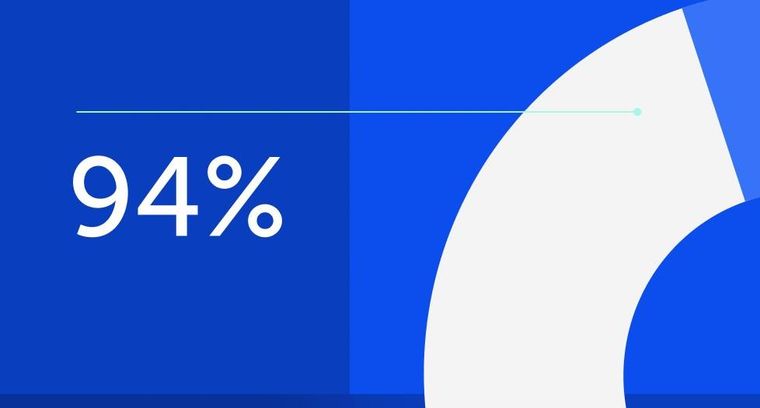
94% of researchers rate our articles as excellent or good
Learn more about the work of our research integrity team to safeguard the quality of each article we publish.
Find out more
ORIGINAL RESEARCH article
Front. Educ., 30 June 2021
Sec. Teacher Education
Volume 6 - 2021 | https://doi.org/10.3389/feduc.2021.693012
The successful development and classroom integration of Virtual (VR) and Augmented Reality (AR) learning environments requires competencies and content knowledge with respect to media didactics and the respective technologies. The paper discusses a pedagogical concept specifically aiming at the interdisciplinary education of pre-service teachers in collaboration with human-computer interaction students. The students’ overarching goal is the interdisciplinary realization and integration of VR/AR learning environments in teaching and learning concepts. To assist this approach, we developed a specific tutorial guiding the developmental process. We evaluate and validate the effectiveness of the overall pedagogical concept by analyzing the change in attitudes regarding 1) the use of VR/AR for educational purposes and in competencies and content knowledge regarding 2) media didactics and 3) technology. Our results indicate a significant improvement in the knowledge of media didactics and technology. We further report on four STEM learning environments that have been developed during the seminar.
Learning new knowledge is a challenging task that requires a high degree of motivation, discipline, and hard work (Ur, 1996). Using Virtual Reality (VR) and Augmented Reality (AR) for educational purposes can lead to an increased motivation, a gain of experience with the direct application of the learning contents, and a higher task performance (Bacca et al., 2014; Stevens and Kincaid, 2015; Makransky and Lilleholt, 2018). These technologies demonstrate learning contents in an audiovisual way and fulfill the conditions for supporting learning (Ericsson et al., 1993; Oberdörfer and Latoschik, 2019a). The latter aspect can be achieved by following a gamified approach (Oberdörfer, 2021). The technologies further enable learners to experience situations which otherwise would not be possible. The learning content could be too dangerous or required instruments are not available, e.g., due to their mere size or an instance of remote teaching (Riegle and Matejka, 2006; Rogers et al., 2017).
For developing these motivating learning environments and integrating them into teaching concepts, developers and educators need to possess interdisciplinary knowledge with regard to 1) media didactics and 2) technologies of VR/AR (Petko, 2012; Knezek and Christensen, 2016). This knowledge allows for an effective teaching with technology and is referred to as Technological Pedagogical Content Knowledge (TPCK) (Koehler and Mishra, 2005). Media didactics refers to teaching with media or the design and use of media content for educational purposes (Tulodziecki, 2012; Tiede and Grafe, 2016). In-depth knowledge of media didactics enables the design and the successful integration of VR/AR learning environments in lessons, e. g, by retaining the students’ attention while using immersive media (Kerawalla et al., 2006; Cuendet et al., 2013). Technological content knowledge allows for a successful development, operation (Dunleavy et al., 2009) and analysis of these systems with regard to crucial qualities, e.g., usability. The technological aspects of highly usable interactive systems are the main focus of Human-Computer Interaction (HCI) (Dix et al., 2004). Thus, an interdisciplinary educational concept that combines the strengths of HCI and pedagogy seems promising to foster the acquisition of TPCK.
Therefore, we designed a pedagogical concept and embedded a corresponding course in the curriculum of pre-service teacher education. To establish an interdisciplinary discussion, we also embedded the course in the curriculum of an HCI bachelor’s and master’s program. The seminar specifically introduces students to the theoretical and practical concepts of integrating technologies of VR/AR in teaching concepts (Bucher and Grafe, 2018; Bucher et al., 2020). The students’ overall goal is to design a learning environment, e.g., a serious game, targeting VR/AR as displayed in Figure 1. Aside from requiring the students to apply their knowledge, this goal fosters the knowledge exchange between the students of the two different disciplines. However, despite an increased accessibility of current game engines, such as Unreal Engine (Epic Games Inc., 2021) and Unity (Unity, 2021), especially pre-service teachers often struggle to implement their learning environments using these frameworks. For assisting this development and hence the learning process of the students, we developed a tutorial accompanying the course. The tutorial introduces the core concepts of using Unity and of developing basic VR/AR applications. The tutorial further acts as a workshop, thus allowing the students to implement their projects in an assisted way.
FIGURE 1. During the seminar and guided by our tutorial, students develop learning environments targeting AR (A) and VR (B). Horst–The Teaching Frog(A) deals with the learning content of the anatomy of vertebrates. VRaccess(B) simulates the experimental setup needed for the production of ammonia.
This paper presents and discusses the interdisciplinary core concepts of the seminar and our tutorial guiding the process of developing VR/AR learning environments. Following the pedagogical concept, pre-service teachers and HCI students gain practical experiences critical for a successful integration of VR/AR applications in teaching and learning concepts. We evaluate the effectiveness of the overall approach by analyzing the change in attitudes regarding 1) the use of VR/AR for educational purposes and in competencies and content knowledge regarding 2) media didactics and 3) technology over the course of the seminar. Our results show a significant improvement in knowledge of media didactics as well as of technology. The study also shows a positive tendency of pre-service teachers to integrate AR systems in their future teaching, while remaining neutral toward the integration of VR. We further report on four projects targeting STEM learning contents that have been developed during the seminar. These learning environments elicit a theoretically grounded educational concept and a good technical realization. This indicates a successful transfer and application of the theoretical learning contents of the seminar. The description of the seminar and tutorial can act as a guideline for the design of further interdisciplinary pedagogy and technology-based courses.
The powerful graphics engines of current game engines, e.g., Unreal Engine and Unity, are commonly used to develop VR/AR applications. These game engines can audiovisually present complex information, accurately simulate physical laws, and are even used to demonstrate complex scientific problems (Lv et al., 2013; Knote et al., 2019, Knote et al., 2020). Thus, interactive learning environments can simulate and demonstrate any knowledge in any form (Perry and DeMaria, 2009).
By embedding the learning process in a serious game, the learning process becomes an engaging, vivid, and inspiring experience (McGonigal, 2011). Serious games feature an educational aspect and are not solely developed for entertainment (Anderson et al., 2009; de Freitas and Liarokapis, 2011). As a result, these games combine classical game design elements with pedagogical aspects to create motivating learning environments (Charsky, 2010). The learning, i.e., the application and demonstration of the learning contents, is achieved by mapping the learning contents to a serious game’s game mechanics (Oberdörfer and Latoschik, 2019b).
Alternatively, to increase a learner’s motivation, non-gaming learning applications can be enhanced by gamification (Seaborn and Fels, 2015). Gamification refers to the implementation of game design elements, e.g., highscores and achievements, in non-gaming contexts to increase a user’s motivation toward using the application (Deterding et al., 2011).
Learning applications targeting VR/AR are used in almost any kind of area, but mostly focus STEM related topics (Bacca et al., 2014; Freina and Ott, 2015).
VR bases on immersion, i.e., “the extent to which the computer displays are capable of delivering an inclusive, extensive, surrounding, and vivid illusion of reality to the senses of a human participant” (Slater and Wilbur, 1997). Immersion is an objective characteristic describing possible actions within a given system (Slater, 2009). The level of immersion increases presence describing the subjective sensation of being in a real place, i.e., accepting the virtual environment as the current location, despite physically being in a different environment (Slater, 2009). Hence, presence indicates the perceived realness of the virtual experience (Skarbez et al., 2017). In comparison to using a regular screen, wearing a Head-Mounted Display (HMD) increases a user’s visual field on the virtual environment. With a higher visual angle, the emotional responses to audiovisual stimuli are increased (Gall and Latoschik, 2020). This is crucial as it has a positive impact on a user in a learning situation, e.g., increased task performance (Stevens and Kincaid, 2015) and learning motivation (Makransky and Lilleholt, 2018). Also, the spatial knowledge presentation enables learners to visualize and to analyze complex learning contents (Dede, 2009; Freina and Ott, 2015), e.g., learning the application of affine transformations (Oberdörfer et al., 2019). The direct and explicit audiovisual demonstration of the knowledge further achieves implicit learning (Slater, 2017).
AR three-dimensionally integrates virtual elements into the real world while simultaneously allowing for an interaction in real time (Azuma, 1997). Using AR applications in an educational context can lead to an increased motivation, improvements with respect to interaction and collaboration, and gaining experience with the direct application of the learning contents (Bacca et al., 2014). For instance, Mathland demonstrates the mathematics behind the Newtonian physics and allows users to modify the physical laws (Khan et al., 2018). Also, AR learning environments can be designed to especially suit the requirements of special needs education (Steinhaeusser et al., 2019).
A learning process leads to a permanent improvement of performance or of performance potential being caused by experience and interaction with the world (Driscoll, 2013). Several paradigms have been formulated to approach a definition of the process of learning, i.e., the paradigms of behaviorism, cognitivism, and constructivism (Kerres, 2018). These paradigms can act as a guideline for the pedagogical design of VR/AR learning environments and serious games.
The learning theory of behaviorism defines an individual learning process as a black box and focuses on feedback. Behavioristic learning takes place through positive or negative reinforcement by the environment and by a repetition of the learning contents (Kerres, 2018; Tulodziecki et al., 2019). In the context of game design, behavioristic learning can be achieved by implementing rewards, punishments, and an episodic gameplay. Reward game mechanics, e.g., highscores, experience points, and loot upon defeating an enemy, provide a player with positive feedback about their actions. Punishments, e.g., reducing the durability of a player’s equipment or simulating an injury slowing down a player, give players negative feedback. An episodic gameplay is achieved by designing short levels of which each represents an individual challenge that can be repeated quickly.
In contrast to behavioristic learning, cognitivism defines learning as a process that leads to the development of internal cognitive structures (Kerres, 2018; Tulodziecki et al., 2019). Following the theory of cognitivism, the learning process can be guided by the provision of a specific problem that motivates players to tackle the learning content, e.g., a background story introducing the player’s goals. The learning process can also be controlled by providing a tutorial that is followed by subsequent challenges leading to further applications of the learning content. The underlying principles and the results of a player’s actions need to be explicitly presented in an audiovisual way to support the learning process. Finally, the difficulty of the gameplay has to be adjusted to match the learning effect and to take gained experience into account.
According to the learning theory of constructivism, learning is defined as an individual knowledge construction process being caused by the experience of a specific situation (Kerres, 2018; Tulodziecki et al., 2019). Learning from a constructivist viewpoint can ideally be achieved by providing an open-world setting with a plethora of interaction possibilities. The mere exploration of the virtual world can initiate the learning process by creating specific situations. The provision of an agent interacting with the player and initiating a reflective learning can be a substitute for the social aspect (Bucher et al., 2018).
For a successful development and integration in lessons of VR/AR learning environment, developers and educators need in-depth content knowledge with respect to media didactics and technology. The technologies of VR/AR can provide helpful opportunities to improve the acquisition of complex learning contents, e.g., by audiovisually demonstrating the underlying principles or increasing a learner’s motivation. Competencies in media didactics enable developers and educators to design media content from a pedagogical point of view, e.g., designing learning activities according to the learning theories. To enable HCI students and pre-service teachers to acquire such an interdisciplinary content knowledge, a specific education in these two areas is needed. This finally leads to an understanding for the complex relationship between technology and pedagogical practices, i.e., TPCK.
The target group of the pedagogical concept “Teaching and Learning with VR/AR” and the corresponding seminar are HCI students and pre-service teachers. The main goals are, that students learn about 1) the pedagogical design of media, 2) the sound integration of VR/AR applications in teaching and learning concepts, and 3) technological qualities of good educational systems (Bucher and Grafe, 2018; Bucher et al., 2020). The pedagogical concept of the seminar follows the paradigms of action-oriented teaching (Tulodziecki et al., 2013; Tulodziecki et al., 2019; Tulodziecki et al., 2017) and learning by design (Mishra and Koehler, 2003; Koehler and Mishra, 2005).
The approach of action-oriented teaching motivates teaching concepts with different complex tasks, such as problems as well as tasks of decision making, of design or of assessment (Tulodziecki et al., 2017). Hence, an interdisciplinary design task was chosen to indicate the relevance of the seminar’s contents. According to the learning by design approach, a self-driven design of media products facilitates the development of an understanding for the complex relationship between the learners, the hardware, and software used and the associated practices (Mishra and Koehler, 2003; Koehler and Mishra, 2005). This leads to an acquisition of TPCK (Koehler and Mishra, 2005). Following these two approaches, the students design own media products, i.e., learning environments targeting VR/AR, as well as related lessons to solve authentic and specific pedagogical and technical challenges. This work is done in interdisciplinary groups to initiate a knowledge exchange between the students. The aim is a reflection of the relationship between technology, learning contents, and pedagogy. The approach of action-oriented teaching and learning already demonstrated to be effective for structuring classroom lessons (Grafe, 2008) as well as for teaching at university level (Weritz, 2008). Also, the learning by design approach yielded positive effects on the technological content knowledge of pre-service and in-service teachers (Koehler and Mishra, 2005; Koehler et al., 2007; Delello, 2014).
Aside from setting the main objective, we decided to leave the conceptual design of the learning environments completely up to the students. This freedom avoids restricting the students’ creativity and makes the learning environments to their projects. This should result in a higher motivation to work on their projects as they are no longer an external task but a personal goal. In selecting learning content for their VR/AR learning environments, the interdisciplinary groups are encouraged to address a topic relevant to the pre-service teachers’ field of study. The application has to be 1) designed from a pedagogical standpoint, 2) designed to provide interaction techniques eliciting a high usability, 3) embedded in a lesson or teaching concept, and 4) running on the selected target platform, e.g., on a smartphone or on a VR workstation. For developing their learning environments, the Unity game engine is used.
Individual sessions as well as their distribution over the course were structured into eight learning steps: 1) Introduction of the complex design task and collection of spontaneous solution proposals, 2) agreement on objectives and discussion of their significance, 3) agreement on further procedure, 4) development of basics for the task solution, 5) task solution, 6) comparison and summary of solutions, 7) application tasks, and 8) continuation and reflection of what has been learned.
Based on the two approaches, the following central principles guided the design of the seminar:
• Interdisciplinary design and implementation of the pedagogical concept
• Presenting a complex design task in the beginning
• Collaborative and student-centered task-processing
• Lecturer as coach, providing continuous feedback
• Support the design process by aligning it with theory and practical examples
• Scaffolding authentic technology experiences
• Fostering reflection of the learning process and its results
• Fostering reflection of related knowledge, attitudes and beliefs
The interdisciplinary core learning contents of the seminar are based on theoretical principles of media didactics as well as HCI as shown in Table 1. This allows for a learning of TPCK relevant for a sound integration of VR/AR applications in teaching concepts, the identification as well as operation of well-designed learning application, and development of basic VR/AR applications. The overall goal of the seminar leads to an application of the content knowledge. Hence, the realization of VR/AR learning environments even evokes a development of relevant skills and hence competencies with regard to media didactics and technology (Tulodziecki, 2012; Tiede and Grafe, 2016). The sessions over the course of the semester are structured as followed:
1. Introduction to the seminar including a hands-on phase on VR/AR applications
2. Conditions of media education and relevance of HCI
3. Conceptual design 1
4. Concepts of usability, user-centered design, and design guidelines
5. Conceptual design 2
6. Conceptual design 3
7. Learning paradigms
8. Empirical findings about learning with AR and VR and Serious Games
9. Principles of 3D user interface design
10. Conceptual design 4
11. Instructional design with AR and VR
12. Evaluation of pedagogical and technical qualities of VR/AR applications
13. Conceptual design 5
14. Final thoughts and farewell
15. Presentation of results
The conceptual design phases are intended to allow students to conceptualize and to implement their VR/AR learning environments. Simultaneously, these phases intend a training of the participants’ technological skills (see Table 1) which are highly relevant for implementing basic VR/AR applications. The distribution of these phases aligns with the presentation of the seminar’s theoretical contents. This allows the students to directly apply these information by discussing and including them in their concepts, thus ultimately fostering their content knowledge and developing competencies.
However, the conceptual design phases are intended to allow for a development and discussion of the conceptual designs with the lecturer. As a result, they are not suitable for introducing students to the concepts of implementing their projects using Unity, i.e., developing technological skills. Thus, providing a separate tutorial introducing the technological skills, i.e., basics of application and game design with respect to VR/AR, is a critical element to enable students completing the seminar’s overall goal.
Aside from merely explaining Unity and the core concepts of developing applications for VR/AR, i.e., the technological skills, the tutorial aims to fulfill two additional goals. First, potentially lacking a previous experience with programming, especially pre-service teachers mostly are intimidated by the seminar’s overarching goal. To help the students to overcome this initial anxiety, the tutorial aims to motivate them and support their self-esteem. We decided to tackle these goals by collaboratively developing a small game step-by-step. Second, the students often feel lost in the design process as it is hard to estimate for them what features are feasible. This also may affect the creativity in conceptualizing their actual projects. Therefore, the tutorial aims to demonstrate how functions are designed. This intends to support students’ understanding of the range of nearly infinity possibilities and support the creative design process.
The tutorial consists of four core weekly sessions. Each of them deals with a specific topic and guides the students through the learning process step-by-step. For the remainder of the semester, the tutorial acts as a workshop where students can receive feedback and help.
The first session deals with the process of installing Unity on the students’ own computers. This step is critical as the game engine provides a broad variety of different optional modules. Identifying necessary modules might be impossible for users without any previous knowledge with respect to this framework. For instance, when developing for AR and targeting Android devices, adding the Android Build Support module is crucial. Therefore, the tutor guides the attendees of the seminar through the setup process.
Subsequently, the students take a look at Unity’s user interface by creating their first projects. Here, the tutor gives an overview of the various windows and explains their functions. This also leads to a development of an understanding for the specific vocabulary, e.g., components, prefabs, and hierarchy window. To facilitate the understanding, short demonstrations are given. For example, a prefab, e.g., a cube, can be created in the hierarchy window. Once created, the students watch it automatically appearing in the actual scene.
The second session follows the goal of playfully introducing the students to developing a small game. The students not only familiarize themselves with Unity’s basic functions, but also build up self-confidence. Instead of targeting VR or AR, the game is a desktop build to avoid overwhelming the participants. During the gameplay, users explore a simplistic virtual environment by the means of typical keybindings, i.e., WASD, and manipulate a toy car. The game is shown in Figure 2.
FIGURE 2. An example of the students’ first computer game created with Unity. In this first person game, the player can move freely in the environment and grab, hold, and drop the toy car lying on the ground.
In particular, the session deals with the following topics and introduces related concepts:
1. Creating virtual environments using basic 3D objects
2. Creating and using monochromatic materials
3. Importing and using assets from the Unity Asset Store
4. Using components, i.e., rigidbodies and colliders to manipulate 3D objects
5. Using ready-to-use scripts to implement interaction possibilities
6. Testing the game in play mode
7. Building the game
In this lesson, the tutor introduces the students to Vuforia (PTC Inc., 2021) that allows them to realize AR projects easily. By implementing a simple project, they learn how to develop an AR application with Unity using the Vuforia plugin. They augment a banknote, i.e., using it as an image target, as illustrated in Figure 3. The session extents the students’ previous knowledge of using Unity for simplistic game design by introducing the use of a plugin.
FIGURE 3. In the students’ first AR app, simple 3D objects appear by scanning a bank note with the smartphone’s camera.
At the beginning, the tutor introduces Vuforia and describe the plugin’s core functions. Students learn to prepare a project for AR by explaining them the settings they must adjust, i.e., the build and player settings. Subsequently, they prepare a scene that includes a camera and an image target. For this, they upload the desired image target to Vuforia’s database and link it to their respective projects. Students then create a 3D content, e.g., three differently colored cubes, assign it to the image target, and build their applications. As soon as the students test their applications on smartphones, they are usually amazed that they managed to augment the real world.
Following the concept of the AR lesson, this session introduces the development for VR as shown in Figure 4. Students again implement a basic application allowing for a direct object manipulation in VR using the SteamVR plugin (Valve Coorperation, 2015–2021). For testing their applications, we provide an HTC Vive pro (HTC Corporation, 2011–2021) to further allow for a state of the art experience of VR. This session fosters the knowledge acquired during the previous lessons, e.g., students develop another own project, while simultaneously introducing a new concept, i.e., the development for VR.
FIGURE 4. This simple VR application was developed during the tutorial. The objects on the table can be grabbed and moved freely. These interactions can be tested directly in Unity with or without a VR headset.
The session starts with a quick explanation of the core functions of the SteamVR plugin. Following this, students create a simple virtual environment featuring some hand-sized objects. Once they finished their designs, the tutor demonstrates how to import the SteamVR plugin from the Unity Asset Store and how to place the included player prefab inside of the scene. This allows them to navigate through the virtual environment. To allow navigation over greater distances, the tutor introduces the concept of teleporting points and areas. Finally, students learn to add the “Throwable” script of SteamVR to the hand-sized objects, thus implementing a direct selection and manipulation.
With the end of the introductory phase, the tutorial changes its format to a weekly workshop. The subsequent sessions allow students to work on their own projects in groups and under supervision. At this point of the semester, students mostly have developed their conceptual designs and start with the implementation.
In the early weeks of the workshop phase, students often ask questions about the implementation of basic functions and solutions that go beyond the ones addressed during the tutorial, e.g., realizing animations. The tutor supports the students by showing them further basic features of Unity and directing them to further sources of information, e.g., documentations, web-based tutorials or videos.
As the students progress through the development, they start to write own scripts. Since this requires a lot of practice, the tutor collaboratively analyzes and repairs their code. This leads to a notable gain in technological skills as students start to produce more advanced code over time.
Since the workshop takes place in a lab, it also acts as a test environment. Here, students have access to the targeted platforms, thus allowing them to test and polish their projects.
We evaluated the educational concept of combining the seminar with our tutorial using a pre-test post-study design. At the beginning of the winter semester 2019/20, we asked the students attending the seminar whether they like to voluntarily participate in a study. All volunteers gave their written consent to participate in the study. Subsequently, during the first introductory session of the seminar, participants filled in a short questionnaire. The questionnaire consisted of three subcomponents assessing a participant’s attitude with respect to 1) using VR/AR for educational purposes, and competencies as well as content knowledge with regard to 2) media didactics, and 3) technology. This assessment was repeated at the end of the lecture period to analyze the change in the participants’ attitude, content knowledge, and competencies.
The questionnaire used in the study consisted of three subcomponents. We administered the questionnaire in the common language at the location of the study.
For assessing the students’ attitude toward using VR/AR for educational purposes, we used the interest and utility subcomponents of the Teachers’ Attitudes Toward Computers (Christensen and Knezek, 2009) questionnaire. We adjusted the questions to the specific needs of this study by replacing the term “computer” with “AR and VR”. Furthermore, we added an additional question to assess the participants’ overall attitude toward using VR/AR for educational purposes: ”Overall, how do you assess the use of AR and VR applications in teaching?”
The final questionnaire consisted of 14 5-point Likert scales (5 = agree). The post-questionnaire featured two additional 5-point Likert scales (5 = agree) assessing a participant’s tendency to integrate VR/AR applications in their future teaching. Table 2 displays the questionnaire’s contents.
For evaluating the students’ media didactics content knowledge and competencies, we designed a specific media didactics questionnaire shown in Table 3. The assessment instrument is based on educational standards for different levels of competencies of student teachers in the field of media didactics (Tulodziecki, 2012; Tiede and Grafe, 2016). We adjusted the questions to the specific needs of this study by replacing the term “media” with “AR and VR”. Q1 - Q2 assess content knowledge with respect to conditions for media education, Q3 - Q5 target theoretical concepts, Q6 - Q9 focus the design of instructional solutions, Q10 - Q12 assess evaluation content knowledge, and Q13 - Q17 investigate a direct application of the media didactics content knowledge. The questionnaire uses a 4-point Likert scale (4 = agree) for each of the 17 questions.
For evaluating the students’ technological content knowledge and competencies, we identified and assessed multiple relevant aspects. Most importantly, we asked the participants whether they possess a general understanding of the functionality of applications targeting VR/AR (Koehler and Mishra, 2009). We also asked the participants to rate their content knowledge and competencies with respect to usage of VR/AR devices and software. This knowledge is important for a successful integration of these applications in teaching concepts (Knezek and Christensen, 2016). As a third aspect, we identified and assessed knowledge with regard to using game engines for a development of VR/AR applications (Bacca et al., 2014; Kerawalla et al., 2006; Tulodziecki, 2017). Finally, participants rated their knowledge in usability. This provided insights into their competencies with regard to underlying principles for a good user interface design (Kerawalla et al., 2006). The technology subcomponent consisted of nine 4-point Likert scales (4 = agree) and is displayed in Table 4.
23 students completed the course held during the winter semester 2019/20 at the University of Würzburg. Seven of them were enrolled in the HCI program and 16 of them studied to become a teacher. Over the course of the seminar, they formed four interdisciplinary groups of which each developed a VR/AR applications targeting a STEM topic.
Of the 23 students, only nine volunteered to take part in our study. Four of them were pre-service teachers (all females) and five of them were studying HCI (1 female, 4 males). On average, the pre-service teachers were in their fourth study semester (
To evaluate the questionnaire, we computed the mean of the average total score for each of the subcomponents. We used non-parametric tests for the evaluation due to the ordinal data gathered and the small sample size. Using the exact Wilcoxon signed rank test, we compared the ratings obtained at the beginning and at the end of the seminar. The effect size was determined by computing Pearson r.
Comparing items 1–14 of the VR/AR attitude subcomponent measurements (
Comparing the media didactics subcomponent measurements (
FIGURE 5. Comparison of the media didactics scores between the pre- and post-questionnaire across HCI students, pre-service teachers (PST), and all students. Error bars indicate standard deviations. *: p < 0.05, **: p < = 0.01.
We further analyzed whether the participants’ field of study had an effect on our measurements. The ratings of the pre-service teachers (
Comparing the technology subcomponent measurements (
FIGURE 6. Comparison of the technology subcomponent scores between the pre- and post-questionnaire across HCI students, pre-service teachers (PST), and all students. Error bars indicate standard deviations. *: p < 0.05, **: p < = 0.01.
We further analyzed whether the participants’ field of study had an effect on our measurements. The ratings of the pre-service teachers (
The empirical analysis suggests an overall positive effect of the seminar on the pedagogical and technological content knowledge, i.e., TPCK, and competencies of the students. However, with respect to their attitude of using VR/AR for educational purposes, we found no significant difference between the two points of measurement. While this is surprising, it could be explained by the already widespread utilization of VR/AR for education and entertainment. Participants most likely had contact to these technologies before the seminar and created their opinion on this topic. This opinion might have led to a strong personal stance toward these technologies. Despite the efforts of discussing positive aspects during the seminar, participants might have not been convinced to overcome their personal hesitations. Another potential explanation lies in the relatively short duration of the seminar. Although lasting for 15 consecutive weeks, the time might still be too short to change the attitude of the participants. This would indicate the importance of a continuous discussion of using VR/AR for educational purposes during pre-service teacher education.
The group of the pre-service teachers reported in the post-test to consider integrating AR systems in their future teaching. This outcome supports the general goal of the seminar. Pre-service teachers recognized the potentials of AR learning environments and felt confident with their operation to consider an integration in their teaching concepts. The neutral stance toward implementing VR systems in their future teaching could be explained by the current availability of VR devices in classrooms. While most students already own AR devices like smartphones, VR systems are rarely available in schools.
The analysis of the media didactics subcomponent revealed a significant improvement across all participants over the course of the seminar. This provides a first evidence for the validity of the structure, concept, and selection of learning contents of the seminar. Following the approaches of action-oriented teaching (Tulodziecki et al., 2017) and learning by design (Mishra and Koehler, 2003; Koehler and Mishra, 2005), the seminar successfully enabled students to acquire theoretical knowledge of media didactics and to apply it while designing their applications and relevant lessons. This significant improvement was also present when only focusing on the HCI students. In this way, our results provide evidence that the concept of the seminar not only supports the acquisition of interdisciplinary learning contents, but also supports an interdisciplinary composition of the students. For the HCI students, the acquired media didactics content knowledge and competencies could be a key component of their overall education. Knowledge in media didactics could be beneficial for their later careers, e.g., for developing educational software or providing tutorials explaining the functionality of a system. Surprisingly, the improvement on the side of the pre-service teachers was not significant. However, we found a large effect size despite having a very small sample of pre-service teachers. As a result, it is possible to assume that the seminar also had a strong positive effect on the media didactics content knowledge and competencies of pre-service teachers.
With regard to the technology subcomponent, we found a significant improvement across all participants over the course of the seminar. This further supports the effectiveness of the structure of the seminar, the used approaches of action-oriented teaching as well as learning by design, and the concept of our tutorial. After undergoing the tutorial, participants successfully designed their own VR/AR learning environments, thus learning about the relationship between technology, learning contents, and pedagogy. Also, our results confirm previous research demonstrating the effectiveness of the learning by design approach (Koehler and Mishra, 2005; Koehler et al., 2007; Delello, 2014). While not statistically different, the participants of the pre-service teacher group showed a strong increase in their ratings with a large effect size over the course of the seminar. Considering the small sample size, this result supports the potential effectiveness of our approach of educating pre-service teachers in integrating the technologies of VR/AR in teaching concepts. The slight improvement on the side of the participants of the HCI group could be explained with their previous knowledge in implementing VR/AR systems gained over the course of their studies.
Following the learning by design approach, the development of a VR/AR learning environment plays is a central component. Thus, we present the four projects that have been developed over the course of the seminar. Since a comprehensive analysis of the individual projects would be out of scope for this paper, we identify the implemented core interaction possibilities as well as the fulfilled aspects of serious games and learning paradigms (see section 3). In this way, the analysis reflects the development of an understanding for the complex relationship between technology and pedagogical practices, i.e., TPCK.
Over the course of the seminar, four different projects were developed. Each group had complete freedom in their design choices. The projects target the 1) anatomy of vertebrates (AR), 2) 2D geometry (AR), 3) climate zones (AR), and 4) production of ammonia (VR). Since the seminar bases on the learning by design approach, the projects provide a further insight into the effectiveness of the seminar.
Learning about the anatomy of vertebrates includes learning about the different organs and their functions. Horst–The Teaching Frog is an AR learning application targeting the learning of a frog’s anatomy (Oberdörfer et al., 2020, Oberdörfer et al., 2021).
Learners can virtually dissect a frog, closely inspect each organ as well as learn about its functions, and test their content knowledge in a quiz. Horst–The Teaching Frog bases on a large, but visually realistic soft toy of a frog named Horst. The frog is augmented with a pouch hiding various image targets, i.e., the frog’s organs. By cutting the frog open, i.e., opening a zipper at the frog’s belly, the image targets become visible and can be scanned using the camera of the smartphone as Figure 1 and Figure 7 display. Scanning the image targets places a 3D model of each organ above the respective target, thus visualizing the internal structure of the animal. As each image target is attached using a small piece of velcro, the frog can be further dissected allowing for a close inspection of each organ. By placing the image target of an organ next to a magnifying lens target, the application displays further biological details about the organ.
Horst–The Teaching Frog includes various modes either simulating the dissection, i.e., a guided dissection and a free mode, or testing a student’s knowledge in a quiz. Each mode also implements a gamification system. The system rewards users with achievements and highscores that are intended to increase a learner’s motivation to repeat the learning contents using the application.
With respect to the learning paradigms, the free mode of the dissection can be helpful to use the application in constructivistic teaching and learning environments in the classroom. The quiz as well as the individual analysis of the organs reflects aspects of the learning paradigm of behaviorism. Following the guided dissection, the application elicits aspects of cognitivism.
Learning about 2D geometry as well as coordinate systems is a difficult challenge for young pupils. They have to develop an understanding for the connection between a coordinate system and the position and dimensions of elements in it. The AR serious game ARes targets the training of this learning content.
The overall goal is to help the little dragon Ares to find its way out of a dungeon as shown in Figure 8. Each dungeon represents a mathematical exercise requiring learners to either determine the position of an object, the distance between two objects, or the angle to which a door has to rotate to allow Ares to fly through it. The exercises are given in form of large image targets, i.e., papery worksheets. Scanning such a target turns the exercise into one of the game’s dungeons. During the gameplay, a user can select objects by touching them and enter correct solutions about the object’s properties using a virtual keyboard. After having solved an assignment, a door inside of the dungeon opens and Ares escapes through it. Finally, ARes displays a summary screen showing a user’s numeric inputs. This debriefing allows to foster the understanding of the learning content (Crookall, 2011).
FIGURE 8. By solving mathematical puzzles, players guide the little dragon Ares through small dungeons. The gameplay fosters the development of an understanding for coordinate systems and 2D geometry.
Solving these mathematical puzzles rewards players with experience points and achievements. While the achievements provide an incentive to try out various approaches, the experience points are designed to cause a longterm motivation to repetitively play the game. These points can be exchanged for different skins of Ares in a virtual shop.
In summary, ARes mostly implements aspects of behaviorism, e. g, short levels and rewards, and partly of cognitivism, e.g., background story and direct application of the learning contents. It further follows core principles of serious games by mapping the application of the learning content to the integral game mechanics.
Developing an understanding for the various climate zones and the geographical structure of the Earth is a central learning goal of young pupils. The AR learning environment Globoli tries to assist students in acquiring this knowledge as Figure 9 illustrates.
FIGURE 9. Globoli displays a virtual Earth on the player’s desk. The overall goal is to assign various animals to the correct continent.
When playing Globoli, learners firstly need to scan the image target of the Earth resulting in the planet being displayed. Using a pinch gesture, users can scale the planet up or down as well as rotate it by touching the 3D model and moving the finger across the touchscreen. On the planet, each continent is intended to be an active area. However, the developing group only implemented Asia and Africa. By pointing with the cursor, i.e., a red dot, at a continent, it can be selected. The overall goal of using Globoli is to assign six animals to the correct continent. Animals are represented on additional image targets that can be placed next to the globe. As soon as the application has recognized a target, a 3D model of the respective animal is shown. By selecting a continent and subsequently touching an animal, learners assign it. Immediate feedback informs learners about the correctness of their selection.
In summary, Globoli mostly implements aspects of behaviorism. It segments the learning content into small units, i.e., the correct assignment of each animal represents an individual learning unit. This allows for a quick repetition of the learning content.
VRaccess deals with the learning content of producing ammonia using the Haber-Bosch process. Using VR, learners can immerse themselves in a virtual laboratory featuring all relevant instruments, e.g., a compressor, a heater, and a catalyst as shown on Figure 1. By directly interacting with the instruments, users can alter respective variables that directly affect the process and hence the purity of the results as depicted in Figure 10. This allows for a self-directed exploration and experimentation, thus targeting constructivistic learning in the classroom. The purity is indicated by the output molecules, green molecules represent ammonia whereas blue and yellow molecules visualize hydrogen and nitrogen.
FIGURE 10. By manipulating the scientific instruments inside the virtual laboratory, users adjust variables affecting the purity of the production of ammonia.
By pointing at an instrument using an inspect interaction, VRaccess displays central information about each instrument at a black board. This decision was made to assist the development of an understanding for the underlying chemical processes. By achieving various degrees of purity, learners unlock achievements that change the appearance of the laboratory. To foster collaborative learning and a reflection of the experiences, VRaccess also provides an external view featuring an overview of the concrete values set by the user immersed in the simulation.
In summary, VRaccess supports constructivistic and behavioristic, i.e., by using gamification, learning, follows the principles of serious games, i.e., it maps the application of the learning content to the integral game mechanics, and implements principles of 3D interface design, e.g., providing feedback compensation by highlighting interaction possibilities with objects as displayed in Figure 10 (LaViola et al., 2017).
The successful development of four learning applications in combination with the significant improvement of the technological content knowledge and competencies supports the concept of the seminar and our specific tutorial. This is an important outcome as it indicates that pre-service teachers and HCI students not only learned about the theoretical concepts during the seminar, but also managed to expand their technological content knowledge and to establish competencies by developing these applications. Each VR/AR learning environment elicits an educational design indicating the successful transfer of the pedagogical contents discussed during the seminar. The projects also reflect a sound technical implementation by providing interaction techniques allowing for a direct application of the encoded learning contents. The projects further follow the fundamental design of serious games by requiring the application of the encoded learning contents as user interactions (Oberdörfer and Latoschik, 2018). Based on these interactions, learners receive immediate feedback about the results of their actions. In this way, the design of the projects supports a successful learning of the relationship between technology, learning contents, and pedagogy, i.e., TPCK, as intended by the learning by design approach. Finally, as the pre-service teachers integrated the VR/AR learning environments in teaching concepts, all of the four projects could already be used in classroom teaching. This further confirms the approach of offering such an interdisciplinary seminar targeting a pedagogy and technology-based education.
The analysis of the effectiveness of the seminar as well as our tutorial was twofold: we empirically evaluated the acquisition of content knowledge as well as competencies and discussed the projects that have been developed during the seminar. The first study revealed a significant improvement in content knowledge and competencies of media didactics as well as technology, i.e., TPCK. Also, the pre-service teachers reported a positive tendency to integrate AR systems in their future teaching, while remaining neutral toward the integration of VR. This outcome supports the overall concept of the seminar. The pre-service teachers acquired content knowledge and competencies that are highly relevant for their future teaching but beyond their typical areas of study. The study of the VR/AR learning contents that have been developed during the seminar revealed theoretically grounded educational concepts and good technical realizations. This result suggests that the learners successfully applied the theoretical learning contents while developing their projects.
Overall, this validates the selection of the seminar’s theoretical learning contents as listed in Table 1 and the requirement of designing a VR/AR learning environment. In this way, the combination of providing and discussing theoretical learning contents while simultaneously requiring their application for designing VR/AR learning environments indicates to be a successful concept. The results might also have been positively influenced by the background of the lecturer and the tutor. The lecturer of the seminar has an interdisciplinary background and hence could explain the theoretical learning contents from the point of view of both disciplines. The tutor has an HCI and VR development background and thus is experienced with the realization of VR/AR projects. This might have increased the understandability of the presented information and facilitated the learning process.
Unfortunately, only nine of the 23 students volunteered to participate in our study. As a result, our sample size is rather small which becomes even more problematic when focusing on the two different groups, i.e., pre-service teachers and HCI students. This could have skewed our measurements. Despite revealing very promising results, it is important to conduct a larger study to confirm our initial measurements.
Also, we did not focus an assessment of the effects of the interdisciplinary composition of the project groups. The results indicate a strong learning effect with respect to media didactics in both groups and technological content knowledge as well as competencies on the side of the pre-service teachers. However, it remains unclear whether this is purely an effect of the learning contents discussed in the seminar or also affected by an interdisciplinary exchange during the collaborative phases. In addition, we did not test whether pre-service teachers can independently develop own VR/AR learning environments after having completed the seminar. Thus, it is important to analyze the effects of such an interdisciplinary composition on the learning outcome in future studies.
Finally, due to a lack of achievement tests with regard to media didactic competencies and technological skills, the measurement was based on self-assessment. In conjunction with a potential social desirability, this might have confounded the results. Future research need to focus on the development of VR/AR-specific standardized competency achievement tests to be used for the evaluation of pedagogical concepts using VR/AR in higher education.
In this paper, we presented the core concepts of an interdisciplinary seminar targeting the education of pre-service teachers and HCI students and in designing integrating technologies of VR/AR in teaching concepts. The seminar combines learning contents of pedagogical design of media, integration of VR/AR applications in teaching concepts, and technological qualities of good educational systems. As an overall goal, students develop and integrate an own VR/AR learning environment. We developed a specific accompanying tutorial guiding students through the developmental process. Attending the tutorial, students gain practical experiences, i.e., technological skills, necessary for a successful integration of VR/AR applications in teaching concepts.
Over the course of the winter semester 2019/20, HCI students and pre-service teachers attended the seminar. By analyzing the development in the attendees’ attitude with respect to 1) using VR/AR for educational purposes, and competencies and content knowledge with regard to 2) media didactics, and 3) technology, we evaluated the effectiveness of the seminar. Our results indicate a significant improvement in content knowledge and competencies of media didactics as well as technology, i.e., TPCK, and a positive tendency of pre-service teachers to integrate AR systems in their future teaching. However, the participants remained neutral toward the integration of VR. We further report four projects targeting STEM learning contents that have been developed during the seminar. These learning environments elicit a theoretically grounded educational concept and a good technical realization. This indicates a successful transfer and application of the theoretical learning contents of the seminar. The description of the seminar and tutorial can act as a guideline for the design of further interdisciplinary pedagogy and technology-based courses.
Future work should target a large-scale evaluation of the effectiveness of the approach presented in this paper. Also, it is important to analyze to what extent the interdisciplinary collaboration between HCI students pre-service teachers affected the learning outcome. Finally, it is critical to analyze to what extend HCI students and pre-service teachers benefit from the content knowledge and competencies acquired over a longer time.
The raw data supporting the conclusion of this article will be made available by the authors, without undue reservation.
Ethical review and approval was not required for the study on human participants in accordance with the local legislation and institutional requirements. The patients/participants provided their written informed consent to participate in this study.
SO, ML, and SG contributed equally to the conception of this research. SO and SB conceptualized and embedded the tutorial. SO and SB designed as well as conducted the user study, and analyzed the data. SO, SB, ML, and SG wrote the manuscript.
This research was performed within the VARyFAST project (Virtual and Augmented Reality together with the Franconian Alliance of Science and Technology), supported by the Bavarian State Governments program Digitaler Campus Bayern. This publication was supported by the Open Access Publication Fund of the University of Würzburg.
The authors declare that the research was conducted in the absence of any commercial or financial relationships that could be construed as a potential conflict of interest.
Anderson, E. F., McLoughlin, L., Liarokapis, F., Peters, C., Petridis, P., and de Freitas, S. (2009). “Serious Games in Cultural Heritage,” in Proceedings of the 10th International Symposium on Virtual Reality, Archaeology and Cultural Heritage VAST, Vallete, Malta, September, 2009 (Valletta, Malta, 29–48.
Azuma, R. T. (1997). A Survey of Augmented Reality. Presence: Teleoperators & Virtual Environments 6, 355–385. doi:10.1162/pres.1997.6.4.355
Bacca, J., Baldiris, S., Fabregat, S., Graf, S., and Kinishuk, (2014). Augmented Reality Trends in Education: A Systematic Review of Research and Applications. Educ. Technol. Soc. 17, 133–149.
Bucher, K., Blome, T., Rudolph, S., and von Mammen, S. (2018). Vreanimate Ii: Training First Aid and Reanimation in Virtual Reality. J. Comput. Educ. 6, 53–78. doi:10.1007/s40692-018-0121-1
Bucher, K., and Grafe, S. (2018). “Designing Augmented and Virtual Reality Applications with Pre-service Teachers,” in Proceedings of the 10th International Conference on Virtual Worlds and Games for Serious Applications (VS Games ’18), Würzburg, Germany, September, 2018 (Würzburg, Germany).
Bucher, K., Oberdörfer, S., Grafe, S., and Latoschik, M. E. (2020). “Von medienbeiträgen und applikationen - ein interdisziplinäres konzept zum lehren und lernen mit augmented und virtual reality für die hochschullehre,” in Schnittstellen und Interfaces - Digitaler Wandel in Bildungseinrichtungen of fraMediale (Munich, Germany: kopaed), 225–238.
Charsky, D. (2010). From Edutainment to Serious Games: A Change in the Use of Game Characteristics. Games Cult. 5, 177–198. doi:10.1177/1555412009354727
Christensen, R., and Knezek, G. (2009). Construct Validity for the Teachers’ Attitudes toward Computers Questionnaire. J. Comput. Teach. Educ. 25, 143–155. doi:10.1080/10402454.2009.10784623
Crookall, D. (2011). Serious Games, Debriefing, and Simulation/gaming as a Discipline. Simulation & Gaming 41, 898–920. doi:10.1177/1046878110390784
Cuendet, S., Bonnard, Q., Do-Lenh, S., and Dillenbourg, P. (2013). Designing Augmented Reality for the Classroom. Comput. Educ. 68, 557–569. doi:10.1016/j.compedu.2013.02.015
de Freitas, S., and Liarokapis, F. (2011). “Serious Games: A New Paradigm for Education?,” in Serious Games and Edutainment Applications. Editors M. Ma, A. Oikonomou, and L. C. Jain (London: Springer-Verlag), 9–23. doi:10.1007/978-1-4471-2161-9–“˙˝210.1007/978-1-4471-2161-9_2
Dede, C. J. (2009). Immersive Interfaces for Engagement and Learning. Science 323, 66-69. doi:10.1126/science.1167311
Delello, J. A. (2014). Insights from Pre-service Teachers Using Science-Based Augmented Reality. J. Comput. Educ. 1, 295–311. doi:10.1007/s40692-014-0021-y
Deterding, S., Dixon, D., Khaled, R., and Nacke, L. (2011). “From Game Design Elements to Gamefulness,” in Proceedings of the 15th International Academic MindTrek Conference: Envisioning Future Media Environments (MindTrek ’11), Tampere, Finland, September, 2011 (Tampere, Finland:ACM), 9–15. doi:10.1145/2181037.2181040
Dix, A., Finlay, J., Abowd, G. D., and Beale, R. (2004). Human-Computer Interaction. third edn.. London, England UK: Pearson Education Limited.
Driscoll, M. P. (2013). Psychology of Learning for Instruction. 3rd edn.. London, England UK: Pearson Education Limited.
Dunleavy, M., Dede, C., and Mitchell, R. (2009). Affordances and Limitations of Immersive Participatory Augmented Reality Simulations for Teaching and Learning. J. Sci. Educ. Technol. 18, 7–22. doi:10.1007/s10956-008-9119-1
Epic Games Inc (2021). Unreal Engine. [Dataset]Available at https://www.unrealengine.com/ (Accessed June 14, 2021).
Ericsson, K. A., Krampe, R. T., and Tesch-Römer, C. (1993). The Role of Deliberate Practice in the Acquisition of Expert Performance. Psychol. Rev. 100, 363–406. doi:10.1037/0033-295X.100.3.363
Freina, L., and Ott, M. (2015). “A Literature Review on Immersive Virtual Reality in Education: State of the Art and Perspectives,” in Proceedings of the 11th eLearning and Software for Education (eLSE ’15), Bucharest, Romania, April, 2015 (Bucharest, Romania).
Gall, D., and Latoschik, M. E. (2020). Visual Angle Modulates Affective Responses to Audiovisual Stimuli. Comput. Hum. Behav. 109, 106346. doi:10.1016/j.chb.2020.106346
Grafe, S. (2008). Förderung von Problemlösefähigkeit beim Lernen mit Computersimulationen: Grundlagen und schulische Anwendungen. Heilbrunn: Klinkhardt.
HTC Corporation (2011–2021). Htc Vive. [Dataset]Available at https://www.vive.com.
Kerawalla, L., Luckin, R., Seljeflot, S., and Woolard, A. (2006). "Making it Real": Exploring the Potential of Augmented Reality for Teaching Primary School Science. Virtual Reality 10, 163–174. doi:10.1007/s10055-006-0036-4
Kerres, M. (2018). Mediendidaktik: Konzeption und Entwicklung digitaler Lernangebote. 5th edn. Berlin/Boston: De Gruyter.
Khan, M., Trujano, F., Choudhury, A., and Maes, P. (2018). “Mathland: Playful Mathematical Learning in Mixed Reality,” in CHI’18 Extended Abstracts, Montréal, Canada, September, 2018 (Montréal, Canada).
Knezek, G., and Christensen, R. (2016). Extending the Will, Skill, Tool Model of Technology Integration: Adding Pedagogy as a New Model Construct. J. Comput. High Educ. 28, 307–325. doi:10.1007/s12528-016-9120-2
Knote, A., Fischer, S. C., Cussat-Blanc, S., Niebling, F., Bernard, D., Cogoni, F., et al. (2019). “Immersive Analysis of 3d Multi-Cellular In-Vitro and In-Silico Cell Cultures,” in 2019 IEEE International Conference on Artificial Intelligence and Virtual Reality, San Diego, CA, USA, December, 2019 (New York, NY: AIVR). doi:10.1109/AIVR46125.2019.00021
Knote, A., von Mammen, S., Gao, Y., and Thorn, A. (2020). “Immersive Analysis of Crystallographic Diffraction Data,” in 26th ACM Symposium on Virtual Reality Software and Technology New York, NY, USA, November, 2020 (New York, NY, USA: Association for Computing Machinery). doi:10.1145/3385956.3422097
Koehler, M. J., and Mishra, P. (2005). What Happens when Teachers Design Educational Technology? the Development of Technological Pedagogical Content Knowledge. J. Educ. Comput. Res. 32, 131–152. doi:10.2190/0ew7-01wb-bkhl-qdyv
Koehler, M. J., Mishra, P., and Yahya, K. (2007). Tracing the Development of Teacher Knowledge in a Design Seminar: Integrating Content, Pedagogy and Technology. Comput. Educ. 49, 740–762. doi:10.1016/j.compedu.2005.11.012
Koehler, M., and Mishra, P. (2009). What Is Technological Pedagogical Content Knowledge?. Contemp. Issues Teachnology Teach. Educ. 9, 60–70.
LaViola, J. J., Kruijff, E., McMahan, R. P., Bowman, D., and Poupyrev, I. P. (2017). 3D User Interfaces: Theory and Practice. Amsterdam, Netherlands: Addison-Wesley Professional.
Lv, Z., Tek, A., Da Silva, F., Empereur-mot, C., Chavent, M., and Baaden, M. (2013). Game on, Science - How Video Game Technology May Help Biologists Tackle Visualization Challenges. PLoS ONE 8, e57990. doi:10.1371/journal.pone.0057990
Makransky, G., and Lilleholt, L. (2018). A Structural Equation Modeling Investigation of the Emotional Value of Immersive Virtual Reality in Education. Educ. Technol. Res. Develop. 66, 1141–1164. doi:10.1007/s11423-018-9581-2
McGonigal, J. (2011). Reality Is Broken: Why Games Make Us Better and How They Can Change the World. 1st edn. New York: Penguin Press.
Mishra, P., and Koehler, M. (2003). “Not ”what” but ”how”. Becoming Design-wise about Educational Technology,” in What Do Teachers Need to Know (Greenwich: Information Age Publishing), 99–122.
Oberdörfer, S. (2021). “Better Learning with Gaming: Knowledge Encoding and Knowledge Learning Using Gamification,” Ph.D. thesis. New York, NY: University of Würzburg, 2021. doi:10.25972/OPUS-21970
Oberdörfer, S., Elsässer, A., Grafe, S., and Latoschik, M. E. (2021). “Grab the Frog: Comparing Intuitive Use and User Experience of a Smartphone-Only, Ar-Only, and Tangible Ar Learning Environment,” in Proceedings of the 23rd International Conference on Human-Computer Interaction with Mobile Devices and Services (MobileHCI ’21)Toulouse, France, September, 2021 (Toulouse, France.
Oberdörfer, S., Elsässer, A., Schraudt, D., Grafe, S., and Latoschik, M. E. (2020). “Horst - the Teaching Frog,” in Proceedings of the 2020 Mensch und Computer Conference Magdeburg, Germany, September, 2020 (Magdeburg, Germany:MuC ’20), 303–307. doi:10.1145/3404983.3410007
Oberdörfer, S., Heidrich, D., and Latoschik, M. E. (2019). “Usability of Gamified Knowledge Learning in Vr and Desktop-3d,” in Proceedings of the 2019 CHI Conference on Human Factors in Computing Systems (CHI ’19) Glasgow, Scotland UK, May, 2019 (Glasgow, Scotland UK:ACM), 1–175. doi:10.1145/3290605.3300405
Oberdörfer, S., and Latoschik, M. E. (2018). “Gamified Knowledge Encoding: Knowledge Training Using Game Mechanics,” in Proceedings of the 10th International Conference on Virtual Worlds and Games for Serious Applications (VS Games ’18) Würzburg, Germany, September, 2018 (Würzburg, Germany: ©2018 IEEE, 2018). Reprinted, with permission. doi:10.1109/VS-Games.2018.8493425
Oberdörfer, S., and Latoschik, M. E. (2019a). Knowledge Encoding in Game Mechanics: Transfer-Oriented Knowledge Learning in Desktop-3d and Vr. Int. J. Comput. Games Technol. 2019, 7626349. doi:10.1155/2019/7626349
Oberdörfer, S., and Latoschik, M. E. (2019b). Predicting Learning Effects of Computer Games Using the Gamified Knowledge Encoding Model. Entertainment Comput. 32, 100315. doi:10.1016/j.entcom.2019.100315
Perry, D., and DeMaria, R. (2009). David Perry on Game Design: A Brainstorming Toolbox. Boston, MA, USA: Course Technology.
Petko, D. (2012). Teachers' Pedagogical Beliefs and Their Use of Digital media in Classrooms: Sharpening the Focus of the 'will, Skill, Tool' Model and Integrating Teachers' Constructivist Orientations. Comput. Educ. 58, 1351–1359. doi:10.1016/j.compedu.2011.12.013
PTC Inc. (2021). Vuforia. [Dataset]Available at https://developer.vuforia.com (Accessed June 14, 2021).
Riegle, R. P., and Matejka, W. A. (2006). “The Learning Guild: Mmorpgs as Educational Environments,” in Proceedings of the 22nd Annual Conference on Distance Teaching and Learning, Madison, WI, USA, August, 2006.
Rogers, C. B., El-Mounaryi, H., Wasfy, T., and Satterwhite, J. (2017). Assessment of Stem E-Learning in an Immersive Virtual Reality (Vr) Environment. Comput. Educ. J. 8, 1–9. doi:10.18260/p.26336
Seaborn, K., and Fels, D. I. (2015). Gamification in Theory and Action: A Survey. Int. J. Human-Computer Stud. 74, 14–31. doi:10.1016/j.ijhcs.2014.09.006
Skarbez, R., Brooks, Jr., F. P., and Whitton, M. C. (2017). A Survey of Presence and Related Concepts. ACM Comput. Surv. 50, 1–39. doi:10.1145/3134301
Slater, M. (2017). “Implicit Learning through Embodiment in Immersive Virtual Reality,” in Virtual, Augmented, and Mixed Realities in Education. Editors D. Liu, C. J. Dede, R. Huang, and J. Richards (Singapoore: SpringerSmart Computing and Intelligence).
Slater, M. (2009). Place Illusion and Plausibility Can lead to Realistic Behaviour in Immersive Virtual Environments. Phil. Trans. R. Soc. B 364, 3549–3557. doi:10.1098/rstb.2009.0138
Slater, M., and Wilbur, S. (1997). A Framework for Immersive Virtual Environments (Five): Speculations on the Role of Presence in Virtual Environments. Presence: Teleoperators & Virtual Environments 6, 603–616. doi:10.1162/pres.1997.6.6.603
Steinhaeusser, S. C., Riedmann, A., Haller, M., Oberdörfer, S., Bucher, K., and Latoschik, M. E. (2019). “Fancy Fruits - an Augmented Reality Application for Special Needs Education,” in Proceedings of the 11th International Conference on Virtual Worlds and Games for Serious Applications (VS Games ’19), Vienna, Austria, September, 2019 (Vienna, Austria:IEEE).
Stevens, J. A., and Kincaid, J. P. (2015). The Relationship between Presence and Performance in Virtual Simulation Training. OJMSi 03, 41–48. doi:10.4236/ojmsi.2015.32005
Tiede, J., and Grafe, S. (2016). Media Pedagogy in German and u.S. Teacher Education. Comunicar: Media Educ. Res. J. 24, 19–28. doi:10.3916/C49-2016-02
Tulodziecki, G., Grafe, S., and Herzig, B. (2013). Gestaltungsorientierte Bildungsforschung und Didaktik in Theorie – Empirie – Praxis (Heilbrunn: Klinkhardt).
Tulodziecki, G., Herzig, B., and Blömeke, S. (2017). Gestaltung von Unterricht. Heilbrunn: Klinkhardt.
Tulodziecki, G., Herzig, B., and Grafe, S. (2019). Medienbildung in Schule und Unterricht in Vollst. Akt. U. Überarb. Auflage (Bad Heilbrunn: Klinkhardt).
Tulodziecki, G. (2012). “Medienpädagogische kompetenz und standards in der lehrerbildung,” in Jahrbuch Medienpädagogik (Springer Fachmedien Wiesbaden GmbH), 9, 271–297. doi:10.1007/978-3-531-94219-3_13
Tulodziecki, G. (2017). Thesen zu einem curriculum zur ”bildung in einer durch digitalisierung und mediatisierung beeinflussten welt”. medien + erziehung 61, 50–56.
Unity (2021). Unity. [Dataset]Available at https://unity.com (Accessed June 14, 2021).
Valve Coorperation (2015–2021). Steamvr Plugin. [Dataset]Available at https://www.assetstore.unity3d.com/en/#!/content/32647 (Accessed June 14, 2021).
Keywords: interdisciplinary education, virtual reality, augmented reality, serious games, learning environments, teacher education
Citation: Oberdörfer S, Birnstiel S, Latoschik ME and Grafe S (2021) Mutual Benefits: Interdisciplinary Education of Pre-Service Teachers and HCI Students in VR/AR Learning Environment Design. Front. Educ. 6:693012. doi: 10.3389/feduc.2021.693012
Received: 09 April 2021; Accepted: 14 June 2021;
Published: 30 June 2021.
Edited by:
Manpreet Kaur Bagga, Partap College of Education, IndiaReviewed by:
Alcinia Sampaio, Technical University of Lisbon, PortugalCopyright © 2021 Oberdörfer, Birnstiel, Latoschik and Grafe. This is an open-access article distributed under the terms of the Creative Commons Attribution License (CC BY). The use, distribution or reproduction in other forums is permitted, provided the original author(s) and the copyright owner(s) are credited and that the original publication in this journal is cited, in accordance with accepted academic practice. No use, distribution or reproduction is permitted which does not comply with these terms.
*Correspondence: Sebastian Oberdörfer, c2ViYXN0aWFuLm9iZXJkb2VyZmVyQHVuaS13dWVyemJ1cmcuZGU=
Disclaimer: All claims expressed in this article are solely those of the authors and do not necessarily represent those of their affiliated organizations, or those of the publisher, the editors and the reviewers. Any product that may be evaluated in this article or claim that may be made by its manufacturer is not guaranteed or endorsed by the publisher.
Research integrity at Frontiers
Learn more about the work of our research integrity team to safeguard the quality of each article we publish.