- 1College of Liberal Arts and Sciences, Department of Geography, Portland State University, Portland, OR, United States
- 2College of Education, Department of Special Education, Portland State University, Portland, OR, United States
This article reports on an empirical evaluation of the experience, performance, and perception of a deafblind adult participant in an experimental case study on pedestrian travel in an urban environment. The case study assessed the degree of seamlessness of the wayfinding experience pertaining to routes that traverse both indoor and outdoor spaces under different modalities of technology-aided pedestrian travel. Specifically, an adult deafblind pedestrian traveler completed three indoor/outdoor routes on an urban college campus using three supplemental wayfinding support tools: a mobile application, written directions, and a tactile map. A convergent parallel mixed-methods approach was used to synthesize insights from a pre-travel questionnaire, route travel video recordings, post-travel questionnaire, and post-travel interview. Our results indicate that wayfinding performance and confidence differed considerably between the three wayfinding support tools. The tactile map afforded the most successful wayfinding and highest confidence. Wayfinding performance and confidence were lowest for the mobile application modality. The simplicity of use of a wayfinding tool is paramount for reducing cognitive load during wayfinding. In addition, information that does not match individual, user-specific information preferences and needs inhibits wayfinding performance. Current practice pertaining to the representation of digital spatial data only marginally accounts for the complexity of pedestrian human wayfinding across the gamut of visual impairment, blindness, and deafblindness. Robust orientation and mobility training and skills remain key for negotiating unexpected or adverse wayfinding situations and scenarios, irrespective of the use of a wayfinding tool. A substantial engagement of the deafblind community in both research and development is critical for achieving universal and equitable usability of mobile wayfinding technology.
1 Introduction
It is commonly understood that individual mobility enables humans to live healthy, sustainable, and fulfilling lives (Golledge and Stimson, 1997). A substantial part of human mobility consists of wayfinding, that is, the goal-directed cognitive process of negotiating movement and navigation towards a destination through the environment (Golledge, 1999; Dalton et al., 2019). People with disabilities, including those who are blind, deafblind, or who have low vision, constitute a sizable and growing minority of the general population (Courtney-Long et al., 2015). This minority continues to face significant barriers to equitable community inclusion and access to public resources, employment, education, and technology (Emerson et al., 2009; Hodge et al., 2013; Wilson et al., 2017; Carroll et al., 2018; Okoro et al., 2018; Clarke et al., 2019; Ehn et al., 2019; Liu, 2019).
As mobile wayfinding technologies have continued to proliferate, so has scholarly interest in technology-mediated pedestrian navigation, including research in the specific context of functional disability and visual impairment (Kane et al., 2009; Swobodzinski and Raubal, 2009; Giudice et al., 2019; Parker et al., 2020; Ponchillia et al., 2020). Despite significant advancements and wide dissemination of mobile technologies among the sighted population (Pew Research Center, 2015), technology designers, creators, and scholars have yet to fully unpack the specific accessibility and information needs of deafblind individuals (Griffin-Shirley et al., 2017; Parker et al., 2020).1 In addition, academic research on human wayfinding predominately subscribes to an artificial demarcation between outdoor and indoor wayfinding, with little change over the last decade (Giudice et al., 2010). Such separation does not apply to typical human travel (Kim and Lehto, 2013; Kray et al., 2013; Winters et al., 2015) and it is one of the factors that inhibits a consolidation of mobile wayfinding software, functionality, and data models for seamless wayfinding through outdoor, indoor, and transitional spaces (Vanclooster et al., 2016; Wagner et al., 2017; Yan et al., 2019). As it stands, few mobile wayfinding solutions consider the information needs of visually impaired, blind, and deafblind individuals, and the desired functionality remains fragmented (Swobodzinski and Parker, 2019).
Among the small number of experimental wayfinding studies that included visually impaired or blind participants (e.g. Connors et al., 2014a,b; Bai et al., 2017; Pissaloux et al., 2017), comorbid impairments, and hearing loss in particular, have received little consideration. This lack of empirical evaluations reflects the scarcity of empirical research studies in the specific context of deafblindness (Ehn et al., 2019; Wittich et al., 2021). Select exceptions to such omission are Ross and Kelly (2009), with two participants who used hearing aids to compensate for hearing loss, and Nicholson et al. (2009) who reported that one visually impaired participant had an unspecified additional disability. Also, Vincent et al. (2014) had four deafblind participants in their study on the effectiveness of two mobility devices (i.e., Miniguide and Breeze) and Parker et al. (2020) leveraged small focus groups with deafblind participants to better understand individual experiences pertaining to the use of mobile wayfinding technology under dual sensory loss. Most noteworthy is that none of the aforementioned studies 1. focused on the inclusion of deafblind participants and the assessment of their wayfinding behavior and 2. employed a cross-comparison among different categories of wayfinding support tools. As it stands, research on technology-mediated human wayfinding under consideration of dual sensory loss is characterized by a scarcity of empirical evaluations, established methodological approaches, and experimental protocols. With the case study presented in the paper we seek to help mitigate this scarcity.
Further relevant for our study is the construct of seamless wayfinding. Seamlessness in wayfinding research entails at the very least the integration of both indoor and outdoor spaces as a component of the experimental design and analysis (Vanclooster and De Maeyer, 2012). In that context, only few recent human-subject studies have explored seamlessness as it comes to individual wayfinding under consideration of visually impairment and blindness. Cheraghi et al. (2019), for instance, presented a smartphone application (CityGuide) that provided turn-by-turn instructions for routes with an indoor origin and an outdoor destination; thus, their routes spanned both indoor and outdoor spaces by design. Bai et al. (2019) reported on a wearable assistive device prototype that combined functionality pertaining to positioning, route calculation, and obstacle avoidance, which they assessed in both indoor and outdoor spaces. However, the experimental routes were separated into indoor and outdoor. A haptic navigation aid (LaserNavigator) supporting obstacle avoidance and distance estimation was evaluated by Röijezon et al. (2019) using separate indoor and outdoor routes. Furthermore, Meshram et al. (2019) assessed the performance of an electronic mobility device (NavCane) with obstacle detection and recognition capabilities with 80 visually impaired participants in well-controlled and separate indoor and outdoor settings. Similarly, Duh et al. (2020) evaluated a wearable wayfinding device (V-Eye) that employed a low-cost camera and computer vision for positioning, body orientation, and scene understanding/obstacle detection in indoor and outdoor spaces. As in Bai et al. (2019), Röijezon et al. (2019), and Meshram et al. (2019), the respective wayfinding routes devised by Duh et al. (2020) were situated either in indoor or outdoor environments, not indoors and outdoors.
In this paper, we report on a case study with an empirical, mixed-methods evaluation of the wayfinding experience of a deafblind adult pedestrian traveler in an urban environment (Aarons et al., 2012; Fetters et al., 2013). To that account, our collaborative research group of researchers and practitioners in geographic information science and Orientation and Mobility (O&M) devised an experimental procedure to capture and evaluate the deafblind participant’s wayfinding performance, confidence, and perceptions about his travel. Specifically, the participant was asked to complete a set of three unfamiliar pedestrian routes on an urban college campus using either a mobile wayfinding application, written directions, or a tactile map. The routes were designed to resemble real-world travel and to explicitly combine indoor and outdoor route segments and transitions between indoor and outdoor spaces. The case study allowed us to perform a comparative assessment of the participant’s wayfinding behavior in relation to the wayfinding support tool used.
The case study discussed in this paper is a part of a larger research project that aims to assess the degree of seamlessness of the wayfinding experiences of visually impaired or blind pedestrian travelers, with a particular focus on those who are deafblind, as it comes to negotiating realistic pedestrian routes. The project builds upon prior work of the lead authors on the use of mobile wayfinding technology by low-vision, blind, and deafblind users (Swobodzinski and Parker, 2019; Parker et al., 2020). Our focus in the paper at hand is on the description and discussion of the qualitative and quantitative factors, both observed and emerged, that informed the wayfinding behavior of the deafblind participant in our case study. The central research question that we address in our case study is: In which way do spatial information provisions afforded by a mobile app, written directions, and a tactile map inform the wayfinding performance and confidence of a deafblind individual?
2 Materials and Methods
2.1 Hypotheses
Prior research evidenced that perceptions on the usefulness of information on distal and proximal landmarks differed among individual travelers depending on the type of wayfinding task undertaken (Tom and Denis, 2003; Li, 2006; Norgate and Ormerod, 2012; Kettunen et al., 2013; Denis et al., 2014; Li et al., 2014; Nuhn and Timpf, 2017; Padmanaban and Krukar, 2017; Sato et al., 2019). In this context, Allen (1999) put forward the conceptual distinction between wayfinding tasks that focus on the exploration of an environment, a wayfinding explore (Allen, 1999), and those that are foremost concerned with reaching an unfamiliar destination, a wayfinding quest (Sato et al., 2019). Given the resemblance of our experimental design to a quest, we hypothesized that 1. the waypoint-centric information provided by the mobile application would most closely match the information needs of the participant for successful wayfinding and 2. the mobile application modality would afford highest route completion performance, that is, fastest completion times and highest satisfaction.
2.2 Study Location
In-person study activities took place at Portland State University (PSU), an urban public research university in Portland, Oregon (Figure 1). PSU originated in 1946 as an educational institution for veterans of World War II and was granted university status in 1969. PSU currently serves approximately 24,000 students2, making it the second largest public university in Oregon. Since 1964, PSU has been housing the Visually Impaired Learner (VIL) program, a graduate teacher training program for teachers of visually impaired, blind, and deafblind students. In 2016, the VIL program was extended by the PSU Orientation and Mobility program—a graduate preparation program aimed at the mitigation of the substantial shortage of Orientation and Mobility (O&M) specialists in the United States (Lawson and Parker, 2020; Schles, 2021). Since 2017, PSU has also been hosting Mobility Matters, an annual summit centered on interdisciplinary conversations by researchers, educators, practitioners, advocates, and members of the disability community at the nexus of accessible mobility, design, infrastructure, and transportation on university campuses (Parker, 2020).
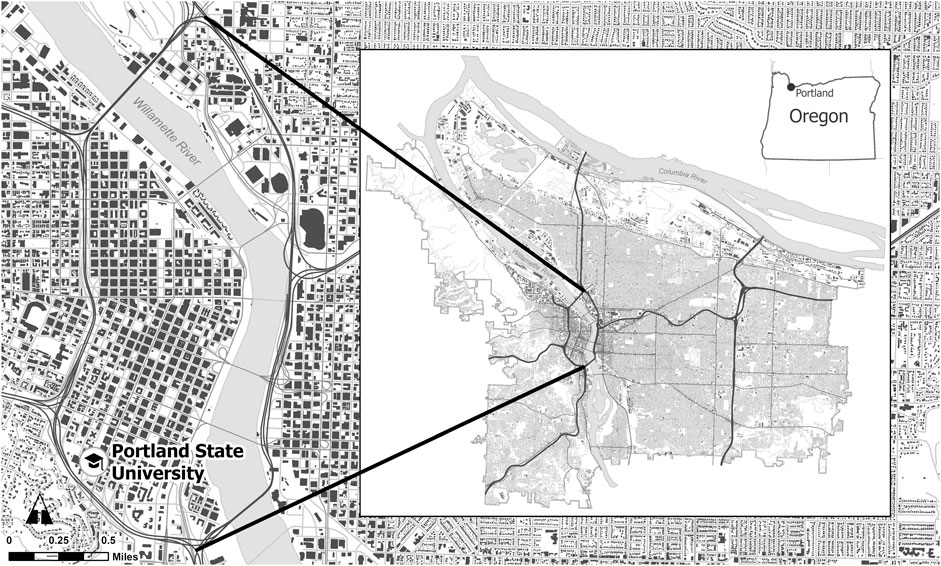
FIGURE 1. Overview map of the location of our wayfinding case study, the campus of Portland State University in the downtown neighborhood of Portland, OR.
PSU’s 49-acre campus is closely integrated with the surrounding urban environment of downtown Portland, including features such as major and minor roads, sidewalks, parking structures, pedestrian footways, and transit stops (i.e., light rail, bus, and streetcar). The central part of the campus consists of a designated pedestrian-only city park and greenspace (i.e., South Park Blocks) that is flanked by university buildings, with paved footways, sizable grassy areas, and trees. It is in the central area of the campus that we situated the routes for our wayfinding case study.
It is also noteworthy that a number of buildings on the PSU campus have been equipped with an array of Bluetooth Low Energy (BLE) beacons during a previous research study of team. In addition, research on wayfinding technology at PSU has been facilitated by the volunteered contributions of collaborators at the American Printing House for the Blind (APH), members of the research team, student volunteers, and members of the general public who digitized various floorplans of PSU buildings and annotated interior rooms and features of interest. The digital spatial data pertaining to these spaces and interior features, including BLE beacon locations and identifiers, have been made available on the OpenStreetMap (OSM)3 open data platform. OSM data for indoor environments is non-standard and requires an OSM mapping client capable of cartographically visualizing indoor features. One example is the OpenLevelUp web map client which can be accessed at the link provided in the footnote, centered on our study area at PSU (Lat. 45.6° N and Lon. 122.7° W).4
2.3 Participant
The participant for this case study was a college educated, self-employed 32 year old white male. Before the participant was invited to partake in the wayfinding experiment, he was asked to complete a 22-item screening survey (Data Supplement 1). Specifically, eligibility for participation in the study required the participant to self-identify as 1. being blind or having low vision, 2. having at least slight confidence pertaining to independent travel, and 3. having at least slight confidence in completing one third of a mile of travel without resting. The participant’s professional work includes the creation of mobility aids for individuals who are blind or have low vision. He reported being married and living with his partner. The onset of his visual impairment was at the age of 19, with Usher syndrome type II and retinitis pigmentosa as the underlying conditions. He self-identified as hard-of-hearing and self-estimated a hearing loss of 60–70 percent. He added that he is commonly using a small in-ear hearing aid5 which allows him to perceive regular conversations and environmental sounds. He also reported being legally blind, with recent rapid diminishing visual fields. He described his current visual function as having no clarity in the left eye and 2° in the right eye. He stated being able to locate large objects and bright lights in his central but not his peripheral vision. He is transitioning to using braille but uses screen reading technology to access print. He received formal O&M training, including guide dog mobility instruction. He also had experience using tactile maps and wayfinding technology which he described as little for tactile maps and much for wayfinding technology. In terms of travel, he reported feeling extremely confident about his ability to travel independently and to complete a route of a third of a mile without resting. He normally uses a guide dog as his primary mobility device and is competent in using a cane for his travels. For his participation in our study, the participant received a honorarium of $75 in the form of an electronic gift card at the conclusion of the research activities.
2.4 Study Design
Our study employed a convergent mixed-methods parallel research design. A convergent-parallel approach calls for the simultaneous collection and analysis of qualitative and quantitative data within two separate parallel tracks (Creswell and Plano Clark, 2017). Subsequently, the individual results are integrated and interpreted together (Edmonds and Kennedy, 2017). This mixed-methods approach was most conducive to the gathering and analysis of the observational quantitative and qualitative data on the participant’s wayfinding behavior.
The primary task for the participant was to complete three routes contained within the central areas of the PSU campus (Figure 2). The participant was not familiar with the PSU campus and the routes were unknown to him. Subsequently, the participant completed a semi-structured interview with questions on the participant’s perceptions on past wayfinding experiences (Figure 3). The participant signed individual consent forms for the screening survey, wayfinding experiment, and semi-structured interview. The consent form for the wayfinding experiment pointed out that our research aimed at better understanding individual experiences with and perceptions on indoor/outdoor travel in relation to wayfinding support tools.
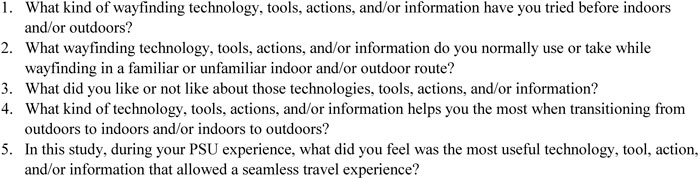
FIGURE 3. Overview of the questions for the semi-structured interview which we conducted with the participant shortly after having completed the wayfinding experiment.
The order in which each route were to be completed was determined through random assignment. For each route, the participant was asked to use a different wayfinding support tool among a selection of three: 1. written directions (Route B), 2. a tactile map (Route C), and 3. a mobile application (Route A; Nearby Explorer Online). The wayfinding support tools were randomly paired with each route so that each support tool was used for a single route and no more than one time. Throughout the wayfinding experiment, the participant was asked to use his preferred primary mobility tool which for the participant was a white, lighted mobility cane with a jumbo roller tip.
2.4.1 Routes
Given our study’s objective to assess realistic travel experiences, the routes in our experiment were designed to resemble routes taken by students to reach commonly sought destinations on the PSU campus. Furthermore, the routes that we devised incorporated best practice in O&M for indoor and outdoor pedestrian travel (Fazzi and Barlow, 2017). Specifically, each route afforded establishing an initial straight line of travel, parallel alignment and squaring off of one’s body to environmental features, shorelining of prominent boundaries between different surface types, walltrailing of interior and exterior walls, as well as leveraging proximal wayfinding landmarks on and along our experimental routes, as was deemed most appropriate by the joint assessment of the O&M specialists on our team. In addition, we aimed at a level of route complexity that we expected would allow a visually impaired, blind, or deafblind individual to complete the experimental protocol in a single, half-day session.
Considering commonly employed metrics of route complexity (Chang et al., 2020; Fernando et al., 2021), the routes were closely matched to be of a comparable length and configuration in terms of the total number of turns. To that account, the three routes averaged a length of 463 feet and nine total turns, with a variation among the routes of ± 10 feet and ± one turn. We purposefully omitted street crossings in favor of travel along pedestrian-only footways on the PSU campus for two reasons. First, adding street crossings with car traffic would increase the risk to participants with no apparent benefit to the study. Second, the inclusion of street crossings would necessitate deviating from the central area of the campus towards its fringe, which would increase overall route lengths. Having said that, all three routes included crossings of wide pedestrian footways whose widths were comparable to those of single-lane streets (i.e., about 20 feet).
All routes originated at an outdoor location and terminated at an indoor location. For Route A the participant traveled from the entrance of the building that houses the PSU Disability Resource Center (i.e., Smith Memorial Student Union) to the Student Accounts desk in the adjacent building (i.e., Fariborz Maseeh Hall). Route B originated at a durable outdoor landmark (i.e., concrete garbage can) and led to the booth of a cafe in the PSU Branford Price Millar Library. Route C originated at another durable landmark (i.e., outdoor lamppost) and led to the door of a conference room in Fariborz Maseeh Hall. Figure 4 depicts the conceptualized course of Route A and Table 1 summarizes the main characteristics of the three conceptualized routes.
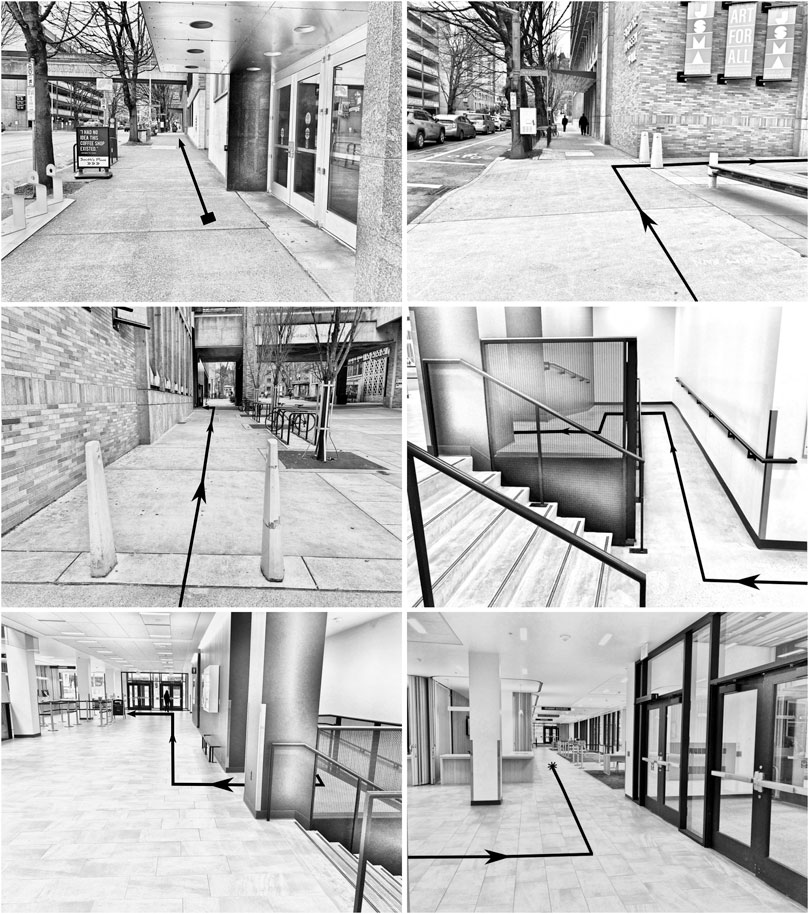
FIGURE 4. Sequence of photos (top to bottom, left to right) illustrating the conceptualized course of Route A from its origin at the entrance of Smith Memorial Student Union to its destination at the Student Accounts desk in the Fariborz Maseeh Hall building.

TABLE 1. Characteristics of the three wayfinding routes that we asked our case study participant to complete in terms of overall length, the length of indoor and outdoor segments, total number of turns, and the number of right-angled and oblique turns.
2.4.2 Wayfinding Support Tools
The three different types of wayfinding support tools were prepared for each route in advance of the experiment to be at hand when required. The written directions were devised by O&M specialists on our team, following O&M best practices. More specifically, the written route descriptions were comprised of a granular list of O&M action steps and descriptive information about the route to be travelled, as well as information on landmarks coincidental with or proximal to the route. The written route descriptions for the three routes also functioned as the route preview scripts which were read by the facilitator to the participant for each route. Hence, for Route B, the written directions were used as both the route preview script and wayfinding support tool. The route description for Route B is provided in Figure 5.
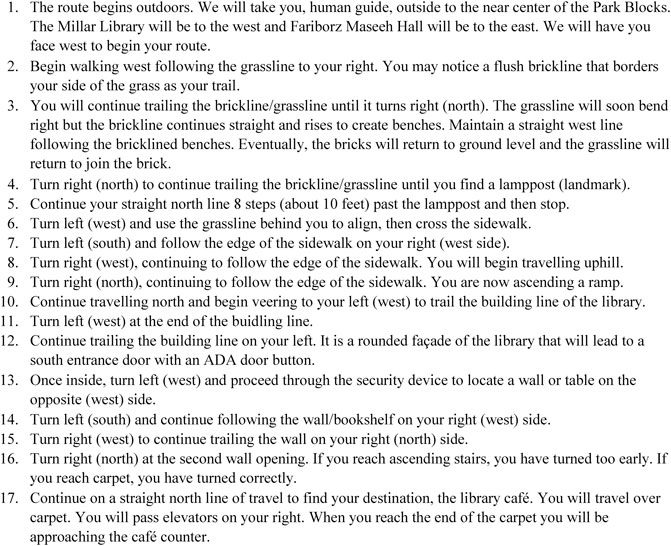
FIGURE 5. Route description for Route B that was read to the participant during the route preview phase. Subsequently, a digital file of the route description was sent to the participant through email for use during the wayfinding experience using Apple VoiceOver on the participant’s iPhone.
The tactile maps were created with simplicity and legibility in mind. We used duct tape, puff paint, and braille labels to convey the layout of a route as well as the locations of named landmarks, for instance, a large tree, a billboard, and entrance doors, or a wall. During the route preview period, the participant was allowed to familiarize himself with the tactile map as the researcher read the description for Route C aloud. During that time two elements were added to the tactile map (representing a garbage can and a public bench) using crafting materials (Wikki Stix) at the request of the participant (Figure 6).
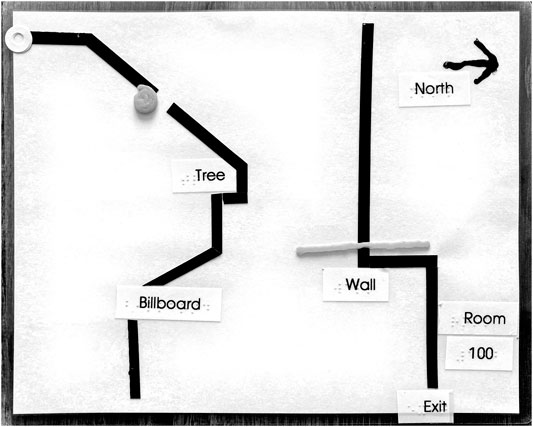
FIGURE 6. Representation of the tactile map that was provided to the participant for Route C. The outdoor segment of the route was captured on the left side of the tactile map and the indoor segment on its right. The tactile map was letter-sized and crafting materials representing a garbage can and a public bench were added to the map on the prompt of the participant.
For the mobile application (mobile app) modality, members of the research team identified suitable indoor waypoints for each route. The waypoints were subsequently incorporated as spatial data into the mobile app in order to be selectable through the app’s regular user interface. For Route A (mobile app modality), six waypoints were created in the Fariborz Maseeh Hall building. In terms of user interaction with the app during wayfinding, waypoints were selected one at a time, in sequence of encounter along the route. This approach was motivated by the mobile app’s capability of alerting the user through vibration when the phone was pointed in the direction of a selected waypoint. For best performance, the cellphone needed to be held in front of the body, in parallel alignment with the ground. Determination of the position of the user in relation to surrounding features in the app was leveraging GPS in outdoor areas and BLE beacons in the indoor areas. In addition, the app provided audio information on points of interest in the surrounding area. Figure 7 shows the locations of BLE beacon locations and waypoints for Route A in Fariborz Maseeh Hall.
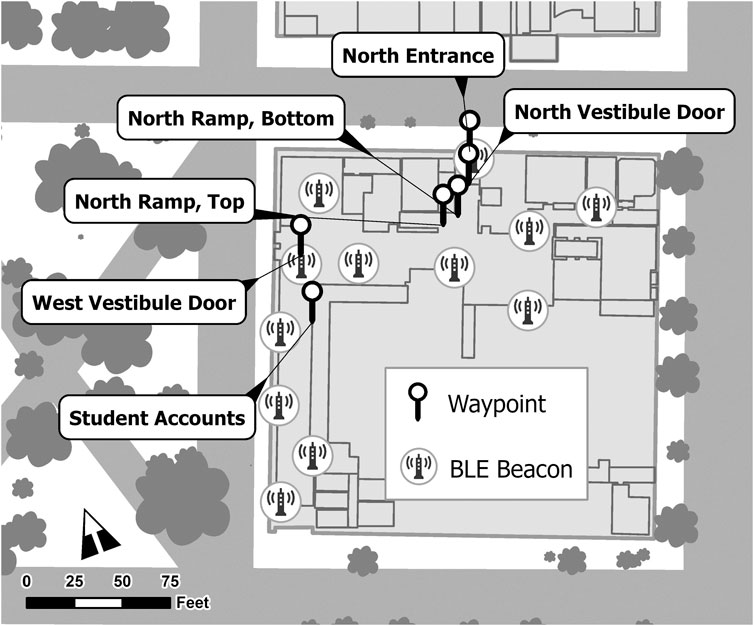
FIGURE 7. Map of the location of Bluetooth Low Energy (BLE) beacons as well as the name and location of waypoints for Route A in the Fariborz Maseeh Hall (FMH) building. The Student Accounts desk and a conference room in FMH served as destinations for two of our routes (i.e., Route A and Route C; not displayed).
2.5 Procedure
The participant was asked to join the members of the research team on the PSU campus in the vicinity of the travel routes that we devised. The core research team for the experiment consisted of three researchers whose respective responsibilities were to 1. function as the facilitator and main point of contact for the participant throughout the wayfinding experiment, 2. capture the participant’s wayfinding behavior using video recording equipment, and 3. collect observational data on the participant’s wayfinding behavior on experiment-specific data collection forms. The random assignment of routes and wayfinding support tools resulted in the participant first completing Route B using written directions, then Route C using a tactile map, and finally Route A using the mobile app. We instructed the participant to seek assistance from the facilitator during wayfinding only if necessary. We also advised the participant to vocalize his thoughts during wayfinding (i.e. Fonteyn et al., 1993, think aloud method).
Each route was introduced during a route preview period before route travel began. During the route preview, route descriptions with step-by-step wayfinding instructions were read to the participant by the facilitator and discussed until the participant indicated that all of his questions had been answered and that he was ready to start. At the end of the route preview, the participant was asked two questions on past experience with the respective wayfinding support tool: 1. Have you ever used this type of wayfinding support tool before (binary; yes/no)? 2 [If yes] How often have you used this type of support tool before (ascending 5-point numerical scale; one through 5)? Upon completion of each route, the participant was asked four additional questions on confidence and usefulness of information during wayfinding: 1. How useful was the preview for navigating the route? 2. How confident did you feel navigating the route? 3. How useful was the in-route information while navigating the route? 4. How confident were you in navigating the indoor/outdoor sections of the route? All post-route questions were answered on an ascending 5-point numerical scale, with values ranging from 1 to 5.
Before the preview for Route A (mobile app modality), the participant was asked to download the app (Nearby Explorer Online) and install it on his phone. Subsequently, the participant was given an approximately 5 min-long introduction on how to use the app. More specifically, the participant was shown how to adjust in-app settings pertaining to haptic or audio feedback during wayfinding. He was also assisted in adding the six waypoints for Route A to the list of favorites within the app for faster access during wayfinding. In addition, the participant was instructed on how to set and clear waypoints in the app. In this context, it is noteworthy that setting a new waypoint during wayfinding required that the facilitator approach the participant when a waypoint was reached to inform the participant of the name of the next waypoint. The participant would then clear the reached waypoint in the app, search for the next waypoint based on name, and set the app to the new waypoint. We considered these exchanges between the facilitator and participant to be necessary, given the functionality of the app.
For Route B (written directions modality), a digital copy of the route description was sent to the participant through email just prior to the start of the travel. The participant then used his personal cellphone (iPhone 6s Plus) to access the route descriptions through Apple VoiceOver and a Bluetooth device connected to his hearing aid, as needed. The participant also used his personal cellphone to run the mobile app that was used for Route A. Overall, the wayfinding experiment took 3 hours to complete and was followed by the hour-long, semi-structured interview on the participant’s perception and reflection on the experiment and indoor/ourdoor wayfinding. A break for lunch was provided in between the wayfinding experiment and the interview.
2.6 Analytical Process
The data that we collected in our case study were as follows: 1. responses to pre-route and post-route questions, 2. responses to questions during the semi-structured interview, and 3. video recording of the participant’s wayfinding behavior. The video recordings of the participant’s wayfinding behavior constituted the major source for the assessment of the participant’s route completion performance. An initial review of the video recordings allowed us to identify an inventory of wayfinding performance metrics that could be extracted from the video recordings, specifically: 1. overall route completion time, 2. number of wayfinding errors, 3. overall duration of recovery from errors, 4. number of facilitator interventions, and 5. number of questions by the participant to the facilitator. We conceptualized errors as an apparent deviation from the route by the participant which might or might not have been self-corrected by the participant. Interventions, on the other hand, were wayfinding incidences during which the participant deviated from the route to an extent that reestablishing orientation without guidance from the facilitator was unlikely. In such cases, the facilitator would stop the participant to clarify the situation and, if necessary, lead the participant to the last decision point or landmark using human guide technique. As a consequence, counts of interventions were subsumed in the count of wayfinding errors.
After we established our inventory of wayfinding performance variables, three members of the research group proceeded with the coding of the video recordings, with a focus on the quantification of the wayfinding performance metrics. This process included the separate review and coding of the video recordings by each researcher (Data Supplement 2). The individual results were then compared among the three researchers. In the case of disagreement pertaining to a time stamp or count of a coded event, a majority approach was adopted in that convergence among two researchers was sufficient to resolve the disagreement. In addition, small differences (of a few seconds) between time stamps of the three researchers were accounted for by the averaging of the time stamps. The averaged time stamp was then recorded as final. In terms of qualitative data, the semi-structured interview as well utterances by the participant during wayfinding were transcribed by one member of the research team and then edited by a second. The transcriptions were then coded and themes identified by the three researchers individually. The three researchers then compared and discussed the individual result in order to establish common themes. The lead authors then reviewed and validated prior findings and the final outcomes of this process are presented in the following section.
3 Results
3.1 Route B
As per the results of a random assignment, the first route completed by the participant was Route B. The wayfinding support tool was written directions which the participant accessed through Apple VoiceOver on his personal cellphone. The route description for Route B is provided in Figure 5 and the three observed routes that were traveled by the participant are presented in Figure 8. The participant held the cellphone in his left hand and his mobility cane in the right. Throughout the route, the participant used constant contact, shorelining, and touch-and-drag cane techniques to navigate.
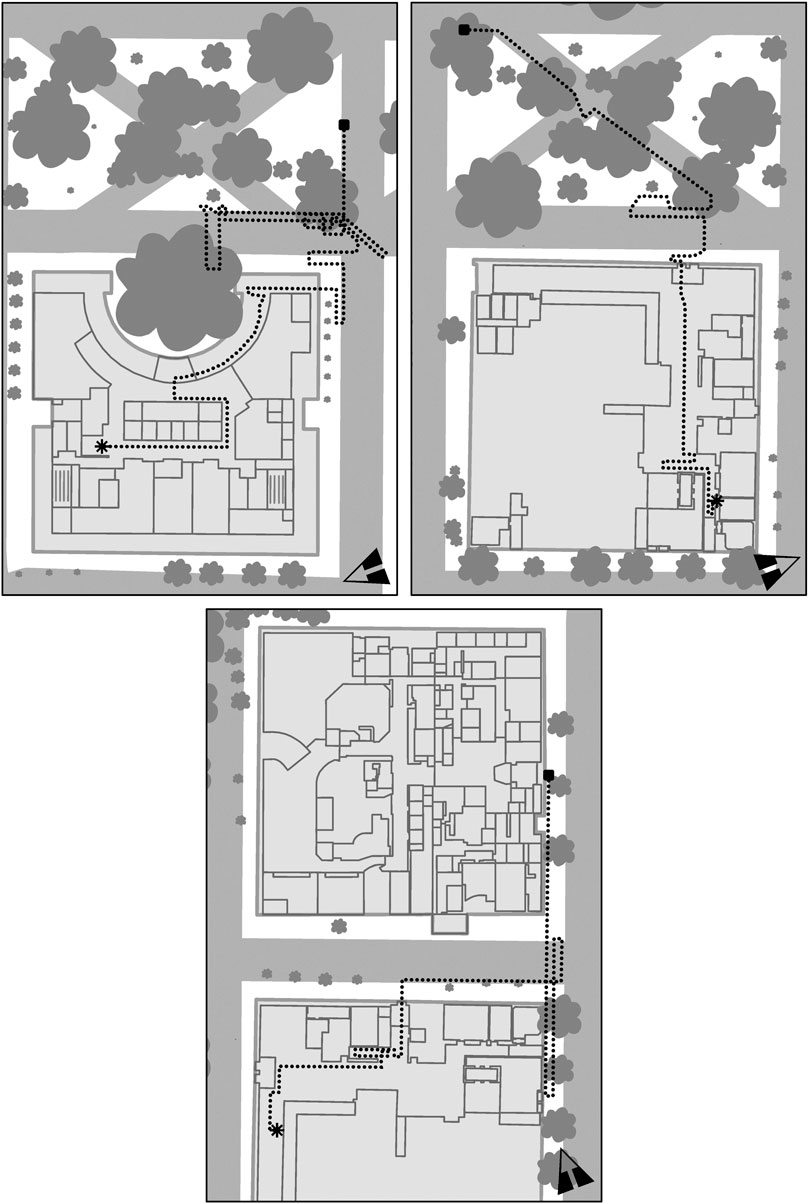
FIGURE 8. Maps of the observed routes that were taken by the participant during the wayfinding experiment. The routes are presented in the order of completion, with Route B (modality: written directions) shown at the top left, Route C (modality: tactile map) at the top right, and Route A (modality: mobile app) at the bottom.
The duration of the route preview for Route B was 5:06 min during which the facilitator read the 17 sequential route directions aloud. The participant did not ask for any of the instructions to be repeated. The participant stated that he had not used written directions for wayfinding before.
The completion time for the route was 14:19 min. During travel, seven errors occurred of which four were self-corrected and three required intervention by the facilitator. Two of the interventions had the facilitator use human guide technique to reposition the participant. Verbal instructions were employed during the third intervention. All of the errors occurred in the outdoor segment of the route in the general area of the first turn and pedestrian footway crossing on the route. The duration of errors and recovery amounted to 7:05 min, for a completion time of 7:14 min with errors disregarded. The participant also directed two questions at the facilitator.
In the post-route survey, the participant rated the usefulness of the route preview as a two and the usefulness of the in-route information as a 5 (on an ascending 5-point numerical scale with values one through 5). In addition, he rated both his confidence in navigating the route as well as his confidence of navigating indoor/outdoor sections of the route as 4.
3.2 Route C
The second route that was completed by the participant was Route C. The wayfinding support tool that was used was a tactile map (Figure 6). The participant held the tactile map with his left hand pressed against his chest and traced the tactile route while progressing on the physical route. His mobility cane remained in his right hand. Throughout the route, the participant used constant contact, shorelining, and touch-and-drag cane techniques to navigate.
The route preview time for Route C was 7:27 min. Before the beginning of the route preview, the participant was able to explore the tactile map for 1:10 min. During the route preview, the facilitator read aloud the 19 sequential route direction steps for Route C. The participant asked to have seven of the step repeated to him. The participant stated that he had used a tactile map for wayfinding before and scored the frequency of prior use as one out of 5.
The completion time for the route was 9:14 min. During travel, three errors occurred, with the participant walking beyond a decision point twice and turning into the wrong direction once. In these three situations, the facilitator intervened with verbal instructions that resolved the errors. Two of the errors occurred outdoors and one indoors. The duration of errors and recovery amounted to 3:31 min, for a completion time of 5:43 min with errors disregarded. The participant also directed four questions to the facilitator.
In the post-route survey, the participant rated the usefulness of the route preview as a four and the usefulness of the in-route information as a 5. In addition, he rated his confidence in navigating the route as a three and his confidence of navigating indoor/outdoor sections of the route as a 5.
3.3 Route A
The third route that was completed by the participant was Route A. The wayfinding support tool used was a mobile app (Nearby Explorer Online) with functionality to track waypoints and other points of interest. At the beginning of the travel, the phone remained in the participant’s left coat pocket. Following technical issues with the localization of the first waypoint and intervention by the facilitator, the participant held his cellphone in his left hand throughout the travel, screen up and parallel to the ground. His mobility cane remained in his right hand. Technical inconsistencies pertaining to the localization of the first waypoint at the entrance of Fariborz Maseeh Hall continued until the entrance was reached based, with the facilitator providing verbal instructions. Throughout the route, the participant used constant contact and shorelining techniques to navigate.
The route preview time for Route A was 4:24 min. Before the beginning of the route previews, the participant was given an introduction on how to use the app for 4:18 min. During the route preview, the facilitator read aloud the 11 sequential route direction steps for Route A. The participant did not ask for any of the instructions to be repeated. However, when the participant was informed that waypoints would have to be manually selected in the app, he asked for clarification on the total number of waypoints, of which there were 6. The participant stated that he had used a mobile app in combination with BLE beacons for wayfinding before and scored the frequency of prior use a two out of 5.
The completion time for the route was 17:07 min. During travel, seven errors occurred, three of which were attributable to technology-related inconsistencies pertaining to the localization of the first waypoint during the outdoor segment of the route. Another error originated in the misinterpretation of information from the app by the participant and was self-corrected. This sequence of errors during the experiment we further elaborated on in the discussion section. Of the seven errors, four were addressed through interventions by the facilitator in the form of verbal instructions. The duration of errors and recovery amounted to 7:04 min, for a completion time of 10:03 min with errors disregarded. The participant also directed four questions to the facilitator.
Table 2 summarizes the quantitative results for the observed wayfinding performance of the participant and Table 3 those for the pre- and post-route survey responses of the participant.
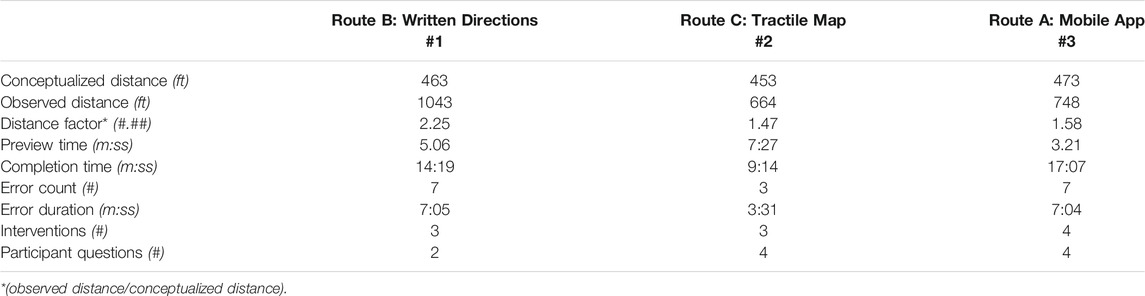
TABLE 2. Route performance measures of the observed wayfinding behavior of our case study participant for the three routes. All observed routes were considerably longer than those that we conceptualized, as conveyed by the distance factor that is provided in the table.
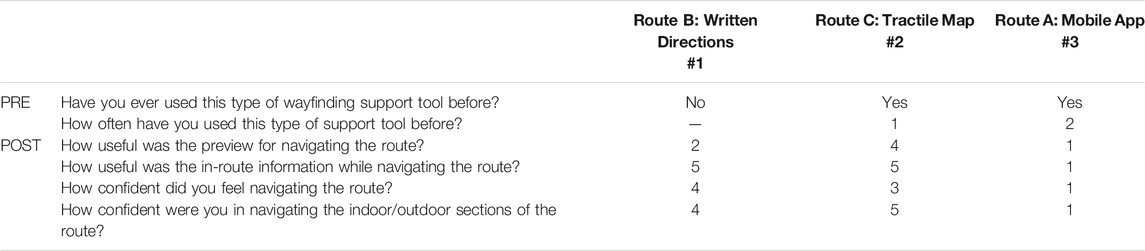
TABLE 3. Responses of the participant to the pre- and post-route questions for each of the three wayfinding routes.
3.4 Interview
During the interview, the participant mentioned Google Maps, Apple Maps, Seeing AI, and AIRA as wayfinding technology that he used in the past, with AIRA often employed when needing to locate a bus stop. In this context he expressed the desire for wayfinding technology to be wearable and inconspicuous, with a user interface that provides feedback in a simple and non-obtrusive way that does neither muffle nor draw attention away from one’s environmental perception and awareness. In terms of route navigation, turn-by-turn instructions should be succinct and be sent at decision points using in-time prompts that indicate the correct direction of travel. He also mentioned being a user of Sunu Band, a small device (“smart band”) that is worn on the wrist which provides obstacle detection with haptic feedback to the user when objects are detected in the wearers path.
The participant also pointed out that the setting of waypoints in the mobile app was non-intuitive to him, in that adjustments to waypoints were too frequent and cumbersome. Considering that his vision continued to weaken, he expressed the personal need to prepare himself, in terms of skills and training, for the time when no visual perception will remain. This perspective profoundly informs his evaluation of wayfinding technology as well as his individual efforts as an inventor of wayfinding technology. As one sense weakens, the other senses become more critical to sustaining mobility.
A regret expressed by the participant was that he did not pay attention to the route preview description for Route A, given that his expectation was that the mobile app would afford intuitive, seamless wayfinding through the outdoor and indoor spaces. He pointed out that transitions from outdoors to indoors and vice versa are often challenging for him in that the differences in lighting and light intensity can render his remaining vision temporarily non-functional. As a consequence, he mentioned that he tends to close his eyes when transitioning from outdoor to indoor or indoor to outdoor, which allows him to focus on the information from the other senses. He added that he is able to navigate better when keeping his eyes closed. He also related that his walking speed was a good indicator of his confidence during travel, which he expected to be visible in the video recordings, with faster walking speed indicating higher confidence.
As for his experience of indoors and outdoors spaces during the experiment, he commented on the differences in information granularity that was provided by the app in terms of points of interest. More specifically, indoors the density of points of interest appeared to be much higher, with points of interest such as bathrooms, stairs, ramps, and counters, compared to the apparent sparseness that he perceived for points of interests outdoors. In addition, he pointed out the large number of instructions for Route B in particular, which he approached by reviewing one or two instructions at a time during travel.
Furthermore, he commented on the importance of information on shorelining/trailing of salient configurations of environmental features for successful wayfinding. In addition to commonly facilitating his daily travel, shorelining the edge between grassy areas and paved pedestrian footways, as identified in the route descriptions, enhanced his wayfinding during the experiment. On the other hand, he pointed out that a decision point/landmark identified in the route description for Route B (written directions modality) did not match his visual perception along one part of the route. There he was able to see a lamppost ahead but did not perceive another lamppost to his side (which functioned as a prominent landmark in the route description; see step 4 in Figure 5), even though he reportedly established contact with the latter using his cane.
In the context of landmarks, he spoke about his expectation of being able to sense the shade of a tree which was functioning as a proximal landmark signalizing a turn in the route description for Route C. Here, he was not able to determine that an environmental feature was indeed the tree in questions until he proceeded to establish contact with the tree (by hugging it). As for the wayfinding support tool that fostered best understanding and instilled highest confidence in him during wayfinding, the participant stated that the tactile map was best for him, followed by the written route descriptions that he accessed through Apple VoiceOver. As a part of his concluding remarks, he pointing out that he preferred route descriptions with granular distance estimates between decision points, and specifically distance estimates provided in feet rather than step counts.
3.4.1 Select Quotations
Quote 1: “But I think where I did really well […] is on the tactile maps. Which is not something that I’ve used a lot […] I could follow the direction of the map and feel the environment change. I kinda just put the map on my chest [gestures like he’s holding the map] and felt and followed with my finger around it. I was able to adapt it to what I needed [. ] So yeah, I think out of the three options that I had, the one that I had the best understanding and comprehension was the tactile maps. Because I could feel it, and then do it.”
Quote 2: “I was relying so heavily on the technology. I didn’t really pay attention to the instructions because I was thinking the technology was going to do all of the work for me [. ] I totally gave up on everything and put everything into this [shakes phone] and that was a bad idea. But that was what I was going for … to get from point A to point B without having to think about it.”
Quote 3: “And this is my biggest pet peeve, is that I’m hard-of-hearing and I’m legally blind and […] probably in the next 10 years I won’t be able to see […]. So I’m trying to prepare everything that I have to be as successful as possible. So when I build technology, I’m thinking about how can I use the technology without interfering with my other five senses […] I already have two senses not working, but we’ve got three senses that are working.”
Quote 4: “Because I thought it was going to be like a big tree [gestures with arms out wide] with wide branches and I was gonna have some shade. I was going to feel the shade. It all comes down to that feeling. I had an expectation of how I was going to feel that turn, well that wasn’t the case.”
4 Discussion
The route performance measures (Table 2), post-route survey responses (Table 3), and responses in the interview indicate sizable differences in performance, participant confidence, and perceived usefulness of the respective wayfinding tools. Whereas the written directions and tactile map indicated moderate to high levels of wayfinding confidence and usefulness of in-route information, the mobile app scored lowest on these self-reported measures. In terms of observed route completion measures, Route A (i.e., mobile app modality) took considerably longer to complete (at 17:07 min), required the highest number of interventions (at four), tied for highest number of total errors (at seven), and tied for largest number of participant questions (at four). A number of usability and technical inconsistencies were apparent in the mobile app experiment. First, the app did not indicate the correct direction to the first waypoint. Second, the app required waypoints to be manually set. At the same time, Route A was comprised of the fewest turns among the three routes and did not include any oblique turns, which suggests that it was the least complex route.
The tactile map, on the other hand, afforded the fastest completion time (at 9:14), with three interventions comprising the total number of errors. Noteworthy here is that Route C in which the tactile map was used was the only route with oblique turns, suggesting comparatively higher route complexity. Also, the participant stated that he only had very little experience with tactile maps as wayfinding support tools. Yet, the tactile map scored highest in regard to confidence in navigating indoor/outdoor sections, second highest for overall confidence, and highest for the usefulness of the route preview and highest for the usefulness of in-route information, as a tie with written directions. These numbers as well as the responses of the participant in the interview (Quote 1) reinforced that the tactile map was the wayfinding tool that afforded highest wayfinding performance, confidence, and satisfaction.
Scholarly research has long established that tactile maps are a powerful means for communicating spatial information and acquiring spatial knowledge in the absence of vision (Franks and Baird, 1971; Armstrong, 1973; Andrews, 1983; Kennedy, 1983; Golledge, 1991; Espinosa et al., 1998). The reflection by the participant speaks to the intuitiveness of the haptic interaction with the tactile map and its alignment with the information needs of the participant. In turn, it fostered the highest wayfinding performance and confidence. The tactile map was the only wayfinding support tool that captured the overall layout of the route and in that conveyed the greatest amount of spatial information among the three wayfinding tools, and in particular the mobile app. In addition, the information on the tactile map was structured into outdoor and indoor sections and prepared at a scale that made the spatial configuration of the route accessible to the participant on an as-needed basis. Yet, extended trip distances and errors were apparent for all three modalities, including the tactile map. Robust orientation and mobility skills allowed the participant to negotiate adverse wayfinding situations.
One important insight emerged from the connections between the quantitative and qualitative data that we collected: The importance for human-subject researchers, in general, and those studying the lived experiences of visually impaired, blind, and deafblind individuals, to carefully and meticulously consider participant expectations in their research designs. As the participant shared at various times during the interview, his expectations towards the mobile app were overly high. This was also evidenced by the high walking pace of the participant at the beginning of the mobile app experiment which, as he also stated during the interview, was a physical indicator of high wayfinding confidence. As a result, he did not pay attention during the route preview for Route A (Quote 2) and also did not ask questions about the route, which manifested in the shortest duration of the route preview among the three routes. In the participant’s own words:
Considering that the participant put his phone away as soon as he started walking, there must have been a presumption that the app offered capabilities that would 1. calculate a route to the destination and 2. provide route instructions through Bluetooth audio. However, the mobile app, as employed by us in the case study, offered neither since it was solely configured to provide haptic feedback (vibration) when the phone was pointed in the direction of a waypoint. To the best of our abilities, high expectations toward the mobile app were not instilled in the participant by the researchers but neither did we have provisions to (pro)actively manage them.
A possible explanatory factor for the high expectations by the participant might have been his professional background in the development of mobility aids for visually-impaired individuals. The participant also stated that his progressing vision loss greatly motivated his personal efforts towards preparation for the time when his residual vision might not be available to him anymore—and (wayfinding) technology that supported his preparatory efforts were an important and desired element. The participant’s evaluation of wayfinding technology was therefore deeply personal, as he conveyed in the interview (Quote 3), which contextualizes the low post-route scores for the mobile app as a result of technical issues, related frustration, and disappointment due to unmanaged expectations.
A crucial and seemingly latent problem that hinders seamless wayfinding is the disjoint between the very granular, multi-sensory experience of wayfinding under consideration of (dual) sensory loss versus the capacities of spatial data models to account for the complexity of such travel. Common practices in O&M evidence that high fidelity in terms of geometric accuracy and representation is necessary to adequately account for configurations and relationships of environmental features typically used in wayfinding—specifically the prominent role that shorelining of the grass/pavement edge had in support of the wayfinding of our participant. In conjunction with the everyday use of shorelining and walltrailing in O&M instruction and training, a sophisticated and faithful representation of features affording shorelining or walltrailing would likely catalyze the development of innovative mobile routing solutions for visually impaired, blind, and deafblind travelers. As it stands, digital representations pertaining to urban features that afford movement of vehicles and humans (e.g., streets, sidewalks, paved footways) are captured by geometric networks of interconnected lines which commonly represent the center of surface features (i.e., centerlines).
Using a typical example from our case study, our wayfinding instructions leveraged, based on O&M practice, difference in textures of adjacent surface features: wide concrete footway with brick lined banding at the perimeter adjacent to grassy areas. Each of these surfaces affords tactile and textual saliency that was instrumental for devising robust wayfinding instructions suitable for a visually-impaired, blind, or deafblind pedestrian traveler (who foremost uses a long cane). It is interesting to note that the tactile map did not capture any of the configural complexity of surface features apart from the shape of the route presented at a suitable scale. Yet, our route descriptions leveraged salient separations between horizontal surfaces for shorelining as well as vertical surfaces for trailing to the extent that these aligned with our conceptualized routes. It stands to reason that the wayfinding performance of the participant would have been worse without indications of environmental features and configurations that are conducive to shorelining and/or walltrailing.
In essence, what is missing are digital spatial repositories with faithful representations of surfaces in three-dimensional space that integrate outdoor and indoor environments. Such repository would allow us to develop algorithms for the computation of the most suitable routes that consider the specific information needs and expectations of visually-impaired, blind, and deafblind individuals. As such a repository would grow, so would the possibilities for the planning, preview, and optimization of pedestrian routes for blind and low-vision travelers well beyond the confines of a small area on an urban college campus. LiDAR technology paired with computer vision and object recognition promises the means of creating digital high-fidelity volumetric representations of indoor and outdoor spaces (Li et al., 2015). As these technologies become more widely disseminated and accessible, we expect that innovation in pedestrian routing and wayfinding under specific consideration of individual abilities, information needs, and preferences will follow.
A related aspect is the conceptualization of landmarks. Much is known about the importance of landmarks for orientation, maintaining a sense of direction, forming mental representations, and integrating environmental knowledge, in particular as it comes to individual experiences of space (Passini and Proulx, 1988; Golledge, 1992; Raubal and Winter 2002; May et al., 2003; Tversky, 2003; Ishikawa, 2013; Rodriguez-Sanchez and Martinez-Romo, 2017; Dong et al., 2020; Fernando et al., 2021). A striking example in our case study that speaks to the highly idiosyncratic role that landmarks play in human wayfinding is that of a tree that was identified as a landmark indicating a turn on Route C. In this context, the participant pointed out that he failed to perceive the tree since he was not able to feel the tree’s shade, as he was expecting (Quote 4). Remarkable about this is that the expectation of the participant towards the tree defies simple conceptualization of landmarks and showcases a scenario in which the saliency of the tree as a landmark is both cognitive and structural (Sorrows and Hirtle, 1999; Klippel and Winter 2005; Yesiltepe et al., 2021), in that it is informed by the cognitive expectation of the participant and the structural elements of the tree interacting with the Sun. Here, again, a complex digital representation of the tree in conjunction with a model of the Sun would be required to capture and instrumentalize such complex expression of saliency within computational solutions to pedestrian routing.
4.1 Recommendations
Based on the experience gained from our in-depth engagement with the participant in our case study, we are able to provide a number of recommendations that would provide for incremental improvements upon our experimental protocol and allow for the adoption of our research design for the assessment of the wayfinding behavior of a larger number of deafblind participants. Foremost, we strongly urge mobile app developers to carefully consider and incorporate the information needs and user interface requirements of deafblind individuals in their software. Specifically, relying on operation system functionality for access to the user interact is mostly limited to audio feedback, and we found that support for mobile braille displays is lacking in terms of both usability and usefulness. For example, an observation by our group during testing revealed that we were able to connect a braille display to researchers’ cellphones and interact with the mobile app. However, no apparent functionality was evident that would allow for the customization of the information provided. This resulted in a stream of information being sent to the braille display, with the tactile information refreshing too rapidly to provide acceptable usability. Until this shortcoming of mobile technology is addressed, we expect that the inclusion of deafblind participants who rely on tactile forms of communication (e.g., pro-tactile sign language and braille devices) would have to be considered as a separate wayfinding modality due to the constraints presented by the user interface and the diminished user interaction experience.
In terms of our experimental protocol, we also have a number of recommendations for amendments to the screening procedure and data collection approach that we presented. First, we used written route descriptions that were detailed and, at times, entailed whole sentences, multiple instructions, and/or consisted of a relatively large number of words. These instructions were then read to the participant through operation system-level voiceover functionality. Even though this user interaction allowed the participant to replay and go back and forth between instructions, the participant remarked at one point that the information could have been shortened. Hence, in order to lower the cognitive load that the processing of verbal route descriptions during wayfinding induce, we suggest the use of shortened route descriptions during route travel, with fully worded route descriptions reserved for route preview.
Furthermore, our data analysis workflow relied heavily on the review and analysis of the video recordings of the participant’s wayfinding behavior. As discussed before, this process entailed, among other steps, the extraction of routes traveled and wayfinding error committed, including the start and end times of errors with annotations of observed reasons for errors and, where applicable, interventions by the experimenter. This a-posteriori extraction of key data from the video recordings was facilitated by a structured, consensus-based process that involved no less than four researchers. As one considers scaling the data collection and subsequent analysis to a larger number of participants, we suggest that key data be captured during the experiment so that they are available for further processing and analysis more immediately. The video recordings could then function as a repository with which the captured key data could be checked and validated instead of the video recordings serving as the main data source.
Lastly, based on our experience conducting the case study, we suggest that researchers who are interested in adopting our experimental approach consider including further assessment of individual-level characteristics pertaining to hearing acuity, spatial environmental ability, and extent of O&M training. Whereas the screening survey that we employed in the case study captured granular self-reported information on the participant’s visual acuity, the participant’s hearing ability, extent of O&M training, and spatial environmental ability were solely captured by binary or short single-response questions. Self-report measures are readily available for hearing ability (Hickson et al., 1999), spatial environmental ability (Hegarty et al., 2002), and O&M training (Cmar, 2015). Our in-depth interaction with the participant in the case study provided us with information on these aspects beyond the screening survey. As future data collection efforts with larger numbers of participants are completed, a standardized means of collecting this information should be utilized to inform a robust analysis of participants’ characteristics in relation to wayfinding performance.
4.2 Limitations and Future Directions
In this paper we presented the results from a mixed-method analysis of the wayfinding experience, perception, and performance of a deafblind participant in an experiment on individual wayfinding on an urban college campus. Overall, our results provide contrary evidence for the two hypotheses stated earlier in the paper, specifically that 1. the waypoint-centric information provided by the mobile app most-closely matches the information needs of a deafblind participant and that 2. the mobile app affords fastest completion time and highest satisfaction by a deafblind participant. Given our findings, the very scarcity of information provided by the mobile app informed the low wayfinding performance and confidence of the participant.
The omission of provisions in our research protocol for the management of the expectations of the participant towards the mobile app introduced the possibility that the framing of our research study in the context of seamless indoor/outdoor wayfinding contributed to the participant’s elevated expectations. Having said that, conclusive answers will require the expansion of our research to a broad representation of visually-impaired, blind, and deafblind participants. In this context it is important to note that the case study framework that we discussed in this paper allowed us to engage with the participant and related empirical research in an in-depth manner. As we anticipate an expansion of our study and data collection to larger numbers of participants, we do not believe that such an in-depth treatment would make the inclusion of a large numbers of participants feasible without a great expansion of our research group’s capacities. However, the insights gained from or case study and the recommendations that we discussed in this paper facilitate the extension of our research protocol to larger sample sizes towards a broad representation of participants across the gamut of dual sensory loss.
Individual trip making behavior entails a wide range of problem solving and decision making steps, for instance, planning and booking a trip, previewing a route, travelling to a transit station, riding a bus, negotiating car traffic, and crossing intersections (Golledge and Stimson, 1997; Golledge, 1999): the Complete Trip (Okunieff et al., 2020). Our results suggest that a greater engagement by the research community with low-cost wayfinding support tools such as tactile maps and written instructions is warranted. In addition, the integration of indoor and outdoor spaces is a necessary yet small component within a broad investigation of human wayfinding. Unpacking the complete trip concept analytically and empirically will provide substantial grounds for future research and create pathways towards seamless wayfinding across the spectrum of individual preferences, capabilities, and needs. Within these research efforts, substantial engagement and the co-creation of knowledge with the disability community will be critical for achieving universal and equitable mobility.
Data Availability Statement
The datasets analyzed in this study are not readily available because of restrictions pertaining to institutional research oversight requirements by the Institutional Review Board at Portland State University. Requests to gain access to any of the data should be directed to the corresponding author.
Ethics Statement
The research described in this article was conducted in accordance with the tenets of the Declaration of Helsinki under the oversight of the Institutional Review Board (IRB) at Portland State University (PSU). All procedures and materials pertaining to our study were approved under PSU IRB protocol #174312. The participant provided their written informed consent for their participation in the study and the publication of any potentially identifiable images or data included in this article.
Author Contributions
MS is the primary author for this paper and the lead for the research study on seamless indoor/outdoor wayfinding under consideration of visual impairment, blindness, and deafblindness, with AP being the co-lead for the study. JW, KH, and BM conducted the data collection, video coding, and initial data analysis. All authors contributed to the writing and editing of this paper, with MS reviewing and validating the initial findings, further analyzing the data, and preparing the main parts of the paper for publication.
Funding
The work presented in this paper was supported by the National Institute for Transportation and Communities (NITC; grant number 1177), a U.S. DOT University Transportation Center.
Conflict of Interest
The authors declare that the research was conducted in the absence of any commercial or financial relationships that could be construed as a potential conflict of interest.
Publisher’s Note
All claims expressed in this article are solely those of the authors and do not necessarily represent those of their affiliated organizations, or those of the publisher, the editors and the reviewers. Any product that may be evaluated in this article, or claim that may be made by its manufacturer, is not guaranteed or endorsed by the publisher.
Acknowledgments
The authors would like to acknowledge the American Printing House for the Blind for their partnership in support of the work presented in this paper, and in particular Elizabeth Schaller, Denise Snow, and Jeremiah Rose for their contributions to our collaborative project. In addition, the authors would like to thank the National Institute for Transportation and Communities for the financial support of our study. Lastly, the authors would like to thank the editor and the three reviewers for their support and constructive comments during the peer review process.
Supplementary Material
The Supplementary Material for this article can be found online at: https://www.frontiersin.org/articles/10.3389/feduc.2021.723098/full#supplementary-material
Footnotes
1For recent reviews of the academic literature on navigation systems, tools, and technologies for blind or low-vision individuals see Real and Araujo (2019), Plikynas et al. (2020), Kuriakose et al. (2020), and Khan et al. (2021).
2https://www.pdx.edu/portland-state-university-facts
4https://openlevelup.net/?l=0\#18/45.51166/-122.68592
5Signia: https://www.signia.net/en-us/hearing-aids/
References
Aarons, G. A., Fettes, D. L., Sommerfeld, D. H., and Palinkas, L. A. (2012). Mixed Methods for Implementation Research: Application to Evidence-Based Practice Implementation and Staff Turnover in Community-Based Organizations Providing Child Welfare Services. Child. Maltreat. 17, 67–79. doi:10.1177/1077559511426908
Allen, G. L. (1999). Cognitive Abilities in the Service of Wayfinding: A Functional Approach. Prof. Geographer 51, 554–561. doi:10.1111/0033-0124.00192
Andrews, S. K. (1983). “Spatial Cognition through Tactual Maps,” in Proceedings of the 1st International Symposium on Maps and Graphics for the Visually Handicapped, March 10-12, 1983, (Washington, DC: Association of American Geographers), 30–40.
Armstrong, J. (1973). The Design and Production of Maps for the Visually Handicapped. Nottingham: Blind Mobility Research Unit, Department of Psychology, University of Nottingham.
Bai, J., Lian, S., Liu, Z., Wang, K., and Liu, D. (2017). Smart Guiding Glasses for Visually Impaired People in Indoor Environment. IEEE Trans. Consumer Electron. 63, 258–266. doi:10.1109/tce.2017.014980
Bai, J., Liu, Z., Lin, Y., Li, Y., Lian, S., and Liu, D. (2019). Wearable Travel Aid for Environment Perception and Navigation of Visually Impaired People. Electronics 8, 697. doi:10.3390/electronics8060697
Carroll, P., Witten, K., Calder-Dawe, O., Smith, M., Kearns, R., Asiasiga, L., et al. (2018). Enabling Participation for Disabled Young People: Study Protocol. BMC Public Health 18, 1–11. doi:10.1186/s12889-018-5652-x
Chang, K.-y. J., Dillon, L. L., Deverell, L., Boon, M. Y., and Keay, L. (2020). Orientation and Mobility Outcome Measures. Clin. Exp. Optom. 103, 434–448. doi:10.1111/cxo.13004
Cheraghi, S. A., Almadan, A., and Namboodiri, V. (2019). “CityGuide: A Seamless Indoor-Outdoor Wayfinding System for People with Vision Impairments,” in ASSETS '19: The 21st International ACM SIGACCESS Conference on Computers and Accessibility, October 28-30, 2019, Pittsburgh, PA, USA. (New York, NY, USA: Association for Computing Machinery) ASSETS ’19, 542–544. doi:10.1145/3308561.3354621
Clarke, P., Twardzik, E., Meade, M. A., Peterson, M. D., and Tate, D. (2019). Social Participation Among Adults Aging with Long-Term Physical Disability: The Role of Socioenvironmental Factors. J. Aging Health 31, 145§–168§. doi:10.1177/0898264318822238
Cmar, J. L. (2015). Orientation and Mobility Skills and Outcome Expectations as Predictors of Employment for Young Adults with Visual Impairments. J. Vis. Impairment Blindness 109, 95–106. doi:10.1177/0145482x1510900205
Connors, E., Chrastil, E., Sanchez, J., and Merabet, L. B. (2014a). Action Video Game Play and Transfer of Navigation and Spatial Cognition Skills in Adolescents Who Are Blind. Front. Hum. Neurosci. 8. doi:10.3389/fnhum.2014.00133
Connors, E. C., Chrastil, E. R., Sánchez, J., and Merabet, L. B. (2014b). Virtual Environments for the Transfer of Navigation Skills in the Blind: A Comparison of Directed Instruction vs. Video Game Based Learning Approaches. Front. Hum. Neurosci. 8. doi:10.3389/fnhum.2014.00223
Courtney-Long, E. A., Carroll, D. D., Zhang, Q. C., Stevens, A. C., Griffin-Blake, S., Armour, B. S., et al. (2015). Prevalence of Disability and Disability Type Among Adults — United States, 2013. MMWR. Morbidity Mortality Weekly Rep. 64, 777–783. doi:10.15585/mmwr.mm6429a2
Creswell, J. W., and Plano Clark, V. (2017). Designing and Conducting Mixed Methods Research. 3rd edn.. Thousand Oaks, CA: Sage Publications, Inc..
Dalton, R. C., Hölscher, C., and Montello, D. R. (2019). Wayfinding as a Social Activity. Front. Psychol. 10, 1–14. doi:10.3389/fpsyg.2019.00142
Denis, M., Mores, C., Gras, D., Gyselinck, V., and Daniel, M. (2014). Is Memory for Routes Enhanced by an Environment’s Richness in Visual Landmarks?. Spat. Cogn. Comput. 14, 284–305. doi:10.1080/13875868.2014.945586
Dong, W., Qin, T., Liao, H., Liu, Y., and Liu, J. (2020). Comparing the Roles of Landmark Visual Salience and Semantic Salience in Visual Guidance during Indoor Wayfinding. Cartography Geogr. Inf. Sci. 47, 229–243. doi:10.1080/15230406.2019.1697965
Duh, P., Sung, Y., Chiang, L. F., Chang, Y., and Chen, K. (2020). V-Eye: A Vision-Based Navigation System for the Visually Impaired. IEEE Trans. Multimedia 23, 1567–1580. doi:10.1109/TMM.2020.3001500
Edmonds, W. A., and Kennedy, T. D. (2017). An Applied Guide to Research Designs: Quantitative, Qualitative, and Mixed Methods. 2nd edn.. Thousand Oaks, CA: Sage Publications, Inc..
Ehn, M., Anderzén-Carlsson, A., Möller, C., and Wahlqvist, M. (2019). Life Strategies of People with Deafblindness Due to Usher Syndrome Type 2a - A Qualitative Study. Int. J. Qual. Stud. Health Well-being 14, 1656790. doi:10.1080/17482631.2019.1656790
Emerson, E., Madden, R., Robertson, J., Graham, H., Hatton, C., and Llewellyn, G. (2009). Intellectual and Physical Disability, Social Mobility, Social Inclusion and Health. Research Report 2009, 2. UK, Lancaster, England: Lancaster University.
Espinosa, M. A., Ungar, S., Ochaita, E., Blades, M., and Spencer, C. (1998). Comparing Methods for Introducing Blind and Visually Impaired People to Unfamiliar Urban Environments. J. Environ. Psychol. 18, 277–287. doi:10.1006/jevp.1998.0097
Fazzi, D. L., and Barlow, J. M. (2017). Orientation and Mobility Techniques: A Guide for the Practitioner. 2nd edn. New York, NY: AFB Press, American Foundation for the Blind.
Fernando, N., McMeekin, D. A., and Murray, I. (2021). Route Planning Methods in Indoor Navigation Tools for Vision Impaired Persons: A Systematic Review. Disabil. Rehabil. Assistive Technol., 1–20. doi:10.1080/17483107.2021.1922522
Fetters, M. D., Curry, L. A., and Creswell, J. W. (2013). Achieving Integration in Mixed Methods Designs—Principles and Practices. Health Serv. Res. 48, 2134–2156. doi:10.1111/1475-6773.12117
Fonteyn, M. E., Kuipers, B., and Grobe, S. J. (1993). A Description of Think Aloud Method and Protocol Analysis. Qual. Health Res. 3, 430–441. doi:10.1177/104973239300300403
Franks, F. L., and Baird, R. M. (1971). Geographical Concepts and the Visually Handicapped. Exceptional Child. 38, 321–324. doi:10.1177/001440297103800404
Giudice, N. A., Walton, L. A., and Worboys, M. (2010). “The Informatics of Indoor and Outdoor Space: A Research Agenda,” in Proceedings of the 2nd ACM SIGSPATIAL International Workshop on Indoor Spatial Awareness (New York, NY, USA: Association for Computing Machinery) ISA ’10, 47–53.
Giudice, N. A., Whalen, W. E., Riehle, T. H., Anderson, S. M., and Doore, S. A. (2019). Evaluation of an Accessible, Real-Time, and Infrastructure-free Indoor Navigation System by Users Who Are Blind in the Mall of America. J. Vis. Impairment Blindness 113, 140–155. doi:10.1177/0145482x19840918
Golledge, R. G. (1992). Place Recognition and Wayfinding: Making Sense of Space. Geoforum 23, 199–214. doi:10.1016/0016-7185(92)90017-x
Golledge, R. G., and Stimson, R. J. (1997). Spatial Behavior: A Geographic Perspective. New York, NY: The Guilford Press.
Golledge, R. (1991). Tactual Strip Maps as Navigational Aids. J. Vis. Impairment Blindness 85, 296–301. doi:10.1177/0145482x9108500708
Griffin-Shirley, N., Banda, D. R., Ajuwon, M. P., Cheon, J., Lee, J., Park, H. R., et al. (2017). A Survey on the Use of mobile Applications for People Who Are Visually Impaired. J. Vis. Impairment Blindness 111, 307–323. doi:10.1177/0145482x1711100402
Hegarty, M., Richardson, A. E., Montello, D. R., Lovelace, K., and Subbiah, I. (2002). Development of a Self-Report Measure of Environmental Spatial Ability. Intelligence 30, 425–447. doi:10.1016/s0160-2896(02)00116-2
Hickson, L., Lind, C., Worrall, L., Yiu, E., Barnett, H., and Lovie-Kitchin, J. (1999). Hearing and Vision in Healthy Older Australians: Objective and Self-Report Measures. Adv. Speech Lang. Pathol. 1, 95–105. doi:10.3109/14417049909167163
Hodge, S., Barr, W., Bowen, L., Leeven, M., and Knox, P. (2013). Exploring the Role of an Emotional Support and Counselling Service for People with Visual Impairments. Br. J. Vis. Impairment 31, 5–19. doi:10.1177/0264619612465168
Ishikawa, T. (2013). Retention of Memory for Large-Scale Spaces. Memory 21, 807–817. doi:10.1080/09658211.2012.758289
Kane, S. K., Jayant, C., Wobbrock, J. O., and Ladner, R. E. (2009). “Freedom to Roam: A Study of mobile Device Adoption and Accessibility for People with Visual and Motor Disabilities,” in Proceedings of the 11th International ACM SIGACCESS Conference on Computers and Accessibility (New York, NY, USA: ACM) Assets ’09, 115–122.
Kennedy, J. (1983). “Pictoral Displays and the Blind: Elements, Patterns, and Metaphors,” in Proceedings of the 1st International Symposium on Maps and Graphics for the Visually Handicapped (Washington, DC: Association of American Geographers), 75–99.
Kettunen, P., Irvankoski, K., Krause, C. M., and Sarjakoski, L. T. (2013). Landmarks in Nature to Support Wayfinding: The Effects of Seasons and Experimental Methods. Cogn. Process. 14, 245–253. doi:10.1007/s10339-013-0538-4
Khan, S., Nazir, S., and Khan, H. U. (2021). Analysis of Navigation Assistants for Blind and Visually Impaired People: A Systematic Review. IEEE Access 9, 26712–26734. doi:10.1109/access.2021.3052415
Kim, S., and Lehto, X. Y. (2013). Travel by Families with Children Possessing Disabilities: Motives and Activities. Tourism Manage. 37, 13–24. doi:10.1016/j.tourman.2012.12.011
Klippel, A., and Winter, S. (2005). “Structural Salience of Landmarks for Route Directions,” in Lecture Notes in Computer Science, 347–362. doi:10.1007/11556114_22
Kray, C., Fritze, H., Fechner, T., Schwering, A., Li, R., and Anacta, V. J. (2013). “Transitional Spaces: Between Indoor and Outdoor Spaces,” in Spatial Information Theory. Editors T. Tenbrink, J. Stell, A. Galton, and Z. Wood (Cham: Springer International Publishing), 14–32. Lecture Notes in Computer Science. doi:10.1007/978-3-319-01790-7_2
Kuriakose, B., Shrestha, R., and Sandnes, F. E. (2020). Tools and Technologies for Blind and Visually Impaired Navigation Support: A Review. IETE Tech. Rev., 1–16. doi:10.1080/02564602.2020.1819893
Lawson, H., and Parker, A. T. (2020). The Visually Impaired Learner (VIL) and Orientation and Mobility (O&M) Programs at Portland State University. Vis. Impairments Deafblind Educ. Q. 65, 15.
Li, C. (2006). User Preferences, Information Transactions and Location-Based Services: A Study of Urban Pedestrian Wayfinding. Comput. Environ. Urban Syst. 30, 726–740. doi:10.1016/j.compenvurbsys.2006.02.008
Li, J., He, X., and Li, J. (2015). “2D LiDAR and Camera Fusion in 3D Modeling of Indoor Environment,” in Proceedings of the 2015 National Aerospace and Electronics Conference (NAECON), June 15-19, 2015, (Dayton, OH, USA: IEEE), 379–383. doi:10.1109/naecon.2015.7443100
Li, R., Korda, A., Radtke, M., and Schwering, A. (2014). Visualising Distant Off-Screen Landmarks on mobile Devices to Support Spatial Orientation. J. Location Based Serv. 8, 166–178. doi:10.1080/17489725.2014.978825
Liu, J. (2019). Social Participation Among Adults with Disability. Am. J. Occup. Ther. 73, 7311515286p1. doi:10.5014/ajot.2019.73s1-po5066
May, A. J., Ross, T., Bayer, S. H., and Tarkiainen, M. J. (2003). Pedestrian Navigation Aids: Information Requirements and Design Implications. Personal. Ubiquitous Comput. 7, 331–338. doi:10.1007/s00779-003-0248-5
Meshram, V. V., Patil, K., Meshram, V. A., and Shu, F. C. (2019). An Astute Assistive Device for Mobility and Object Recognition for Visually Impaired People. IEEE Trans. Human-Machine Syst. 49, 449–460. doi:10.1109/thms.2019.2931745
Nicholson, J., Kulyukin, V., and Coster, D. (2009). ShopTalk: Independent Blind Shopping through Verbal Route Directions and Barcode Scans. Open Rehabil. J. 2, 11–23. doi:10.2174/1874943700902010011
Norgate, S. H., and Ormerod, M. G. (2012). Landmarks in Tourist Wayfinding: A Review. Proc. Inst. Civil Eng. - Urban Des. Plann. 165, 79–87. doi:10.1680/udap.9.00053
Nuhn, E., and Timpf, S. (2017). A Multidimensional Model for Selecting Personalised Landmarks. J. Location Based Serv. 11, 153–180. doi:10.1080/17489725.2017.1401129
Okoro, C. A., Hollis, N. D., Cyrus, A. C., and Griffin-Blake, S. (2018). Prevalence of Disabilities and Health Care Access by Disability Status and Type Among Adults — United States, 2016. Morbidity Mortality Weekly Rep. 67, 882–887. doi:10.15585/mmwr.mm6732a3
Okunieff, P. E., Brown, L., Heggedal, K., O’Reilly, K., Weisenberger, T., Guan, A., et al. (2020). Multimodal and Accessible Travel Standards Assessment – Outreach Report. Washington, DC: US Department of Transportation. Intelligent Transportation Systems Joint Program Office. Tech. Rep. FHWA-JPO-21-844.
Padmanaban, R., and Krukar, J. (2017). “Increasing the Density of Local Landmarks in Wayfinding Instructions for the Visually Impaired,” in Progress in Location-Based Services 2016. Editors G. Gartner, and H. Huang (Cham, Switzerland: Springer International Publishing), Lecture Notes in Geoinformation and Cartography), 131–150. doi:10.1007/978-3-319-47289-8_7
Parker, A. T. (2020). Mobility Matters: Interdisciplinary Partnerships to Create More Accessible Cities. Vis. Impairments Deafblind Educ. Q. 65, 7.
Parker, A. T., Swobodzinski, M., Brown-Ogilvie, T., and Beresheim-Kools, J. (2020). The Use of Wayfinding Apps by Deafblind Travelers in an Urban Environment: Insights from Focus Groups. Front. Educ. 5, 145S–168S. doi:10.3389/feduc.2020.572641
Passini, R., and Proulx, G. (1988). Wayfinding without Vision: An experiment with Congenitally Totally Blind People. Environ. Behav. 20, 227–252. doi:10.1177/0013916588202006
Pew Research Center (2015). The Smartphone Difference. Washington, DC: Pew Research Center. Tech. rep..
Pissaloux, E. E., Velázquez, R., and Maingreaud, F. (2017). A New Framework for Cognitive Mobility of Visually Impaired Users in Using Tactile Device. IEEE Trans. Human-Machine Syst. 47, 1040–1051. doi:10.1109/thms.2017.2736888
Plikynas, D., Žvironas, A., Budrionis, A., and Gudauskis, M. (2020). Indoor Navigation Systems for Visually Impaired Persons: Mapping the Features of Existing Technologies to User Needs. Sensors 20. doi:10.3390/s20030636
Ponchillia, P. E., Jo, S., Casey, K., and Harding, S. (2020). Developing an Indoor Navigation Application: Identifying the Needs and Preferences of Users Who Are Visually Impaired. J. Vis. Impairment Blindness 114, 344–355. doi:10.1177/0145482x20953279
Raubal, M., and Winter, S. (2002). “Enriching Wayfinding Instructions with Local Landmarks,” in Geographic Information Science. Editors M. J. Egenhofer, and D. M. Mark (Berlin, Heidelberg: Springer), 243–259. Lecture Notes in Computer Science. doi:10.1007/3-540-45799-2_17
Real, S., and Araujo, A. (2019). Navigation Systems for the Blind and Visually Impaired: Past Work, Challenges, and Open Problems. Sensors 19, 3404. doi:10.3390/s19153404
R. G. Golledge (1999). Wayfinding Behavior: Cognitive Mapping and Other Spatial Processes (Baltimore, MA: Johns Hopkins University Press).
Rodriguez-Sanchez, M., and Martinez-Romo, J. (2017). GAWA – Manager for Accessibility Wayfinding Apps. Int. J. Inf. Manage. 37, 505–519. doi:10.1016/j.ijinfomgt.2017.05.011
Röijezon, U., Prellwitz, M., Innala Ahlmark, D., van Deventer, J., Nikolakopoulos, G., and Hyyppä, K. (2019). A Haptic Navigation Aid for Individuals with Visual Impairments: Indoor and Outdoor Feasibility Evaluations of the LaserNavigator. J. Vis. Impairment Blindness 113, 194–201. doi:10.1177/0145482x19842491
Ross, D. A., and Kelly, G. W. (2009). Filling the Gaps for Indoor Wayfinding. J. Vis. Impairment Blindness 103, 229–234. doi:10.1177/0145482x0910300406
Sato, D., Oh, U., Guerreiro, J., Ahmetovic, D., Naito, K., Takagi, H., et al. (2019). NavCog3 in the Wild: Large-Scale Blind Indoor Navigation Assistant with Semantic Features. Acm Trans. Accessible Comput. 12, 14. doi:10.1145/3340319
Schles, R. A. (2021). Population Data for Students with Visual Impairments in the united states. J. Vis. Impairment Blindness 115, 117–189. doi:10.1177/0145482x211016124
Sorrows, M., and Hirtle, S. (1999). “The Nature of Landmarks for Real and Electronic Spaces,” in Spatial Information Theory. Cognitive and Computational Foundations of Geographic Information Science. COSIT 1999.. Editors C. Freska, and D. M. Mark (Berlin, Heidelberg, Germany: Springer), 37–50. vol. 1661 of Lecture Notes in Computer Science. doi:10.1007/3-540-48384-5_3
Swobodzinski, M., and Parker, A. T. (2019). “A Comprehensive Examination of Electronic Wayfinding Technology for Visually Impaired Travelers in an Urban Environment,” in Final Report NITC-RR-1177 (Portland, OR: Transportation Research and Education Center (TREC)). doi:10.15760/trec.227
Swobodzinski, M., and Raubal, M. (2009). An Indoor Routing Algorithm for the Blind: Development and Comparison to a Routing Algorithm for the Sighted. Int. J. Geographical Inf. Sci. 23, 1315–1343. doi:10.1080/13658810802421115
Tom, A., and Denis, M. (2003). “Referring to Landmark or Street Information in Route Directions: What Difference Does it Make?,” in Spatial Information Theory. Foundations of Geographic Information Science. Editors W. Kuhn, M. F. Worboys, and S. Timpf (Berlin, Heidelberg: Springer), 362–374. Lecture Notes in Computer Science. doi:10.1007/978-3-540-39923-0_24
Tversky, B. (2003). Structures of Mental Spaces: How People Think about Space. Environ. Behav. 35, 66–80. doi:10.1177/0013916502238865
Vanclooster, A., and De Maeyer, P. (2012). “Combining Indoor and Outdoor Navigation: The Current Approach of Route Planners,” in Advances in Location-Based Services: 8th International Symposium on Location-Based Services, Vienna 2011, November 21-23, 2011, Vienna, Austria. Editors G. Gartner, and F. Ortag (Berlin, Heidelberg: Springer), 283–303. Lecture Notes in Geoinformation and Cartography. doi:10.1007/978-3-642-24198-7_19
Vanclooster, A., Weghe, N. V. d., and Maeyer, P. D. (2016). Integrating Indoor and Outdoor Spaces for Pedestrian Navigation Guidance: A Review. Trans. GIS 20, 491–525. doi:10.1111/tgis.12178
Vincent, C., Routhier, F., Martel, V., Mottard, M.-v., Dumont, F., Côté, L., et al. (2014). Field Testing of Two Electronic Mobility Aid Devices for Persons Who Are Deaf-Blind. Disabil. Rehabil. Assistive Technol. 9, 414–420. doi:10.3109/17483107.2013.825929
Wagner, S., Fet, N., Handte, M., and Marrón, P. J. (2017). “An Approach for Hybrid Indoor/outdoor Navigation,” in 2017 International Conference on Intelligent Environments (IE), 36–43. doi:10.1109/ie.2017.22
Wilson, C., McColl, M. A., Zhang, F., and McKinnon, P. (2017). Measuring Integration of Disabled Persons: Evidence from Canada’s Time Use Databases. Can. J. Disabil. Stud. 6, 105–127. doi:10.15353/cjds.v6i1.335
Winters, M., Voss, C., Ashe, M. C., Gutteridge, K., McKay, H., and Sims-Gould, J. (2015). Where Do They Go and How Do They Get There? Older Adults’ Travel Behaviour in a Highly Walkable Environment. Soc. Sci. Med. 133, 304–312. doi:10.1016/j.socscimed.2014.07.006
Wittich, W., Granberg, S., Wahlqvist, M., Pichora-Fuller, M. K., and Mäki-Torkko, E. (2021). Device Abandonment in Deafblindness: A Scoping Review of the Intersection of Functionality and Usability through the International Classification of Functioning, Disability and Health Lens. BMJ Open 11, e044873. doi:10.1136/bmjopen-2020-044873
Yan, J., Diakité, A. A., and Zlatanova, S. (2019). A Generic Space Definition Framework to Support Seamless Indoor/outdoor Navigation Systems. Trans. GIS 23, 1273–1295. doi:10.1111/tgis.12574
Keywords: wayfinding, indoor and outdoor navigation, deafblindness, case study, mobile technology, pedestrian travel
Citation: Swobodzinski M, Parker AT, Wright JD, Hansen K and Morton B (2021) Seamless Wayfinding by a Deafblind Adult on an Urban College Campus: A Case Study on Wayfinding Performance, Information Preferences, and Technology Requirements. Front. Educ. 6:723098. doi: 10.3389/feduc.2021.723098
Received: 10 June 2021; Accepted: 25 August 2021;
Published: 15 September 2021.
Edited by:
Mohamed A. Ali, Grand Canyon University, United StatesReviewed by:
Fariza Hanis Abdul Razak, MARA University of Technology, MalaysiaHassan Karimi, University of Pittsburgh, United States
Walter Wittich, Université de Montréal, Canada
Copyright © 2021 Swobodzinski, Parker, Wright, Hansen and Morton. This is an open-access article distributed under the terms of the Creative Commons Attribution License (CC BY). The use, distribution or reproduction in other forums is permitted, provided the original author(s) and the copyright owner(s) are credited and that the original publication in this journal is cited, in accordance with accepted academic practice. No use, distribution or reproduction is permitted which does not comply with these terms.
*Correspondence: Martin Swobodzinski, c3dvYm9kQHBkeC5lZHU=