.611, df = 3, p = 0.657). Alternative conceptions that some students had were rooted in shared ordinary lives and perceptional experiences, then there was no evidence that either male or female students had a better understanding of these conceptions or related experiences. The students interpreted the diagram based on alternative conceptions of astronomical phenomena. This could be another plausible reason to support that no gender differences were found, additionally, the students were younger than participants in studies where gender differences were reported. Generally speaking, a phenomenological understanding emphasizes learning through direct observation of actual phenomena in the science curriculum in lower grades. It could be inferred that the fourth-grade students in this study lacked opportunities to develop visuospatial thinking skills in their science lessons.
Students’ visuospatial thinking skills in domain-general and domain-specific learning
Overall, by examining students’ visuospatial thinking skills, such as visuospatial perception and visual memory, in domain-general thinking and domain-specific logical visuospatial thinking almost no statistically significant differences between male and female students were found in their responses to the two paper-pencil tests, as mentioned above. The students’ achievement levels on the WAVES test, which were evaluated based on their VPECI and VPI scores, and the number of correct answers to the solar cell problems (marked as excellent or good) are shown in Table 5. For older students, it was reported that male students tend to outperform female students (Uttal et al., 2013; McCormack, 2017). However, this trend did not apply to the primary school students in this study. Both male and female students had the same level of factual knowledge, and they reasoned in similar ways to answer the questions.
The results implied that differences in students’ responses to questions and their use of visuospatial thinking were not influenced by gender. Instead, their responses were influenced by alternative conceptions held before this study or by the framing of the questions as visuospatial problems. In addition, no statistically significant differences were found in the measures between achievement and the number of correct answers (chi-square test: χ2 = 8.983, df = 8, p = 0.344). Then, the correlation between these measures was weak (ρ = 0.221, p = 0.032). In this study, there seemed to be no significant relationship between visuospatial perception, measured as domain-general thinking, and logical visuospatial thinking skills, measured as domain-specific thinking. Even if visuospatial thinking skills are malleable and transferable across the domains, students’ visuospatial thinking skills could depend on their domain-specific knowledge, which is a body of contextual knowledge in science.
Conclusion
Many different representations are used in science and science education. The cognitive process of transformation from a 2D to 3D representation, or from external representation to internal representation, is a key function of visuospatial thinking. Visuospatial thinking is characterized by both logical and creative processing of internal representations to solve problems, create new ideas, and improve skills. Visuospatial thinking is constructed from concepts of space, representation tools, and thinking skills. It is suggested that the taxonomy of visual thinking skills in science education has a pyramid structure that is layered with visuospatial perception, visuospatial memory, logical visuospatial thinking, and creative visuospatial thinking. This study examined whether there were primary school students with difficulties developing visuospatial thinking skills in domain-general learning. The study also explored how primary school students respond to visuospatial tasks that require interpretation of a diagrammatic representation and gender differences in visuospatial thinking task answers.
The student’s cognitive level of visuospatial thinking skills was examined through the WAVES test, and four index scores were calculated: ECAI, ECGI, VPECI, and VPI. Female students outperformed male students in terms of their ability to write and draw using their eyes and hands in coordinated ways, as shown in the ECAI scores. However, no statistically significant differences were found in the ECGI, VPECI, and VPI scores of male and female students. The results show that the cognitive level of visuospatial perception by the students in this study was not as expected based on the recommendation from WAVES. Overall, there was a limited number of students identified as low achievers on both VPECI and VPI scores.
The students’ use of visuospatial thinking skills in interpreting the diagram was examined by answering another assignment about the relationship between the movement of the sun and the behaviors of solar cells placed in different places (solar cell problems). From students’ responses to the questions, most had the factual knowledge that the sun travels from east to south to west in the sky during the daytime, and the stronger the sunlight on the solar cell, the faster the motor rotates. When students were asked about the motor workings in different places at the same time, it was easy for them to reason a suitable place for the solar cells at noon, using factual knowledge of the sun’s path. However, some students struggled to reason where the cells should be placed at other times of day, which requires mental rotation and visualization. When students were asked about the motor workings in different places at the same time, a limited number of students answered correctly, with the use of perspective-taking and/or visualization. Some students had an alternative conception of the sunlight intensity and the sun’s path in the sky. They reasoned the problem from their alternative conceptions without using visuospatial information or taking different perspectives from the original perspective given in the diagram. No statistically significant differences were found in the relationship between achievement in the domain-general test and the number of correct answers in the domain-specific test between male and female students.
The implications for science teaching from this study are to encourage students to engage in visuospatial thinking practices, such as perspective-taking. In addition, teachers should emphasize that the concepts of space, such as dimension, and conventional knowledge of different representations, such as a map, are drawn from one perspective. This study was limited in that it examined primary school students’ visuospatial perception through a domain-general test and domain-specific logical visuospatial thinking through the interpretation of a diagram. The standardized test used in this study did not fully cover visuospatial thinking skills in the domain-general. A more targeted instrument for examining specific visuospatial thinking skills such as mental rotation would be needed to know students’ cognitive level of visuospatial thinking skills. The study did not investigate students’ developmental differences in visuospatial thinking in domain-general and domain-specific or the relationship between them. From the theoretical framework of visuospatial thinking, which is constructed from concepts of space, tools of representation, and thinking skills, further research on students’ learning progress, including alternative conceptions like domain-specific knowledge, is recommended.
Data availability statement
The raw data supporting the conclusions of this article will be made available by the authors, without undue reservation.
Author contributions
Both were involved in the study design collaboratively. KK was involved in collecting and analyzing data. SU led the analysis and set an overarching argument for the study and was involved in writing the manuscript.
Funding
This work was supported by the Japan Society for the Promotion of Science (JSPS) Grant-in-Aid for Scientific Research (Grant Numbers JP19H01680 and JP22H01014).
Conflict of interest
The authors declare that the research was conducted in the absence of any commercial or financial relationships that could be construed as a potential conflict of interest.
Publisher’s note
All claims expressed in this article are solely those of the authors and do not necessarily represent those of their affiliated organizations, or those of the publisher, the editors and the reviewers. Any product that may be evaluated in this article, or claim that may be made by its manufacturer, is not guaranteed or endorsed by the publisher.
Acknowledgments
We are thankful to the students, science teachers, and our colleagues in this study.
References
Åberg-Bengtsson, L., Karlsson, K. G., and Ottosson, T. (2017). Can there be a full moon at daytime? Young students making sense of illustrations of the lunar phases. Sci. Educ. 101, 616–638. doi: 10.1002/sce.21279
Barke, H. D., Hazari, A., and Yitbarek, S. (2009). Misconceptions in Chemistry: Addressing Perceptions in Chemical Education. Berlin: Springer.
Cheng, Y. L. (2017). “The improvement of spatial ability and its relation to spatial training,” in Visual-Spatial Ability in STEM Education, ed. M. Khine (Berlin: Springer), 143–172. doi: 10.1007/978-3-319-44385-0_8
Daniel, K. L. (2018). Towards a Framework for Representational Competence in Science Education, Dordrecht: Springer.
diSessa, A. A. (2004). Metarepresentation: Native competence and targets for instruction. Cogn. Instr. 22, 293–331. doi: 10.1207/s1532690xci2203_2
Driver, R., Guesne, E., and Tiberghien, A. (eds) (1985). Children’s Ideas in Science. Buckingham: Open University Press.
Gagnier, K. M., Holochwost, S. J., and Fisher, K. R. (2022). Spatial thinking in science, technology, engineering, and mathematics: Elementary teachers’ beliefs, perceptions, and self-efficacy. J. Res. Sci. Teach. 59, 95–126. doi: 10.1002/tea.21722
Gilbert, J. K. (2008). “Visualization: An emergent field of practice and enquiry in science education,” in Visualization: Theory and Practice in Science Education, eds J. K. Gilbert, M. Reiner, and M. Nakhleh (Dordrecht: Springer), 3–24.
Groffman, S. (2006). “The relationship between visual perceptual problems and learning,” in Optometric Management of Learning-Related Vision Problems, eds M. M. Scheiman and M. W. Rouse (St. Louis, MO: Mosby Elsevier), 241–280.
Halpern, D., and Collaer, M. (2005). “Sex differences in visuospatial abilities: More than meets the eye,” in The Cambridge Handbook of Visuospatial Thinking, eds P. Shah and A. Miyake (Cambridge: Cambridge University Press), 170–212. doi: 10.1017/CBO9780511610448.006
Heywood, D., Parker, J., and Rowlands, M. (2013). Exploring the visuospatial challenge of learning about day and night and the Sun’s path. Sci. Educ. 97, 772–796. doi: 10.1002/sce.21071
Khine, M. S. (2017). “Spatial cognition: Key to STEM success,” in Visual-Spatial Ability in STEM Education, ed. M. Khine (Berlin: Springer), 3–8. doi: 10.1007/978-3-319-44385-0_1
Kiernan, N. A., Manches, A., and Seery, M. K. (2021). The role of visuospatial thinking in students’ predictions of molecular geometry. Chem. Educ. Res. Pract. 22, 626–639. doi: 10.1039/D0RP00354A
Kyriakopoulou, N., and Vosniadou, S. (2014). Using theory of mind to promote conceptual change in science. Sci. Educ. 23, 1447–1462. doi: 10.1007/s11191-013-9663-9
Kyriakopoulou, N., and Vosniadou, S. (2020). Theory of mind, personal epistemology, and science learning: Exploring common conceptual components. Front. Psychol. 11:1140. doi: 10.3389/fpsyg.2020.01140
Lane, A. K. (2005). Developing Ocular Motor and Visual Perceptual Skills: An Activity Workbook. Lewisville: Slack Incorporated.
Mathewson, J. H. (1999). Visual-spatial thinking: An aspect of science overlooked by educators. Sci. Educ. 83, 33–54.
Matsubara, K. (2018). “Elementary science education in Japan,” in Primary Science Education in East Asia: Contemporary Trends and Issues in Science Education, eds Y. J. Lee and J. Tan (Berlin: Springer), 49–77. doi: 10.1007/978-3-319-97167-4_3
McCormack, A. J. (2017). “Developing visual/spatial thinking in science education,” in Science Education. An International Course Companion, eds K. S. Taber and B. Akpan (Rotterdam: Springer), 143–156. doi: 10.1007/978-94-6300-749-8_11
Ministry of Education, Culture, Sports, Science, and Technology (2008). Shougakkou gakushuu sidou youryou kaisetsu Rika-Hen [Explanation for the course of study for elementary school: Science]. Tokyo: Dainippon Tosho.
National Research Council (2006). Learning to Think Spatially. Washington, DC: The National Academies Press, doi: 10.17226/11019
Newcombe, N. S., and Stieff, M. (2012). Six myths about spatial thinking. Int. J. Sci. Educ. 34, 955–971. doi: 10.1080/09500693.2011.588728
Novick, L. R. (2006). “The importance of both diagrammatic conventions and domain-specific knowledge for diagram literacy in science: The hierarchy as an illustrative case,” in Diagrammatic Representation and Inference, eds D. Barker-Plumm, G. Stapleton, S. Linker, C. Legg, E. Manalo, P. Viana, et al. (Berlin: Springer), 1–11. doi: 10.1007/11783183_1
Okada, D., and Matsuura, T. (2014). Tenmonbunnya niokeru zidou seito no kuukanninshiki nikansuru hikakukenkyuu (in Japanese) [A Comparative Study on the Spatial Cognition of Students in Astronomy]. J. Grap Sci. Jap. 48, 3–10. doi: 10.5989/jsgs.48.2-3_3
Okumura, T., Miura, T., Nakanishi, M., Fukui, M., Toshikawa, M., Shimakawa, S., et al. (2020). Validity of the wide-range assessment of vision-related essential skills in Japanese children with learning problems. Optom. Vis. Sci. 97, 275–285. doi: 10.1097/OPX.0000000000001504
Okumurara, T., and Miura, T. (2014). Guidebook for Wide-Range Assessment of Visual Essential Skills (in Japanese). Tokyo: Gakken.
Pande, P., and Chandrasekharan, S. (2017). Representational competence: Towards a distributed and embodied cognition account. Stud. Sci. Educ. 53, 1–43. doi: 10.1080/03057267.2017.1248627
Ramadas, J. (2009). Visual and spatial modes in science learning. Int. J. Sci. Educ. 31, 301–318. doi: 10.1080/09500690802595763
Roth, W.-M., and McGinn, M. K. (1998). Inscriptions: Toward a theory of representing as social practice. Res. Rev. Educ. 68, 35–59. doi: 10.3102/00346543068001035
Roth, W.-M., and Tobin, K. (1997). Cascades of inscriptions and the re-presentation of nature: How numbers, tables, graphs, and money come to re-present a rolling ball. Int. J. Sci. Educ. 19, 1075–1091. doi: 10.1080/0950069970190906
Shah, P., and Miyake, A. (eds) (2005). the Cambridge Handbook of Visuospatial Thinking. Cambridge: Cambridge University Press, doi: 10.1017/CBO9780511610448
Sorby, S., Veurink, N., and Streiner, S. (2018). Does spatial skills instruction improve STEM outcomes? The answer is “yes.”. Learn. Indiv. Differ. 67, 209–222. doi: 10.1016/j.lindif.2018.09.001
Stieff, M. (2007). Mental rotation and diagrammatic reasoning in science. Learn. Instr. 17, 219–234. doi: 10.1016/j.learninstruc.2007.01.012
Stieff, M. (2011). When is a molecule three dimensional? A task-specific role for imagistic reasoning in advanced chemistry. Sci. Educ. 95, 310–336. doi: 10.1002/sce.20427
Stieff, M., and Uttal, D. (2015). How much can spatial training improve STEM achievement? Educ. Psychol. Rev. 27, 607–615. doi: 10.1007/s10648-015-9304-8
Stieff, M., Hegarty, M., and Dixon, B. (2010). “Alternative strategies for spatial reasoning with diagrams,” in Diagrammatic Representation and Inference. Diagrams 2010: Lecture Notes in Computer Science, 6170, eds A. K. Goel, M. Jamnik, and N. H. Narayanan (Berlin & Heidelberg: Springer), 115–127. doi: 10.1007/978-3-642-14600-8_13
Tippett, C. D. (2016). What recent research on diagrams suggests about learning with rather than learning from visual representations in science. Int. J. Sci. Educ. 38, 725–746. doi: 10.1080/09500693.2016.1158435
Tversky, B. (2005). “Visuospatial reasoning,” in The Cambridge Handbook of Thinking and Reasoning, eds K. J. Holyoak and R. G. Morrison (Cambridge: Cambridge University Press), 209–240.
Uttal, D. H., and Cohen, C. A. (2012). Spatial thinking and STEM education? Psychol. Learn. Motiv. 57, 147–181. doi: 10.1016/B978-0-12-394293-7.00004-2
Uttal, D. H., Meadow, N. G., Tipton, E., Hand, L. L., Alden, A. R., Warren, C., et al. (2013). The malleability of spatial skills: A meta-analysis of training studies. Psychol. Bull. 139, 352–402. doi: 10.1037/a0028446
von Aufschnaiter, C., and Rogge, C. (2015). “Conceptual change in learning,” in Encyclopedia of Science Education, ed. R. Gunstone (Dordrecht: Springer), 209–219. doi: 10.1007/978-94-007-2150-0_99
Vosniadou, S. (2013). International Handbook of Research on Conceptual Change. New York, NY: Routledge.
Vosniadou, S., and Brewer, W. F. (1992). Mental models of the earth: A study of conceptual change in childhood. Cogn. Psychol. 24, 535–585. doi: 10.1016/0010-0285(92)90018-W
Vosniadou, S., and Brewer, W. F. (1994). Mental models of the day/night cycle. Cogn. Sci. 18, 123–183. doi: 10.1207/s15516709cog1801_4
Wai, J., and Kell, H. J. (2017). “What innovations have we already lost? The importance of identifying and developing spatial talent,” in Visual-Spatial Ability in STEM Education, ed. M. Khine (Berlin: Springer), 109–124. doi: 10.1007/978-3-319-44385-0_6
Wai, J., Lubinski, D., and Benbow, C. P. (2009). Spatial ability for STEM domains: Aligning over 50 years of cumulative psychological knowledge solidifies its importance. J. Educ. Psychol. 101, 817–835. doi: 10.1037/a0016127
Wu, H.-K., and Shah, P. (2004). Exploring visuospatial thinking in chemistry learning. Sci. Educ. 88, 465–492. doi: 10.1002/sce.10126
Yanagimoto, T., and Ohtaka, I. (2008). ‘Tsuki no michikake’ no rikai to nisyurui no kage “kage to kage” no rikai tono kankei: Syougaku 4 nensei niokeru zittai (in Japanese) [A Study on the Relationship of the Understanding between ‘Waxing and Waning of the Moon,’ and between ‘Shadow and Shade’ in the fourth-grade science class]. J. Res. Sci. Educ. 49, 81–92.
Keywords: external representation, internal representation, primary school science, science education, scientific diagrams, visuospatial thinking
Citation: Uchinokura S and Koba K (2022) Examination of children’s visuospatial thinking skills in domain-general learning and interpretation of scientific diagrams. Front. Educ. 7:892362. doi: 10.3389/feduc.2022.892362
Received: 09 March 2022; Accepted: 06 July 2022;
Published: 22 July 2022.
Edited by:
Vasilia Christidou, Aristotle University of Thessaloniki, GreeceReviewed by:
Steven J. Holochwost, Lehman College, United StatesIrini Skopeliti, University of Patras, Greece
Copyright © 2022 Uchinokura and Koba. This is an open-access article distributed under the terms of the Creative Commons Attribution License (CC BY). The use, distribution or reproduction in other forums is permitted, provided the original author(s) and the copyright owner(s) are credited and that the original publication in this journal is cited, in accordance with accepted academic practice. No use, distribution or reproduction is permitted which does not comply with these terms.
*Correspondence: Shingo Uchinokura, dWNoaW5va3VyYUBlZHUua2Fnb3NoaW1hLXUuYWMuanA=