- 1Department of Biology, Lawrence University, Appleton, WI, United States
- 2School of Integrative Plant Science and The L. H. Bailey Hortorium, Cornell University, Ithaca, NY, United States
- 3Department of Entomology, Cornell University, Ithaca, NY, United States
- 4Department of Integrative Biology, University of Texas at Austin, Austin, TX, United States
Organismal courses are inherently integrative, incorporating concepts from genetics, physiology, ecology and other disciplines linked through a comparative and phylogenetic framework. In a comprehensive organismal course, the organisms themselves are a lens through which students view and learn major concepts in evolutionary biology. Here, we present the learning goals of five core concepts (phylogenetics, biogeography, biodiversity, evo-devo, and key traits) we are using to transform organismal courses. We argue that by focusing on organismal knowledge and authentic examples, students learn foundational concepts and investigate biological hypotheses through the content that is unique to individual organismal groups. By using active learning strategies to teach core concepts, instructors can promote an inclusive classroom designed to engage students from diverse backgrounds and facilitate mastery and retention to test understanding of core biological concepts. This paper provides justification for why organismal biology needs to be kept as part of the biology curriculum, outlines the framework we are using to transform organismal courses, and provides examples of different ways instructors can incorporate active learning strategies and in-class activities in organismal courses in ways that enable their application to further investigation of both foundational and translational sciences for students.
Introduction
The changing curriculum landscape and resulting decline of traditional organismal biology courses (e.g., Entomology, Herpetology, Ornithology, Mammalogy, Botany) is bemoaned in various publications that emphasize the importance of comparative organismal biology as a means of broadly training students in modern research practices and methods, including genetics, genomics, developmental biology, and data science (Riley, 1931; Greene, 2005; Losos et al., 2013; Hosmani et al., 2019). Organismal biology courses are taxonomically-focused courses that study the evolution and ecology of an organism by looking at changes in morphology, physiology, and behavior of closely related species. These differ from other biology courses because, while organisms themselves are intrinsic to all biological studies, organismal biology is often taught within the context of many sub-disciplines of biology (molecular and cell biology, genetics, physiology, ecology) rather than offered as a separate course that teaches about organisms from a natural history perspective (Boyd, 2007).
The past decades have seen significant changes in requirements and offerings of organismal courses across universities and colleges. For instance, Cheesman et al. (2007) noted a decrease in required zoology courses in favor of courses that emphasize a particular sub-discipline of biology (e.g., genetics, or ecology) or mathematics (e.g., calculus, statistics). Over 40% of the biology departments surveyed required math and discipline-specific biology courses as part of the core department curriculum, yet have reduced their zoology core offerings by an average of 49% (Cheesman et al., 2007). Concurrently, there has been a reduction in organismal botany courses and degrees within both general biology and plant biology or plant science (formerly botany) curricula (Woodland, 2007; Drea, 2011). Courses focusing on genetic and molecular tools are required at the expense of comparative anatomy, morphology and whole organism courses contributing to phenomena such as plant blindness (Wandersee and Schussler, 1999; Allen, 2003) and a lack of opportunities to obtain a broad organismal understanding across undergraduate and graduate curricula (Futuyma, 1998; McGlynn, 2008). Modern genetics and statistics courses dominate the curricular maps that address collecting, analyzing, and interpreting data, which are essential skills to many biology careers (Gross, 2004). Yet, organismal biology with its attendant data sets in biodiversity, comparative, and spatial analyses provide a potentially better path for students to gain those competencies, particularly in introductory courses. Therefore, we are missing an opportunity; modern organismal courses should be whole organism-focused courses with a concepts-first approach that seamlessly incorporate critical statistic and data-driven analyses and interpretations with the diversity of the organisms themselves. By using active learning and online tools, students can work with real data through biodiversity and DNA databases and use those data to answer scientific questions.
Recognizing the need for an integrative perspective across biological disciplines, and realizing the renaissance of organismal biology sparked by advances spanning all scales of the life sciences, a team of researchers and educators generated a formal report on the “Grand Challenges in Organismal Biology” (Schwenk et al., 2009). In this report, organisms themselves take center stage as the “nexus” of many important ecological and evolutionary processes acting at the sub- and supra-organismal levels. Organismal knowledge is identified as fundamental to understanding and organizing information across all areas of biology (Schwenk et al., 2009) and answering fundamental research questions regarding evolution and adaptation while identifying the underlying molecular mechanisms (Denny and Helmuth, 2009; Denver et al., 2009; Halanych and Goertzen, 2009). Thus, organismal biology could be a way to lay the foundation for connecting across biological disciplines while also reinforcing information for teaching biology from first principles.
Recent research on student learning has shown that interdisciplinary team-work (often called social learning) is an important skill. Through effective collaboration, students learn how to communicate with peers and recognize the inherent importance of diversity and social interdependence in finding creative solutions (Gropp, 2009; Kültz et al., 2013). Education and broad training at the undergraduate and graduate level are essential to developing the content knowledge base necessary to address current environmental and ecological problems and to identify science-based solutions to alleviate social disparity (Schwenk, 2010; Stillman et al., 2011; Zamer, 2011). However, content knowledge alone cannot solve these large-scale issues. Students that actively work through difficult material with peers develop skills for listening to and integrating diverse perspectives when applying content knowledge to a problem. Active learning (engaging and applying content knowledge to deepen understanding of material) coupled with social learning could improve retention of content knowledge (Keyser, 2000). Therefore, we are in need of courses that bring together these components: the active, integrative learning brought by an organismal lens for teaching, and the problem-solving skills afforded by social learning. This manuscript presents a framework for teaching organismal biology using those two components.
Aims and Rational
Integrative course design employing active learning strategies (see Figure 1) can help resolve the dissonance between what students retain from a course relative to what they need to know for future careers. Here we describe our framework and efforts to connect the benefits of active learning with a concepts-based approach to teaching organismal biology. We present an efficient model for transforming organismal courses that instructors can implement across courses to help improve student learning through active engagement. We developed this framework by first identifying learning objectives that are commonly taught and that most immediately lend themselves to conversion to active learning. We then developed active learning modules to support these learning objectives that were transferable across three organismal courses. By focusing on concepts common across biodiversity science, we contribute to innovation in pedagogical methods tailored specifically for organismal and diversity-focused courses in the biological sciences.
Pedagogical Framework
Biology educators across the country are responding to multiple national calls to reform undergraduate biology education (National Research Council, 2003; American Association for the Advancement of Science [AAAS], 2011; Musante, 2011) by incorporating curricular changes that address key concepts and skills that explicitly help prepare students for careers both within and outside of the biological sciences. Successfully using organismal biology to teach fundamental concepts that integrate biological disciplines will maximize preparation of the next generation of scientists, educators, and professional degree practitioners to address the greatest biological challenges. To build upon the efforts that others have successfully established, we believe the combination of organismal biology and active learning becomes a logical intersection to address the importance of incorporating broad organismal knowledge within a modern Biology curriculum. We propose that using a concept-centric approach in organismal courses will provide a framework for reinforcing the principles of biology and making connections across disciplines. By using active pedagogical methods that promote inclusivity, we may also narrow achievement gaps for underrepresented students and retain a greater diversity of students in STEM (Ballen et al., 2017; Theobald et al., 2020).
We integrate five core evolutionary concepts—phylogenetics, biogeography, biodiversity, evo-devo, and trait evolution—and apply them across three organismal courses (Figure 2) as an example of how to potentially structure organismal courses. We chose these five concepts because they are fundamental to organismal and evolutionary biology, represent central concepts for understanding biodiversity and their relationship on the Tree of Life, and specifically address learning goals that target common misconceptions. We designed this so these concepts are implemented the same way in each course, but the content changes to use examples specific to organisms that are the focus of each course. With this framework, we hope to improve student understanding, retention and mastery of both organismal and evolutionary concepts by providing opportunities to practice these concepts in multiple contexts (i.e., different courses) and through different worksheets, clicker questions, and technology-supported active learning. This approach also enables students to place concepts learned across a range of biological scales, from genetic interactions to ecosystem services, in a larger perspective that will enhance their ability to function as interdisciplinary scientific thinkers. The adaptability of this framework may also benefit instructors and departments aiming to include active learning into their courses and transform multiple courses at once.
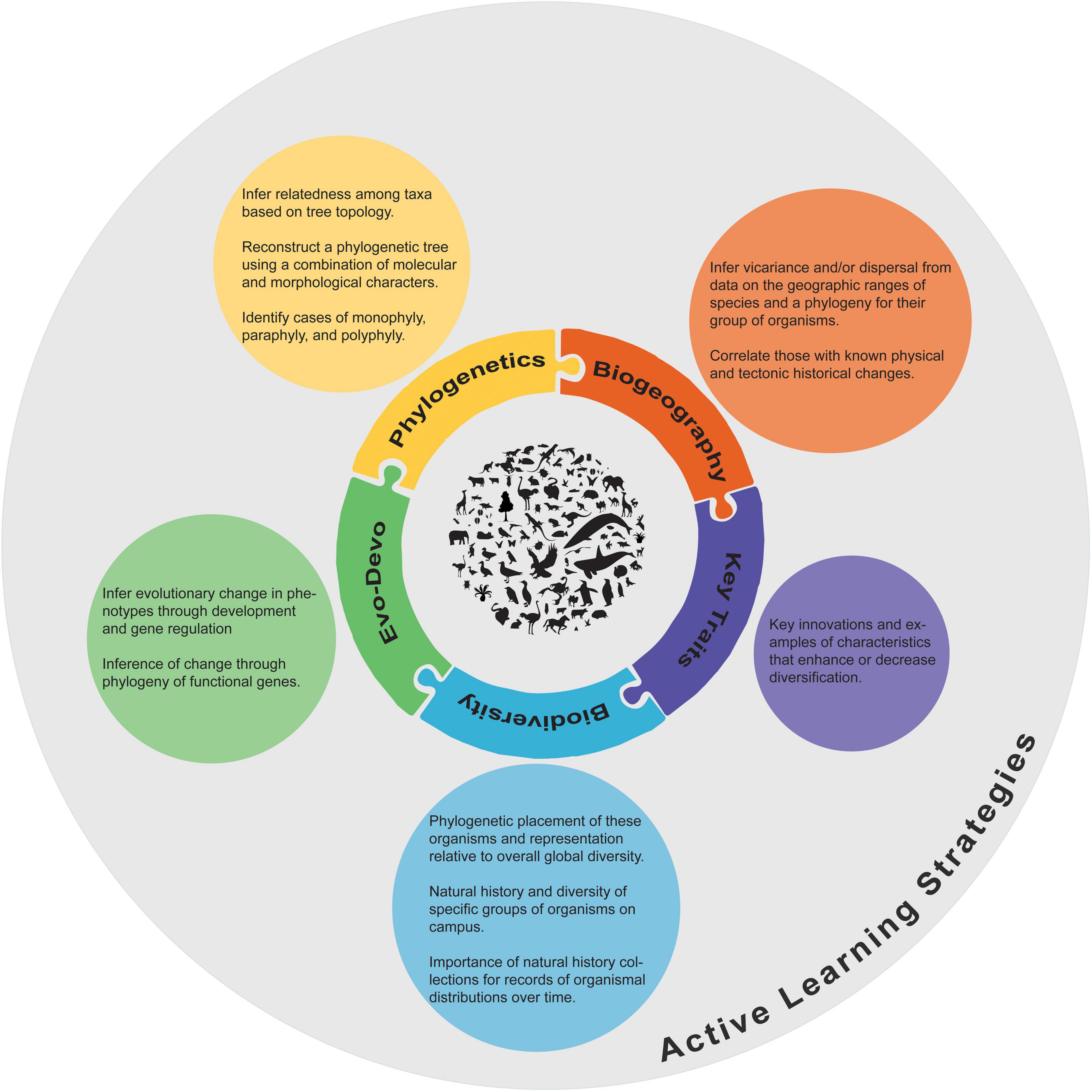
Figure 2. The five concepts we chose to exemplify how to design active learning in concept-based organismal courses. The learning objectives for each concept are listed in the outer circles. In addition to concepts, the courses exist in a matrix of best practices supported by the use of active learning and inclusive strategies to engage students in learning about organismal biology. The concepts unify organismal courses because they use the same content to teach about a concept through a different organismal lens.
Teaching Modern Concepts in an Organism-Centric Curriculum
Imagine yourself in front of a classroom full of students- “From this tree, you can see that a lizard is more closely related to a bird than to a frog.” Although this is likely not the first-time students have encountered a lesson on the Tree of Life, students often struggle with understanding evolutionary relationships and using phylogenies to test hypotheses related to the evolution of biodiversity. This lack of understanding of tree-thinking paired with general misconceptions about phylogenetic history presents challenges to faculty who need to first correct student fallacies about organismal relationships in order to teach fundamental concepts in evolutionary biology. Once learned correctly, tree-thinking enables students to gain a holistic understanding of natural history and the diversity of life. Many educators have been advocating for the use of phylogentic trees in education and are publishing modules, assessments and results of student learning of this content (Cooper et al., 2006; Meisel, 2010; University of California Museum of Paleontology, 2020). These tools and others help students gain an understanding of key organismal relationships and evolutionary concepts in a phylogenetic framework, that they can then apply more broadly to investigate scientific problems that are relevant across organismal lineages. For instance, one can provide a phylogeny of the Drosophila planitibia species group and ask students to approximate divergence times and hypothesize the mechanisms by which each species established on the Hawaiian Islands. Students would use their understanding of phylogenetic trees and molecular evolution to estimate diversification rates and understanding of evolutionary processes to generate biogeographic hypotheses for the species in this group. This exercise is adaptable to any taxon; examples could be drawn from Reed frogs on the Gulf of Guinea archipelago islands or the biogeography of the Crassulaceae family across several African countries and surrounding islands (Karimi et al., 2017). Due to the transferability of concept knowledge, student learning occurs in different organismal contexts but the students are taught to think about the concepts and apply those concepts across different evolutionary scales and across diverse organismal lineages.
A concepts-first approach to teaching allows students to grasp “big picture” ideas, rather than memorize stand-alone facts that are disengaged from the fundamental understanding of science (Olson, 2008). When done successfully, students learn about subject-specific content, while also integrating and applying that content to the broader concepts. Ideally, the concepts are reinforced throughout course topics with various examples, and applied broadly to other related courses with different content yet overlapping concepts (such as the biogeography examples from above). Concept-first teaching methods reinvigorate courses, allowing the content to populate the framework provided by the concepts. This approach increases student understanding of course content and helps students better connect to content within the context of a problem they are trying to solve (Jacobs and Moore, 1998; Harland, 2002), thereby facilitating the retention and recall of information. We advocate applying a concept-first framework because it provides students with a foundational structure for organizing new material, teaching students how to integrate knowledge across topics to build their scientific fluency to support more complex and integrative learning within and across courses. Courses that use concept-first framework additionally reflect the way that science is practiced, and as such are critical for preparing students to meet the rapidly growing needs of our next generation of scientists.
Organismal courses lend themselves to an integrative concept-first approach (Zupanc, 2008; Russell, 2009) as they systematically bridge the gaps across disciplines in biology (e.g., evolution, ecology, genetics, morphology, physiology), and provide a holistic perspective that unites the principle concepts across disparate fields (Pianka et al., 1998; Greene, 2005; Russell, 2009; Schwenk et al., 2009; Padilla et al., 2014). For instance, students in a Genetics class may learn about what causes a mutation; in an organismal course, students will apply that knowledge to understand how a mutation may manifest within a particular species or lineage, and how those mutations affect the evolution of the organisms leading to key adaptations or diversification in form and/or function. By linking organismal content (e.g., synapomorphies for various insect clades) to specific concepts (e.g., tree thinking or evo-devo), students are provided with a mechanism for learning and retaining the phylogenetic history of key traits while placing this knowledge in the context of evolutionary principles that cross biological disciplines.
Finally, many of the major advances in science are achieved by collaborative teams with complementary specialization, where each researcher contributes expertise to find an interdisciplinary solution. This approach is necessary because scientists are typically not trained in all fields at the depth required for scientific advancement; however, if each discipline becomes excessively siloed, the benefits of integration will become more difficult. For instance, the revolution of DNA sequencing technologies over the past 20 years has resulted in the use of molecular characters for phylogenetic reconstruction of all extant lineages across the tree of life. However, a holistic understanding of the entire organism from multiple disciplinary angles (including morphology, ecology, and behavior) enables scientists to characterize evolutionary changes occurring across these lineages and to relate the evolution of organismal traits to fundamental biological principles. While advances in genetics has led to an understanding of basic organismal processes in a handful of model organisms (e.g., development in Drosophila; Nusslein-Volhard, etc.), it is only through a comparative understanding of whole organisms that we can we see how the genetic processes detailed in model systems can lead to diversification of form and function across the tree of life, in deep and recent time, and at local and global scales.
Teaching With Active Learning-Inclusivity and Core Concepts
Active learning practices (see Figure 1), when taken as a whole, improve collaboration, critical thinking, problem-solving skills, and student performance. Small-group and project-based learning are instructional modalities often associated with active learning and have been shown to increase student scores, reduce achievement gaps, and build skills that are useful to scientists outside of the classroom environment (Gaudet et al., 2010; David, 2018). Project-based learning gives students the opportunity to use current technology and scientific methods to learn theories, collect data, and interpret their results, which increases engagement and improves critical thinking skills through critiques of their own work and the work of peers (Switzer and Shriner, 2000; David, 2018). Small-group learning also benefits students by improving student attitudes toward and confidence in group work (Harland, 2002; Gaudet et al., 2010) and is thus important not only for increasing accessibility of content within a class but also providing students with relevant skills in building and managing effective collaborations.
Active learning in STEM courses provides students opportunities to practice higher-order thinking skills and improve overall performance (Wieman, 2007; Freeman et al., 2014) while preparing students to join the scientific workforce. For instance, active learning techniques such as clicker questions, case studies, and worksheets reinforce content knowledge and give students opportunities to learn the material in novel ways (Bonwell and Eison, 1991), enhancing understanding and providing a framework for linking concepts across disciplines. Incorporating hands-on learning activities that emphasize transferrable knowledge and skills are more engaging, and help students develop deeper conceptual and practical understandings of scientific content through direct engagement in problem solving that mimics actual scientific discovery. We believe that using active learning methods in a concept-first organismal biology course is a valuable way to foster the use of scientific practices and provide a platform to explore concepts from various perspectives for all students.
Multiple studies show active learning exercises using small-group learning paired with low-risk or non-exam assessments can broaden participation and increase inclusivity in science by reducing achievement and persistence gaps that exist for female, first-generation, and underrepresented minority (URM) students (Lorenzo et al., 2006; Beichner et al., 2007; Ballen et al., 2017, 2018; Theobald et al., 2020). Greater performance outcomes for these groups of students improve student confidence and self-efficacy (Ballen et al., 2017), thereby fostering intrinsic motivation (Musante, 2012), raising awareness and understanding of potential career opportunities (Mead et al., 2015) and reducing attrition rates. In addition, active learning has the benefit of promoting collaboration and decreasing competition between individual students in the classroom (DeGrandi et al., 2018), providing a more inclusive environment for student engagement. This is particularly important for organismal courses, as these tend to attract fewer URM students despite the demonstrated importance of evolutionary science in advancing many STEM disciplines (Mead et al., 2015).
Active learning strategies lend themselves well to organismal knowledge focused on evolutionary or ecological concepts. For example, traditional biodiversity teaching methods present students with a list of taxonomic groups (e.g., families of flowering plants), along with characteristics or traits of each group that are used to distinguish among lineages. Most current biodiversity courses use a phylogenetic context, or “tree thinking,” to explore taxa and their traits, empowering students to use comparative methods to conceptually understand how biodiversity arose within and across lineages in both space and time. This context helps students gain a better understanding of the relationships and homologies among organisms (Ballen and Greene, 2017), and provides a framework for exploring the genetic or biogeographic associations of particular traits or ecological functions. Students benefit most when they can rationalize, rather than memorize, biotic evolutionary histories through learning about common ancestry, monophyletic lineages, and key traits (Ballen and Greene, 2017). Using specific case studies and active learning techniques, the traditional lecture on taxonomy becomes instead an exploration of trait evolution across a clade.
Learning Environment
We chose five concepts (phylogenetic inference, biogeography, biodiversity, key trait evolution, and the link between evolution and development) to integrate across Entomology, Botany, and Herpetology courses at Cornell University. The courses we describe include students at all levels of study—from freshman to graduate students. These courses have previously been taught using only lecture and a mix of application-based multiple choice and short answer exams. The activities we have developed are specifically designed to deliver the same amount of content but deepen conceptual knowledge and assess student acquired abilities to apply concepts to novel contexts. These activities will represent a diversity of hypothesis-driven activities including case studies, model simulations, problem-solving activities using examples from primary literature to increase classroom engagement and collaborative learning and provide students opportunities to practice scientific skills. The activities will be scaffolded throughout the course as low-stake assignments and students will work on these activities in small groups; all supporting an inclusive classroom environment. We provide examples of how we envision the implementation of these activities (see Figures 3, 4), with an emphasis on the ways in which we support our framework (concepts and content) using active learning strategies, engage students with the material, unify concepts within and across courses, and create inclusive learning environments.
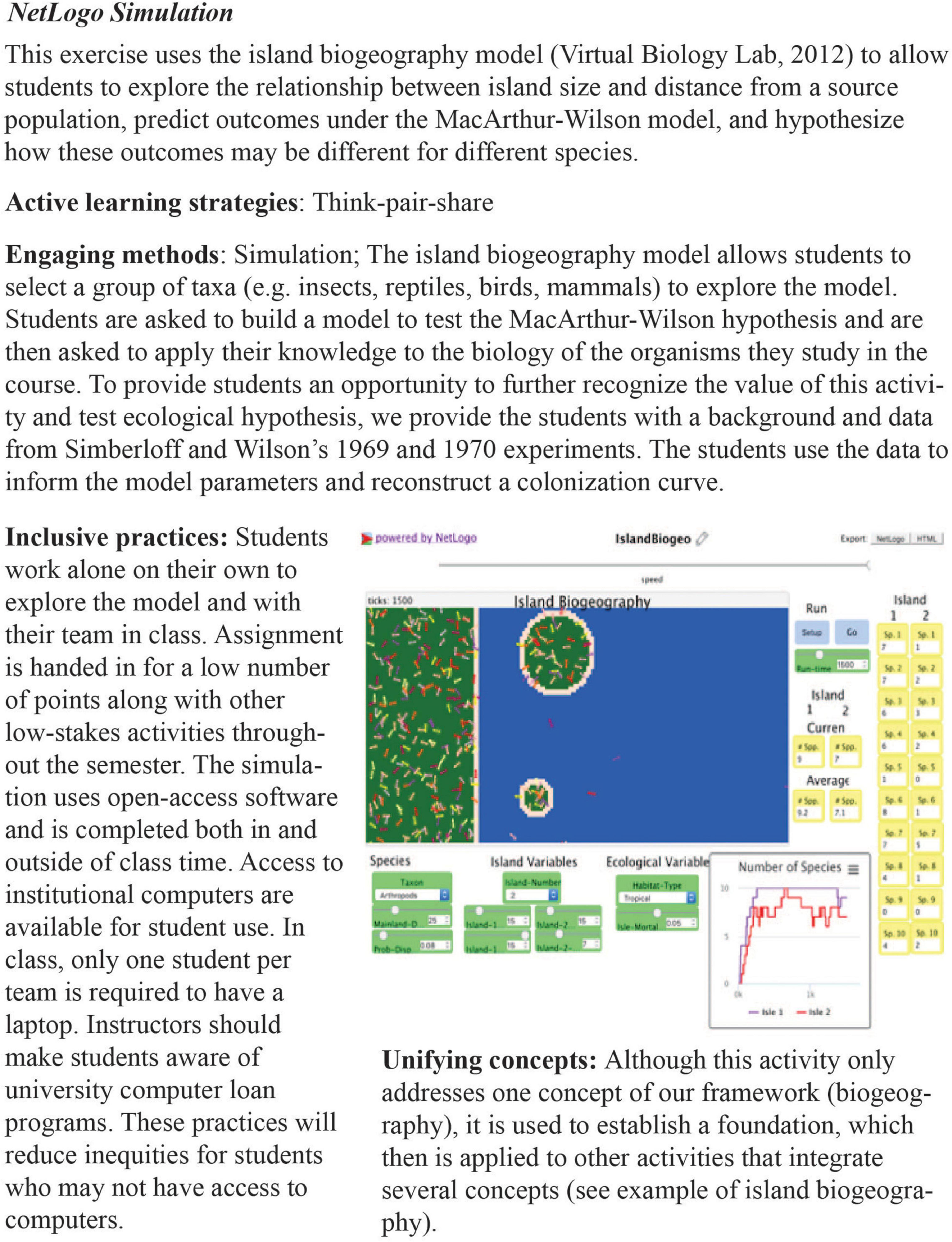
Figure 3. An example of an activity that is easily adapted for multiple courses. By changing the taxon, students can have discussions relevant to course content, even though the same concepts will be present regardless of taxa. Most of the time, the lessons will have to be adapted more specifically to the course content, but can still hit the same learning objectives and concepts.
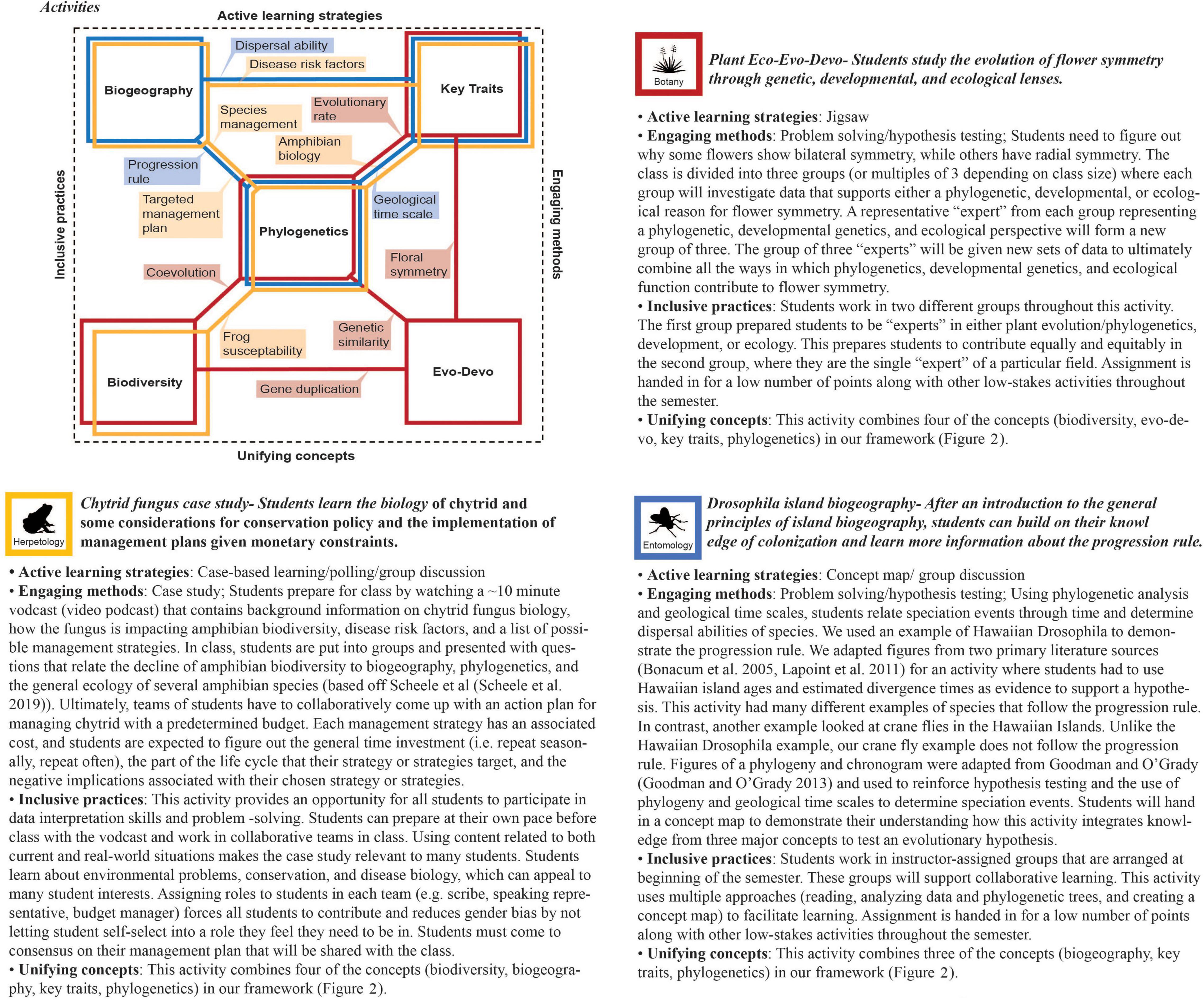
Figure 4. An example of activities that are developed for specific organismal courses, but integrate multiple concepts into a single lesson. These concepts are being taught in similar ways through each course.
Results
We plan to assess the transformation of organismal courses using this framework by collecting data on the efficacy of teaching improvements and student understanding. To characterize the transformation of these courses from traditional lecture courses (lectures without active learning before this framework was implemented) to active learning courses (after implementation of this framework), we will collect course observation data using the Classroom Observation Protocol for Undergraduate STEM (COPUS; Smith et al., 2013). Based on classroom observations, instructional practices can be categorized as didactic, interactive lectures, or student-centered (Stains et al., 2018) using COPUSprofiles.org. We will use this to assess how our instruction changed before and after the course transformation. We will also survey students about their engagement with the material, interest to learn, enthusiasm, and satisfaction with the class environment. These affective measures are important for learning and are often reflected in more inclusive classrooms and improved performance across demographic groups. The survey will include questions about how the course has contributed to their understanding of the learning objectives in organismal biology. We will compare student surveys across the three classes over the implementation of active learning modules.
To evaluate increases in student learning, students will be given Pre-Post knowledge assessments of the five concepts in both the traditional lecture and active learning courses. We will use multilevel modeling and model selection to look at changes from students pre and post scores, while accounting for demographic variables and other random effects (Theobald, 2018). We will also be using student exam data to compare student understanding in previous traditional lecture versions of the course with that achieved in semesters with active learning. From these data, we will be able to quantitatively (% correct) and qualitatively (coding for themes) analyze student answers. Student retention of concept knowledge will be tested by comparing the Pre-Post knowledge assessment and improvement of concepts in midterm and final exams. Students that take more than one of the transformed organismal courses will have their scores on the Pre-Post knowledge assessment and analyzed concepts in midterm and final exams modeled over different semester to look for changes in retention based on the context of the course. Retention test for conceptual understanding will also occur 6 months to a year after course completion. Formative assessments will primarily be used for student feedback rather than graded assessments. These ideas outline our assessment plan as we attempt to delineate a framework to be used in modern organismal biology courses.
Discussion and Limitations
Biology education is shifting toward engaging students in scientific practices and conceptual understanding using active learning strategies that emulate modern scientific practices. Organismal biology is an integral part of understanding the context of our scientific findings. We are applying modern concept framework across three organismal-focused courses. The concepts that unite these courses are central to understanding biology and uniting independently taught disciplines within the context of a group of organisms. We believe incorporating active learning-based educational resources and strategies using an inclusive approach to engage students from diverse backgrounds will facilitate mastery and retention of core biological concepts and enable their application to further investigation into both basic and applied sciences.
Our framework is designed to be adapted by instructors to any organismal context and the general theme of the concept-centric framework can be applied even more broadly across disciplines. Obviously not all universities will have the student body mass to teach taxon-specific courses. However, including organismic-centric examples as part of active learning will provide knowledge and learning enhancement. We understand that not all colleges and universities can offer organism-specific courses, however, these concepts and organismal examples would also serve well in courses of a more general, non-specific taxonomic topic, such as Ecology, Biodiversity, Biogeography, Evolution, and others. By using a concept-first approach, instructors can easily transform or infuse active learning into multiple courses at once. Instructors that are part of a faculty team trying to redesign courses, or looking to make changes to your own courses, should establish the concepts that are common to your courses. We first chose concepts that focused on the evolution of biodiversity and underlying biological processes. We then selected content that addressed one or more of the concepts and generated analogous activities using the content specific to each taxon-specific organismal course. For courses that are not taxon specific, a diversity of organismal examples could be used to demonstrate these fundamental concepts. The concepts serve as the course learning objectives, but each activity should first establish learning objectives that contribute to the major concept. We are using the backwards design approach to build course activities. This approach requires faculty to first identify specific content or skills they want their students to know (student-learning objectives), then find a way to show evidence that the students are learning (assess student-learning objectives), and finally plan how to teach this information (design activities to engage students in the learning process) (Wiggins and McTighe, 1998; Reynolds and Kearns, 2017). Our activities are being designed using examples from primary literature to draw from authentic examples of biological problems and ways to address research questions. We are also trying to design activities that capitalize on new approaches to provide students with opportunities for recognizing the practical uses of modern scientific methods (e.g., CRISPR, Gene Drive, Microbiomes, etc.).
Students also benefit from these concept-first courses as student learning tends to improve through active engagement. Using a variety of activities (i.e., simulations, case studies, problem-solving) active learning strategies (i.e., think-pair-share, polling, jigsaw), and assessment strategies (i.e., low-stakes quizzes and activity points, individual and group work) may mitigate the “leaky” pipeline by reducing the student achievement gap (Freeman et al., 2011; Haak et al., 2011; Theobald et al., 2020) and improve overall student performance in courses by learning about concepts and making connections between concepts in different ways (Allen-Ramdial and Campbell, 2014).
Using active learning in organismal-focused courses may also help increase participation of URMs and female students. URMs leave biomedical and behavioral science (e.g., zoology) majors more than white and Asian students (Chang et al., 2011). By implementing active learning strategies, particularly in organismal courses, it could draw in a new pool of students by improving student interest and motivation and set up future career options for groups that were traditionally discouraged from organismal biology fields (Rivers, 2006). It also may help retain female students in STEM careers as previous work indicates that female students underperform on exams relative to males, but tend to outperform males on non-exam assessments (Ballen et al., 2018). Students form scientific identities during their undergraduate years, so it is important for students to have exposure to different courses and career options. It is especially important for URM students to develop science identities, gain a sense of belonging, and increase their self-efficacy in order to persist in STEM careers (Trujillo and Tanner, 2014). To facilitate this, it is important to use examples that display the diversity of scientists and research in organismal biology so students can relate to these examples and gain a sense of belonging (Schinske et al., 2016).
We encourage faculty to use and build upon this framework. When designing your organismal courses, take advantage of the resources that help students gain transferrable knowledge and scientific skills to make your courses active, inclusive, and engaging. By teaching concepts with an active approach, we can help improve student learning and diversify our student body and the pipeline training the next generation of scientists, educators, and professional degree practitioners.
Data Availability Statement
The original contributions presented in the study are included in the article/supplementary material, further inquiries can be directed to the corresponding author/s.
Author Contributions
PO’G, CS, and KZ obtained the funding for this project. NC, CS, PO’G, and KZ conceived of the study and developed modules Herpetology (KZ), Entomology (PO’G), and Introductory Plant Sciences (CS) courses and wrote up the results. NC conducted in-class assessments to track impact of course changes. All authors contributed to the article and approved the submitted version.
Funding
This work was supported by the Active Learning Grant, College of Agriculture and Life Sciences, Cornell University.
Conflict of Interest
The authors declare that the research was conducted in the absence of any commercial or financial relationships that could be construed as a potential conflict of interest.
Publisher’s Note
All claims expressed in this article are solely those of the authors and do not necessarily represent those of their affiliated organizations, or those of the publisher, the editors and the reviewers. Any product that may be evaluated in this article, or claim that may be made by its manufacturer, is not guaranteed or endorsed by the publisher.
Acknowledgments
We thank Michelle Smith, Natasha Holmes, Claire Meaders, Frank Castelli, Emily Smith, and the CDER group for feedback on this manuscript. We also thank the College of Agriculture and Life Sciences Initiatives at Cornell University for funding this project.
References
Allen, W. (2003). Plant blindness. Bioscience 53, 926–926. doi: 10.1641/0006-3568(2003)053[0926:pb]2.0.co;2
Allen-Ramdial, S.-A. A., and Campbell, A. G. (2014). Reimagining the pipeline: advancing STEM diversity, persistence, and success. Bioscience 64, 612–618.
American Association for the Advancement of Science [AAAS] (2011). Vision And Change In Undergraduate Biology Education: A Call To Action. Washington, D.C.: American Association for the Advancement of Science.
Ballen, C. J., Aguillon, S. M., Brunelli, R., Drake, A. G., Wassenberg, D., Weiss, S. L., et al. (2018). Do small classes in higher education reduce performance gaps in STEM? Bioscience 68, 593–600. doi: 10.1093/biosci/biy056
Ballen, C. J., and Greene, H. W. (2017). Walking and talking the tree of life: why and how to teach about biodiversity. PLoS Biol. 15:e2001630. doi: 10.1371/journal.pbio.2001630
Ballen, C. J., Wieman, C., Salehi, S., Searle, J. B., and Zamudio, K. R. (2017). Enhancing diversity in undergraduate science: self-efficacy drives performance gains with active learning. CBE Life Sci. Educ. 16:ar56. doi: 10.1187/cbe.16-12-0344
Beichner, R., Saul, J. M., Abbott, D. S., Morse, J. J., Deardorff, D. L., Allain, R. J., et al. (2007). The Student-Centered Activities For Large Enrollment Undergraduate Programs (SCALE-UP) Project. Research-Based Reform in University Physics College Park, MD. Maryland, MD: American Association of Physics Teachers, 2–39.
Bonwell, C. C., and Eison, J. A. (1991). Active Learning: Creating Excitement in the Classroom. ASHE-ERIC Higher Education Report. Washington DC: School of Education and Human Development, George Washington University.
Boyd, I. L. (2007). Zoology: a search for pattern in form and function. J. Zool. 271, 1–2. doi: 10.1111/j.1469-7998.2006.00290.x
Chang, M. J., Eagan, M. K., Lin, M. H., and Hurtado, S. (2011). Considering the impact of racial stigmas and science identity: persistence among biomedical and behavioral science aspirants. J. High. Educ. 82, 564–596. doi: 10.1353/jhe.2011.0030
Cheesman, K., French, D., Cheesman, I., Swails, N., and Thomas, J. (2007). Is there any common curriculum for undergraduate biology majors in the 21st century? Bioscience 57, 516–522. doi: 10.1641/B570609
Cooper, S., Hanmer, D., and Cerbin, B. (2006). Problem-solving modules in large introductory biology lectures enhance student understanding. Am. Biol. Teach. 68, 524–529. doi: 10.2307/4452059
David, A. A. (2018). Using project-based learning to teach phylogenetic reconstruction for advanced undergraduate biology students: molluscan evolution as a case study. Am. Biol. Teach. 80, 278–284. doi: 10.1525/abt.2018.80.4.278
DeGrandi, C., Mochrie, S. G. J., and Ramos, R. (2018). “Pedagogical strategies to increase students’ engagement and motivation,” in Concepts, Strategies and Models to Enhance Physics Teaching and Learning, eds E. McLoughlin and P. van Kampen (Switzerland: Springer International Publishing), 215–227. doi: 10.1007/978-3-030-18137-6_19
Denny, M., and Helmuth, B. (2009). Confronting the physiological bottleneck: a challenge from ecomechanics. Integr. Comp. Biol. 49, 197–201. doi: 10.1093/icb/icp070
Denver, R. J., Hopkins, P. M., McCormick, S. D., Propper, C. R., Riddiford, L., Sower, S. A., et al. (2009). Comparative endocrinology in the 21st century. Integr. Comp. Biol. 49, 339–348. doi: 10.1093/icb/icp082
Drea, S. (2011). The end of the botany degree in the UK. Biosci. Educ. 17, 1–7. doi: 10.3108/beej.17.2
Freeman, S., Eddy, S. L., McDonough, M., Smith, M. K., Okoroafor, N., Jordt, H., et al. (2014). Active learning increases student performance in science, engineering, and mathematics. PNAS 111, 8410–8415. doi: 10.1073/pnas.1319030111
Freeman, S., Haak, D., and Wenderoth, M. P. (2011). Increased course structure improves performance in introductory biology. CBE Life Sci. Educ. 10, 175–186. doi: 10.1187/cbe.10-08-0105
Gaudet, A. D., Ramer, L. M., Nakonechny, J., Cragg, J. J., and Ramer, M. S. (2010). Small-group learning in an upper-level university biology class enhances academic performance and student attitudes toward group work. PLoS One 5:e15821. doi: 10.1371/journal.pone.0015821
Greene, H. W. (2005). Organisms in nature as a central focus for biology. Trends Ecol. Evol. 20, 23–27. doi: 10.1016/j.tree.2004.11.005
Gropp, R. E. (2009). A research and education agenda for biology? Bioscience 59, 932–932. doi: 10.1525/bio.2009.59.11.4
Gross, L. J. (2004). Points of view: the interface of mathematics and biology. Cell Biol. Educ. 3, 85–87. doi: 10.1187/cbe.04-03-0040
Haak, D. C., HilleRisLambers, J., Pitre, E., and Freeman, S. (2011). Increased structure and active learning reduce the achievement gap in introductory biology. Science 332, 1213–1216. doi: 10.1126/science.1204820
Halanych, K. M., and Goertzen, L. R. (2009). Grand challenges in organismal biology: the need to develop both theory and resources. Integr. Comp. Biol. 49, 475–479. doi: 10.1093/icb/icp105
Harland, T. (2002). Zoology students’ experiences of collaborative enquiry in problem-based learning. Teach. High. Educ. 7, 3–15. doi: 10.1080/13562510120100355
Hosmani, P. S., Shippy, T., Miller, S., Benoit, J. B., Munoz-Torres, M., Flores-Gonzalez, M., et al. (2019). A quick guide for student-driven community genome annotation. PLoS Comput. Biol. 15:e1006682. doi: 10.1371/journal.pcbi.1006682
Jacobs, D., and Moore, R. (1998). Concept-driven teaching and assessment in invertebrate zoology. J. Biol. Educ. 32, 191–199. doi: 10.1080/00219266.1998.9655620
Karimi, N., Parks, B. M., Rouse, D., Martin, K., Dong, X., Rajangam, P. C., et al. (2017). Building Trees: Introducing Evolutionary Concepts By Exploring Crassulaceae Phylogeny And Biogeography. London: CourseSource. doi: 10.24918/cs.2017.16
Keyser, M. W. (2000). Active learning and cooperative learning: understanding the difference and using both styles effectively. Res. Strategies 17, 35–44. doi: 10.2174/1874210601711010109
Kültz, D., Clayton, D. F., Robinson, G. E., Albertson, C., Carey, H. V., Cummings, M. E., et al. (2013). New frontiers for organismal biology. Bioscience 63, 464–471. doi: 10.1525/bio.2013.63.6.8
Lorenzo, M., Crouch, C. H., and Mazur, E. (2006). Reducing the gender gap in the physics classroom. Am. J. Phys. 74, 118–122. doi: 10.1119/1.2162549
Losos, J. B., Arnold, S. J., Bejerano, G., Iii, E. D. B., Hibbett, D., Hoekstra, H. E., et al. (2013). Evolutionary biology for the 21st century. PLoS Biol. 11:e1001466. doi: 10.1371/journal.pbio.1001466
McGlynn, T. P. (2008). Natural history education for students heading into the century of biology. Am. Biol. Teach. 70, 109–111. doi: 10.2307/30163213
Mead, L. S., Clarke, J. B., Forcino, F., and Graves, J. L. (2015). Factors influencing minority student decisions to consider a career in evolutionary biology. Evolution 8:6. doi: 10.1186/s12052-015-0034-7
Meisel, R. P. (2010). Teaching tree-thinking to undergraduate biology students. Evolution 3, 621–628. doi: 10.1007/s12052-010-0254-9
Musante, S. (2011). Upgrading undergraduate biology education. Bioscience 61, 512–513. doi: 10.1525/bio.2011.61.7.5
Musante, S. (2012). Motivating tomorrow’s biologists. Bioscience 62, 16–16. doi: 10.1525/bio.2012.62.1.5
National Research Council (2003). BIO2010: Transforming Undergraduate Education for Future Research Biologists. Washington, D.C.: The National Academies Press. doi: 10.17226/10497
Padilla, D. K., Daniel, T. L., Dickinson, P. S., Grünbaum, D., Hayashi, C., Manahan, D. T., et al. (2014). Addressing grand challenges in organismal biology: the need for synthesis. Bioscience 64, 1178–1187. doi: 10.1093/icb/icu038
Pianka, E. R., Hillis, D. M., Cannatella, D. C., Ryan, M. J., and Wiens, J. J. (1998). Teaching herpetology. Herpetologica 54, S3–S5.
Reynolds, H. L., and Kearns, K. D. (2017). A planning tool for incorporating backward design, active learning, and authentic assessment in the college classroom. Coll. Teach. 65, 17–27. doi: 10.1080/87567555.2016.1222575
Riley, W. A. (1931). Some present-day problems of zoology teaching. Science 73, 169–173. doi: 10.1126/science.73.1885.169
Rivers, D. (2006). Teaching general entomology to disinterested undergraduates. Am. Entomol. 52, 24–28. doi: 10.1093/ae/52.1.24
Russell, A. P. (2009). Situating and teaching 21st century zoology: revealing pattern in the form and function of animals. Integr. Zool. 4, 309–315. doi: 10.1111/j.1749-4877.2009.00165.x
Schinske, J. N., Perkins, H., Snyder, A., and Wyer, M. (2016). Scientist spotlight homework assignments shift students’ stereotypes of scientists and enhance science identity in a diverse introductory science class. CBE Life Sci. Educ. 15:ar47. doi: 10.1187/cbe.16-01-0002
Schwenk, K. (2010). Implementing the organismal agenda. Bioscience 60, 673–674. doi: 10.1525/bio.2010.60.9.2
Schwenk, K., Padilla, D. K., Bakken, G. S., and Full, R. J. (2009). Grand challenges in organismal biology. Integr. Comp. Biol. 49, 7–14. doi: 10.1093/icb/icp034
Smith, M. K., Jones, F. H. M., Gilbert, S. L., and Wieman, C. E. (2013). The classroom observation protocol for undergraduate STEM (COPUS): a new instrument to characterize university STEM classroom practices. CBE Life Sci. Educ. 12, 618–627. doi: 10.1187/cbe.13-08-0154
Stains, M., Harshman, J., Barker, M. K., Chasteen, S. V., Cole, R., DeChenne-Peters, S. E., et al. (2018). Anatomy of STEM teaching in North American universities. Science 359, 1468–1470. doi: 10.1126/science.aap8892
Stillman, J. H., Denny, M., Padilla, D. K., Wake, M. H., Patek, S., and Tsukimura, B. (2011). grand opportunities: strategies for addressing grand challenges in organismal animal biology. Integr. Comp. Biol. 51, 7–13. doi: 10.1093/icb/icr052
Switzer, P. V., and Shriner, W. M. (2000). Mimicking the scientific process in the upper-division laboratory. Bioscience 50, 157–162. doi: 10.1641/0006-3568(2000)050[0157:mtspit]2.3.co;2
Theobald, E. (2018). Students are rarely independent: when, why, and how to use random effects in discipline-based education research. CBE Life Sci. Educ. 17:rm2. doi: 10.1187/cbe.17-12-0280
Theobald, E. J., Hill, M. J., Tran, E., Agrawal, S., Arroyo, E. N., Behling, S., et al. (2020). Active learning narrows achievement gaps for underrepresented students in undergraduate science, technology, engineering, and math. Proc. Natl. Acad. Sci. U.S.A. 117, 6476–6483. doi: 10.1073/pnas.1916903117
Trujillo, G., and Tanner, K. D. (2014). Considering the role of affect in learning: monitoring students’ self-efficacy, sense of belonging, and science identity. CBE Life Sci. Educ. 13, 6–15. doi: 10.1187/cbe.13-12-0241
University of California Museum of Paleontology (2020). National Center for Science Education. [Internet]. Available online at: https://evolution.berkeley.edu/evolibrary/home.php (accessed March, 2020).
Wandersee, J. H., and Schussler, E. E. (1999). Preventing plant blindness. Am. Biol. Teach. 61, 82–86. doi: 10.2307/4450624
Wieman, C. (2007). Why not try a scientific approach to science education? Change 39, 9–15. doi: 10.3200/CHNG.39.5.9-15
Wiggins, G., and McTighe, J. (1998). “Backward design,” in Understanding by Design, eds G. Wiggins and J. McTighe (Alexandria, VA: ASCD), 13–34.
Woodland, D. W. (2007). Are botanists becoming the dinosaurs of biology in the 21st century? South Afr. J. Bot. 73, 343–346. doi: 10.1016/j.sajb.2007.03.005
Zamer, W. E. (2011). A cohesive biology of organisms is on the horizon. Bioscience 61, 848–849. doi: 10.1525/bio.2011.61.11.3
Keywords: active learning, organismal biology, inclusive teaching, STEM curricula, entomology, herpetology, botany
Citation: Chodkowski N, O’Grady PM, Specht CD and Zamudio KR (2022) Active Learning Strategies for Biodiversity Science. Front. Educ. 7:849300. doi: 10.3389/feduc.2022.849300
Received: 05 January 2022; Accepted: 31 March 2022;
Published: 26 April 2022.
Edited by:
Subramaniam Ramanathan, Nanyang Technological University, SingaporeReviewed by:
Watcharee Ketpichainarong, Mahidol University, ThailandHui Luan, National Taiwan Normal University, Taiwan
Copyright © 2022 Chodkowski, O’Grady, Specht and Zamudio. This is an open-access article distributed under the terms of the Creative Commons Attribution License (CC BY). The use, distribution or reproduction in other forums is permitted, provided the original author(s) and the copyright owner(s) are credited and that the original publication in this journal is cited, in accordance with accepted academic practice. No use, distribution or reproduction is permitted which does not comply with these terms.
*Correspondence: Nicole Chodkowski, nicole.chodkowski@lawrence.edu