- 1Chair of Autonomous Systems and Mechatronics, Department of Electrical Engineering, Friedrich-Alexander-Universität Erlangen-Nürnberg, Erlangen, Germany
- 2Department of Artificial Intelligence in Biomedical Engineering, Friedrich-Alexander-Universität Erlangen-Nürnberg, Erlangen, Germany
- 3Hertie Institute for Clinical Brain Research and Center for Integrative Neuroscience, University of Tübingen, Tubingen, Germany
- 4Institute for Modelling and Simulation of Biomechanical Systems, Computational Biophysics and Biorobotics, University of Stuttgart, Stuttgart, Germany
- 5Cognitive Modeling in Dynamic Human-Machine Systems, Technical University of Berlin, Berlin, Germany
1. Introduction
Learning interdisciplinary matters requires to adapt (previous) knowledge and to align one's own capacities to the current educational task (Ivanitskaya et al., 2002). This becomes particularly challenging in fields of study that are strongly interdisciplinary, e.g., at the boundary of human and engineering sciences. In this position paper, we put forward four considerations based on our teaching experiences in the context of human-robot interaction (Siciliano et al., 2008; Bartneck et al., 2020) and stimulated by university didactic training. We root our position on teaching experience from six German universities.
Namely, we recommend putting emphasis on communication, interaction, blending teaching methods, and providing students with orientation. Results from student evaluations of interdisciplinary courses with participants from different study programs are provided to give a practical perspective on these four considerations.
2. Four considerations
To develop common ground for interdisciplinary learning, it is important to specify and comprehend different learning objectives, i.e., what engineering students should know about human-related considerations and what human science students should know about technology. While we develop our four considerations from our overall experience, we substantiate our opinions with results from curricular evaluations of the students attending our courses. Particularly, we analyzed the open item responses of the following evaluations:
• Computational Motor Control (Summer term 2019 and 2020, N = 27. M.Sc. in neuroscience and computer science. University of Tübingen, Germany).
• Biorobotics (Summer term 2020 and 2021, N = 40. M.Sc. in biomedical engineering, computer science, and related programs. University of Tübingen and University of Stuttgart, Germany).
• Human-centered Robotics (Winter term 2021, N = 13. M.Sc. in electrical and robotic engineering. TU Dortmund, Germany).
• Human-Mechatronics Systems (Winter term 2021, N = 23. M.Sc. in mechancial and eletrical engineering. TU Darmstadt, Germany).
• Human-centered Mechatronics and Robotics (Summer term 2021, N = 15, M.Sc. in electrical, mechatronic, and medical engineering, FAU Erlangen-Nürnberg, Germany).
• Introduction to Cognitive Modeling (Summer term 2022, N = 14. M.Sc. in Human Factors. TU Berlin, Germany).
• Applied Cognitive Modeling (Winter term 2021, N = 10. M.Sc. in Human Factors. TU Berlin, Germany).
Considering human-robot interaction, engineering students need to learn biomechanical and psychological topics beyond technical aspects, e.g., biomechanical signals and models (Burdet et al., 2013) or empirical study designs (Gravetter and Forzano, 2018). In contrast to that, students of the human sciences require a certain level of technical knowledge, e.g., how to mechanically design a prosthesis and how to implement a controller to best match neuro-muscular properties (Eilenberg et al., 2010) or which signals can be used to interface between the human and the robot (Soekadar et al., 2015; Sharbafi et al., 2020). Furthermore, students need to understand why the other disciplines are important to the field, e.g., potentials or issues arising if one perspective would or would not be taken into account, and how they approach challenges, e.g., social robotics considering humans' expectations toward a robot. Through that, students can become aware of what would be missing when limiting research to a single discipline and how interdisciplinary approaches provided added value by extending perspectives, e.g., matching users' needs in technical design. Discipline-specific learning objectives provide a frame for our four considerations that outlined in Figure 1 and particularized below.
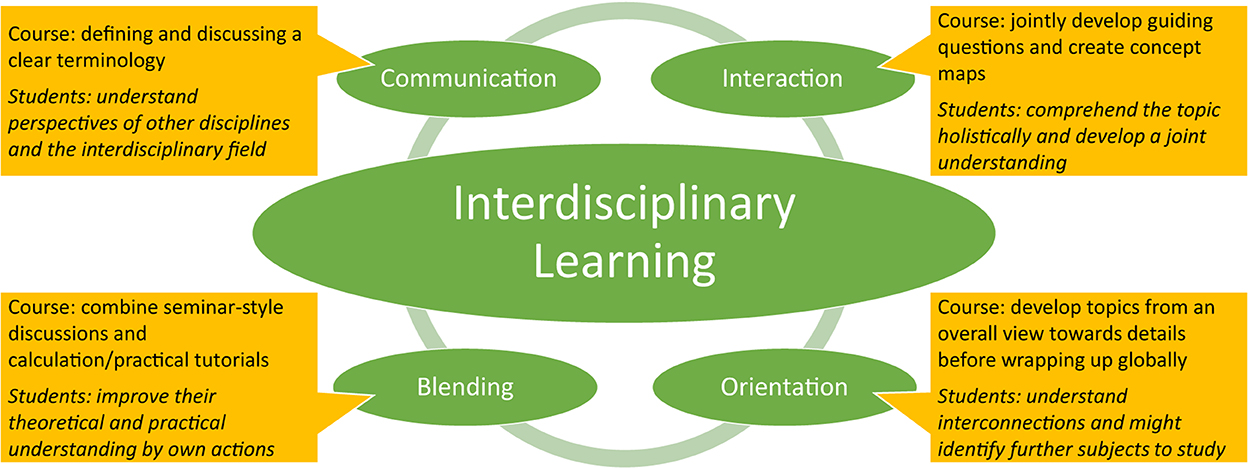
Figure 1. We propose emphasis on developing joint communication, promoting interaction, blending teaching elements, and proving students with orientation to improve their interdisciplinary learning. The yellow boxes suggest concrete actions that could be taken for implementation, which are describe in more detail in the text.
2.1. Communication
Practical insights from the authors' teaching and research show that Communication is a key aspect: simple questions like “What is a model?” may have very different answers in different branches of science. Therefore, collecting those definitions and determining a clear terminology for a given topic is a crucial step at the beginning of a course. A continuous discussion of terminology helps the students to understand perspectives of the other disciplines and thereby developing a better understanding of the interdisciplinary field as a whole. This is supported by a student's evaluation comment: “I learned a lot preparing the papers. They helped tremendously putting everything into context.” Integrating students from different backgrounds while considering knowledge uncertainties can further be fostered by using tangible examples, e.g., social robots in elderly care, collaborative robots supporting workers, or science-fiction companion robots, as is supported by the students liking “examples from instructors own experiences.”
2.2. Interaction
If both, students and lecturers are aware of the different learning goals, they can Interactively develop guiding questions for the course, e.g., “How can we predict the users' intentions?” or “When is the system/study considered successful?.” Interactive project tasks and discussions in small groups help students not only to comprehend the topic holistically but also to develop a joint understanding. Evaluating our courses, students fed back that “It's very great to make as much effort to make the course interactive, even if there are a few answers from the student.” Besides their self-critical view, they also suggest: “Maybe having more links between the seminars and the course could help to be more interactive in the class.” Therefore, we recommend jointly creating concept maps (Novak, 1990; Ivanitskaya et al., 2002) to structure the content of the interdisciplinary matter by linking concepts. Applying this promising interactive measure, we asked students to watch a recorded video by the lecturer and actively analyze the topic (reading related publications, searching important terms on the internet). Subsequently, the students were discussing the information and jointly condensed it into a 1-page concept map, which enabled them report their findings very accurately and concisely. In evaluation, students acknowledged that “the exam [...] in the form of an essay and a concept map, [allows to] explain your knowledge much better.” Furthermore, students stated to enjoy discussions about differing perspectives even if there is no definite answer.
Generally, flip-the-classroom methods seem promising as students report them to “get us involved more in the lecture.” These methods can further be extended by asking students to assume different roles to broaden their perspectives on requirements and challenges to be considered, e.g., view point of elderly users or a nurse using an assistive robotic system.
2.3. Blending
Accordingly, interaction can further be promoted by Blending teaching approaches to integrate interdisciplinary knowledge, e.g., we use seminar-style discussions for human-centered research while we apply calculation/practical tutorials for engineering aspects. Both approaches can even be integrated in small-scale projects where students are asked to analyze and solve a human-centered design task as a team. The usefulness of such projects is substantiated by students reporting that “having small projects is way more “natural” than a lecture where we would be asked about theoretical aspects. Receiving sources and learning on our own is a very cool approach to conveying knowledge,” particularly topics that involve hand-on projects. Yet, theoretical aspects can also benefit from blending as becomes obvious in responses like “The idea of offering a seminar in addition to the lecture that puts the contents of the lecture into practice [was good].” or “The course is a good mix of knowledge transfer and discussion.” Moreover, students verbally mentioned that only through the project they “gained real understanding” about the theoretical knowledge obtained earlier.
2.4. Orientation
To convey how those methods synergistically connect in the considered interdisciplinary matter, we postulate to provide Orientation to the students by outlining and elaborating interconnections through the structure of the course: a human-robot interaction course for instance might develop topics from an overall view toward the human and then the robot perspective before wrapping up globally again. However, interdisciplinary courses will always face a compromise of broadness and depth. Students accordingly state “it is always more helpful to get a broad overview and just dive into one or two topics deeply” or, oppositely, that “new content would need to be elaborated a bit more in order to understand it in the long run.” However, they might also identify further subjects to study from such experiences. In advanced courses, providing a few striking research articles could contribute to strengthen the orientation in addition to the previously mentioned concept maps.
3. Discussion
Remarkably, our four considerations can reinforce each other. Seminar elements increase interactions compared to frontal lectures and can further help the students to gain orientation. Similarly, promoting interaction and providing orientation can push developing a joint communication basis. One straightforward approach for implementation that we applied successfully is flipping the classroom in the end of frontal lecture sessions to interactively summarize the key take home messages. By that students are not only triggered to recapture the content but also to verbalize and discuss the main topics. Some students seem to doubt their interdisciplinary capabilities expecting that they need to know everything. Hence, making them aware that knowing how to methodically connect approaches from different fields as well as comprehending and considering knowledge of an expert from the other field are main learning objectives.
Besides the four considerations, we see a high potential in not only applying those in existing seminars or lectures, but molding them into teaching projects, which convey the interdisciplinary challenges when working on a practical issue. Past studies have already shown that engineering students benefit from course projects that connect theoretical knowledge with real-world problems, as such projects can help prepare them for working in the industry as well (Chang et al., 2022; Fan et al., 2022). In addition to designing and working on projects aiming at solving real-world problems, students also need to be prepared for teamwork and technical communication (Mihret Dessie et al., 2022). Similar to the considerations themselves, this is underlined by student evaluations, e.g., “the idea of doing [a project] is great”), which also show the challenges of such approaches. e.g., temporal constraints: “short time frame of the project.” To this end, classical exercise slots can be re-used to implement team projects, as we for instance did to make students explore how to succeed (and fail) as a team working on an engineering task.
Although focusing on human-robot interaction and the boundary of human and engineering sciences, we believe that other interdisciplinary fields will benefit from our considerations.
Author contributions
PB conceptualized the article and coordinated its development as well as the integration of individual contributions. All authors contributed the content, opinions, and references as well as discussed and revised the manuscript.
Funding
This research was supported by the Volkswagen Foundation (Az. 9B 007).
Acknowledgments
The authors thank their students for feeding back their opinions in the teaching evaluations.
Conflict of interest
The authors declare that the research was conducted in the absence of any commercial or financial relationships that could be construed as a potential conflict of interest.
Publisher's note
All claims expressed in this article are solely those of the authors and do not necessarily represent those of their affiliated organizations, or those of the publisher, the editors and the reviewers. Any product that may be evaluated in this article, or claim that may be made by its manufacturer, is not guaranteed or endorsed by the publisher.
References
Bartneck, C., Belpaeme, T., Eyssel, F., Kanda, T., Keijsers, M., and Šabanović, S. (2020). Human-Robot Interaction: An Introduction. Cambridge: Cambridge University Press.
Burdet, E., Franklin, D. W., and Milner, T. E. (2013). Human Robotics: Neuromechanics and Motor Control. Cambridge, MA: MIT Press.
Chang, T.-S., Wang, H.-C., Haynes, A. M., Song, M.-M., Lai, S.-Y., and Hsieh, S.-H. (2022). Enhancing student creativity through an interdisciplinary, project-oriented problem-based learning undergraduate curriculum. Thinking Skills Creat. 46, 101173. doi: 10.1016/j.tsc.2022.101173
Eilenberg, M., Geyer, H., and Hugh, H. (2010). Control of a powered ankle-foot prosthesis based on a neuromuscular model. IEEE Trans. Neural Syst. Rehabil. Eng. 18, 164-173. doi: 10.1109/TNSRE.2009.2039620
Fan, H., Xie, H., Feng, Q., Bonizzoni, E., Heidari, H., McEwan, M. P., and Ghannam, R. (2022). Interdisciplinary project-based learning: experiences and reflections from teaching electronic engineering in china. IEEE Trans. Educ. 1–10. doi: 10.1109/TE.2022.3186184
Gravetter, F. J., and Forzano, L. A. B. (2018). Research Methods for the Behavioral Sciences. Cengage Learning.
Ivanitskaya, L., Clark, D., Montgomery, G., and Primeau, R. (2002). Interdisciplinary learning: process and outcomes. Innovat. Higher Educ. 27, 95–111. doi: 10.1023/A:1021105309984
Mihret Dessie, W., Kassahun Bewuket, H., and Esubalew Tariku, M. (2022). Technical communication for civil engineering: academia-industry interdisciplinary needs. Eur. J. Eng. Educ. 47, 774–792. doi: 10.1080/03043797.2022.2081532
Novak, J. D. (1990). Concept mapping: a useful tool for science education. J. Res. Sci. Teach. 27, 937-949. doi: 10.1002/tea.3660271003
Sharbafi, M., Naseri, A., Seyfarth, A., and Grimmer, M. (2020). “Neural control in prostheses and exoskeletons,” in Powered Prostheses (Academic Press), 153–178.
Siciliano, B., Khatib, O., and Kröger, T. (2008). Springer Handbook of Robotics, Vol. 200. Berlin: Springer.
Keywords: interdisciplinary learning, teaching evaluation, human-robot interaction, human sciences, engineering sciences
Citation: Beckerle P, Hao C, Haeufle DFB and Russwinkel N (2022) Four considerations on interdisciplinary learning at the boundaries of human and engineering sciences. Front. Educ. 7:1096111. doi: 10.3389/feduc.2022.1096111
Received: 11 November 2022; Accepted: 25 November 2022;
Published: 07 December 2022.
Edited by:
Kostas Nizamis, University of Twente, NetherlandsReviewed by:
Pinaki Chakraborty, Netaji Subhas University of Technology, IndiaCopyright © 2022 Beckerle, Hao, Haeufle and Russwinkel. This is an open-access article distributed under the terms of the Creative Commons Attribution License (CC BY). The use, distribution or reproduction in other forums is permitted, provided the original author(s) and the copyright owner(s) are credited and that the original publication in this journal is cited, in accordance with accepted academic practice. No use, distribution or reproduction is permitted which does not comply with these terms.
*Correspondence: Philipp Beckerle, cGhpbGlwcC5iZWNrZXJsZUBmYXUuZGU=