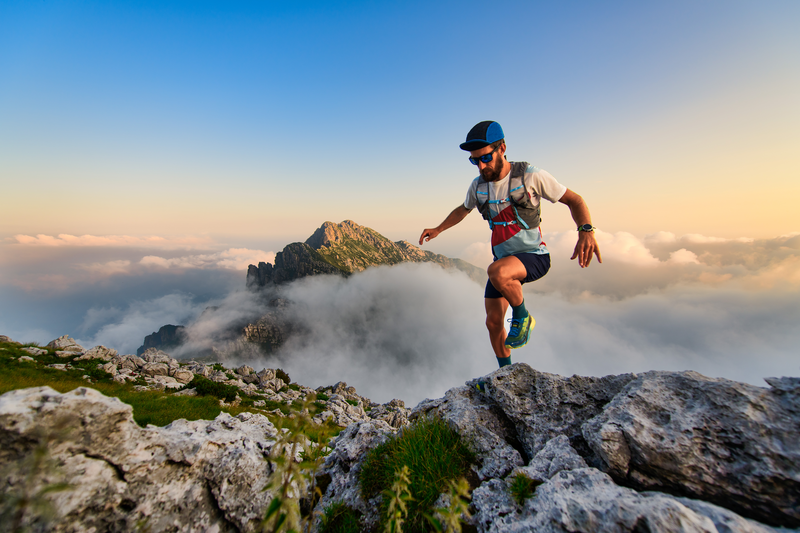
94% of researchers rate our articles as excellent or good
Learn more about the work of our research integrity team to safeguard the quality of each article we publish.
Find out more
ORIGINAL RESEARCH article
Front. Earth Sci. , 14 October 2024
Sec. Structural Geology and Tectonics
Volume 12 - 2024 | https://doi.org/10.3389/feart.2024.1474036
The Inner Northern Apennines (Italy) are a region with a dominant N-S to NNW-SSE fault system, but dissected and offset by several E-W to NE-SW trending structures and lineaments. The knowledge about the nature of these transverse structures, their origin, activity and role in current tectonic motions is limited and debated. To better establish the location, subsurface shape, and kinematics of faults related to the Livorno-Empoli lineament, one of the major transverse structures in the Northern Apennines, we analysed the seismicity in western Tuscany. In the Viareggio Basin we identified and relocated two distinct earthquake clusters as well as calculated 12 new focal mechanisms. The results show that the clusters consisted of several swarms from the years 2006, 2015, 2016 and 2021. The events had a depth between 2 and 15 km and were located along a NE-SW oriented, SE dipping fault system dissecting the Viareggio Basin. Focal mechanisms show oblique normal slip. We interpret the fault system to form a connection between the Viareggio Basin and the Lucca Basin to the east as well as continuing offshore. The results show that the transversal faults of the Inner Northern Apennines are seismogenic, with the length, position and onshore to offshore nature of the fault suggesting reactivation of pre-existing structures.
Transverse tectonic structures are orientated perpendicular or highly oblique to the main fault trend and are a common feature in different geologic settings. Such structures are generally not consistent with the Andersonian fault theory in that the fault characteristics are inconsistent with the regional stress field. They can be found in compressional settings such as the Alps (Laubscher, 1985; Viola et al., 2001; Zanchi et al., 2012) but also in ancient as well as tectonically active extensional areas, e.g., Rhine Graben Rift System, East African Rift, Basin and Range (Illies, 1972; Davis and Burchfiel, 1973; Chorowicz et al., 1989; Martin et al., 1993; Corti et al., 2018; 2022; Muirhead and Kattenhorn, 2018). Their morphology, scale, sense of slip motion can vary considerably. Some are active strike-slip faults, e.g., Garlock Fault (Davis and Burchfiel, 1973) while other structures can only be described as lineaments, inferred from the offset of faults and basins as well as changes within the local tectonic regime on either side of the lineament (Illies, 1972; Laubscher, 1985; Martin et al., 1993; Zanchi et al., 2012). On a larger scale transverse structures often form the border between different kinematic domains or control large scale segmentation of the tectonic system, and accordingly in extensional settings they often play a substantial role in basin development (Davis and Burchfiel, 1973; Gibbs, 1984; Laubscher, 1985; Chorowicz et al., 1989; Morley, 1995; Corti et al., 2018; 2022). Despite their varying nature and size, transverse structures are most commonly interpreted to be related to pre-existing structures, which may be reactivated in the new tectonic field (Illies, 1972; Laubscher, 1985; Chorowicz et al., 1989; Martin et al., 1993; Zanchi et al., 2012; Corti et al., 2018; 2022; Muirhead and Kattenhorn, 2018). Indeed, analogue models have shown that fault formation based on pre-existing structures or weakness zones does not strictly have to obey the Andersonian fault model, but reactivation is also possible with a less favourable orientation regarding the new stress field. Their orientation relative to the new tectonic field, however, influences their role in the newly forming system (Collettini and Sibson, 2001; Bellahsen and Daniel, 2005; Zwaan and Schreurs, 2017; Molnar et al., 2019; Maestrelli et al., 2020; Osagiede et al., 2021).
The Northern Apennines of Italy are tectonically active and the mountain crest marks the transition from orogenic compression in the east to upper plate extension in the west. Though the Northern Apennines mountain range is dominated by N-S to NNW-SSE oriented faults, several E-W to NE-SW oriented faults have been recognised of different size and importance – they follow the so called ‘antiapennine-orientation’ (Liotta, 1991; Sorgi et al., 1998; Pascucci et al., 2007; Viti, 2020; Molli et al., 2021). Despite the clear E-W offset of several N-S oriented basins and structures, as well as some transverse structures being traced across the entire Italian peninsula, these transverse structures are often only expressed as lineaments and their existence is inferred based on structural and stratigraphic considerations (Liotta, 1991; Sorgi et al., 1998; Nirta et al., 2007; Pascucci et al., 2007; Bonini, 2009; Brogi et al., 2014; Rosenbaum and Piana Agostinetti, 2015; Viti, 2020). The nature of these structures, their origin and role in current tectonic motions is debated (Sorgi et al., 1998; Nirta et al., 2007; Pascucci et al., 2007). Their current activity so far has mainly been interpreted from offset Quaternary strata and travertine deposits (Cantini et al., 2001; Pascucci et al., 2007; Brogi et al., 2014). Constraints from seismicity are few, for example, the 2013 June 21st Mw 5.1 Lunigiana earthquake has been attributed to an E-W striking structure north of the Apuan Alps (Molli et al., 2016).
In this study, we focus on the analysis of seismicity in the Viareggio Basin in the Northern Apennines adjacent to one of the main transverse structures of the Apennines, the Livorno-Empoli lineament (e.g., Cantini et al., 2001; Nirta et al., 2007; Pascucci et al., 2007; Rosenbaum and Piana Agostinetti, 2015) to establish the location, subsurface shape, and kinematics of the faults. We analysed the Italian Seismological Instrumental and Parametric Data-Base (ISIDe) (ISIDe Working Group, 2007) earthquake catalogue from the “Istituto Nazionale di Geofisica e Vulcanologia” (INGV) between 1985 and 2021 and located as well as relocated two clusters (onshore and offshore) near Pisa that were active between 2006 and 2021. We show that both clusters can be attributed to transverse E-W trending structures, showing that these anti-apennine faults are currently active and seismogenic, are steeply dipping, and show oblique normal-slip.
The Northern Apennines are a NW-SE trending northwards bent fold-and-thrust belt in northern to central Italy. Their development started in the Oligocene with the subduction of Adria-continental crust after the closure of the Alpine Tethys ocean (Late Cretaceous-Paleocene) (e.g., Boccaletti et al., 1971; Kligfield, 1979; Carmignani and Kligfield, 1990; Jolivet et al., 1990; Molli, 2008; Vignaroli et al., 2008; Argnani, 2012). Since then, the deformation front has been continuously moving to the north-east and has been replaced in the back by an extension dominated setting in the west, now called the Inner Northern Apennines (Figure 1A) (Carmignani and Kligfield, 1990; Argnani et al., 1997; Pascucci et al., 1999; Carmignani et al., 2004; Barchi, 2010; Loreto et al., 2021). The way in which the switch from compression to extension occurred in the Inner Apennines since the Miocene is still under debate (e.g., Boccaletti and Sani, 1998; Bonini et al., 2014). However, the current crustal structure, depicted by several geophysical studies (e.g., Ferretti et al., 2002; Mele and Sandvol, 2003; Li et al., 2007; Di Stefano et al., 2009; 2011; Piana Agostinetti and Amato, 2009; Di Stefano and Ciaccio, 2014), shows clear extensional characteristics west of the Apennines crest with a relatively thin crust of <20 km in some parts of the Ligurian and Tyrrhenian Sea (Di Stefano and Ciaccio, 2014; Dannowski et al., 2020). Along the Tyrrhenian coast of the southern part of the Northern Apennines the Moho is generally estimated to have a depth of 20–25 km (Ferretti et al., 2002; Mele and Sandvol, 2003; Li et al., 2007; Piana Agostinetti and Amato, 2009; Di Stefano et al., 2011; Di Stefano and Ciaccio, 2014) with a continuously thickening crust towards the east, reaching a maximum thickness of ∼40 km under the Apennines divide (Ferretti et al., 2002; Mele and Sandvol, 2003; Li et al., 2007; Piana Agostinetti and Amato, 2009; Di Stefano et al., 2011; Di Stefano and Ciaccio, 2014).
Figure 1. (A) Sketch of Northern Italy with main tectonic setting, the arrow is showing the general movement direction according to GNNS data. Inset showing the Italian Peninsula with the location of Fig. 1B. (B) INGV ISIDe earthquake catalogue of the Inner Northern Apennines and stress map (IPSI) with the seismic stations (University of Genoa, 1967; MedNet Project Partner Institutions, 1990; Istituto Nazionale di Geofisica e Vulcanologia, 2005; AlpArray Seismic Network, 2015) used for location and the orientation of the minimum horizontal stress (Shmin).
GNSS measurements show a relative north-eastwards movement of the Inner Northern Apennines with respect to the Eurasian plate, with some local variation showing a more northwards movement along the western side of Apennine chain (Bennett et al., 2012; Cenni et al., 2012; Viti, 2020; Serpelloni et al., 2022). The velocities decrease in a westward direction from the eastern side of the chain (3–4 mm/yr) to the Tyrrhenian coast (<1 mm/yr) (Bennett et al., 2012; Viti, 2020). However, as can be seen in Bennett et al. (2012), Cenni et al. (2012) and Viti (2020) the number of stations along the coast considered in each velocity field is very sparse. The NE movement is in line with the Italian Present-Day Stress Indicators (IPSI) (Montone and Mariucci, 2016; Mariucci and Montone, 2020; 2022) showing that the general minimum horizontal stress (Shmin) west of the water divide is NE-SW oriented (Figure 1B). However, the stress field changes eastwards to a NW-SE oriented Shmin on the outer mountain side (IPSI). Based on the stress distribution, the Inner Northern Apennines are generally assumed to be under extension in ∼NE-SW direction (Frepoli and Amato, 2000; Montone et al., 2004; Cenni et al., 2012; Montone and Mariucci, 2016).
The area of interest is located in the southern to central part of the Viareggio Basin, the only large basin north of the Arno river plain (Figure 2A). The NW-SE oriented extensional basin consists of an offshore and onshore part and in total is about 85 km long and up to 40 km wide (Bartole et al., 1991; Argnani et al., 1997; Pascucci et al., 2006; Molli et al., 2021). The basin fill reaches 3,500 m thickness of Neogene-Quaternary deposits (mostly marine sand and clay) resting unconformably on Oligocene to lower Miocene sandstones (Pascucci et al., 2006; Molli et al., 2021). The basin has a half-graben geometry with a SW-dipping listric master fault in its southern part. In the northern part the master fault is associated with a series of SW-dipping normal faults, creating a step like margin (Pascucci et al., 2006; Molli et al., 2021).
Figure 2. (A) Map of the Viareggio Basin with main normal faults (red lines and red dashed lines) (mapped after Molli et al. (2021)), available focal mechanisms (see text for details) and earthquake distribution (ISIDe Working Group, 2007). Coloured events occurred in years of increased seismicity (compare Fig. 2C) mostly consisting of the two discussed clusters. Events which are part of the relocated clusters are highlighted with a dark outline. (LEL: Livorno-Empoli-Liniament, NLF: Northern Lucca Fault, MP: Monte Pisano, GP: Guappero Fault, AP: Apuan Alps), (B) Number of earthquake per year 1985–2021, (C) Magnitude distribution by year 1985–2021, colour corresponding to Fig. 2A, (D) Gutenberg-Richter Plot for events after 2005 (b-value = 0.89)
The topographic borders in the north-east are the Mt. Pisani as well as the Apuan Alps north of them, both formed mostly by continental metamorphic units (Conti et al., 2020). The southern border is considered to be the anti-apennine Livorno-Empoli transversal lineament which, based on structural consideration, is thought to have acted as left-lateral strike-slip structure in the Quaternary (Nirta et al., 2007; Pascucci et al., 2007; Rosenbaum and Piana Agostinetti, 2015). Offshore the basin is bounded to the south by the Meloria Shoal fault which is interpreted to be the westwards continuation of the Livorno-Empoli lineament (Cantini et al., 2001; Molli et al., 2021). The northern and southern parts of the Viareggio Basin is assumed to be separated by another transverse structure, inferred from geophysical data, which possibly can be extended eastward into the Guappero fault line crossing the Mt. Pisani in the east (Pascucci et al., 2006; 2007) (Figure 2A).
We use the publicly available ISIDe record of seismic activity from 1985 to 2021 to understand the subsurface structure and kinematics of faults. The seismic network underwent major improvement to the number of seismic stations and accordingly number of recorded events in 2005 (Figure 2B) (Saccorotti et al., 2022), after which the catalogue can be considered complete above magnitude 1.2 (Figure 2D). The catalogue shows extensive seismicity within the Apennine mountain chain but a rather low seismic activity in the Inner Northern Apennines especially towards the coast (Figures 1B, 2A), where no large magnitude events have been recorded over the past century. Still, some distinct clusters were recorded. The existing focal mechanism in the region (from 1984, 2006, 2013 & 2022) (see Supplementary Material S1) (Pondrelli and Salimbeni, 2006; Scognamiglio et al., 2006), of which three are located offshore and one onshore, are in line with the general NE-SW oriented Shmin (Figure 2A). They all, except for one, display normal to oblique normal faulting, the exception being the only onshore event which shows a nearly vertical NW-SE oriented fault plane. The number of earthquakes recorded in the Viareggio Basin are not distributed evenly in space and time. Clear peaks in the seismicity rate occurred in 2006, 2015, 2016 and 2021 (Figure 2C). These peaks can be correlated with two distinct clusters consisting of 40 and 80 events. The first cluster, which we call the “Onshore Cluster”, is located about 10 km west of Lucca and north of Pisa, just at the southern foot of the Apuan Alps (Figure 2A). The second cluster, which we call the “Offshore Cluster”, is located, mostly offshore, about 15 km west to south-west of the Onshore Cluster. The magnitude of the events in the clusters is generally low with a maximum magnitude of 2.5 in the Onshore Cluster and 3 in the Offshore Cluster.
A limitation of the INGV catalogue is that the earthquakes are located using a single velocity model consisting of a two-layered crust of 11.1 and 26.9 km thickness (Vp 5 km/s and 6.5 km/s) plus a mantle layer (Vp 8.05 km/s) for the whole country (e.g., Scudero et al., 2021; Latorre et al., 2023). In addition, earthquakes are initially picked and located automatically, with only earthquakes above magnitude 2.5 undergoing an additional step of a detailed manual quality control and reprocessing (Scudero et al., 2021). As a result, the catalogue and especially small magnitude earthquake clusters, are commonly not well located and difficult to associate to known tectonic structures, with location errors at more than a kilometre scale (Scudero et al., 2021). Furthermore, the INGV generally only calculates focal mechanism for events ML ≥3.5, and therefore none are available for the clusters in question leading to a lack of structural information (Scudero et al., 2021).
In order to improve the INGV catalogue locations (Figures 3A, 4A), we located the two earthquake clusters using Hypo2000 utilising an improved velocity model appropriate for the region, and then relocated the clusters with the double difference relocation code HypoDD. Over the course of processing the number of events reduced from 120 to 68. Focal mechanisms for 12 earthquakes were calculated from P- and S-wave polarity measurements with the programme Focmec.
Figure 3. Earthquake distribution of the Onshore Cluster (A) INGV catalogue, (B) Location by Hypo2000 with maximum and mean horizontal error (transparent and grey circle (circle extend scaled to map scale)), (C) Relocation with HypoDD (horizontal error below marker size), (D) Newly calculated focal mechanism with T-trend distribution.
Figure 4. Earthquake distribution of the Offshore Cluster (A) INGV catalogue, (B) Location by Hypo2000 with maximum and mean horizontal error (transparent and grey circle (circle extend scaled to map scale)), (C) Relocation with HypoDD (horizontal error below marker size), (D) Newly calculated focal mechanism with T-trend distribution.
The identified clusters are part of the INGV ISIDe earthquake catalogue (1985–2021) (ISIDe Working Group, 2007). The initial earthquake location and arrival times were downloaded from the INGV database, for the Onshore Cluster from the Italian Seismic Bulletin (BSI) (Margheriti et al., 2016; Nardi et al., 2020; Latorre et al., 2022) and for the Offshore Cluster from the INGV Earthquake list (https://terremoti.ingv.it/, downloaded on: 22.11.2023). The station locations as well as the waveforms used for the focal mechanisms were downloaded from the INGV Database European Integrated Data Archive EIDA (Danecek et al., 2021; Mandiello et al., 2023).
The initial earthquake location was done with the programme Hypo2000 by Klein (2002) using an appropriate velocity model. To find the best model a variety of velocity models were considered based on their extent, layering structure and data source (e.g., Chiarabba and Frepoli, 1997; Ferretti et al., 2002; Li et al., 2007; De Luca et al., 2009). Eventually, a velocity model based on the 3D Vp/Vs grid model by Di Stefano and Ciaccio (2020), created as described in Di Stefano and Ciaccio (2014), was constructed and used for the earthquake location. This model was chosen partly due to the availability of discrete 1D models at 15x15 km grids size. The grid structure offered the possibility to calculate an average velocity model for an 8.000 km2 area covering both clusters as well as equally including the onshore and offshore area. The velocity model used in the location consists of four layers and can be seen in Table 1. We used Vp/Vs ratio of 1.7, consistent with the continental nature of the crust (Piana Agostinetti and Amato, 2009; Di Stefano and Ciaccio, 2014). The minimum numbers of stations used to locate an earthquake was 4. We tested several setups in Hypo2000 and eventually used settings shown in Table 2, which lead to the best RMS values.
We used the earthquake locations as an input for double difference earthquake relocation carried out with the programme HypoDD by Waldhauser and Ellsworth (2000). HypoDD is a common tool to improve earthquake location through relative re-location (e.g., Tan et al., 2011; Saccorotti et al., 2022; Raggiunti et al., 2023; Tan, 2024). Due to the double difference method, HypoDD is less dependable on the input velocity model and has more accurate relative event locations especially in relation to the event depths. The minimum numbers of links to define an event pair was set to eight and the minimum number of observations per event pair to 1, as suggested for small clusters by the HypoDD manual (Waldhauser, 2001). For the relocated we used singular value decomposition (SVD) approach for the Offshore Cluster, as this is the recommended approach for small clusters (Waldhauser, 2001). The number of events in the Onshore Cluster is too large for the SVD approach so the conjugated gradient method (LSQR) was used to relocate the entire cluster. As a quality control the individual swarms of the Onshore Cluster were relocated as subsets with SVD, which lead to comparable results and error estimation. The relocation for both clusters was done in 10 iterations during which the P- & S-phases were weighted 1 and 0.5, respectively. To remove outliers and misfits a dynamic cutoff of five was set and the maximal event separation was set to 15 km. These values were chosen according to the recommendation in the examples by Waldhauser (2001) and adjusted to the clusters. The relocation with HypoDD lead to a loss of events of 33% in the Onshore Cluster and 50% in the Offshore Cluster. (See Supplementary Material S2 for relocated solutions).
The focal mechanisms were calculated with the programme FOCMEC by Snoke (2003) and the beach balls created with the Matlab script “bb.m” (Boyd, 2023). The earthquake location, phase arrival azimuth and take-off angle were taken from the previous processing steps. To be considered for focal mechanism calculation the events needed to have a minimum station gap of 180° and at least a total of 14 P- and S-polarity recordings. We measured the polarity of the Sh & Sv waves on the transverse and radial component, respectively, after rotating the seismogram to the ray-path coordinate frame. The average variation in the calculated plane orientations was ± 15° for the strike and dip of the possible nodal planes (see Supplementary Material S1 for detailed focal mechanism solutions).
The Onshore Cluster activity was recorded from June 2015 until November 2021, with three distinct swarms occurring over 1–3 months in 2015, 2016 and 2021. Locating the Onshore Cluster with Hypo 2000 (Figure 3B) lead to an ∼E-W elongate cluster that is ∼5-km-wide by ∼9-km-long. With a spatial separation between a shallower set of events to the NW (2015 swarm) and a cumulation of events with increasing depth to the SE becoming visible. The relocation with HypoDD (Figure 3C) significantly improved the location errors from a mean horizontal error of 0.9 km in Hypo2000 to 0.2 km in HypoDD. The mean error in depth after the relocation with HypoDD is 0.3 km. The relocation lead to a clearer definition of the cluster in map view and in depth distribution, making the individual swarms even more distinguishable (Figures 3C, 5B). The depth distribution of the cluster is from 2 km to 15 km, with the 2015 swarm distinctly shallower than the other two swarms. Overall, a deepening of the events towards the SE is clearly visible. The cross-section furthermore shows the 2015 and 2016 cluster being aligned creating a steeply SE dipping, NE-SW striking fault plane (Figure 5D). The plane is curved and steepens upwards, becoming subvertical in the upper few kms.
Figure 5. Relocated earthquakes of the (A) Offshore Cluster and (B) Onshore Cluster with 2D cross-section (orange lines) and updated (red line) fault map (black lines) showing the depth distribution of the (C) Offshore and (D) Onshore Cluster in NW-SE and SW-NE direction (see Supplementary Material S1 for a 3D cross-section).
Nine focal mechanisms were calculated for the cluster (Figure 3D; Table 3). Most focal mechanism show NE-SW oriented normal fault with an oblique component that can be more or less pronounced, while three show a near vertical NE-SW striking fault. These three focal mechanisms are all in the shallowest part of the cluster and agree well with the steepening of the shallow part of the fault visible in the cross-section. The oblique components of the normal faults are right-lateral in the shallower 2015 events and left-lateral for the deeper 2016 and 2021 events. The T-Axis is NW-SE orientated, which is oblique to the regional recorded stress field as inferred for the area from the very sparse GNSS measurements and few past focal mechanisms.
The Offshore Cluster was predominately active over 2 weeks in August 2006. The earthquake location produced a swarm of ∼5 km x 6 km spatial extent consisting of 25 events (Figure 4B). The earthquake relocation (Figures 4C, 5A) of 15 events of the cluster improved the location errors from a mean horizontal error of 4.3 km in Hypo200 to 0.20 km in HypoDD and a mean vertical error of 0.8 km. It also improved the spatial definition of the cluster, reducing the cluster extend to ∼3-km-wide by 5-km-long, leading to a very narrow cluster. The depth distribution is from 3 to 13 km, with depth increasing towards the south. This increase in depth is clearly visible also in the cross-section (Figure 5C), which also delineates a possible fault dipping to the south or south-east and striking NE-SW. Three meaningful focal mechanism were calculated for the cluster (Table 3). All show normal faulting with generally E-W to NE-SW oriented planes and more or less pronounced right-lateral component (Figure 4D). This would agree quite well with the distribution visible in the cross-section. The T-axes of all focal mechanism are N-S oriented.
The results of the earthquake relocation improved the spatial definition of the two clusters. The map view and cross-section relocations, as well as the focal mechanisms show that the two clusters are both located along NE-SW striking, SE dipping faults. The clusters clearly show that these faults are seismogenic.
The orientation of the faults is consistent with that from previous studies on transversal structures in the region (e.g., Pascucci et al., 2007; Viti, 2020; Molli et al., 2021) of which one structure is supposed to be bounding the Viareggio Basin in the south and one crossing the middle of the basin (Pascucci et al., 2006; 2007). Both relocated clusters, however, are too far north to be attributed to either of these previously inferred structures. The fault map from Molli et al. (2021) shows another E-W trending fault ∼4 km north of the Onshore Cluster. Which could be, if extended offshore, related to the Offshore Cluster. Based on the currently mapped location of the fault, this fault is too far north to be related to the Onshore Cluster given the cluster’s steep fault plane. However, as none of the E-W striking faults display any morphology evidence at the surface its precise location might need to be reconsidered. Based on its location and orientation the Onshore Cluster can be reasonable associated to be the westwards continuation of the Northern Lucca Fault (Molli et al., 2021), bounding the Apuan Alps to the south-east.
The 3D geometry of the interpreted faults on which the two relocated clusters occurred are co-linear and sub-parallel. From this, we interpret the earthquake clusters likely occurred on the same fault or fault system, suggesting that the transversal structures identified onshore likely continue offshore. An onshore-offshore connection would support previous tentative interpretations that other major transverse faults in the region, such as the Livorno-Empoli lineament and the Guappero fault, are potentially also continuing offshore (Cantini et al., 2001; Pascucci et al., 2006; 2007).
The two clusters also show that the associated transverse structures do not display pure normal or strike-slip faulting but are mostly oblique slip. While the Offshore Cluster only shows a right-lateral component, the slip direction in the Onshore Cluster is not unique and changes with depth along the fault (Figures 3D, 4D). In the shallowest part the focal mechanisms of the Onshore Cluster show a vertical fault plane and with an increase in depth the dip angle decreases to 60–70° while the focal mechanisms become more oblique slip. The sense of obliquity switches below 9 km depth from right-lateral to left-lateral. This change occurs despite the orientation of the slip vector and T-axes not changing with depth, with the change in slip type fully consistent with the decrease in dip angle and anti-clockwise rotation of the fault strike with increasing depth.
A clear difference between the Onshore and Offshore clusters is the orientation of the T-Axis, which also differs from the regional trend (Figures 1B, 3D, 4D). In the region the general observed direction of horizontal minimum stress is NE-SW (Cenni et al., 2012; Viti, 2020). The Offshore Cluster shows a slight change from the general trend with a N-S oriented T-Axis. The bigger deviation from the general trend is visible in the Onshore Cluster, which shows a NW-SE direction, so a 90° change from the regional trend. Such variations in the stress field are not unusual and examples can be found worldwide (Tingay et al., 2006; Heidbach et al., 2007; 2010; Pierdominici and Heidbach, 2012). One example is the spatially variable stress direction in the North German Basin attributed to regional effects (e.g. an inherited suture zone, postglacial rebound, increase of lithospheric strength or crustal thickness, salt diapirs) (Tingay et al., 2006; Heidbach et al., 2007; Reiter, 2021). Such variations are due to the fact that the stress orientation is not just influenced by the plate boundary forces, but is also sensitive to second- and third-order factors (e.g. topography, density contrast active faults and open fractures) influencing the stress field on much a smaller scale (Bell, 1996; Tingay et al., 2006; Heidbach et al., 2007). Accordingly, one possible, local, cause, for the change in the Onshore Cluster is the proximity between the Apuan Alps and the Viareggio Basin and the concomitant material contrast leading to a contact parallel orientation of the maximum horizontal stress. Also, weak faults and open fractures, acting as a free surface, lead to reorientation of the maximum horizontal stress parallel to the fault plane (Bell, 1996; Tingay et al., 2006).
In extensional systems transverse faults often have their origin in inherited heterogeneities in the crust, and these faults commonly mark the connection between two step-over faults or basins oriented in line with the main fault trend (Illies, 1972; Morley, 1995; Zanchi et al., 2012). The lineaments inferred in the Northern Apennines might be related to inherited heterogeneities going as far back as the continental margin of Adria in the opening of the Ligurian-Piedmont ocean in the Jurassic or even further back in time to the Triassic (Liotta, 1991; Nirta et al., 2007) and could have experienced reactivation during the Oligocene thrusting as well as during the following extension (Sorgi et al., 1998). The Northern Lucca Fault and its now identified westwards continuation could be the connection between the master fault of the Viareggio Basin and the faults bounding the Lucca basin to the east. Assuming both clusters are indeed along one fault system would indicate that it is a larger structure, which may influence linkage between en-echelon basins such as the Viareggio Basin to the Lucca Basin in the east. The lateral step of about 20 km between these two basins is similar to what is suggested for other offset basins in the Northern Apennines supporting the interpretation that the basin segmentation can be attributed to the presence of transverse structures (Liotta, 1991; Sorgi et al., 1998; Pascucci et al., 2007). Indeed, further to the south, in the central Apennines, similar NE-SW transverse structures have been interpreted as transfer faults, which developed in a significantly stretched crust between the NW-SE oriented normal faults (Acocella and Funiciello, 2006).
Large scale transverse structures are often marking the transition between rift segments accommodating differential extension (Davis and Burchfiel, 1973; Laubscher, 1985; Martin et al., 1993; Corti et al., 2018). These differences are often expressed at the surface in large scale morphological changes. Indeed the faults associated with the two clusters are located at the transition between major topographic–geomorphic changes visible north and south of the Arno Plain. This difference in morphology is thought to be the surface expression of the differently developed extensional systems in the region (Carmignani and Kligfield, 1990; Pascucci et al., 2007; Rosenbaum and Piana Agostinetti, 2015). Generally the transition between the two extensional regimes is thought to be accommodate mainly by the Livorno-Empoli lineament (Nirta et al., 2007; Rosenbaum and Piana Agostinetti, 2015). Molli et al. (2021) suggests that some faults of the transverse E-W trending system might be reactivated by oblique slip along the dominant N-S trending fault system. This might also be the case for the Northern Lucca fault and its westward continuation. Furthermore, it is likely that this fault system might, due to its size and location at a major topographic step, additionally accommodate part of the movement generally attributed to the Livorno-Empoli lineament. The origin of the differential development of the extensional regime in the region is still under debate. Rosenbaum and Piana Agostinetti (2015) attributes the different extensional development to deep seated processes connected to along strike variation in slab rollback rates such as an increase in slab rollback velocity south of the Arno plain and possibly underplating north of it (Di Stefano et al., 2009; Chiarabba et al., 2014) leading to a possible tearing of the subducting Adratic slab. Another possible explanation or influence factor for the differential extension is the rotational, counter-clockwise, movement of the Apennines-Adriatic system (Le Breton et al., 2017) since the Oligocene leading to the bending of the mountain chain and possibly causing the Northern Apennines to move as separate, independent blocks with the transverse structures accommodating the movement between the blocks (Nirta et al., 2007; Cenni et al., 2012; Rosenbaum and Piana Agostinetti, 2015).
We analysed and relocated one onshore and one offshore earthquake cluster in the Viareggio Basin, Northern Tuscany, occurring between 2006 and 2021 – to improve the spatial and depth distribution of seismicity. The results for the Onshore Cluster showed clear spatial separation between three swarms at a depth of 2–15 km. The Offshore Cluster consisted of a single swarm 3–13 km deep. We also calculated 12 new focal mechanisms from P- and S-polarity recordings. All data showed a coherent picture of an active NE-SW striking, SE dipping transversal fault system with oblique normal-slip with a predominately right-lateral component, but with a left-lateral component for the deeper swarm (>9 km) of the Onshore Cluster. The clusters are co-linear and we interpret them to be part of a single fault system, likely the westwards continuation of the Northern Lucca fault. The system is dissecting the Viareggio Basin and connects it with the Lucca basin, possibly influencing the basin segmentation and development. The results show that the transversal structures of the Inner Northern Apennines, many of which lack clear surface morphologic expression, are seismogenic. The length, position and onshore-to-offshore nature of the fault systems suggests reactivation of pre-existing structures.
Publicly available datasets were analyzed in this study. This data can be found here: INGV earthquake catalogue (https://terremoti.ingv.it/), Italian Seismic Bulletin (BSI) (10.13127/BSI/201502; 10.13127/BSI/201602.01; 10.13127/BSI/202103; https://terremoti.ingv.it/bsi), Database European Integrated Data Archive EIDA (https://eida.ingv.it/it/).
LK: Writing–original draft, Writing–review and editing, Formal Analysis, Funding acquisition, Investigation, Visualization. CD: Writing–review and editing, Supervision. PV: Writing–review and editing, Funding acquisition, Supervision. GM: Writing–review and editing, Supervision. CP: Writing–review and editing, Resources, Software. DK: Writing–original draft, Writing–review and editing, Conceptualization, Methodology, Supervision.
The author(s) declare that financial support was received for the research, authorship, and/or publication of this article. This study was part of the PhD thesis of LK, at the University of Florence. LK was supported by a scholarship of the University of Florence, Department of Earth Sciences (dottorato XXXVII ciclo). DK was partly supported through NERC grant NE/L013932.
We thank the University of Pisa to allow the use of their server infrastructure. Part of the research was carried out during a research stay of LK at the School of Ocean and Earth Science of the University of Southampton, which is thanked for hosting. We used the publicly available data sets of the Italian National Institute of Geophysics and Volcanology (INGV). Figures and maps were created with the programmes Esri ArcGIS, Matlab & Inkscape. We thank the Domenico Di Giacomo and second reviewer for their constructive comments on the paper as well as the Associated Editor Ziyadin Cakir and Chief Editor Valerio Acocella for effectively handling the paper.
The authors declare that the research was conducted in the absence of any commercial or financial relationships that could be construed as a potential conflict of interest.
The author(s) declared that they were an editorial board member of Frontiers, at the time of submission. This had no impact on the peer review process and the final decision.
All claims expressed in this article are solely those of the authors and do not necessarily represent those of their affiliated organizations, or those of the publisher, the editors and the reviewers. Any product that may be evaluated in this article, or claim that may be made by its manufacturer, is not guaranteed or endorsed by the publisher.
The Supplementary Material for this article can be found online at: https://www.frontiersin.org/articles/10.3389/feart.2024.1474036/full#supplementary-material
Acocella, V., and Funiciello, R. (2006). Transverse systems along the extensional Tyrrhenian margin of central Italy and their influence on volcanism. Tectonics 25, 2005TC001845. doi:10.1029/2005TC001845
AlpArray Seismic Network (2015). AlpArray seismic network (AASN) temporary component. doi:10.12686/ALPARRAY/Z3_2015
Argnani, A. (2012). Plate motion and the evolution of alpine corsica and northern Apennines. Tectonophysics 579, 207–219. doi:10.1016/j.tecto.2012.06.010
Argnani, A., Bernini, M., Di Dio, G. M., Papani, G., and Rogledi, S. (1997). Stratigraphic record of crustal-scale tectonics in the quaternary of the Northern Apennines (Italy). Il Quat. Italian J. Quat. Sci. 10, 595–602.
Barchi, M. R. (2010). The Neogene-Quaternary evolution of the Northern Apennines: crustal structure, style of deformation and seismicity. J. Virt. Ex. 36. doi:10.3809/jvirtex.2010.00220
Bartole, R., Torelli, L., Mattei, G., Peis, D., and Brancolini, G. (1991). Assetto stratigrafico-strutturale del Tirreno settentrionale: stato dell’arte. Studi Geol. Camerti 1, 115–140.
Bell, J. S. (1996). Petro geoscience 2. in situ stresses in sedimentary rocks (part 2): applications of stress measurements. Geosci. Can. 23, 135–153.
Bellahsen, N., and Daniel, J. M. (2005). Fault reactivation control on normal fault growth: an experimental study. J. Struct. Geol. 27, 769–780. doi:10.1016/j.jsg.2004.12.003
Bennett, R. A., Serpelloni, E., Hreinsdóttir, S., Brandon, M. T., Buble, G., Basic, T., et al. (2012). Syn-convergent extension observed using the RETREAT GPS network, northern Apennines, Italy. J. Geophys. Res. 117, 2011JB008744. doi:10.1029/2011JB008744
Boccaletti, M., Elter, P., and Guazzone, G. (1971). Plate tectonic models for the development of the western Alps and northern Apennines. Nat. Phys. Sci. 234, 108–111. doi:10.1038/physci234108a0
Boccaletti, M., and Sani, F. (1998). Cover thrust reactivations related to internal basement involvement during Neogene-Quaternary evolution of the northern Apennines. Tectonics 17, 112–130. doi:10.1029/97TC02067
Bonini, M. (2009). Structural controls on a carbon dioxide-driven mud volcano field in the Northern Apennines (Pieve Santo Stefano, Italy): relations with pre-existing steep discontinuities and seismicity. J. Struct. Geol. 31, 44–54. doi:10.1016/j.jsg.2008.10.003
Bonini, M., Sani, F., Stucchi, E. M., Moratti, G., Benvenuti, M., Menanno, G., et al. (2014). Late Miocene shortening of the northern Apennines back-arc. J. Geodyn. 74, 1–31. doi:10.1016/j.jog.2013.11.002
Boyd, O. (2023). bb.m. Personal. Web pages A. T. CER Softw. Available at: http://www.ceri.memphis.edu/people/olboyd/Software/bb.m (Accessed June 28, 2024).
Brogi, A., Capezzuoli, E., Martini, I., Picozzi, M., and Sandrelli, F. (2014). Late quaternary tectonics in the inner northern Apennines (siena basin, southern Tuscany, Italy) and their seismotectonic implication. J. Geodyn. 76, 25–45. doi:10.1016/j.jog.2014.03.001
Cantini, P., Testa, G., Zanchetta, G., and Cavallini, R. (2001). The Plio–Pleistocene evolution of extensional tectonics in northern Tuscany, as constrained by new gravimetric data from the Montecarlo Basin (lower Arno Valley, Italy). Tectonophysics 330, 25–43. doi:10.1016/S0040-1951(00)00217-1
Carmignani, L., Conti, P., Cornamusini, G., and Meccheri, M. (2004). The internal Northern Apennines, the northern Tyrrhenian sea and the Sardinia-Corsica block. Geol. Italy. Special Volume, Italian Geol. Soc. IGC, 59–77.
Carmignani, L., and Kligfield, R. (1990). Crustal extension in the northern Apennines: the transition from compression to extension in the alpi apuane core complex. Tectonics 9, 1275–1303. doi:10.1029/TC009i006p01275
Cenni, N., Mantovani, E., Baldi, P., and Viti, M. (2012). Present kinematics of Central and Northern Italy from continuous GPS measurements. J. Geodyn. 58, 62–72. doi:10.1016/j.jog.2012.02.004
Chiarabba, C., and Frepoli, A. (1997). Minimum 1D velocity models in Central and Southern Italy: a contribution to better constrain hypocentral determinations. Available at: https://www.earth-prints.org/handle/2122/1597 (Accessed June 19, 2024).40. doi:10.4401/ag-3888
Chiarabba, C., Giacomuzzi, G., Bianchi, I., Agostinetti, N. P., and Park, J. (2014). From underplating to delamination-retreat in the northern Apennines. Earth Planet. Sci. Lett. 403, 108–116. doi:10.1016/j.epsl.2014.06.041
Chorowicz, J., Deffontaines, B., and Villemin, T. (1989). Interpretation of the NE-SW transverse structures in the Rhine graben in terms of transfer faults. Contribution of multisource data. C. R. Acad. Sci. Paris 309, 1067–1073.
Collettini, C., and Sibson, R. H. (2001). Normal faults, normal friction? Geology 29, 927–930. doi:10.1130/0091-7613(2001)029<0927:NFNF>2.0.CO;2
Conti, P., Cornamusini, G., and Carmignani, L. (2020). An outline of the geology of the Northern Apennines (Italy), with geological map at 1:250,000 scale. IJG 139, 149–194. doi:10.3301/IJG.2019.25
Corti, G., Maestrelli, D., and Sani, F. (2022). Large-to local-scale control of pre-existing structures on continental rifting: examples from the main Ethiopian rift, east africa. Front. Earth Sci. 10, 808503. doi:10.3389/feart.2022.808503
Corti, G., Sani, F., Agostini, S., Philippon, M., Sokoutis, D., and Willingshofer, E. (2018). Off-axis volcano-tectonic activity during continental rifting: insights from the transversal Goba-Bonga lineament, Main Ethiopian Rift (East Africa). Tectonophysics 728–729, 75–91. doi:10.1016/j.tecto.2018.02.011
Danecek, P., Pintore, S., Mazza, S., Mandiello, A., Fares, M., Carluccio, I., et al. (2021). The Italian node of the European integrated data archive. Seismol. Res. Lett. 92, 1726–1737. doi:10.1785/0220200409
Dannowski, A., Kopp, H., Grevemeyer, I., Lange, D., Thorwart, M., Bialas, J., et al. (2020). Seismic evidence for failed rifting in the ligurian basin, western alpine domain. Solid Earth 11, 873–887. doi:10.5194/se-11-873-2020
Davis, G. A., and Burchfiel, B. C. (1973). Garlock Fault: an intracontinental transform structure, southern California. Geol. Soc. Am. Bull. 84, 1407. doi:10.1130/0016-7606(1973)84<1407:GFAITS>2.0.CO;2
De Luca, G., Cattaneo, M., Monachesi, G., and Amato, A. (2009). Seismicity in Central and Northern Apennines integrating the Italian national and regional networks. Tectonophysics 476, 121–135. doi:10.1016/j.tecto.2008.11.032
Di Stefano, R., Bianchi, I., Ciaccio, M. G., Carrara, G., and Kissling, E. (2011). Three-dimensional Moho topography in Italy: new constraints from receiver functions and controlled source seismology. Geochem. Geophys. Geosyst. 12, n/a-n–a. doi:10.1029/2011GC003649
Di Stefano, R., and Ciaccio, M. G. (2014). The lithosphere and asthenosphere system in Italy as inferred from the Vp and vs 3D velocity model and Moho map. J. Geodyn. 82, 16–25. doi:10.1016/j.jog.2014.09.006
Di Stefano, R., and Ciaccio, M. G. (2020). Seismic velocity model of P- and S-waves for the Italian lithosphere. doi:10.13127/tomorama.1
Di Stefano, R., Kissling, E., Chiarabba, C., Amato, A., and Giardini, D. (2009). Shallow subduction beneath Italy: three-dimensional images of the Adriatic-European-Tyrrhenian lithosphere system based on high-quality P wave arrival times. J. Geophys. Res. 114, 2008JB005641. doi:10.1029/2008JB005641
Ferretti, G., Solarino, S., and Eva, E. (2002). Crustal structure of the Lunigiana-Garfagnana area (Tuscany, Italy): seismicity, fault-plane solutions, and seismic tomography. Boll. Geofis. Teor. Appl. 43, 221–238.
Frepoli, A., and Amato, A. (2000). Spatial variation in stresses in peninsular Italy and Sicily from background seismicity. Tectonophysics 317, 109–124. doi:10.1016/S0040-1951(99)00265-6
Gibbs, A. D. (1984). Structural evolution of extensional basin margins. JGS 141, 609–620. doi:10.1144/gsjgs.141.4.0609
Heidbach, O., Reinecker, J., Tingay, M., Müller, B., Sperner, B., Fuchs, K., et al. (2007). Plate boundary forces are not enough: second- and third-order stress patterns highlighted in the World Stress Map database. Tectonics 26, TC6014. doi:10.1029/2007TC002133
Heidbach, O., Tingay, M., Barth, A., Reinecker, J., Kurfeß, D., and Müller, B. (2010). Global crustal stress pattern based on the World Stress Map database release 2008. Tectonophysics 482, 3–15. doi:10.1016/j.tecto.2009.07.023
Illies, J. H. (1972). The Rhine graben rift system-plate tectonics and transform faulting. Geophys. Surv. 1, 27–60. doi:10.1007/BF01449550
ISIDe Working Group (2007). Italian seismological instrumental and parametric database (ISIDe). 366000 earthquakes, 500 stations. doi:10.13127/ISIDE
Istituto Nazionale di Geofisica e Vulcanologia (INGV) (2005). Rete Sismica Nazionale (RSN). approx. 27 GB per day of new waveform data, approx. 415 active seismic stations, the archive totals to more than 600 distinct seismic stations. doi:10.13127/SD/X0FXNH7QFY
Jolivet, L., Dubois, R., Fournier, M., Goffé, B., Michard, A., and Jourdan, C. (1990). Ductile extension in alpine Corsica. Geol 18, 1007. doi:10.1130/0091-7613(1990)018<1007:DEIAC>2.3.CO;2
Klein, F. W. (2002). User’s guide to HYPOINVERSE-2000, a Fortran program to solve for earthquake locations and magnitudes. U.S. Geological Survey Open-File Report 02-171. Available at: https://pubs.usgs.gov/of/2002/0171/.
Kligfield, R. (1979). The northern Apennines as a collisional orogen. Am. J. Sci. 279, 676–691. doi:10.2475/ajs.279.6.676
Latorre, D., Castellano, C., Melorio, C., Miconi, L., Pagliuca, N. M., Baccheschi, P., et al. (2022). Bollettino sismico italiano (BSI), III quadrimestre 2021 (Version 1). doi:10.13127/BSI/202103
Latorre, D., Di Stefano, R., Castello, B., Michele, M., and Chiaraluce, L. (2023). An updated view of the Italian seismicity from probabilistic location in 3D velocity models: the 1981–2018 Italian catalog of absolute earthquake locations (CLASS). Tectonophysics 846, 229664. doi:10.1016/j.tecto.2022.229664
Laubscher, H. P. (1985). Large-scale, thin-skinned thrusting in the southern Alps: kinematic models. Geol. Soc. Am. Bull. 96, 710. doi:10.1130/0016-7606(1985)96<710:LTTITS>2.0.CO;2
Le Breton, E., Handy, M. R., Molli, G., and Ustaszewski, K. (2017). Post-20 ma motion of the adriatic plate: new constraints from surrounding orogens and implications for crust-mantle decoupling. Tectonics 36, 3135–3154. doi:10.1002/2016TC004443
Li, H., Michelini, A., Zhu, L., Bernardi, F., and Spada, M. (2007). Crustal velocity structure in Italy from analysis of regional seismic waveforms. Bull. Seismol. Soc. Am. 97, 2024–2039. doi:10.1785/0120070071
Liotta, D. (1991). The arbia-val marecchia line, northern Apennines. Eclogae Geol. Helvetiae 84, 413–430.
Loreto, M. F., Zitellini, N., Ranero, C. R., Palmiotto, C., and Prada, M. (2021). Extensional tectonics during the Tyrrhenian back-arc basin formation and a new morpho-tectonic map. Basin Res. 33, 138–158. doi:10.1111/bre.12458
Maestrelli, D., Montanari, D., Corti, G., Del Ventisette, C., Moratti, G., and Bonini, M. (2020). Exploring the interactions between rift propagation and inherited crustal fabrics through experimental modeling. Tectonics 39, e2020TC006211. doi:10.1029/2020TC006211
Mandiello, A., Fares, M., Peter, D., Pintore, S., Emiliano, D. B., Franceschi, D., et al. (2023). EIDA Italian node: seismic data curation preservation e dissemination. doi:10.57757/IUGG23-3367
Margheriti, L., Mele, F. M., Marchetti, A., and Nardi, A. (2016). Bollettino sismico italiano (BSI), II quadrimestre 2015. doi:10.13127/BSI/201502
Mariucci, M. T., and Montone, P. (2020). Database of Italian present-day stress indicators, IPSI 1.4. Sci. Data 7, 298. doi:10.1038/s41597-020-00640-w
Mariucci, M. T., and Montone, P. (2022). IPSI 1.5, Italian present-day stress indicators dataset. Database Horiz. stress orientations crust. doi:10.13127/IPSI.1.5
Martin, M. W., Glazner, A. F., Walker, J. D., and Schermer, E. R. (1993). Evidence for right-lateral transfer faulting accommodating en echelon Miocene extension, Mojave Desert, California. Geol 21, 355. doi:10.1130/0091-7613(1993)021<0355:EFRLTF>2.3.CO;2
MedNet Project Partner Institutions (1990). “Mediterranean Very Broadband Seismographic Network (MedNet). approx. 1.1 GB/day of new data, approx. 25 active seismic broadband stations,” in 35 distinct seismic broadband stations. doi:10.13127/SD/FBBBTDTD6Q
Mele, G., and Sandvol, E. (2003). Deep crustal roots beneath the northern Apennines inferred from teleseismic receiver functions. Earth Planet. Sci. Lett. 211, 69–78. doi:10.1016/S0012-821X(03)00185-7
Molli, G. (2008). Northern Apennine–Corsica orogenic system: an updated overview. SP 298, 413–442. doi:10.1144/SP298.19
Molli, G., Manighetti, I., Bennett, R., Malavieille, J., Serpelloni, E., Storti, F., et al. (2021). Active Fault systems in the inner northwest Apennines, Italy: a reappraisal one century after the 1920 Mw ∼6.5 fivizzano earthquake. Geosciences 11, 139. doi:10.3390/geosciences11030139
Molli, G., Torelli, L., and Storti, F. (2016). The 2013 Lunigiana (Central Italy) earthquake: seismic source analysis from DInSar and seismological data, and geodynamic implications for the northern Apennines. A discussion. Tectonophysics 668–669, 108–112. doi:10.1016/j.tecto.2015.07.041
Molnar, N. E., Cruden, A. R., and Betts, P. G. (2019). Interactions between propagating rifts and linear weaknesses in the lower crust. Geosphere 15, 1617–1640. doi:10.1130/GES02119.1
Montone, P., and Mariucci, M. T. (2016). The new release of the Italian contemporary stress map. Geophys. J. Int. 205, 1525–1531. doi:10.1093/gji/ggw100
Montone, P., Mariucci, M. T., Pondrelli, S., and Amato, A. (2004). An improved stress map for Italy and surrounding regions (central Mediterranean). J. Geophys. Res. 109, 2003JB002703. doi:10.1029/2003JB002703
Morley, C. K. (1995). Developments in the structural geology of rifts over the last decade and their impact on hydrocarbon exploration. SP 80, 1–32. doi:10.1144/GSL.SP.1995.080.01.01
Muirhead, J. D., and Kattenhorn, S. A. (2018). Activation of preexisting transverse structures in an evolving magmatic rift in East Africa. J. Struct. Geol. 106, 1–18. doi:10.1016/j.jsg.2017.11.004
Nardi, A., Latorre, D., Margheriti, L., Mele, F. M., Marchetti, A., Berardi, M., et al. (2020). Bollettino sismico italiano (BSI), II quadrimestre 2016 (versione 2). 10542 eqs, 5501 eqs_ge_1.5, 76 eqs_ge_3.5, 441 stations, 82932 picks_p, 55466 picks_s. doi:10.13127/BSI/201602.01
Nirta, G., Principi, G., and Vannucchi, P. (2007). The Ligurian Units of Western Tuscany (Northern Apennines): insight on the influence of pre-existing weakness zones during ocean closure. Geodin. Acta 20, 71–97. doi:10.3166/ga.20.71-97
Osagiede, E. E., Rosenau, M., Rotevatn, A., Gawthorpe, R., Jackson, C. A., and Rudolf, M. (2021). Influence of zones of pre-existing crustal weakness on strain localization and partitioning during rifting: insights from analog modeling using high-resolution 3D digital image correlation. Tectonics 40, e2021TC006970. doi:10.1029/2021TC006970
Pascucci, M., Merlini, S., and Martini,, I. P. (1999). Seismic stratigraphy of the miocene–pleistocene sedimentary basins of the Northern Tyrrhenian Sea and western Tuscany (Italy). Basin Res. 11, 337–356. doi:10.1046/j.1365-2117.1999.00104.x
Pascucci, V., Costantini, A., Martini, I. P., and Dringoli, R. (2006). Tectono-sedimentary analysis of a complex, extensional, Neogene basin formed on thrust-faulted, Northern Apennines hinterland: radicofani Basin, Italy. Sediment. Geol. 183, 71–97. doi:10.1016/j.sedgeo.2005.09.009
Pascucci, V., Martini, I. P., Sagri, M., and Sandrelli, F. (2007). “Effects of transverse structural lineaments on the neogene–quaternary basins of Tuscany (inner northern Apennines, Italy),” in Sedimentary processes, environments and basins. Editors G. Nichols, E. Williams, and C. Paola (Wiley), 155–182. doi:10.1002/9781444304411.ch8
Piana Agostinetti, N., and Amato, A. (2009). Moho depth and V p/V s ratio in peninsular Italy from teleseismic receiver functions. J. Geophys. Res. 114, 2008JB005899. doi:10.1029/2008JB005899
Pierdominici, S., and Heidbach, O. (2012). Stress field of Italy — mean stress orientation at different depths and wave-length of the stress pattern. Tectonophysics 532–535, 301–311. doi:10.1016/j.tecto.2012.02.018
Raggiunti, M., Keir, D., Pagli, C., and Lavayssière, A. (2023). Evidence of fluid induced earthquake swarms from high resolution earthquake relocation in the main Ethiopian rift. Geochem Geophys Geosyst 24, e2022GC010765. doi:10.1029/2022GC010765
Reiter, K. (2021). Stress rotation – impact and interaction of rock stiffness and faults. Solid Earth 12, 1287–1307. doi:10.5194/se-12-1287-2021
Rosenbaum, G., and Piana Agostinetti, N. (2015). Crustal and upper mantle responses to lithospheric segmentation in the northern Apennines: response to lithospheric segmentation. Tectonics 34, 648–661. doi:10.1002/2013TC003498
Saccorotti, G., Bruni, R., Bonini, M., Corti, G., Keir, D., and Sani, F. (2022). Recent seismic sequences and activation of normal fault systems in the mugello Basin and surrounding areas (northern Apennines, Italy). Front. Earth Sci. 10, 879160. doi:10.3389/feart.2022.879160
Scognamiglio, L., Tinti, E., and Quintiliani, M. (2006). Time domain moment tensor (TDMT). doi:10.13127/TDMT
Scudero, S., Marcocci, C., and D’Alessandro, A. (2021). Insights on the Italian seismic network from location uncertainties. J. Seismol. 25, 1061–1076. doi:10.1007/s10950-021-10011-6
Serpelloni, E., Cavaliere, A., Martelli, L., Pintori, F., Anderlini, L., Borghi, A., et al. (2022). Surface velocities and strain-rates in the euro-mediterranean region from massive GPS data processing. Front. Earth Sci. 10, 907897. doi:10.3389/feart.2022.907897
Snoke, J. A. (2003). “85.12 - FOCMEC: FOCal MEChanism determinations,” in International handbook of earthquake and engineering seismology, Part B. Editors W. H. K. Lee, H. Kanamori, P. C. Jennings, and C. Kisslinger (Academic Press), 1629–1630. doi:10.1016/S0074-6142(03)80291-7
Sorgi, C., Deffontaines, B., Hippolyte, J. C., and Cadet, J. P. (1998). An integrated analysis of transverse structures in the northern Apennines, Italy. Geomorphology 25, 193–206. doi:10.1016/S0169-555X(98)00041-5
Tan, O. (2024). Long-term aftershock properties of the catastrophic 6 february 2023 kahramanmaraş (Türkiye) earthquake sequence. Acta geophys., doi:10.1007/s11600-024-01419-y
Tan, O., Pabuçcu, Z., Tapırdamaz, M. C., İnan, S., Ergintav, S., Eyidoğan, H., et al. (2011). Aftershock study and seismotectonic implications of the 8 March 2010 Kovancılar (Elazığ, Turkey) earthquake (M W = 6.1). Geophys. Res. Lett. 38, n/a. doi:10.1029/2011GL047702
Tingay, M., Muller, B., Reinecker, J., and Heidbach, O. (2006). “State and origin of the present-day stress field in sedimentary basins: new results from the world stress map project,” in 41st US rock mechanics symposium (Golden Rocks 2006): 50 years of rock mechanics-landmarks and future challenges. Editors D. P. Yale (Golden, Colorado, United States: Curran Associates).
Vignaroli, G., Faccenna, C., Jolivet, L., Piromallo, C., and Rossetti, F. (2008). Subduction polarity reversal at the junction between the western Alps and the northern Apennines, Italy. Tectonophysics 450, 34–50. doi:10.1016/j.tecto.2007.12.012
Viola, G., Mancktelow, N. S., and Seward, D. (2001). Late oligocene-neogene evolution of europe-adria collision: new structural and geochronological evidence from the giudicarie fault system (Italian eastern Alps). Tectonics 20, 999–1020. doi:10.1029/2001TC900021
Viti, M. (2020). Active tectonics in Tuscany (Central Italy): ten years of seismicity (2009-2019). IJG 11, 613–650. doi:10.4236/ijg.2020.1110032
Waldhauser, F. (2001). hypoDD—A proogram to compute double-difference hypocenter locations., Version 1.0. USGS Earthq. Sci. Center Open-File Report 2001-113. doi:10.3133/ofr01113
Waldhauser, F., and Ellsworth, W. L. (2000). A double-difference earthquake location algorithm: method and application to the northern hayward fault, California. Bull. Seismol. Soc. Am. 90, 1353–1368. doi:10.1785/0120000006
Zanchi, A., D’Adda, P., Zanchetta, S., and Berra, F. (2012). Syn-thrust deformation across a transverse zone: the Grem–Vedra fault system (central Southern Alps, N Italy). Swiss J. Geosci. 105, 19–38. doi:10.1007/s00015-011-0089-6
Keywords: seismicity and tectonics, transversal structures, earthquakes, Apennines, Italy
Citation: Kaerger L, Del Ventisette C, Vannucchi P, Molli G, Pagli C and Keir D (2024) Relocation of earthquake clusters show seismogenic transverse structures in the Inner Northern Apennines (Italy). Front. Earth Sci. 12:1474036. doi: 10.3389/feart.2024.1474036
Received: 31 July 2024; Accepted: 24 September 2024;
Published: 14 October 2024.
Edited by:
Ziyadin Cakir, Istanbul Technical University, TürkiyeReviewed by:
Domenico Di Giacomo, International Seismological Centre (ISC), United KingdomCopyright © 2024 Kaerger, Del Ventisette, Vannucchi, Molli, Pagli and Keir. This is an open-access article distributed under the terms of the Creative Commons Attribution License (CC BY). The use, distribution or reproduction in other forums is permitted, provided the original author(s) and the copyright owner(s) are credited and that the original publication in this journal is cited, in accordance with accepted academic practice. No use, distribution or reproduction is permitted which does not comply with these terms.
*Correspondence: Lauretta Kaerger, bGF1cmV0dGEua2FlcmdlckB1bmlmaS5pdA==
Disclaimer: All claims expressed in this article are solely those of the authors and do not necessarily represent those of their affiliated organizations, or those of the publisher, the editors and the reviewers. Any product that may be evaluated in this article or claim that may be made by its manufacturer is not guaranteed or endorsed by the publisher.
Research integrity at Frontiers
Learn more about the work of our research integrity team to safeguard the quality of each article we publish.