- 1Yunnan Geological Engineering Second Survey Institute Co., Ltd., Kunming, China
- 2Chinese Academy of Geological Sciences, Beijing, China
- 3China Institute of Geo-Environment Monitoring, Beijing, China
Debris flow is a common geological disaster in mountainous areas, which occurs frequently in the rainy season and has strong mobility and destructiveness, often bringing serious loss of life and property. Previous studies have focused more on single outbreaks of debris flow disasters, and less on multiple outbreaks of debris flow disasters in the same gully during the rainy season, which makes it more difficult to carry out prevention and control work for similar disasters. Because the occurrence of the disaster has its corresponding geomechanical model as the basis, which provides the necessary scientific basis for the prevention and control of the disaster. Through data collection, field investigation, drone aerial photography, modelling and rainfall data analysis, the geological environment of Yizhong River Basin and the frequent occurrence mechanism of debris flow disasters were investigated, and a conjugate shear-type disaster-inducing geomechanical model was proposed. With the help of RAMMS numerical simulation software, the dynamic mechanism of the disaster threatening the life and property safety of the residents in Deqin County was analysed to provide the necessary scientific basis for the prevention and control of the Yizhong River debris flow disaster. Base on the characteristics of the geological environment in alpine and gorge areas, the necessity and advantage of applying the nap-of-the-object photogrammetry technology to engineering geological survey in alpine and gorge areas are put forward.
Introduction
Debris flow disaster is one of the common geological disasters in mountainous areas, which is hidden and sudden, often bringing serious casualties and property losses (Hu et al., 2012; Yin et al., 2016). In the past, the research on debris flow disasters has paid more attention to the mechanism and dynamics of the single occurrence of debris flow disasters, and has obtained abundant research results (Iverson et al., 2011; Yin et al., 2016; Pudasaini and Fischer, 2020), but there are fewer studies on the mechanism of multiple outbreaks of debris flow disasters in the same debris flow gully during the rainy season, which makes it more difficult to carry out the prevention and control work of similar disasters. Because the disaster occurs on the basis of its corresponding geomechanical model of pregnant disaster, and different geomechanical model of pregnant disaster will determine the characteristics of the disaster and the internal mechanism (Yin et al., 2023), which provides the necessary scientific basis for the prevention and control of the disaster.
With the development of information technology, several numerical simulation packages have been used in order to better understand and predict the mobility of such geological hazards, including: dynamic analysis of landslides/dynamic analysis of landslide motion in three dimensions (DAN-W/DAN3D), landslide simulation (LS)-RAPID, smoothed particle hydrodynamics (SPH), and others (Hungr, 1995; Davies and McSaveney, 1999; Hungr and Evans, 2004; Hungr, 2008; Sassa et al., 2010; Xu et al., 2010; Zhang et al., 2010; Xing et al., 2015). The Rapid Mass Motion Simulation (RAMMS) software can simulate the motion evolution of debris flows and mudslides, and the trajectory of rockfalls and avalanches under complex terrain conditions, and accurately predict the jumping distance, flow velocity, and impact force of the objects’ motions, which is widely used for analysing the dynamics of the processes of geohazards such as debris flows, avalanches, and rockfalls in alpine areas (Christen et al., 2010a; b; Aydin et al., 2014) to carry out disaster dynamics mechanisms or disaster risk studies, among others. The software uses the Voellmy-Salm (VS) model to simulate the disaster dynamics process, which has been widely used in recent disaster case studies (Mahboob et al., 2015; Gan and Zhang, 2019; Singh et al., 2020; Zhang et al., 2022; Zhuang et al., 2023). RAMMS has an advanced three-dimensional (3D) visualisation interface that allows the results to be combined with digital elevation models, aerial imagery and topographic maps.
In this study, the geological environment, geomechanical model, frequency mechanism, dynamical mechanism and development trend of the Yizhong River debris flow disaster were analysed through data collection, field investigation, borehole survey, unmanned aerial vehicle (UAV) aerial photography and modelling, rainfall data analysis and RAMMS simulation. A conjugate shear-type geomechanical model is proposed for analysing the frequent occurrence mechanism of the disaster. According to the RAMMS simulation results, the debris flow power decreases rapidly when it reaches the G214 national highway, so the threat of the disaster mainly lies in the blockage of the confluence of the Yizhong River and the Zhiqu River, as well as the damage to the surrounding infrastructures after the overflow. In order to make up for the shortage of manual survey, it is proposed that the technology of the nap-of-the-object photogrammetry should be applied to the engineering geological survey in alpine and gorge areas.
Geological environment
Geological tectonic background
Deqin County is located at the top of the lower arc in the middle section of the Hengduan Mountains in the southeastern Qinghai-Tibet Plateau (Figure 1). The tectonic area belongs to the middle section of the Changdu-Lanping-Simao fold system, sandwiched between the Gangdise-Nyainqentanglha fold system and the Songpan-Ganzi fold system, near the contact zone between the Deqin fault block and the Xueyunshan fault block. In general, the fault block uplift is the main movement, and the fault structure occupies a controlling position. The tectonic line is nearly north-south, and the secondary tectonic is nearly east-west, forming a lattice structure (Figure 2A).
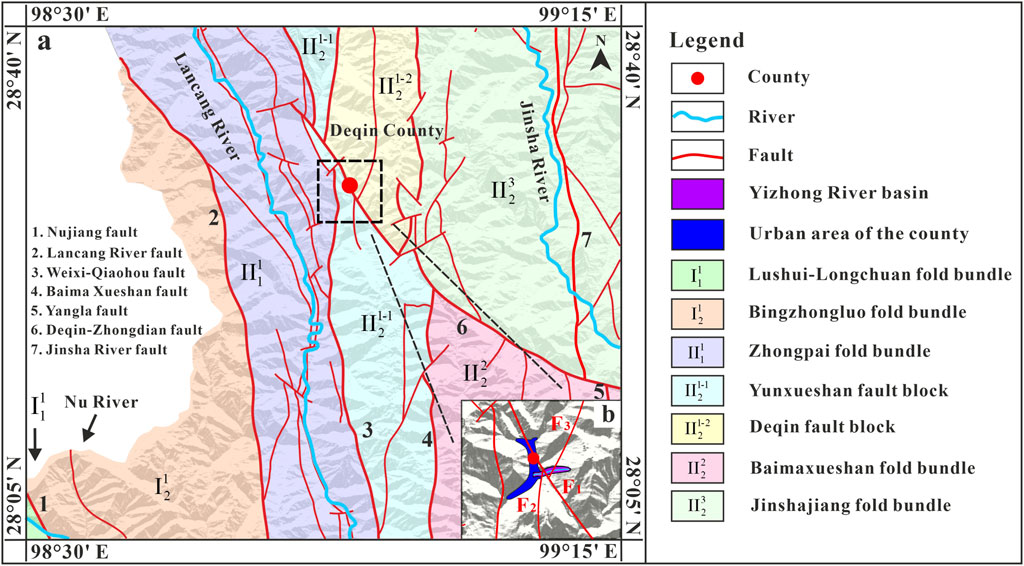
Figure 2. Tectonic map. (A) regional geological tectonic background of Deqin County; (B) geological tectonic background of Deqin County.
The Yizhonghe-Buyanlazha Niuchang fault (F3), the Deqin-Zhongdian fault zone (F1), and the Deqin-Wunongding fault (F2) passes through the upper, middle and lower reaches of the Yizhong River respectively (Figure 2B). Among them, the Deqin-Zhongdian fault zone has experienced many moderate earthquakes of magnitude 5.9 in 2013 (Yang et al., 2015; Wu et al., 2019). Affected by fault zones and faults in the Yizhong River Basin, structural joints are developed in the area, with a fracture rate of 1.7%∼15%. The strike is mainly NNE, and the dip is mainly SSE, followed by NNW. The dip angle is generally steep, mostly at 50°∼80°. The joints are generally opened by 1∼5 mm, and there is no filling, and the rock mass is cut into rectangular or diamond-shaped blocks. The complex and fragile geological conditions make disasters prone and frequent (Yin, 2009; Yin et al., 2017; He et al., 2023), forming the unique disaster-bearing environment of Yizhong River.
Stratigraphic lithology
The exposed strata in the study area includes the Mesozoic Middle Triassic Shanglan Formation (T2s) and the Upper Triassic Pantiange Formation (T2p) strata, and glacial layers (Qpgl) are common along the slope area on the right side of the channel. There are sporadic residual slope accumulations in the gentle slopes of the ridges on both sides of the bank. There are debris flow accumulations (Qhsef) near the intersection of Yizhong River and Zhiqu River. The specific conditions and descriptions are shown in Figure 3.
Terrain
The debris flow of the Yizhong River is in the source area at an altitude of 3,900 m∼4,250 m, with a treacherous terrain and an average longitudinal slope drop of 914‰ and a local upright shape. At an altitude of 3,300 m∼3,900 m, it is the circulation area, where there is a large amount of loose accumulation on both sides of the channel to supplement the material source when the debris flow occurs, with an average longitudinal slope drop of 578‰. Elevation 3,030 m∼3,300 m is the accumulation area, with an average longitudinal slope drop of 293‰ (Figure 4).
Due to the complexity of the debris flow gullies in the source area and the difficulty of carrying out surveys by conventional methods, surveys were carried out using rope bridge techniques (Figure 5A). The survey results show that the bedrock is controlled by two groups of joint planes and stratification planes, the plane occurrence is 99°∠55°, and the two groups of joints are 231°∠54° and 324°∠75°, respectively (Figures 5B, C). On average, there are 8-9 joint planes per meter (Figure 5D). According to Eq 1 and Table 1, it can be judged that the integrity of the rock mass is broken. The reason why the rock mass is in a stable state is that the bedding plane of the bedrock in the source area is in a counter-tilt state, and the lower bedrock supports the upper broken rock mass. However, due to the severe weathering of the surface layer, blocks collapse and accumulate frequently in the valley, blocking the valley (Figure 5E), forming the source of some of the coarse particles of debris flow in the rainy season (Chen et al., 2009; Cui et al., 2011; Hu et al., 2014). The northern bedrock surface is covered by a dense moraine deposit, with average thickness of 7.5 m and an area of 4×104 m2, The potential source of debris flow is 3×105 m3 (Figures 6A, B). During the retrogressive erosion of the northern gully, a large number of moraines constantly collapse or slide into the gully under the action of rainfall (Takahashi, 1981; Iverson et al., 1997; Zhou et al., 2013; Ye et al., 2024), forming rainfall landslides (Qiu et al., 2024), which are further converted into debris flows during movement, further contributing to the formation and expansion of debris flow gullies (Figure 6C).
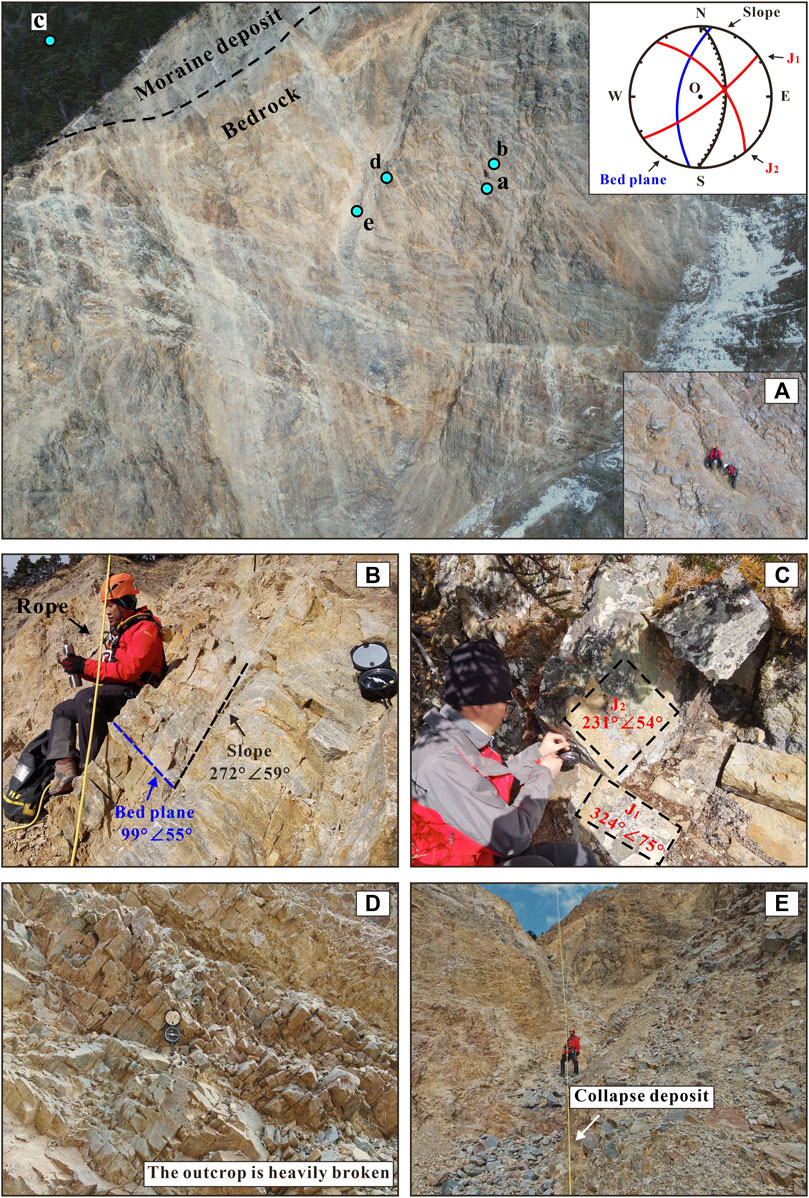
Figure 5. Survey of exposed bedrock in the source area. (A) Schematic diagram of rope bridge conveyance survey; (B) bedrock and slope attitude; (C) joint attitude; (D) exposed fractured rock mass; (E) slope failure material accumulation in gully.
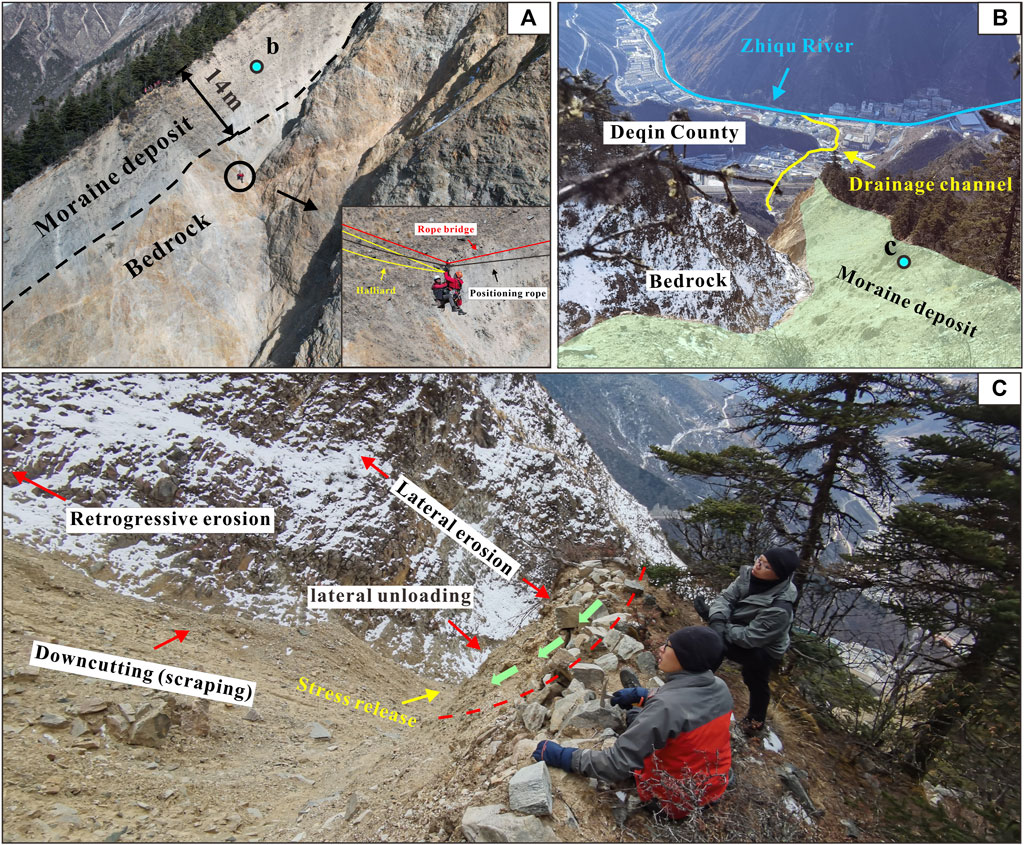
Figure 6. Moraine deposits in the northern part of the source area. (A) Schematic diagram of the location of moraine deposits in the northern part of the source area; (B) Schematic diagram of moraine deposits and their hazard to urban areas; (C) Rainfall-induced destabilisation of moraine deposits.
Where Jv is the number of rock mass volume joints (stripes/m3); Sn is the number of joints in the nth group per meter long survey line; Sk is the number of ungrouped joints per cubic meter of rock mass.
The trench in the circulation area is “V” and deep “V” shaped, with a narrow and straight bed, steep valley slopes, 30°–70° slopes on both sides, locally nearly upright, and mostly exposed rock faces. The width of the bottom of the ditch is 1.0–2.5 m, and the slump and gullies is developed. Because of the large longitudinal slope, the debris flow has a strong downward erosion ability, and the groove bed is undercut obviously. The continuous downward erosion reduces the stability of the slopes on both banks, and promotes the slopes on both banks to slide into the channel, forming a landslide L1 with a volume of 3.21 x 105 m3 (Zhang et al., 2024a; Zhang et al., 2024b) and an unstable slope Us1 with a volume of 3.5 x 104 m3 (Figure 7), forming a typical debris flow-landslide disaster chain (Cui and Guo, 2021; Zhao et al., 2021). Due to the previous geological action, moraine deposits were deposited along the channel with a thickness of 5–20 m. Whenever a debris flow breaks out in the upper part, after the high potential energy is converted into kinetic energy, the loose accumulation mass accumulated on both sides of the gully in the circulation area is scraped, which promotes the debris flow to be constantly supplemented by the source material along the way, forming a volume amplification effect (Berti et al., 1999; Wang et al., 2003; Godt and Coe, 2007; Li et al., 2019).
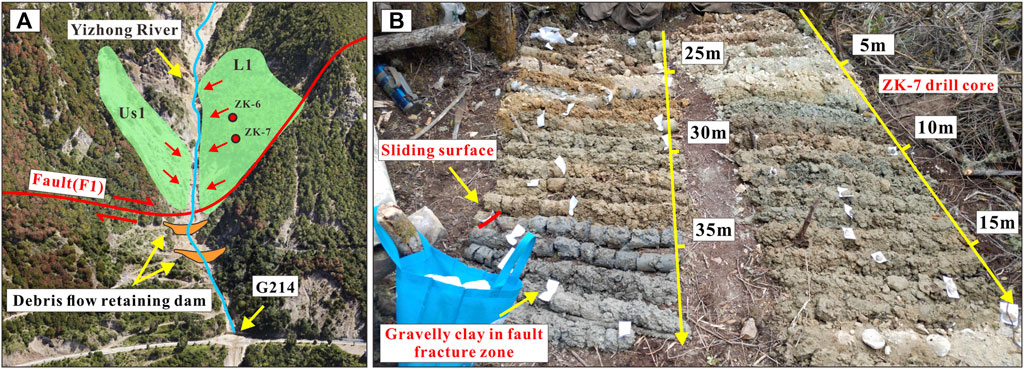
Figure 7. Secondary landslide hazards induced by debris flow. (A) Landslide distribution location; (B) ZK-7 drill core.
Constrained by the terrain, the accumulation area is shaped like a long tongue. Due to the construction of the drainage channel, the debris flow directly flows into the Zhiqu River from below the national highway G214 along the drainage channel (Figure 6B), but the mouth of the ditch at the intersection with the Zhiqu River is relatively gentle, which result in the debris flow easily clogging the ditch mouth and posing a threat to the urban area.
Geomechanical model
The occurrence of disasters has a corresponding disaster-generating process, which reflects the corresponding geomechanical model and determines the type, instability mechanism, and motion characteristics of the disaster (Yin et al., 2023). For example, the Zengziyan debris flow landslide that occurred in 2004 was a typical tower-shaped landslide. Under the action of gravity, the lower rock mass fractured and buckled, causing the overall collapse and fragmentation of the landslide mass into a debris flow slide (Feng et al., 2014). The Jiwei mountain landslide occurred in 2009, the sliding body sliding by the key block blocking, after the key blocking block by the slide thrust damage, the slide body destabilised to form a high level of landslide and fall collision disintegration, and ultimately the formation of debris flow disasters (Gao et al., 2018). The 2011 landslide at the Areletuobie landfill, the source of which had a decreasing factor of safety due to the long-term infiltration of rainfall, ultimately induced a landslide-type debris flow disaster (Wang et al., 2019). The Xinmo landslide, which occurred in 2017, is in a strong seismic zone and is a seismically fractured mountain, and through the comparison of multi-period satellite images, it was found that cracks were continuously generated and gradually expanded before the landslide destabilised (Yin et al., 2017). After reaching the critical state, the local destabilised blocks were the first to be dislodged, and the subsequent impacts generated a chain reaction, leading to the initiation of the landslide with a volume of about 1.8×107 m3, and ultimately the formation of a high-level avalanche debris flow disaster (Hu et al., 2018). The high level and long run-out geological hazard at Chamoli, India, in 2021, in which a wedge-shaped body became unstable and collapsed after crack development reached a critical value, and broke up and disintegrated to form a debris flow, which ultimately converted into a debris flow hazard (Zhang et al., 2022). Each disaster has its own geomechanical model, which is the basis for identifying the causes of the disaster and providing scientific prevention and treatment programmes.
After data collection and analysis and site investigation, it was confirmed that conjugate shear was formed in the source area of the Yizhong River under the action of internal reverse fault tectonic movement. The bedrock is structurally fragmented under conjugate shearing (Young et al., 2000; Payne et al., 2004; Yin and Taylor, 2011), forming a fractured shear zone (Figure 8A). Under the erosive action of external rainfall, the rock and soil bodies in the fracture zone are continuously eroded and form debris flow hazards (Figure 8B), resulting in the continuous shaping and further development of debris flow channels (Figure 8C).
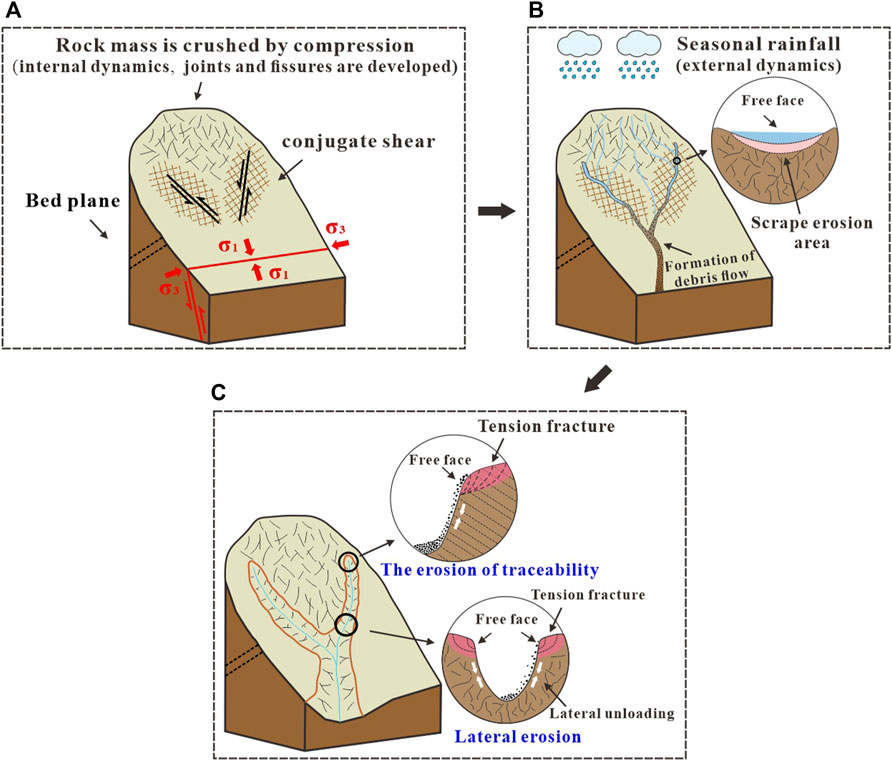
Figure 8. Schematic diagram of the conjugate shear geomechanical model. (A) Conjugate shear formation by tectonic action; (B) Rainfall contributes to debris flow hazards; (C) Formation and further development of “Y”-shaped debris flow channels.
Under the coupling of internal and external dynamics, the geomorphology of the Yizhong River Basin is prompted to evolve, which is accompanied by the frequent occurrence of debris flow disasters. Although the Yizhong River basin is rich in the amount of loose material sources, the area of the basin above national highway G214 is only 0.52 km2, so the limited amount of single rainfall catchment and the corresponding limited amount of material source initiation are important factors limiting the scale of single outbreaks of debris flow disasters.
Debris flow in 2021
Rainfall is one of the important factors for inducing debris flow disasters, such as the Zhouqu debris flow in Gansu Province on 7 August 2010 (Tang et al., 2011; Zhang and Matsushima, 2016), and the debris flow in Sanxi Village in Dujiangyan on 10 July 2013 (Yin et al., 2016; Gao et al., 2017; Guo et al., 2022). After entering the rainy season in 2021, under the action of rainfall, multiple debris flows erupted in the Yizhong River (Table 2). Although the scale of each outbreak was different, the debris flows slowed down rapidly as they moved up the national highway G214, the damage caused to the national highway G214 degree of damage is low, so that the threat posed by the disaster is mainly concentrated in Yizhong River and the confluence of the Zhiqu River. Therefore, Yizhong River debris flow in the upper part of the national highway G214 movement of the dynamics of the mechanism of research, to reveal the movement mechanism of the disaster and the risk assessment of the disaster is of great significance.
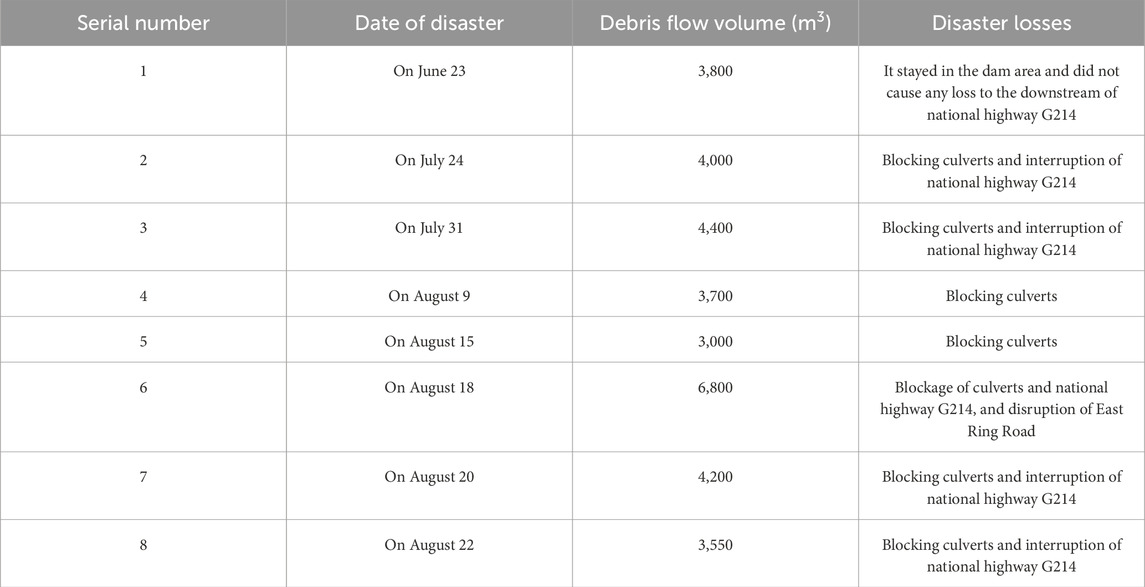
Table 2. Statistics of the occurrence of disasters in the rainy season of Yizhong River in Deqin County in 2021.
After the debris flow starts in the source area, it erodes the loose deposits along the way, and then flows along the valley to the national highway G214. Based on the video information recorded on site, it was estimated that the velocity of debris flow out of the gully mouth was about 4.5 m/s, and it decelerates rapidly to less than 1 m/s on national highway G214, forming a fan-like accumulation and blocking national highway G214, posing a threat to passing vehicles and pedestrians.
The debris flow then flows into the lower drainage channel from the national highway G214 (Figure 9A). As there is a corner area of the drainage channel under the G214 national highway that changes sharply from 34.2° to 20.9°, the eroding force of the debris flow on the corner of the drainage channel is strengthened, resulting in serious damage to the bottom of the drainage channel and the concrete floor. The soil at the bottom of the drainage channel is further eroded, and the erosion continues to trace the source, which seriously threatens the stability of the foundation of national highway G214 and the safety of passing vehicles and pedestrians (Figure 9B). Under the scouring action of multiple debris flows, a part of the right bank side wall in the middle of the drainage channel collapsed, causing the debris flow to overflow, posing a major threat to road traffic and residential areas below the drainage channel (Figure 9C). At the intersection of Yizhong River and Zhiqu River, due to the slow slope, the debris flow in the early stage is accumulated in the culvert under Kawagebo Avenue, blocking the circulation channel, causing the debris flow in the later stage to continuously deposit in the culvert, causing major safety hazards to the pavement pedestrians, residents and administrative areas (Figure 9D). The debris flow scale of a single eruption in 2019 is larger than that in 2021, with volume of approximately 1×104 m3. The debris flow blocked the culvert and overflowed the channel, which led to the burial of Kawagebo Avenue, and the debris flow flowed down Kawagebo for about 2 km. Due to the diversion effect of Kawagebo Avenue, the debris flow did not cause damage or casualties to the buildings in the lower reaches of the Deqin County No. One Middle School and the county government, but only damaged the road facilities and some houses along the road (Figures 9E, F). It can be seen that when the eruption volume of Yizhong River debris flow increases to more than 1×104 m3, it will form a huge safety hazard to the county and urban areas. Especially after the Zhiqu River is blocked, the secondary dammed lake will burst into flood disaster (Gallino and Pierson, 1984; Wei et al., 2018; Zhang et al., 2022), making half of the county in the flooded area. The complex engineering geological conditions, climate environment, human economic activities and engineering construction of Deqin County have caused serious geological hazards in the urban area of the county, so scientific management of the hazards is a matter of urgency.
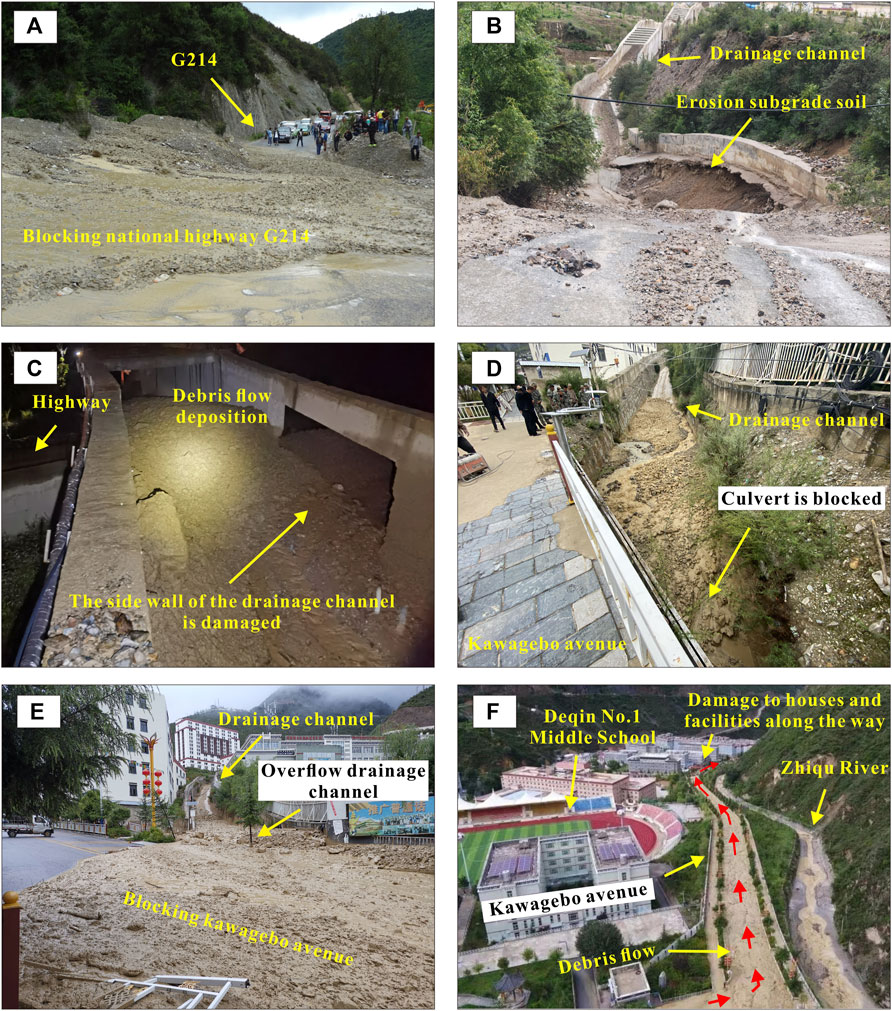
Figure 9. Debris flow outbreak situation. (A) blockage of national highway G214; (B) erosion of the drainage channel; (C) damage to the side walls of the drainage channel causing debris flow spillage; (D) blockage of the culvert; (E) Debris flow overflowing the culvert; (F) Debris flowing down the Kawagebo avenue.
Numerical simulation by RAMMS
RAMMS is a professional dynamic numerical simulation analysis software for debris avalanches designed by the swiss federal institute for snow and avalanche research (SLF) of the swiss federal institute for forest, snow and landscape research (WSL) (Christen et al., 2010a; Christen et al., 2010b). RAMMS: DEBRIS FLOW module is used for numerical calculations in this study. The RAMMS debris flow model is based on 2D depth-averaged shallow-water equations for granular flows. The calculation process mainly includes three steps: basic data input, model parameter setting, and the output of results. The basic data includes the terrain, the release zone, and physical and mechanical parameters of the material mass. The RAMMS runout model parameters mainly include the friction coefficient and the turbulence coefficient.
Numerical model
RAMMS uses VS model to simulate the dynamic process of the debris avalanche or debris flow. RAMMS modified the Voellmy–Salm (VS) model by considering the yield stress, which is more suitable for ideal plastic material:
where S is the friction resistance (Pa), ρ is the density (kg/m3), g is the gravitational acceleration, μ is the friction coefficient, v is the flow velocity (m/s), ξ is the turbulence coefficient (m/s2), N is the normal stress (Pa), and N0 is the yield stress (Pa). The parameter μ dominates the moment when the flow begins to stop, and ξ dominates the moment when the flow is running quickly.
Recommended parameters
Four parameters need to be input in RAMMS: (i) the digital terrain model, (ii) release area, (iii) material data, and (iv) friction coefficients. The digital terrain model in RAMMS was generated by the nap-of-the-object photogrammetry technology, the grid resolution in this model was 2 m (Figure 10). The release areas can be directly delineated in RAMMS according to the results of field investigations or satellite image interpretation. The debris flow density was 2000 kg/m3 based on sampling of debris flow deposits. The calibration of the RAMMS model has been carried out through a back-analysis procedure that mainly is committed to the two friction coefficients (μ and ξ), which affect the simulation results. In this study, μ is 0.2 and ξ is 200 m/s2.
Analysis of the simulation results
It is difficult to establish a model considering the artificially built drainage groove under national highway G214 and underground pipelines in many places. Moreover, the movement process of debris flow on the upper part of national highway G214 is the focus of the dynamic analysis. Therefore, in this paper, a high-precision 3D model of the upper area of national highway G214 (including national highway G214) was established by using the UAV nap-of-the-object photogrammetry technology (Figure 10), which was used as the terrain data input by RAMMS simulation software.
Due to the different scales of debris flow in Yizhong River, in order to analyze the dynamic mechanism of debris flow disasters, this study takes the simulation results with a volume of 3,000 m3 as a case for analysis. Figure 11 shows the maximum flow thickness, velocity and pressure at each location when the debris flow volume is 3,000 m3. Figure 11A show that the flow thickness of debris flow has little change in the flow process. Since the a-d section is located in a narrow valley and the f-h section is located in multiple retaining dams, the flow thickness is slightly higher than that of the d-f section. Figure 11B show that the maximum flow velocity presents three stages: a-d, d-f and f-h, and the velocity gradually decreases. It shows that after the debris flow in Yizhong River broke out, the potential energy quickly changed into kinetic energy, and the velocity increased rapidly. However, in the subsequent movement process, although the potential energy continues to be converted into kinetic energy, the speed does not continue to increase due to the restriction of the terrain, but gradually decreases. After reaching national highway G214 (h point), the overall speed drops to less than 0.5 m/s. Figure 11C show that the maximum flow pressure also shows three stages, a-d, d-f and f-h, and gradually decreases. Among them, the maximum flow pressure in the a-d section reaches 224 kPa, indicating that the downward erosion capacity of debris flow is strong, which will further aggravate the depth of the valley, form retrogressive erosion, and reduce the stability of the slopes on both banks and the bedrock in the source area. At points f and g, debris flow velocity decreases and flow pressure decreases rapidly due to terrain levelling and check dams, resulting in reduced disturbance of landslides L1 and Unstable slopes Us1, making the slopes relatively stable during small-scale debris flow outbursts. After reaching the national highway G214 (h point), the pressure of the debris flow is almost zero (mainly from the gravity of the debris flow fluid itself), so the destructive force of the debris flow on the national highway G214 is small, which is consistent with the field observation results.
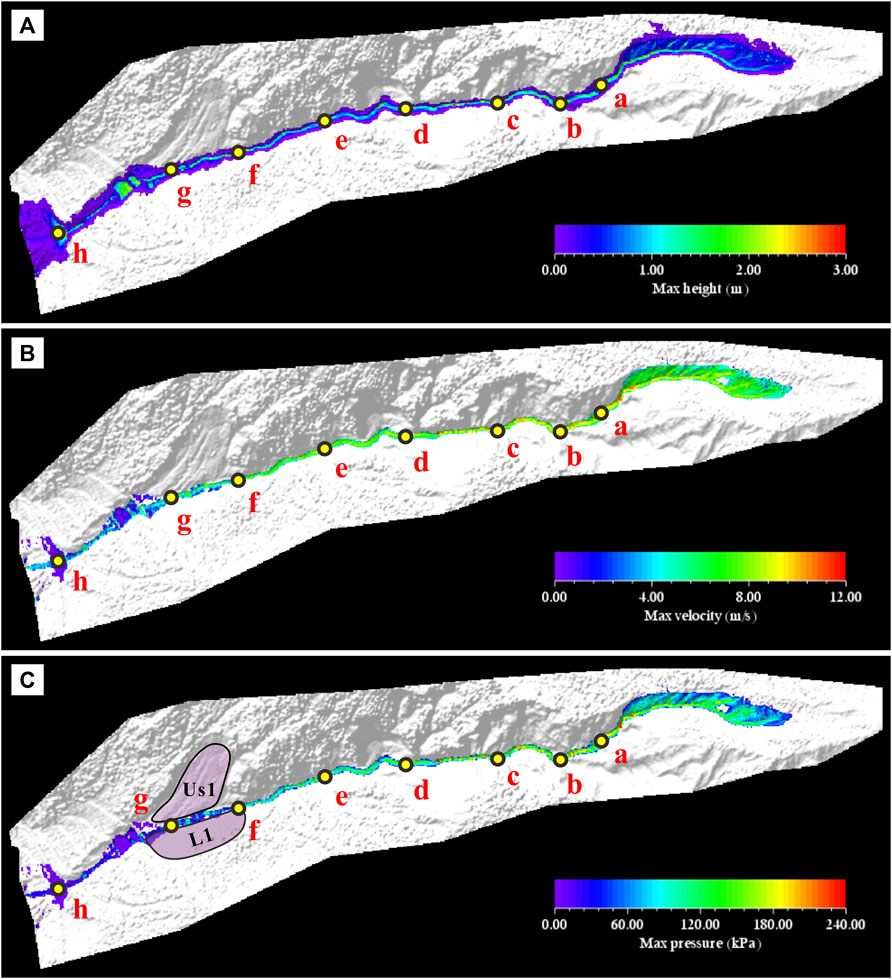
Figure 11. Results of RAMMS simulation of the 3,000 m³ square volume debris flow. (A) Diagram of maximum flow thickness; (B) Diagram of maximum flow velocity; (C) Diagram of maximum flow pressure.
In order to study the dynamic mechanism of debris flow disaster in Yizhong River with the increase of volume, six groups of simulation tests ranging from 3,000 m3 to 8,000 m3 were conducted in this study, and the results are shown in Figure 12. As can be seen from the point line diagram made from the data extracted from each point, with the increase of volume, the flow thickness, flow velocity and flow pressure of each point show an increasing trend, but the increase is not significant. In Figure 12B, in section a to c, the maximum flow thickness tends to increase, in section c to d, the maximum flow thickness decreases very rapidly, and in section d to h, the maximum flow thickness increases slowly and then stabilises. In Figures 12A–C section, the maximum flow velocity is slowly decreasing trend, c to e section of the maximum flow velocity change amplitude is not large, in a stable state, d to h section of the maximum flow velocity is rapidly reduced, the maximum flow velocity of each working condition in the point of h (national highway G214) are reduced to less than 1 m/s. In Figure 12D, the trend of the maximum flow pressure is the same as that of the maximum flow velocity, and the pressure decreases to almost zero when reaching the h point for each working condition.
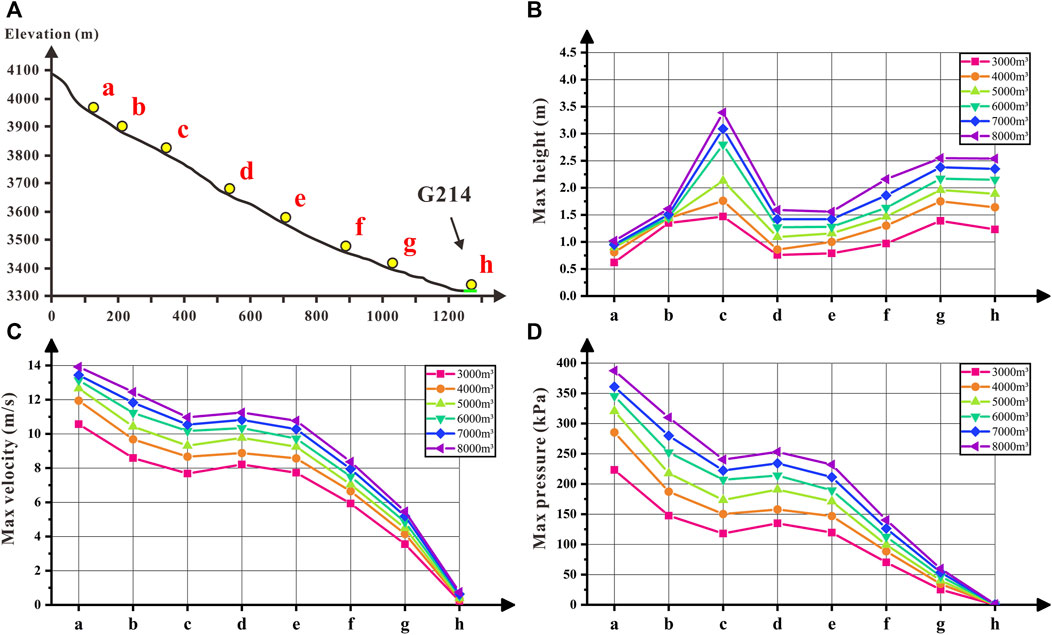
Figure 12. Comparison chart of RAMMS simulation results. (A) Schematic diagram of the location of the data extraction points; (B) Comparison of the maximum flow thickness at each point; (C) Comparison of the maximum flow velocity at each point; (D) Comparison of the maximum flow pressure at the flow surface at each point.
According to the simulation results, although the volume increases, the velocity of debris flow in Yizhong River is still slow when it flows into national highway G214 from the gully mouth, because the debris flow is limited by the terrain conditions during its movement. Therefore, combined with the analysis of the disaster record data, it can be seen that, with the increase in the volume of debris flow outbreaks, the threat of the Yizhong River debris flow disaster to the national highway G214 mainly lies in the blockage and damage to the road. The threat to the county urban area lies mainly in the fact that, as the volume of debris flow increases, the debris flow will silt up and block the culvert at the confluence of the Yizhong River and the Zhiqu River, and at the same time, overflow into the Kawagabo Highway, destroying the surrounding infrastructures and posing a threat to the No. One Middle School in Deqin County and the residential areas.
Discussion
The Yizhong River is located in the Lancang River tectonic denudation alpine valley geomorphology area, with dense active fractures, broken rock structure, complex and fragile geological conditions, and three faults directly through the Yizhong River. After on-site investigation, it is determined that the bedrock in the source area is controlled by bedding planes and two groups of joints. The bedrock rock mass is cut into rectangular or rhombus-shaped blocks (conjugate shear), and the bedding plane is inversely inclined, so that when the whole is relatively stable, the surface weathered blocks are easy to peel off and accumulate in the valleys of the source area to form potential provenance. The bedrock on the north is covered with moraine, and the scale is huge. Under the action of rainfall, the combination of retrogressive erosion in the northern gullies and the collapse of blocks to the south becomes the initial source of debris flow occurrence. Therefore, the same as the single outbreak debris flow disaster is that the material source initiates the formation of debris flow under the action of rainfall (Yin et al., 2016; Zhang and Matsushima, 2016), and the difference is that the geomechanical model of Yizhong River debris flow gully responds to the process of continuous evolution of the geomorphology of Yizhong River debris flow gully under the action of internal and external dynamics coupling and thus reflects the characteristics of the frequency of the debris flow disaster in the rainy season.
It can be seen from Figure 13 that although the daily rainfall of Deqin County in the rainy season in 2021 is less than that in 2019 and 2020, the number of outbreaks increases instead, as well as the consideration of climate change impacts on permafrost (Pei et al., 2021; Liu et al., 2024), it indicates that retrogressive erosion in source area and weathering and denudation of exposed bedrock are accelerating, thus providing material sources for the formation of debris flow. Therefore, it also provides the possibility of large-scale debris flow disaster, which is a great threat to the county town in the downstream. As can be seen from the results of the dynamic simulation, the main disaster caused by the outbreak of large-scale debris flow is that it will block the culvert at the lower part of Kawagebo Avenue, which will further cause damage to the county city. Therefore, the main problems should be solved in stages. First of all, the box culvert under Kawagebo Avenue at the intersection of Yizhong River and Zhiqu River will be deeply excavated to increase the longitudinal gradient, so as to ensure that the small-scale debris flow can be smoothly discharged into Zhiqu River and then discharged through the river. To prevent debris flow from silting up in the culvert below Kawagebo avenue, which will cause multi-stage blockage, and then the debris flow overflow culvert will pose a threat to the surrounding buildings. The second is to carry out the source fixation and drainage work in the source area, so as to control the debris flow disaster in the Yizhong River from the source. In addition, the emergency evacuation plan of Zhiqu River blocked by large-scale debris flow must be well prepared. Adequate prevention and treatment will significantly reduce disaster risk (He et al., 2024).
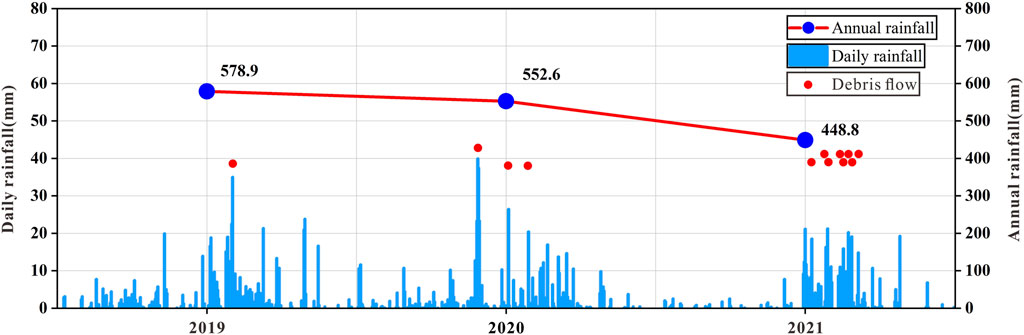
Figure 13. Rainfall data and records of debris flow outbreaks in Deqin County from 2019 to 2021 (Data from china meteorological administration).
Because the debris flow in the Yizhong River is located at a high altitude and the ditch is deep and wide, the traditional on-site survey method is difficult to carry out survey work, and the risk is extremely high (during the survey in the ditch, there are rockfalls on the steep slope without warning). Therefore, although the geological disaster of debris flow in the Yizhong River has already attracted widespread attention (Wang, 2016), due to the limitation of objective conditions, it has not been able to solve the detailed survey work and provide accurate geological models and data. As a result, the root causes of disasters are not clearly understood and disasters cannot be dealt with from the root. It is more to adopt middle and lower reaches blocking technology to passively protect against disasters. This can lead to high long-term maintenance costs for the project, as well as a lack of a clear understanding of potential safety hazards, thus lacking the necessary application of early monitoring and warning techniques, and plans and measures to respond to emergencies, which is a fatal hazard for the urban areas of the county downstream of the Yizhong River. Therefore, solving the problem of detailed geological exploration in alpine gorge area is an urgent problem that needs to be broken through. Hence, in alpine gorge areas, the geological survey work needs to introduce new survey techniques to deal with the above problems. Therefore, the rope bridge technique is proposed in this paper to transport the surveyor to the corresponding predetermined location for the survey, and the nap-of-the-object photogrammetry is used to establish a refined three-dimensional model (He, 2019; Liang et al., 2020), which has vector coordinates, the required geological information can be extracted and analyzed indoors. The combination of the two can carry out a “point-surface” combination of disaster points that are difficult to conduct on-the-spot investigation in the alpine, deep ditch and gorge areas to carry out regional detailed investigations and collect required information.
Conclusion
In this paper, the geological environment, geomechanical model and disaster-causing kinetic mechanism of the Yizhong River debris flow disaster in Deqin County, Yunnan Province, China, were studied, as well as the combination of point and surface survey methods based on the topographic and geomorphological features of the alpine gorge area and the limitations of the on-site survey were proposed. The main results are as follows:
(a) Under the action of tectonic movement, the source area of Yizhong River debris flow disaster is subjected to conjugate shear action, forming a fracture zone, resulting in rock structure fragmentation, and becoming a source of debris flow material under the action of retrospective erosion and weathering and spalling, which provides a basis for the breeding of the disaster. The northern part of the source area has accumulated 3×105 m3 of moraine, which is prone to avalanches and slides to form the source of debris flow under the induced action of rainfall. Therefore, during the rainy season, under the action of frequent rainfall (external force), the loose material is prompted to start and frequent outbreaks of debris flow disasters. Since the scale of debris flow outbreaks is limited by the catchment area of the watershed, small and medium-sized outbreaks are dominant. The conjugate shear-type geomechanical model is proposed to explain the mechanism of the frequent occurrence of disasters, and at the same time, provide a scientific basis for the prevention and control of disasters.
(b) After the debris flow starts in the source area, the potential energy is rapidly converted into kinetic energy for downward movement, but the speed gradually decreases due to the topographic conditions, and eventually drops below 1 m/s when it reaches national highway G214, and then flows into the drainage channel below. Therefore, the threat posed by the Yizhong River debris flow disaster to urban residents is mainly concentrated on the confluence of the Yizhong River and the Zhiqu River. For the management of the disaster, it is necessary to solve the drainage problem of the confluence in the short term, and secondly, for the long-term development, it is necessary to solidify the source of the loose accumulation and broken rock in the source area, as well as to do the drainage work. In this way the debris flow hazard of the Yizhong River can be managed at its root. The corresponding management ideas can provide reference for similar disaster management.
(c) The complex geological conditions in alpine valley areas make it difficult to carry out traditional on-site survey work, which is a major problem in disaster prevention and control work in alpine valley areas. Through the application of the nap-of-the-object photogrammetry technology and the creation of high-precision three-dimensional models, detailed survey work can be carried out on hazard sites to compensate for the lack of manual surveys.
Data availability statement
The original contributions presented in the study are included in the article/Supplementary material, further inquiries can be directed to the corresponding author.
Ethics statement
Written informed consent was obtained from the individual(s) for the publication of any identifiable images or data included in this article.
Author contributions
YW: Writing–original draft, Validation, Resources, Project administration, Methodology, Data curation. QH: Validation, Writing–review and editing, Supervision, Software, Resources, Methodology, Investigation, Funding acquisition, Data curation. WW: Writing–review and editing, Methodology, Funding acquisition, Conceptualization. NZ: Writing–review and editing, Resources, Methodology, Formal Analysis, Conceptualization. LC: Writing–original draft, Resources, Project administration, Investigation, Formal Analysis, Data curation, Conceptualization. ZL: Writing–original draft, Resources, Investigation, Formal Analysis, Data curation, Conceptualization. DC: Writing–original draft, Visualization, Resources, Project administration, Investigation, Formal Analysis, Data curation. LY: Writing–original draft, Validation, Resources, Project administration, Methodology, Investigation, Formal Analysis, Data curation, Conceptualization.
Funding
The author(s) declare that financial support was received for the research, authorship, and/or publication of this article. This study was supported by Co-funded projects of the National Natural Science Foundation of China (U2244227), and National Key R&D Program of China (2022YFC3004301), and Geological Survey Project of China Geological Survey: Investigation and Evaluation of Geological Disaster Risks in Key Areas (DD20221748).
Conflict of interest
Authors YW, LC, ZL, DC, and LY were employed by Yunnan Geological Engineering Second Survey Institute Co., Ltd.
The remaining authors declare that the research was conducted in the absence of any commercial or financial relationships that could be construed as a potential conflict of interest.
Publisher’s note
All claims expressed in this article are solely those of the authors and do not necessarily represent those of their affiliated organizations, or those of the publisher, the editors and the reviewers. Any product that may be evaluated in this article, or claim that may be made by its manufacturer, is not guaranteed or endorsed by the publisher.
References
Aydin, A., Buhler, Y., Christen, M., and Gürer, I. (2014). Avalanche situation in Turkey and back calculation of selected events. Nat. Hazards Earth Syst. Sci. 14 (5), 1145–1154. doi:10.5194/nhess-14-1145-2014
Berti, M., Genevois, R., Simoni, A., and Tecca, P. R. (1999). Field observations of a debris flow event in the Dolomites. Geomorphology 29 (3), 265–274. doi:10.1016/s0169-555x(99)00018-5
Chen, N. S., Yang, C. L., Zhou, W., Hu, G., Li, H., and Hand, D. (2009). The critical rainfall characteristics for torrents and debris flows in the wenchuan earthquake stricken area. J. Mt. Sci. 6 (004), 362–372. doi:10.1007/s11629-009-1064-9
Christen, M., Bartelt, P., and Kowalski, J. (2010a). Back calculation of the in den Arelen avalanche with RAMMS: interpretation of model results. Ann. Glaciol. 51 (54), 161–168. doi:10.3189/172756410791386553
Christen, M., Kowalski, J., and Bartelt, P. (2010b). RAMMS: numerical simulation of dense snow avalanches in three-dimensional terrain. Cold Reg. Sci. Technol. 63 (1-2), 1–14. doi:10.1016/j.coldregions.2010.04.005
Cui, P., Chen, X. Q., Zhu, Y. Y., Su, F. H., Wei, F. Q., Han, Y. S., et al. (2011). The wenchuan earthquake (may 12, 2008), sichuan Province, China, and resulting geohazards. Nat. Hazards 56 (1), 19–36. doi:10.1007/s11069-009-9392-1
Cui, P., and Guo, J. (2021). Evolution models,risk prevention and control countermeasures of the valley disaster chain. Adv. Eng. Sci. 53 (3), 5–18 (in Chinese). doi:10.15961/j.jsuese.202100285
Davies, T. R., and McSaveney, M. J. (1999). Runout of dry granular avalanches. Can. Geotech. J. 36, 313–320. doi:10.1139/t98-108
Feng, Z., Li, B., Yin, Y., and He, K. (2014). Rockslides on limestone cliffs with subhorizontal bedding in the southwestern calcareous area of China. Nat. Hazards Earth Syst. Sci. 14, 2627–2635. doi:10.5194/nhess-14-2627-2014
Gallino, G. L., and Pierson, T. C. (1984). The 1980 Polallie Creek debris flow and subsequent dam-break flood. East Fork Hood River basin, Or. Open-File Rep.
Gan, J. J., and Zhang, Y. X. (2019). Numerical simulation of debris flow runout using RAMMS: a case study of Luzhuang Gully in China. CMES-Comp. Model. Eng. Sci. 121 (3), 981–1009. doi:10.32604/cmes.2019.07337
Gao, Y., Yin, Y., Li, B., et al. (2018). Characteristics and numerical runout modeling analysis of the Jiweishan landslide, Chongqing, China. Environ. Eng. Geoscience 2018. doi:10.5555/XX.XX.XX
Gao, Y., Yin, Y., Li, B., Feng, Z., Wang, W., Zhang, N., et al. (2017). Characteristics and numerical runout modeling of the heavy rainfall-induced catastrophic landslide–debris flow at Sanxicun, Dujiangyan, China, following the Wenchuan Ms 8.0 earthquake. Landslides 14 (4), 1361–1374. doi:10.1007/s10346-016-0793-4
Godt, J. W., and Coe, J. A. (2007). Alpine debris flows triggered by a 28 July 1999 thunderstorm in the central Front Range, Colorado. Geomorphology 84 (1-2), 80–97. doi:10.1016/j.geomorph.2006.07.009
Guo, J., Cui, Y., Xu, W., Yin, Y., Li, Y., and Jin, W. (2022). Numerical investigation of the landslide-debris flow transformation process considering topographic and entrainment effects: a case study. Landslides 19 (4), 773–788. doi:10.1007/s10346-021-01791-6
He, J. N. (2019). Nap-of-the-Object photogrammetry and its key techniques. Wuhan: Wuhan University. Ph.D. Dissertation.
He, Q., Guo, F. Y., Li, R. D., Wang, L., Wang, W., Zhang, N., et al. (2023). Characteristics, mobility and dynamic of the Yahuokou flow-like landslide in Zhouqu, Gansu, China. Gansu, China. Landslides 20, 629–643. doi:10.1007/s10346-022-02000-8
He, Q., Wang, Y., Wang, W. P., Xu, W., Zhao, G., Chen, L., et al. (2024). Mechanism of the November 2018 landslide at the Kunming landfill and the geotechnical engineering risk control in the process of urbanization. Bull. Eng. Geol. Environ. 83, 196. doi:10.1007/s10064-024-03703-z
Hu, K. H., Cui, P., and Zhang, J. Q. (2012). Characteristics of damage to buildings by debris flows on 7 August 2010 in Zhouqu, Western China. Nat. hazards earth Syst. Sci. 12 (7), 2209–2217. doi:10.5194/nhess-12-2209-2012
Hu, K. H., Wu, C. H., Tang, J. B., Pasuto, A., Li, Y., and Yan, S. (2018). New understandings of the june 24th 2017 xinmo LandslideMaoxian, sichuan, China. Landslides 15 (12), 2465–2474. doi:10.1007/s10346-018-1073-2
Hu, W., Xu, Q., Asch, T. V., and Zhu, X. (2014). Flume tests to study the initiation of huge debris flows after the Wenchuan earthquake in S-W China. Eng. Geol. 182, 121–129. doi:10.1016/j.enggeo.2014.04.006
Hungr, O. (1995). A model for the runout analysis of rapid flow slides, debris flows, and avalanches. Can. Geotech. J. 32 (4), 610–623. doi:10.1139/t95-063
Hungr, O. (2008). Simplified models of spreading flow of dry granular material. Can. Geotech. J. 45 (8), 1156–1168. doi:10.1139/t08-059
Hungr, O., and Evans, S. G. (2004). Entrainment of debris in rock avalanches: an analysis of a long–runout mechanism. Geol. Soc. Am. Bull. 116, 1240–1252. doi:10.1130/b25362.1
Iverson, R. M., Reid, M., and LaHusen, R. G. (1997). Debris-flow mobilization from landslides. Annu. Rev. Earth Planet. Sci. 25, 85–138. doi:10.1146/annurev.earth.25.1.85
Iverson, R. M., Reid, M. E., Logan, M., LaHusen, R. G., Godt, J. W., and Griswold, J. P. (2011) Positive feedback and momentum growth during debris-flow entrainment of wet bed sediment. Nat. Geosci., 4(2):116–121. doi:10.1038/ngeo1040
Li, Y., Ma, C., and Wang, Y. (2019). Landslides and debris flows caused by an extreme rainstorm on 21 July 2012 in mountains near Beijing, China. Bull. Eng. Geol. Environ. 78 (2), 1265–1280. doi:10.1007/s10064-017-1187-0
Liang, J. T., Tie, Y. B., Zhao, C., et al. (2020). Technology and method research on the early detection of high-level collapse based on the nap-of-the-object photograph. Geol. Surv. China 7 (5), 107–113 (in Chinese). doi:10.19388/j.zgdzdc.2020.05.12
Liu, Y., Qiu, H. J., Ulrich, K., Wang, N., Wang, J., Huang, C., et al. (2024). Higher temperature sensitivity of retrogressive thaw slump activity in the Arctic compared to the Third Pole. Sci. Total Environ. 914, 170007. doi:10.1016/j.scitotenv.2024.170007
Mahboob, M. A., Iqbal, J., and Atif, I. (2015). Modeling and simulation of glacier avalanche: a case study of Gayari sector glaciers hazards assessment. IEEE Throughput Geosci. Remote. 53 (11), 5824–5834. doi:10.1109/tgrs.2015.2419171
Payne, S. J., James, E. Z., and David, W. R. (2004). Stress triggering of conjugate normal faulting: late aftershocks of the 1983 ms 7.3 borah peak, Idaho, earthquake. Bull. Seismol. Soc. Am. 94 (3), 828–844. doi:10.1785/0120030122
Pei, Y. Q., Qiu, H. J., Hu, S., Yang, D., Zhang, Y., Ma, S., et al. (2021). Appraisal of tectonic-geomorphic features in the hindu kush- himalayas. Earth Space Sci. 8, e2020EA001386. doi:10.1029/2020ea001386
Pudasaini, S. P., and Fischer, J. T. (2020). A mechanical model for phase separation in debris flow. Int. J. Multiph. Flow 129, 103292. doi:10.1016/j.ijmultiphaseflow.2020.103292
Qiu, H. J., Su, L. L., Tang, B. Z., Yang, D., Ullah, M., Zhu, Y., et al. (2024). The effect of location andgeometric properties of landslides caused by rainstorms andearthquakes. Earth Surf. Process. Landforms, 1–13. Available from. doi:10.1002/esp.5816
Sassa, K., Nagai, O., Solidum, R., Yamazaki, Y., and Ohta, H. (2010). An integrated model simulating the initiation and motion of earthquake and rain induced rapid landslides and its application to the 2006 Leyte landslide. Landslides 7 (3), 219–236. doi:10.1007/s10346-010-0230-z
Singh, D. K., Mishra, V. D., and Gusain, H. S. (2020). Simulation and analysis of a snow avalanche accident in lower western Himalaya, India. J. Indian Soc. Remote Sens. 48 (11), 1555–1565. doi:10.1007/s12524-020-01178-5
Takahashi, T. (1981). Debris flow. Annu. Rev. Fluid Mech. 13, 57–77. doi:10.1146/annurev.fl.13.010181.000421
Tang, C., Rengers, N., ThAsch, W. J. van, Yang, Y. H., and Wang, G. F. (2011). Triggering conditions and depositional characteristics of a disastrous debris flow event in Zhouqu city, Gansu Province, northwestern China. Nat. Hazards Earth Syst. Sci. 11, 2903–2912. doi:10.5194/nhess-11-2903-2011
Wang, G., Sassa, K., and Fukuoka, H. (2003). Downslope volume enlargement of a debris slide–debris flow in the 1999 Hiroshima, Japan, rainstorm. Eng. Geol. 69 (3-4), 309–330. doi:10.1016/s0013-7952(02)00289-2
Wang, W. P., Yin, Y. P., Zhu, S. N., Wei, Y., Zhang, N., and Yan, J. (2019). Dynamic analysis of a long-runout, flow-like landslide at Areletuobie, Yili River valley, northwestern China. B Eng. Geol. Environ. 78, 3143–3157. doi:10.1007/s10064-018-1322-6
Wang, Y. (2016). The Forming conditions and engineering revention of Yizhong river debris flow in Yunnan Province Deqin county, Engineering Master's Thesis. Beijing), Beijing: China University of Geosciences.
Wei, R., Zeng, Q., Davies, T., Yuan, G., Wang, K., Xue, X., et al. (2018). Geohazard cascade and mechanism of large debris flows in Tianmo gully, SE Tibetan Plateau and implications to hazard monitoring. Eng. Geol. 233, 172–182. doi:10.1016/j.enggeo.2017.12.013
Wu, F. Y., Jiang, L. W., Zhang, G. Z., et al. (2019). The fault activity and seismic hazard assessment of central north segment of the Deqin-Zhongdian fault, southeastern Qinghai-Tibet plateau. Acta Geol. Sin. 93 (10), 10 (in Chinese). doi:10.19762/j.cnki.dizhixuebao.2019261
Xing, A. G., Wang, G. H., Li, B., Jiang, Y., Feng, Z., and Kamai, T. (2015). Long-runout mechanism and landsliding behaviour of large catastrophic landslide triggered by heavy rainfall in Guanling, Guizhou, China. Can. Geotech. J. 52 (7), 971–981. doi:10.1139/cgj-2014-0122
Xu, Q., Fan, X. M., and Dong, X. J. (2010). Characteristics and formation mechanism of a catastrophic rainfall–induced rock avalanche–mud flow in Sichuan, China. Landslides 9, 143–154. doi:10.1007/s10346-011-0278-4
Yang, J., Zeng, Z. X., Li, M. H., et al. (2015). The seismo-geological hazards and seismogenic structure of the 2013 deqing-derong 5.9 earthquake. Earth Sci. - J. China Univ. 10, 9 (in Chinese). doi:10.3799/dqkx.2015.153
Ye, B. F., Qiu, H. J., Tang, B. Z., Liu, Y., Liu, Z., Jiang, X., et al. (2024). Creep deformation monitoring of landslides in a reservoir area. J. Hydrology 632, 130905. doi:10.1016/j.jhydrol.2024.130905
Yin, A., and Taylor, M. H. (2011). Mechanics of V-shaped conjugate strike-slip faults and the corresponding continuum mode of continental deformation. Geol. Soc. Amercia Bull. 123 (9-10), 1798–1821. doi:10.1130/b30159.1
Yin, Y., Li, B., Gao, Y., Wang, W., Zhang, S., and Zhang, N. (2023). Geostructures, dynamics and risk mitigation of high-altitude and long-runout rockslides. J. Rock Mech. Geotech. 15 (1), 66–101. doi:10.1016/j.jrmge.2022.11.001
Yin, Y. P. (2009). Features of landslides triggered by the wenchuan earthquake. J. Eng. Geol. (01), 31–40 (in Chinese).
Yin, Y. P., Cheng, Y. L., Liang, J. T., and Wang, W. (2016). Heavy-rainfall-induced catastrophic rockslide-debris flow at sanxicun, dujiangyan, after the wenchuan ms 8.0 earthquake. Landslides 13 (1), 9–23. doi:10.1007/s10346-015-0554-9
Yin, Y. P., Wang, W. P., Zhang, N., et al. (2017). The June 2017 Maoxian landslide:Geological disaster in an earthquake area after the Wenchuan Ms 8.0 earthquake. Sci. ChinaTechnological Sci. (11), 170–174.
Young, S., and Pollard, D. D. (2000). Conjugate normal faults in layered sedimentary rocks. Geol. Soc. Amercia Bull. 31.
Zhang, J. R., Tang, H. M., Li, C. D., Gong, W., Zhou, B., and Zhang, Y. (2024a). Deformation stage division and early warning of landslides based on the statistical characteristics of landslide kinematic features. Landslides 21, 717–735. doi:10.1007/s10346-023-02192-7
Zhang, J. R., Tang, H. M., Tan, Q. W., Mao, M., Zhou, B., and Zhang, Y. (2024b). A generalized early warning criterion for the landslide risk assessment: deformation probability index (DPI). Acta Geotech. doi:10.1007/s11440-023-02199-3
Zhang, M., Yin, Y. P., and Wu, S. R. (2010). Development status and prospects of studies on kinematics of long runout rock avalanches. J. Eng. Geol. 18 (6), 805–817 (in Chinese). doi:10.3969/j.issn.1004-9665.2010.06.001
Zhang, N., and Matsushima, T. (2016). Simulation of rainfall-induced debris flow considering material entrainment. Eng. Geol. 214 (Complete), 107–115. doi:10.1016/j.enggeo.2016.10.005
Zhang, T. T., Yin, Y. P., Li, B., Liu, X., Wang, M., Gao, Y., et al. (2022). Characteristics and dynamic analysis of the February 2021 long-runout disaster chain triggered by massive rock and ice avalanche at Chamoli, Indian Himalaya. J. Rock Mech. Geotechnical Eng. 15, 296–308. doi:10.1016/j.jrmge.2022.04.003
Zhao, B., Zhang, H. Q., Liao, H. J., Li, W., He, W., et al. (2021). Emergency response to the reactivated Aniangzhai landslide resulting from a rainstorm-triggered debris flow, Sichuan Province, China. Landslides 18 (3), 1115–1130. doi:10.1007/s10346-020-01612-2
Zhou, J. W., Cui, P., Yang, X. G., Su, Z. m., and Guo, X. j. (2013). Debris flows introduced in landslide deposits under rainfall conditions:the case of wenjiagou gully. J. Mt. Sci. 010 (002), 249–260. doi:10.1007/s11629-013-2492-0
Keywords: debris flow, frequent occurrence mechanism, geomechanical model, dynamical mechanism, photogrammetry
Citation: Wang Y, He Q, Wang W, Zhang N, Chen L, Liu Z, Chen D and Yang L (2024) Analysis on the mechanism and dynamics of frequent debris flows in typical alpine gorges areas—a case study of Yizhong river in Deqin County, Yunnan, China. Front. Earth Sci. 12:1418763. doi: 10.3389/feart.2024.1418763
Received: 17 April 2024; Accepted: 22 May 2024;
Published: 12 June 2024.
Edited by:
Junrong Zhang, China University of Geosciences Wuhan, ChinaReviewed by:
Haijun Qiu, Northwest University, ChinaSha Lu, China University of Geosciences Wuhan, China
Copyright © 2024 Wang, He, Wang, Zhang, Chen, Liu, Chen and Yang. This is an open-access article distributed under the terms of the Creative Commons Attribution License (CC BY). The use, distribution or reproduction in other forums is permitted, provided the original author(s) and the copyright owner(s) are credited and that the original publication in this journal is cited, in accordance with accepted academic practice. No use, distribution or reproduction is permitted which does not comply with these terms.
*Correspondence: Qing He, 1361715761@qq.com