- 1PowerChina Northwest Engineering Corporation Limited, Xi’an, China
- 2College of Transportation Engineering, Nanjing Tech University, Nanjing, China
- 3Infrastructure Security Protection Research Center, Chongqing University Industrial Technology Research Institute, Chongqing, China
- 4Beijing Municipal Road and Bridge Building Material Group Co. Ltd., Beijing, China
- 5Institute of Coal Chemical Industry Technology China Energy Group Ningxia Coal Industry Co., Ltd., Ningxia, China
This study takes the bank collapse at the left bank of Badi Township of the Lidi Hydropower Station in the Lancang Area as an example. To address the lack of in-depth research on the prediction model and mechanisms of bank collapse, this study conducts field drilling surveys, investigates influencing factors, analyzes causal mechanisms, and proposes prediction methods based on the geological survey data of bank collapse in the reservoir area. The study explores the stratigraphic lithology, determines the key parameters for bank collapse prediction, and studies the failure modes and bank collapse mechanisms. It also proposes the finite element method of bank collapse to predict the width and elevation of bank collapse and provides the influence on the surrounding environment. The results show that the collapsed bank on the left bank of Badi Township is a high and steep soil-like slope, and forms the collapse and retreat type of failure mode after impoundment. The Kachukin method, the bank slope structure method, and the proposed finite element method are used to predict the width and elevation of the collapsed bank. The results indicate that the Kachujin method predicts a larger range of bank collapses and is biased towards safety. In contrast, the prediction ranges of the bank slope structure method and the finite element method are close. The bank collapse position is located in the middle and rear of the residential houses in Badi Township, which is consistent with the on-site investigation and has strong reliability. The predicted width of the bank collapse is about 34 m and the elevation is about 1,845 m. The research results can be directly applied to preventing and controlling bank collapse and have powerful practical application value.
1 Introduction
Reservoir bank collapse means that after the reservoir is filled with water, the groundwater level inside the bank slope also rises. The rock and soil masses are subjected to long-term erosion, soaking, wave erosion, and cyclical changes in water levels, which are coupled. This leads to a significant reduction in the strength of the rock and soil mass, a weakening of its structure, and changes in hydrological and engineering geological conditions. These factors induce deformation and destruction over a certain range of the slope, intensifying the occurrence of new slips, collapses, and retreat of the bank slope, forming a new reservoir bank shape that adapts to the latest environmental conditions, that is, reservoir bank reconstruction (Meng et al., 2024; Yang et al., 2023; Li et al., 2024; Zhang et al., 2024a). The collapse of the reservoir bank slope is a complex problem involving multiple fields such as engineering geology and environmental geology. It can harm the normal operation of the reservoir and the geological environment of the surrounding areas, resulting in a reduction of land resources in the reservoir area, serious soil erosion, forced relocation of surrounding residents, increased river siltation, induced the revival of ancient landslides, and caused serious hazards. It has become a major geological disaster affecting the economic development of the reservoir area and the property safety of the people around the reservoir (Chen and Peng, 2015; Qin et al., 2021; Zhang et al., 2024b). One of the most serious consequences of bank collapse is that it poses a serious threat to railways, roads, and buildings along the collapsed area, leading to the destruction of farmland and houses, traffic interruption, damage to public facilities, and other urgent social problems threatening the stability of life of people on both sides of the reservoir.
China’s large-scale water conservancy projects, in terms of the number of reservoirs and total storage capacity, have secured a leading position globally. During the operation of reservoirs, bank collapse is an inevitable major geological disaster, especially in the river reservoirs in the high mountain and canyon areas of the southwest, where the diversity of topography and landforms and the complexity of geological conditions, coupled with the active geological structure and the strong dynamic effect of reservoir water, have jointly contributed to the diversity of bank collapse phenomena. These collapse points are widely distributed and cover a large area of influence. The Lancang River Basin is primarily characterized by gorge-type reservoirs, with eight cascading hydropower stations planned and arranged in the river’s upper reaches, such as the Lidi, Toba, Wunonglong, and other large-scale hydropower stations mainly for power generation. According to the investigation, it was found that when the reservoirs in the basin were filled, as the water level in the reservoir rises, there may be varying degrees of local adjustments and deformations on the slopes, leading to new areas affected by landslides, deformed reservoir shores, and collapses. This has resulted in the subsidence of infrastructure, collapses, cracks, and deformations in some houses, severely affecting residents’ normal production and life. Lidi Hydropower Station is mainly engaged in power generation, according to incomplete statistics, there are as many as 10 collapsed bank sections of varying sizes in the area, most of which are located at the front edge of the existing landslide and the soil slope section.
Since the 1960s, scholars at home and abroad have already begun to focus on the problem of reservoir bank collapse (Duong and Do, 2019; Ji et al., 2021; Yao et al., 2024; Yin et al., 2022; Zhang et al., 2024c). Bank collapse has always been a very complex comprehensive geological phenomenon of bank slope stability, where the bank slopes are directly affected by reservoir water level, giving rise to various forms of reservoir bank reconstruction. Scholars have conducted comprehensive and in-depth research on aspects of bank collapse, including the influencing factors, the formation mechanism, the destruction mechanism, the collapse patterns and process, and the prediction methods.
Reservoir bank collapse is a complex geological dynamic evolution process, influenced and controlled by many factors. In practice, it is often difficult to meet the in-depth needs of bank collapse prediction due to the large scope of the reservoir bank, poor transportation conditions, and limitations of research methods (Ma et al., 2023a; Chen et al., 2022). The study of the mechanisms of bank collapse typically involves an in-depth investigation of the geological conditions and influencing factors at the collapse site, including the composition and structure of the slopes, morphological characteristics, fluctuations in reservoir water levels, wave action, long-term water immersion, and freeze-thaw cycles, among other factors (Wang et al., 2020; Zhang et al., 2022; Chang et al., 2023). Riverbank erosion is one of the most significant factors in reservoir bank collapse. The characteristics and factors of the collapse depend on hydrological factors (including flow velocity, wave height impacting the shore, and reservoir dynamics) and geological and topographical factors (the attitude of the eroded rock strata, the physical and mechanical properties of the soil, and rock mass, the uniformity of the eroded soil and rock mass, and the morphology of the slope). These two sets of factors determine the level and extent of the bank collapse. Wang and Qiao (2013) started from the perspective of river erosion to study the relationship between the loss of bank slope materials and the changes in river channel topography, proposing some models and methods for calculating the amount of erosion and predicting the bank slope morphology when reaching an equilibrium state. The most common triggers of bank collapse are the deepening of riverbed erosion and the scouring of the riverbank, both of which depend on the flow conditions. The greater the intensity of the water flow, the greater the likelihood and severity of bank collapse will correspondingly increase. Therefore, the occurrence of bank collapse is primarily constrained by the flow conditions, which are closely related to the flow and boundary conditions. The water flow conditions play a key role in the generation and development of bank collapse, being the main driving factor. Other factors, such as geological structure, stability of bank materials, and vegetation cover, typically, indirectly affect the severity of bank collapse by altering the water flow or boundary conditions.
Through extensive field investigations of landslides, bank collapses, debris flows, and other geological disasters at large hydropower stations in the Lancang River Basin, the mechanisms of bank deformation, failure, and instability can be classified into types such as erosion-weathering type, collapse type, sliding type, collapse type, and flow soil type (Zhang et al., 2018). The collapse type of failure mode can generally be divided into retreat after collapse, scouring wave ridge type, and collapse type. The sliding type can be divided into sliding along the base interface or potential sliding surface, surface sliding, and reactivation of ancient landslides. Currently, the classification research on bank collapse modes of reservoir banks at home and abroad is not systematic and perfect, and it is difficult to meet the needs of bank collapse analysis and research in the mountainous areas of Southwest China. Bank collapses in reservoirs in the mountainous areas of Southwest China are often not a single mode but a combination of multiple modes (Ji et al., 2021).
Wang et al. (2000) in the past 10 years, through in-depth investigation and research on dozens of reservoir bank collapse cases, proposed the “two-stage method” to guide the prediction and design work of the Waifeng Railway Line (connecting Waiyang and Fuzhou). Although the “two-stage method” is used to predict the width of bank collapse, in the application process, it still needs to be compared and analyzed with the Kachugin method to comprehensively evaluate its rationality and make a scientific choice. That is, the applicability of the “two-stage method” still needs to be further verified and confirmed through extensive practical application (Han et al., 2022). Ji et al. (2019) based on a deep systematic analysis of existing bank collapse prediction methods, combined with the characteristics of the slope structure of the Three Gorges Reservoir and the analog reservoir, proposed three bank collapse prediction methods, the slope structure method (Maihemuti et al., 2015; Yao et al., 2024; Yin et al., 2022; Huang et al., 2024a), the three-stage method, and the multiple regression analysis methods, forming prediction methods for sliding type bank collapse, collapse type bank collapse, and flow soil type bank collapse. Scholars (Ji et al., 2018; Zhou et al., 2020; Huang et al., 2024b) in the theoretical analysis of the collapsed bank, numerical simulation as an effective method is used to study the stability of bank collapse and predict the width and elevation of bank collapse. This paper, based on the traditional finite element analysis method, has developed a finite element analysis method suitable for soil bank slopes (Guo and Griffiths, 2020; Guo et al., 2020; Huang et al., 2024c).
After the reservoir was filled, the main bank collapses were found in the area of the No. 4 accumulation body at Nongdu and the front edge of the No. 3 accumulation body downstream of Badie Township. Both collapses have led to the subsidence of the foundation of the Dewen Highway, affecting local traffic. Additionally, partial erosion and collapse occurred on the near-water slope of the left bank of Badie Township, but it did not impact the residents. This paper takes the near-water slope on the left bank of Badie Township as an example, investigating the geological conditions, lithology of strata, and groundwater conditions at the collapse points. The most typical collapse section is selected as the geological prototype. Based on different water level elevations, the collapse mode and mechanism of the left bank of Badie Township are analyzed, and the collapse process and mechanism of the slope are studied. Prediction and evaluation methods for bank collapse, such as the Kachugin method, slope structure method, and the finite element method proposed in this paper, are used to predict the width and elevation of the bank collapse on the left bank of Badie Township. This provides a basis for decision-making in the prevention and control of bank collapse on the left bank of Badie Township, which is of great significance for the construction of local resettlement sites, the safety of the relocation of residents, and the promotion of sustainable economic development in the areas on both sides of the reservoir.
2 Reservoir bank collapse prediction methods and failure mechanisms
2.1 Reservoir bank collapse processes
In the upper reaches of the Lancang River Basin, eight cascade hydropower stations have been planned and arranged. After geological disaster surveys, various degrees of landslides, deformed reservoir shores, collapses, bank collapses, and other disaster types were found in the reservoir area. Influenced by different factors, the collapse deformation and destruction patterns and evolution processes in the reservoir area are also different. On-site investigations in the Lancang River found a large number of different bank collapse patterns and mechanisms caused by heavy rain erosion, reservoir water level fluctuations, and wind wave action in the accumulation bodies and slopes (Figure 1), which have caused serious damage to both the rear edge landslides and the traffic lanes as well as the resettlement sites for villagers.

Figure 1. Investigate the main failure modes of the Lancang River reservoir bank collapse. (A) Alluvial erosion bank collapse; (B) Scour-notched bank collapse; (C) retreat-type bank collapse; (D) tractional sliding-type bank collapse.
2.2 The collapse retreat type of bank collapse failure process and failure mechanism
Under the continuous scouring and erosion of water flow, the underwater slope gradually forms a relatively gentle erosion accumulation area, namely, a shoal. At the same time, the bank wall after the collapse will show a relatively steep slope, with the lower part usually exceeding 35°, and the upper part may even reach a steepness of 70°–80° in the short term. As relentless wind and waves persistently erode the shoreline, the deterioration of the reservoir bank escalates, prompting a steady recession of the water’s edge. Concurrently, the adjacent underwater shallows expand, mirroring the shoreline’s retreat. This process will cycle repeatedly until the bank wall above the water and the shallow area below the water reach a relatively stable state (Figure 2). At this stage, the destruction of the bank wall by the scouring action of water flow and waves has ended, and the reservoir bank begins to stabilize. In the instance of a collapse retreat type of bank erosion, it is distinguished by a rapid pace of withdrawal and an extensive territory over which the retreat occurs.
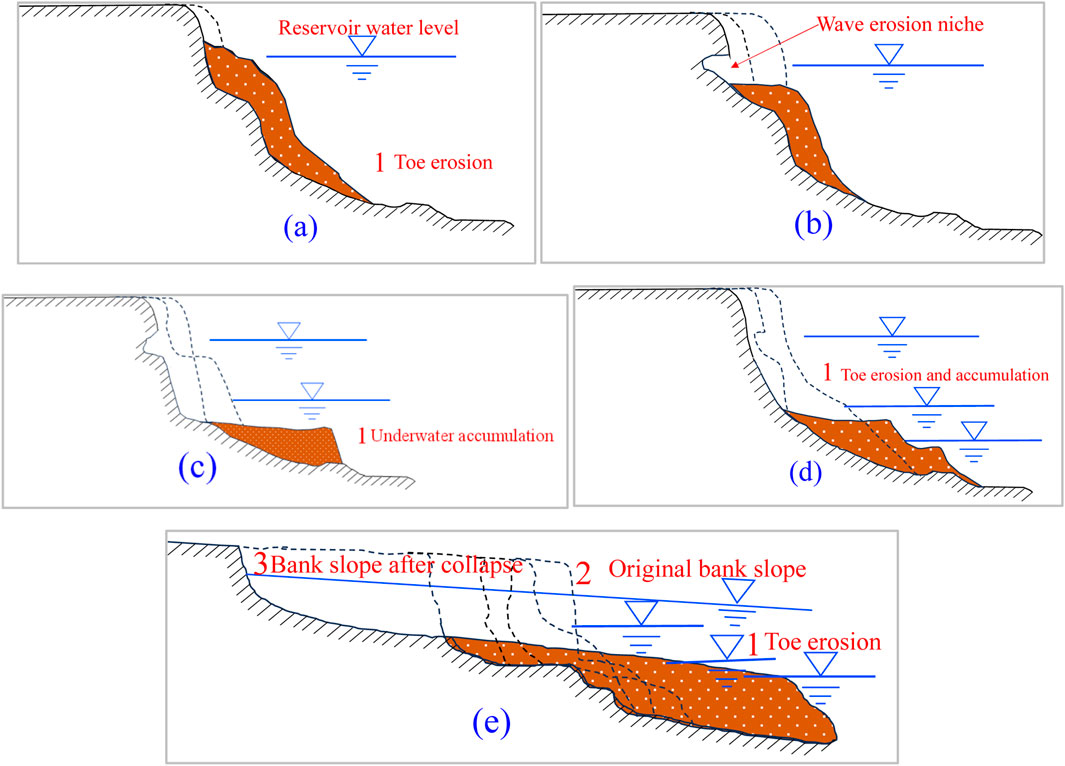
Figure 2. The evolution process of bank collapse with retreat-type. (A) The initial period of water storage; (B) Appearing wave erosion niches and the embryonic form of underwater shoals; (C) Appearing underwater shoals; (D) The bank wall recedes, and the shoal area expands. (E) Stable bank slope, expansion and termination of shallow shoals.
3 Engineering overview and causal mechanisms of the left bank of Badi Township
3.1 Engineering overview of the left bank of Badi Township
The bank collapse impact area on the left bank of Badi Township is located on the left bank of the reservoir area, opposite the Badie landslide, about 6 km away from the Lidi Hydropower Station Dam, and is the location of the Badie Township Government in Weixi County. This section of the reservoir bank is relatively open, forming a relatively wide valley section of the Lidi reservoir area. The left bank is a gentle slope of the floodplain accumulation terrace, while the right bank is a gentle slope terrace formed by the middle and lower parts of the Badie landslide. The Lancang River cuts through the terraces on both sides, forming a narrow “V” shaped river channel, with the water surface width near the normal water level of 115–180 m, and the flow direction changes from upstream to downstream from the SN arc to the EW direction. The upstream of the left bank terrace is the Gubu Migrant Bridge, and the downstream is the Badie Gully, which is irregularly shaped on the plane, with a maximum width of about 350 m. The terrain below the normal water level of the left bank terrace is relatively gentle, with a natural slope of 20°–28°. The terrain with a natural slope of 45°–52° is steeper from the normal water level of 1,818 m to the front edge of 1,840 m, and the natural slope of the terrace above 1,840 m is 8°–12°, which is mostly residential and office, commercial and other various building areas, with a small amount of farmland. The geomorphology before and after the water storage is shown in Figures 3, 4.
3.2 Analysis of the mechanisms and destruction of the bank collapse
Based on surface surveys and drilling, it is revealed that the geological strata within the site include the Quaternary Holocene artificial filling layer (
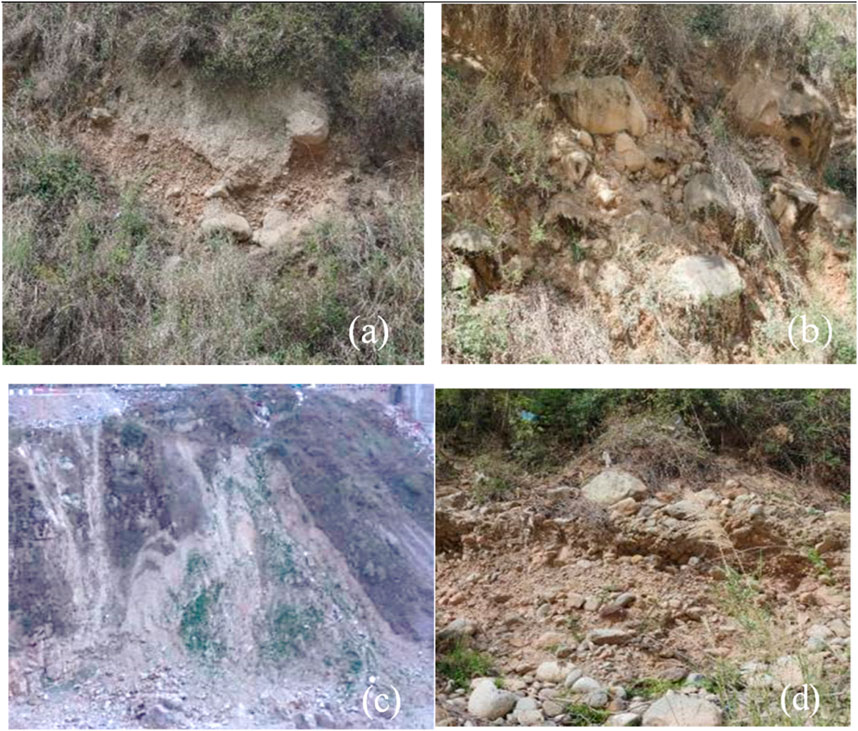
Figure 5. Overburden on the left bank of Badi Township. (A) The alluvial-diluvial strata exposed above; (B) The alluvial layer exposed at the edge of the highway; (C) The artificial accumulation next to the highway before water storage; (D) The alluvial sand and gravel along the riverbank before water storage.
4 Analysis of the mechanism and process of bank collapse
4.1 The phenomenon of bank slope deformation and destruction
Before the impoundment of the reservoir in 2018, the natural river water level during the flat-water period was below the foundation of the upstream side terrace, and only occasionally reached the foot of the covering layer during high flood levels. When the water level was high on the downstream side, the flood temporarily submerged the first-level terrace, with overall scouring being relatively minor, and the overall stability of the bank slope remained stable for a long time. From the time of reservoir impoundment in 2018 to the start of the rainy season in 2020, this section was generally in a mostly stable state according to patrols and monitoring, and no evidence of bank collapse or deformation had been discovered. At the beginning of 2020, it was found that local erosion had formed a pit-like bank collapse on the slope, while a resident living on the downstream side reported that the soil occasionally collapsed into the water with a loud noise in front of their house, which they believed posed a threat to their living (Figure 6A). During the flood season in 2020, the bank collapse continued to develop, and the resident reported that the walls of their house had cracks and deformation (Figure 6B).
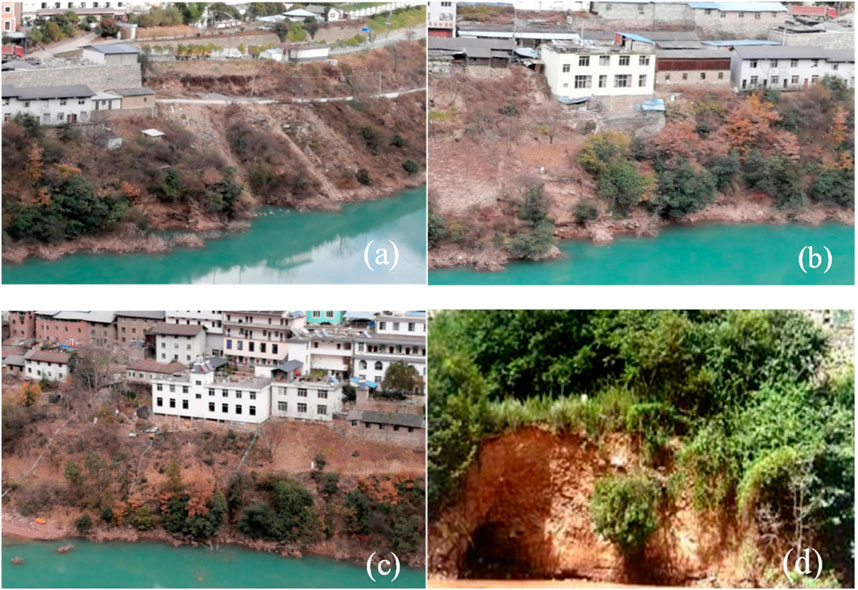
Figure 6. The geomorphological status of the bank collapsed on the left bank of Badi Township. (A) Bank collapse at the downstream side of the site; (B) Bank collapse in the middle of the site; (C) Bank collapse upstream of the site; (D) Bank collapse detail.
Based on on-site investigations, the bank collapse generally occurred in a pre-predicted position, which is different from the flake-like collapses in other parts of the reservoir area. The collapses in this area are mostly in the form of pit-like depressions on the plane, and the bank reconstruction is relatively slight, without exceeding the range predicted earlier. According to the investigation, there are 6 pit-like depressions caused by bank collapse, distributed over a length of 230 m and usually extended into the bank slope-like pockets. The diameter of the pits is generally small, with an average mouth width of 3–5 m, extending into the bank slope by 5–8 m, and the collapse height above the water surface is 1–3 m. The distance to the nearest house foundation is generally 20–30 m (Figures 6A–C). The most severe part of the bank collapse is located below the aforementioned residential area, with 3 pits. The largest pit is elliptical on the plane, slanting towards the downstream right bank along the long axis, with a mouth width of about 6–8 m, and it extends into the bank slope up to about 10 m. The maximum collapse height is about 5 m, near the water surface of the local area, is inclined, and the distance from the bank collapse to the house foundation is about 12 m. Coarse particles are estimated to be exposed on the section, and the content of fine particles is relatively small (Figure 6D).
The resident building is a 3-story building. According to the visit, the foundation was excavated and placed on a relatively compact alluvial layer, and no cracking phenomena were found in the early stage. Upon observation of the house walls, no cracks were found on the exterior walls, while there are some minor cracks on the interior walls, but no obvious signs of expansion were seen.
4.2 Analysis of the deformation mechanism of bank collapse
The current geomorphological status of the bank collapse on the left bank of Badixiang is characterized by typical collapse-type bank collapse features due to long-term erosion of the slope toe by the reservoir water level. The soil near the reservoir water level is softened and eroded, and the upper soil layer peels off layer by layer under the action of its weight. The overall water depth in front of the dam is about 30 m, and the shoreline is relatively straight. However, in terms of fine geomorphological details, there are many original terrain gullies and cuts in this area, where the water depth is relatively deeper, the water flow is prone to form backflow, and the current bank collapse deformation mostly occurs in these areas. From the longitudinal section, the original bank slope is a soil slope with a steep gradient (locally up to 50°–60°), and the stability of the relatively high and steep soil slope deteriorates after being soaked by the reservoir water level.
The upper Quaternary deposits exhibit a dual structure, with the surface layer being a silty loam dominated by fine particles and relatively weak permeability, while the lower part consists of sand and gravel with pebbles and cobble-sized particles, with a small amount of sandy and muddy materials filling the interstices. After water storage, under the action of wind waves and groundwater, processes such as scouring or subsurface erosion occur. The finer particles such as sand and silt within the pores of the gravel and pebbles are washed out, leaving the coarse particles to collapse into the water, forming niches on the scoured face. Over time, the upper soil layer also collapses into the water due to the lack of support. Since subsurface erosion is a slow process, no obvious signs of bank collapse were observed in the early stages of water storage.
From the perspective of hydrogeological conditions, the water in this section of the reservoir is generally deep, and the impact of waves and water currents on the bank slope is intense. The saturation of the soil by the reservoir water not only reduces the soil’s ability to resist sliding but also, when the water level drops, the resulting water level gradient and dynamic water pressure, due to the seepage effect, carry fine particles out of the slope, leading to the collapse of the coarse particles that are left unsupported. Analyzing the stress and strain conditions, changes in the stress conditions of the bank slope after water storage will inevitably lead to local stress adjustments.
After the reservoir water level rises, the water depth in this section is relatively deep, and the slope above the water surface is quite steep. Wind waves and water currents exert infiltration and erosion effects on the soil, causing the soil at the toe of the slope near the water storage level to be eroded into a concave shape. At the same time, due to the dynamic water pressure generated when the water level drops, fine particles in the soil are washed out, leaving the coarse particles unsupported, leading to the collapse of the surface soil. After the bank collapse occurs, its expansion speed and magnitude are relatively large. Since there are local concave terrains on the bank slope, the collapses in the affected area occur at these locations, exhibiting a minor pit-collapse type of bank collapse morphology.
5 Reservoir bank stability analysis and prediction
5.1 Constructing the calculation model and selecting parameters
The cross-sectional underwater topography at the bank collapse section has become less steep compared to the original terrain, with an overall slope of approximately 25° and minimal variations in undulation. In comparison with the original terrain, the equilibrium point is located near the elevation of 1818m (Figure 7). To evaluate the compaction and other physical and mechanical properties of the collapsing soil, a large number of exploration works were carried out in the reservoir area during the survey process of the Lidi Hydropower Station. In this survey, multiple sets of heavy dynamic penetration tests (N63.5) were conducted in two boreholes, and due to the presence of many large blocks, 23 sets are available for statistical analysis. Combined with the results of physical and mechanical tests of the soil and rock mass in other parts of the Lidi Hydropower Station reservoir and dam area, the physical and mechanical parameters of the soil and rock mass at the bank collapse section are proposed as shown in Table 1. To analyze and evaluate the bank collapse evaluation parameters of the site in the bank collapse impact area, a rough statistical analysis of the stable slope angle of the soil at the site and its surroundings was conducted, and the results are shown in Table 2.
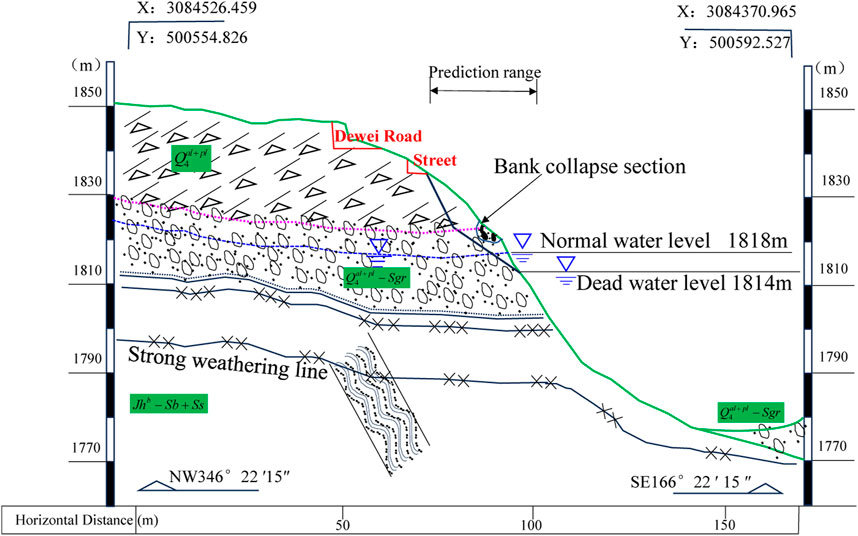
Figure 7. The cross-sectional profile of the bank collapse section on the left bank of Badixiang Township.
5.2 Calculation method and result analysis
The Kakuchin Method was first proposed by Kakuchin in 1949 as a reservoir bank collapse prediction method with practical application value. This method takes the point where the still water level is reduced by the wave erosion depth as the starting point, and it is suitable for predicting bank collapses in soil or loess-like soil layers, widely used in soil and loess areas of reservoirs. Through detailed on-site geological surveys, the collapse area is located on the downstream side of the terrace in Badixiang Township, with no bedrock exposure in the collapse area, and it generally belongs to a high and steep soil-like bank slope, where the Kakuchin method can be used for predicting the range of bank collapse.
Starting from the flood season of 2020, the bank slope gradually experienced continuous stripping collapse towards the bank. On 22 May 2021, the intensity of the bank collapse intensified, with the slope occasionally experiencing a stripping collapse of soil into the water, gradually developing into a retreat-type bank collapse similar to a pit collapse. For the prediction of bank collapses of this type, both the general two-stage method and the bank slope structure method are very applicable.
5.2.1 The Kakuchin method
The starting point of the Kakuchin Method is the design low water level minus the wave erosion depth, and the boundary point is the maximum wave erosion height. The reservoir bank slope is divided into above-water and underwater parts. Based on this boundary point, the appropriate above-water stable slope angle and underwater stable slope angle are selected to predict the width and elevation of the bank collapse (Ma et al., 2023b; Zhang et al., 2024d), as shown in Figure 8. The Kakuchin method predicts the width of the reservoir bank collapse as in Formula 1:
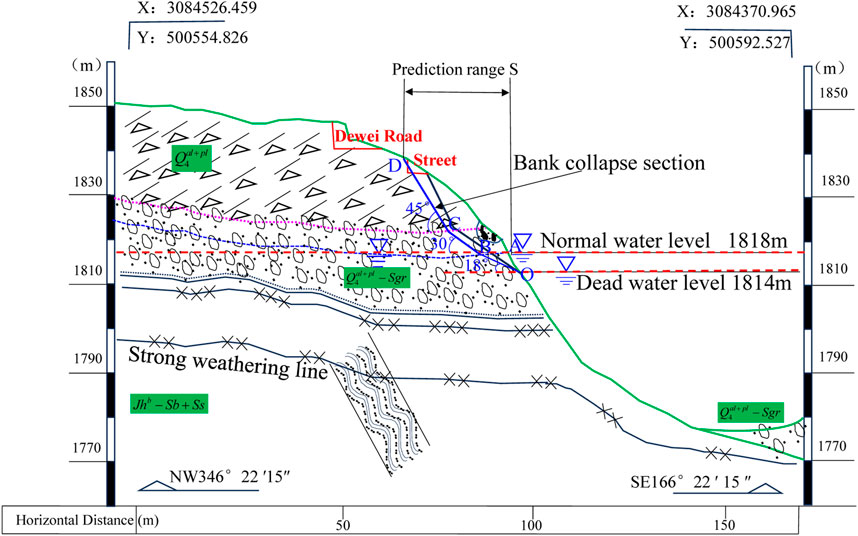
Figure 8. Prediction of bank collapse at the left slope of Badi Township by bank slope structure method.
5.2.2 The bank structure method
Based on the previously determined parameters for bank collapse prediction, the width of the bank collapse on the left bank of Badixiang Township is predicted using the universal bisection method. The universal two-stage method only considers the dead water level and the normal water storage level, and the prediction parameters only take into account the erosion angle and the above-water stable slope angle. Using the dead water level as the starting point for the bank collapse, the specific prediction steps for the width of the bank collapse on the left bank of Badixiang Township are as follows:
(1) Draw the calculated stratigraphy and geological section of the bank slope where the left bank of Badixiang is located, as shown in Figure 8.
(2) Indicate the normal water storage danger level (normal water storage elevation 1818.0 m) and the dead water level line (design low water level 1,814 m).
(3) Starting from the intersection point O of the dead water level line with the slope surface profile, use the scouring angle of the loose covering layer soil
(4) Taking point A as the starting point, with the scouring angle of sand and gravel, crushed stone soil
(5) Taking point B as the starting point, with the stable slope angle of the loose covering layer above water γ=45° as the inclination, draw the bank collapse line to intersect with the topographic line at point C. BC is the predicted bank collapse line above the normal water storage level.
(6) The horizontal distance between points C and F, denoted as S represents the final predicted horizontal impact width of the bank collapse. According to the elevation on the geological profile, the elevation at point C is the expected impact elevation for the bank collapse profile.
After the Lidi Hydropower Station is filled to an elevation of 1818.00 m, the covering layer soil between elevations 1814.00 m and 1818.00 m will become unstable and collapse under the action of reservoir water level fluctuations and waves, resulting in frontal bank collapse. Taking a typical section of the bank slope, as an example, the final bank collapse elevation is calculated to be 1843.0 m, with a bank collapse width of approximately 35.24 m. The houses of the residents of Badixiang Township and most of the Dewen Highway are distributed between elevations 1840.00–1850.00 m. It is predicted that after the normal water storage of the reservoir, bank collapse and reshaping within this reservoir bank range will occur, which may endanger the safety of public rental housing construction and disrupt road traffic safety.
5.2.3 Finite element method proposed in this paper
The collapses occurring in loose accumulation bodies all manifest step-by-step, and based on the actual analysis of more than 20 cases of bank slope collapses in the Lidi Hydropower Station reservoir area of the Lancang River Deqin-Weixi section, a set of prediction methods for the range of collapses induced by different controlling factors is explored.
1. Analysis of the Main Controlling Factors Affecting the Range of Reservoir Bank Collapse.
The factors considered in predicting the range of collapses mainly include:
Hydraulic Conditions: Collapses are caused by frequent changes in reservoir water levels, with both rising and falling water levels affecting the stability of the bank slope. Therefore, the impact of the reservoir water level is mainly reflected in two aspects, First, the change in the water level difference of surface water affects the stability of the bank slope, with the indicative index represented by the elevation difference
The composition of slope materials: This includes the material components that make up the slope (particle size composition), degree of cementation, compaction, etc., which reflect the physical and mechanical properties of the slopes. The indicative index can use strength parameters, namely the dimensionless cohesion strength ratio (c/γH), the internal friction angle
The geometric form of the slope: The main factor for the stability of loose accumulation bodies is the steepness and height of the terrain. The indicative index is the slope angle
2. Prediction equation of bank collapse stability based on the circular sliding surface search.
Combining the above analysis of the bank collapse range of various loose accumulation bodies with different causes in the Lidi Hydropower Station reservoir area (a total of 20 sites), representative cross-sections of bank collapses are selected, and different controlling factors related to the collapses are listed. The relationship curves for slopes with angles less than 45° show a linear change, while the relationship curves for slopes with angles greater than 45° all have a clear turning point of the safety factor. Under different foundation depth ratios, slopes are divided into gentle slopes (
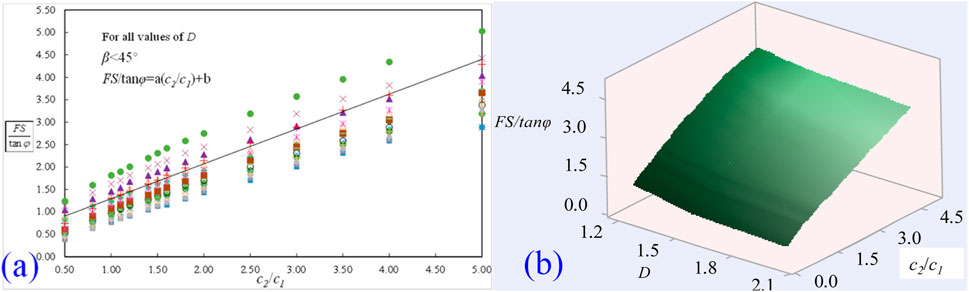
Figure 10. The relationships of flat slopes have three influence factors. (A) The relationship between strength parameters and slope angle with stability; (B) The relationship between strength parameters, foundation depth ratio, and stability.
From the regression equation, it can be derived that the stability of loose accumulation bodies or soil slopes in the Lidi Hydropower Station reservoir area is positively correlated with the strength ratio of different soil layers, and negatively correlated with the terrain slope angle
Selecting the bank collapse section on the left bank of Badixiang Township, as shown in Figure 11, the comprehensive internal friction angle of the covering layer at the collapse section is approximately 42° < 45°. The stability of the bank collapse and the range of instability can refer to Formula 2, and based on the parameters in Tables 1, 2, the position of the potential sliding surface and the morphology of the sliding surface can be calculated, as shown in Figure 12.
The potential sliding surface of the front edge bank collapse at the Cuzhong resettlement site can be seen in Figures 11, 12. The bank collapse area takes into account the main loads such as the self-weight of the slope, the base of the upper building, and the static water pressure. Two critical sliding surfaces appear in the slopes, with the instability mode being the shallow and deep instability of the slopes. The deeper sliding arc is in contact with the base to form a deep instability mode, while the shallower sliding arc does not intersect with the base, is relatively shallow, and finally emerges and converges on the right bank of the slope. The safety factor FS of the shallow sliding surface is 1.058, which is basically in a state of poor stability to basically stable according to the standard of stable state division. The shallow sliding surface is located near the main road of Badixiang Township, and the deep critical sliding surface is mainly concentrated on the Dewen Highway at the top of the slope. The estimated final bank collapse elevation is 1843.0 m, and the bank collapse width is about 33.754 m. The calculation results are quite consistent with the actual collapse situation, and the collapse and sliding are mainly concentrated on the main road inside the front edge.
If this slope is not systematically and permanently treated to greatly enhance its resistance to erosion and self-stability, it can be judged that with the passage of time, the slope will further deform and relax, or when the environmental conditions change, the risk of overall instability of the slope is still large, especially under abnormal conditions such as heavy rain and earthquakes. The potential sliding surface may develop into a deeper sliding surface, which may lead to the collapse and destruction of the housing in Badixiang Township.
5.3 Comparison of calculation results
5.3.1 Comparison results
The engineering region belongs to the Dianxi Mountain and Plain geomorphological unit, located in the Sanjiang Trough Fold System I-level tectonic unit. It has been determined the bank slope gradient, slope surface morphology, valley cutting condition, as well as the composition of the bank material, bank slope structure, and the distribution of special strata. Three different methods were used to predict the range of bank collapse, and the calculation results are displayed in Table 3.
Comparing the calculation results in Table 3, the Kakuchin method predicts a larger width and elevation, with a larger bank collapse range, and the results are biased towards safety. The bank slope structure method and the finite element method used in this paper predict similar final widths and elevations of the bank collapse, with not much difference, indicating that these two methods are more appropriate and the results are reliable. The locations predicted by the bank slope structure method and the finite element method in this paper are also relatively close, located in the middle and slightly towards the rear of the housing in Badixiang Township, which is consistent with the on-site investigation results.
5.3.2 Impact of bank collapse on the surrounding environment
The left bank of Badixiang Township is a broad terrace formed by flood and alluvial deposits. The front edge of the covering layer has a steep slope, which, after water storage, experiences slight pit-collapse type bank collapses due to the weakening of the soil’s physical and mechanical properties and seepage effects. The overall range of the bank collapse is not large and is in line with the early predictions.
According to the analysis, it is unlikely that the natural state of the left bank slope of Badixiang Township will produce a large-scale bank collapse, and the possibility under heavy rain conditions is also not significant. However, there is a potential for a large-scale bank collapse on the front edge under earthquake conditions. Most of the residential houses in this area were newly built during the construction period of the Lidi Hydropower Station and are located near the top of the slope. The load of the buildings has a certain impact on the stability of the slopes.
The analysis suggests that, given the current minor bank collapses and considering the proximity to a resident’s house downstream, which has affected the residence’s safety, it is recommended to relocate and resettle. At the same time, it is recommended to take appropriate protective measures for the front edge of the slope and to strengthen the inspection and monitoring measures during the operation period of the power station.
6 Conclusion
This study focuses on the left bank collapse in Badi Township located in the reservoir area of Lidi Hydropower Sta-tion as its research subject. It investigates the collapse modes and mechanisms, proposes the finite element method of this paper, and predicts the width and elevation of the collapse. The main conclusions are as follows:
(1) The terrace where the collapse section is located is a river alluvial fan of the third order, with a flat surface topography and a higher and steeper soil-like slope at the front edge. The groundwater in the collapse area is mainly pore water in the covering layer, receiving lateral replenishment.
(2) After impoundment, the soil bank slope was eroded by wind and waves, forming a retreat-type collapse. In May 2021, it collapsed again under the inducement of rainfall and human activities.
(3) The Kakuchin method predicts a larger range, biased toward safety, while the bank slope structure method and the finite element method of this paper predict a similar range, with strong reliability. The expected collapse width is about 34 m, and the elevation is about 1,945 m.
(4) Considering the safety of nearby residences, relocation and appropriate protective measures for the front edge of the slopes are recommended, along with strengthened inspection and monitoring during the power station’s operation period.
Data availability statement
The original contributions presented in the study are included in the article/supplementary material, further inquiries can be directed to the corresponding author.
Author contributions
XA: Conceptualization, Project administration, Writing–original draft. SF: Conceptualization, Funding acquisition, Writing–review and editing. GH: Investigation, Methodology, Writing–review and editing. HB: Methodology, Validation, Writing–review and editing. HH: Formal Analysis, Visualization, Writing–review and editing. ZW: Data curation, Validation, Writing–review and editing.
Funding
The author(s) declare that financial support was received for the research, authorship, and/or publication of this article. The authors gratefully acknowledge the support of the Nature Science Foundation of China of Jiangsu Province (NO. BK20230334), the National Nature Science Foundation of China (NSFC NO. 42407258), and the Fellowship of China Postdoctoral Science Foundation (NO. 2021M701688 and 2024M750682), Natural Science Foundation of Chongqing (Grant No. CSTB2024NSCQ-MSX1040).
Conflict of interest
Authors XA, G-HJ, HB, and HH were employed by PowerChina Northwest Engineering Corporation Limited. Author ZW was employed by Beijing Municipal Road and Bridge Building Material Group Co. Ltd. Author YH was employed by Institute of Coal Chemical Industry Technology China Energy Group Ningxia Coal Industry Co., Ltd.
The remaining author declares that the research was conducted in the absence of any commercial or financial relationships that could be construed as a potential conflict of interest.
Publisher’s note
All claims expressed in this article are solely those of the authors and do not necessarily represent those of their affiliated organizations, or those of the publisher, the editors and the reviewers. Any product that may be evaluated in this article, or claim that may be made by its manufacturer, is not guaranteed or endorsed by the publisher.
References
Chang, Z., Huang, F., Huang, J., Jiang, S. H., Liu, Y., Meena, S. R., et al. (2023). An updating of landslide susceptibility prediction from the perspective of space and time. Geosci. Front. 14 (5), 101619. doi:10.1016/j.gsf.2023.101619
Chen, M., Wei, W., and Jiang, Q. (2022). Use of quantile regression with fukui–okubo model for prediction and early warning of Reservoir Bank slope failure. Rock Mech. Rock Eng. 55, 7145–7169. doi:10.1007/s00603-022-02975-9
Chen, W. D., and Peng, S. X. (2015). Reservoir bank failure prediction. Beijing: China Water and Power Press.
Duong, T. T., and Do, M. D. (2019). Riverbank stability assessment under river water level changes and hydraulic erosion. Water 11 (12), 2598. doi:10.3390/w11122598
Guo, S. F., and Griffiths, D. V. (2020). Failure mechanisms in two-layer undrained slopes. Can. Geotechnical J. 57 (10), 1617–1621. doi:10.1139/cgj-2019-0642
Guo, S. F., Li, N., Liu, W. P., Ma, Z., and Lv, G. (2020). Influence of both soil properties and geometric parameters on failure mechanisms and stability of two-layer undrained slopes. Adv. Mater. Sci. Eng. 2020 (3), 1–13. doi:10.1155/2020/4253026
Han, Z., JianKang, C., Jian, K. Q., Liang, P., and Huibao, H. (2022). A fast prediction method for stability safety and reliability of Reservoir Bank rock slopes based on deformation monitoring data. Front. Earth Sci. 10, 944299. doi:10.3389/feart.2022.944299
Huang, F., Teng, Z., Yao, C., Catani, F., Chen, W., Huang, J., et al. (2024b). Uncertainties of landslide susceptibility prediction: influences of random errors in landslide conditioning factors and errors reduction by low pass filter method. J. Rock Mech. Geotechnical Eng. 16 (1), 213–230. doi:10.1016/j.jrmge.2023.11.001
Huang, F., Xiong, H., Jiang, S. H., Yao, C., Fan, X., Catani, F., et al. (2024c). Modelling landslide susceptibility prediction: a review and construction of semi-supervised imbalanced theory. Earth Sci. Rev. 250, 104700. doi:10.1016/j.earscirev.2024.104700
Huang, F., Mao, D., Jiang, S. H., Zhou, C., Fan, X., Zeng, Z., et al. (2024a). Uncertainties in landslide susceptibility prediction modeling: a review on the incompleteness of landslide inventory and its influence rules. Geosci. Front. 15, 101886. doi:10.1016/j.gsf.2024.101886
Ji, F., Liu, C., Shi, Y., Feng, W., and Wang, D. (2019). Characteristics and parameters of bank collapse in coarse-grained-material reservoirs based on back analysis and long sequence monitoring. Geomorphology 333, 92–104. doi:10.1016/j.geomorph.2019.02.018
Ji, F., Liu, C., Zhou, H., Liu, H., and Liao, Y. (2018). Identifying the influences of geological factors on reservoir bank collapse by a model test. Bull. Eng. Geol. Environ. 77, 127–139. doi:10.1007/s10064-016-0951-x
Ji, F., Shi, Y., Li, R., Zhou, H., Wang, D., and Zhang, J. (2021). Progressive geomorphic evolution of reservoir bank in coarse-grained soil in East China – insights from long-term observations and physical model test. Eng. Geol. 281, 105966. doi:10.1016/j.enggeo.2020.105966
Li, Y. H., Xu, X. Z., and Guo, S. L. (2024). Coupled effects of heavy rainfall and falling water-level exhibit the most significant influences on reservoir bank failures. Earth Surf. Process. Landforms 24 (5). doi:10.1002/esp.5883
Ma, X., Li, Q., Zhang, H., Gao, D., Long, J., and Li, Z. (2023a). Prediction of Reservoir Bank Collapse based on the limit equilibrium theory. Adv. Civ. Eng. 2023 (1), 1–9. doi:10.1155/2023/9050873
Ma, X., Li, T., Gao, D., Li, Q., Wang, C., Zhang, H., et al. (2023b). Study on prediction method of reservoir bank collapse in loess area. Bull. Eng. Geol. Environ. 82, 335. doi:10.1007/s10064-023-03368-0
Maihemuti, B., Wang, E., Hudan, T., and Xu, Q. J. (2015). Numerical simulation analysis of Reservoir Bank fractured rock-slope deformation and failure processes. Int. J. Geomechanics 16, 04015053. doi:10.1061/(ASCE)GM.1943-5622.0000533
Meng, F., Pei, H., Ye, M., and He, X. (2024). Stability analysis of reservoir slope under water-level drawdown considering stratigraphic uncertainty and spatial variability of soil property. Comput. Geotechnics 169, 106199. doi:10.1016/j.compgeo.2024.106199
Qin, Z., Lai, Y., and Tian, Y. (2021). Study on failure mechanism of a plain irrigation reservoir soil bank slope under wind wave erosion. Nat. Hazards 109, 567–592. doi:10.1007/s11069-021-04849-9
Wang, L., Guo, F., and Wang, S. (2020). Prediction model of the collapse of bank slope under the erosion effect of wind-induced wave in the Three Gorges Reservoir Area, China. Environ. Earth Sci. 79, 421. doi:10.1007/s12665-020-09169-y
Wang, M., and Qiao, J. (2013). Reservoir-landslide hazard assessment based on GIS: a case study in Wanzhou section of the Three Gorges Reservoir. J. Mt. Sci. 10, 1085–1096. doi:10.1007/s11629-013-2498-7
Wang, Y. M., Tang, J. H., and Ling, J. M. (2000). Study on prediction method for reservoir bank caving. Chin. J. Geotechnical Eng. 22 (5), 569–571. doi:10.3321/j.issn:1000-4548.2000.05.013
Yang, Z., Xi, W., Yang, Z., Shi, Z., Huang, G., Guo, J., et al. (2023). Time-lag response of landslide to reservoir water level fluctuations during the storage period: a case study of baihetan reservoir. Water 15 (15), 2732. doi:10.3390/w15152732
Yao, C., Li, L., Yao, X., Li, R., Ren, K., Jiang, S., et al. (2024). Study on the identification, failure mode, and spatial distribution of bank collapses after the initial impoundment in the head section of baihetan reservoir in jinsha river, China. Remote Sens. 16 (12), 2253. doi:10.3390/rs16122253
Yin, Y., Wang, L., Zhang, W., Zhang, Z., and Dai, Z. (2022). Research on the collapse process of a thick-layer dangerous rock on the reservoir bank. Bull. Eng. Geol. Environ. 81, 109. doi:10.1007/s10064-022-02618-x
Zhang, J., Lin, C., Tang, H., Wen, T., Tannant, D. D., and Zhang, B. (2024b). Input-parameter optimization using a SVR based ensemble model to predict landslide displacements in a reservoir area–A comparative study. Appl. Soft Comput. 150, 111107. doi:10.1016/j.asoc.2023.111107
Zhang, J., Tang, H., Tan, Q., Mao, M., Zhou, B., and Zhang, Y. (2024a). A generalized early warning criterion for the landslide risk assessment: deformation probability index (DPI). Acta Geotech. 19, 2607–2627. doi:10.1007/s11440-023-02199-3
Zhang, K., Wang, L., Dai, Z., Huang, B., and Zhang, Z. (2022). Evolution trend of the Huangyanwo rock mass under the action of reservoir water fluctuation. Nat. Hazards 113, 1583–1600. doi:10.1007/s11069-022-05359-y
Zhang, S. L., Lv, P. F., Yang, X. G., Chen, X. Z., and Zhou, J. W. (2018). Spatiotemporal distribution and failure mechanism analyses of reservoir landslides in the Dagangshan reservoir, south-west China. Geomatics, Nat. Hazards Risk 9 (1), 791–815. doi:10.1080/19475705.2018.1480536
Zhang, W., He, X., Wang, L., Meng, X., and Liu, S. (2024c). Deterministic and probabilistic analysis of a multi-sliding zone reservoir bank landslide influenced by the combined effects of reservoir water level fluctuation and precipitation. Bull. Eng. Geol. Environ. 83, 176. doi:10.1007/s10064-024-03675-0
Zhang, W., Liu, S., Wang, L., Sun, W., He, Y., Wang, Y., et al. (2024d). The overall stability of a partially unstable reservoir bank slope to water fluctuation and rainfall based on Bayesian theory. Landslides 21, 2021–2032. doi:10.1007/s10346-024-02250-8
Keywords: reservoir bank collapse, soil slope, impoundment process, retreat type bank collapse, prediction method of bank collapse
Citation: An X, Guo S, Ju G, Bai H, Hu H, Wang Z and Hu Y (2024) Unveiling the prediction model and mechanism of the collapse of bank slope in the lancangjiang area. Front. Earth Sci. 12:1501648. doi: 10.3389/feart.2024.1501648
Received: 25 September 2024; Accepted: 14 October 2024;
Published: 28 October 2024.
Edited by:
Faming Huang, Nanchang University, ChinaReviewed by:
Yijiang Zhang, Nanjing Hydraulic Research Institute, ChinaZhihua Zhang, Changjiang River Scientific Research Institute (CRSRI), China
Copyright © 2024 An, Guo, Ju, Bai, Hu, Wang and Hu. This is an open-access article distributed under the terms of the Creative Commons Attribution License (CC BY). The use, distribution or reproduction in other forums is permitted, provided the original author(s) and the copyright owner(s) are credited and that the original publication in this journal is cited, in accordance with accepted academic practice. No use, distribution or reproduction is permitted which does not comply with these terms.
*Correspondence: Shuangfeng Guo, c2h1YW5nZmVuZ2d1b0BuanRlY2guZWR1LmNu