- 1Wuhan Center, China Geological Survey, Geosciences Innovation Center of Central South China, Wuhan, Hubei, China
- 2Faculty of Engineering, China University of Geosciences, Wuhan, China
- 3Hubei Key Laboratory of Disaster Prevention and Mitigation, China Three Gorges University, Yichang, Hubei, China
- 4College of Civil Engineering and Architecture, China Three Gorges University, Yichang, Hubei, China
Potential geohazards triggered by the rock mass deterioration (RMD) of the hydro-fluctuation belt (HFB) in the Three Gorges Reservoir area (TGRA) severely threaten the lives and property of people, it is necessary and urgent to timely identify this kind of potential geohazard. Aiming at this issue, several typical evolution modes of potential RMD-induced geohazards were generalized, and an unmanned aerial vehicle (UAV) photographic method integrating the orthophotography, three-dimensional multi-angle oblique photography and high-precision digital elevation model (DEM) was proposed to early recognize the newly formed potential RMD-induced geohazards. By resolving the problem of poor precision in the orthophotographic images of high steep bank slopes, the proposed method improved the recognition reliability of potential reservoir geohazards in the HFBs. Taking the bank slopes from Zigui County to Badong County as the study area, 116 new potential RMD-induced geohazard sites were identified by the presented approach, including 64 and 52 sites on the left and right bank, respectively. Through field verifications, 17 and 19 sites on the left and right bank were found to be consistent with existing hazards, indicating the effectiveness of the proposed approach in avoiding the false and missing recognitions of potential RMD-induced reservoir geohazards at current levels of knowledge and technology. The study can provide a valuable reference for the early identification of RMD-induced geohazards in reservoir areas.
1 Introduction
In the Three Gorges Reservoir area (TGRA), periodic reservoir water impoundment and drawdown led to the repeated water-level fluctuations (Yin et al., 2016), causing periodic changes in the geological environment conditions of the bank slopes. These changes have dramatically degraded the structure and the physio-mechanical properties of the rock masses in the hydro-fluctuation belt (HFB) (Liu et al., 2008; Liu et al., 2009; Deng et al., 2012a; Deng et al., 2012b; Sun and Zhang, 2019).
Since the implementation of the 175 m experimental impoundment in the TGRA in 2008, geological disasters caused by rock mass deterioration (RMD) in the HFB have grown more common (Huang et al., 2018; Tang et al., 2019; Zhang et al., 2022). By now, a series of studies related to the characteristics, mechanisms, consequences and evaluations of the RMD of the HFB have been carried out (Liu et al., 2008; Liu et al., 2009; Liu et al., 2018; Liu et al., 2020; Kang et al., 2019; Huang et al., 2019; Huang et al., 2020a; Huang et al., 2020b; Huang et al., 2021; Yao et al., 2020; Yan et al., 2020; Yin et al., 2020a; Yin et al., 2020b; Wang et al., 2020a; Wang et al., 2020b; Gu et al., 2021), and it is believed that the RMD of the HFB is an emerging adverse factor affecting the stability of the reservoir bank slopes. For instance, the Kamenziwan landslide (Figure 1A), which occurred in Zigui County of the TGRA on 10 December 2019, was a typical rockslide triggered by the RMD of the HFB. This rockslide destroyed a highway for the transportation of 12,300 people lived in nearby areas, and also damaged citrus groves of 90 acres, several water supply and power lines in the town. Fortunately, no casualties were reported in this landslide event owing to the reliable early warning and effective countermeasures (Yin et al., 2020a). Besides, the continuous RMD of the HFB significantly increased the instability risk of emerging and existing dangerous rock masses (e.g., the Jianchuandong, Banbiyan, Guanmuling, and Huangyanwo dangerous rock masses in the Wuxia Gorge and the Xiling Gorge), severely threatening the safety of water transportation in the Yangtze River and the lives and property of people living in these areas (Zhang C Y et al., 2021; Yin et al., 2022). Taking the Jianchuandong dangerous rock mass (Figure 1B) as an example, the cumulative displacement of its base has reached 55 mm so far. Once it collapses suddenly, the consequences can be devastating, just like the tragedy that a towering slab of rock broke from a cliff and toppled onto pleasure boaters drifting near a waterfall on the Furnas Lake, Brazil, occurred on 8 January 2022 (Figure 2). According to the video images and related reports of this catastrophic accident, there were reasons to believe that the long-term continuous RMD of the HFB played a crucial role. Therefore, early identification and warning of potential geohazards in the reservoir bank slopes from the perspective of the RMD of the HFB is highly prioritized and important for the disaster prevention and mitigation.
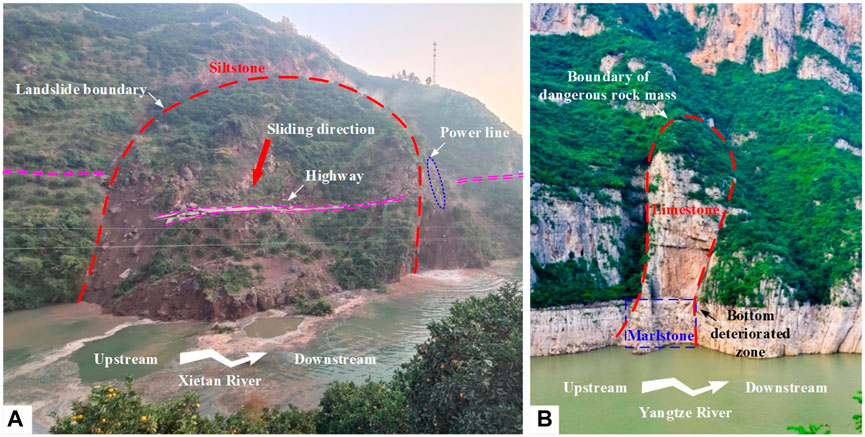
Figure 1. Typical geohazards related to the RMD of the HFB in the TGRA: (A) Kamenziwan landslide; (B) Jianchuandong dangerous rock mass.
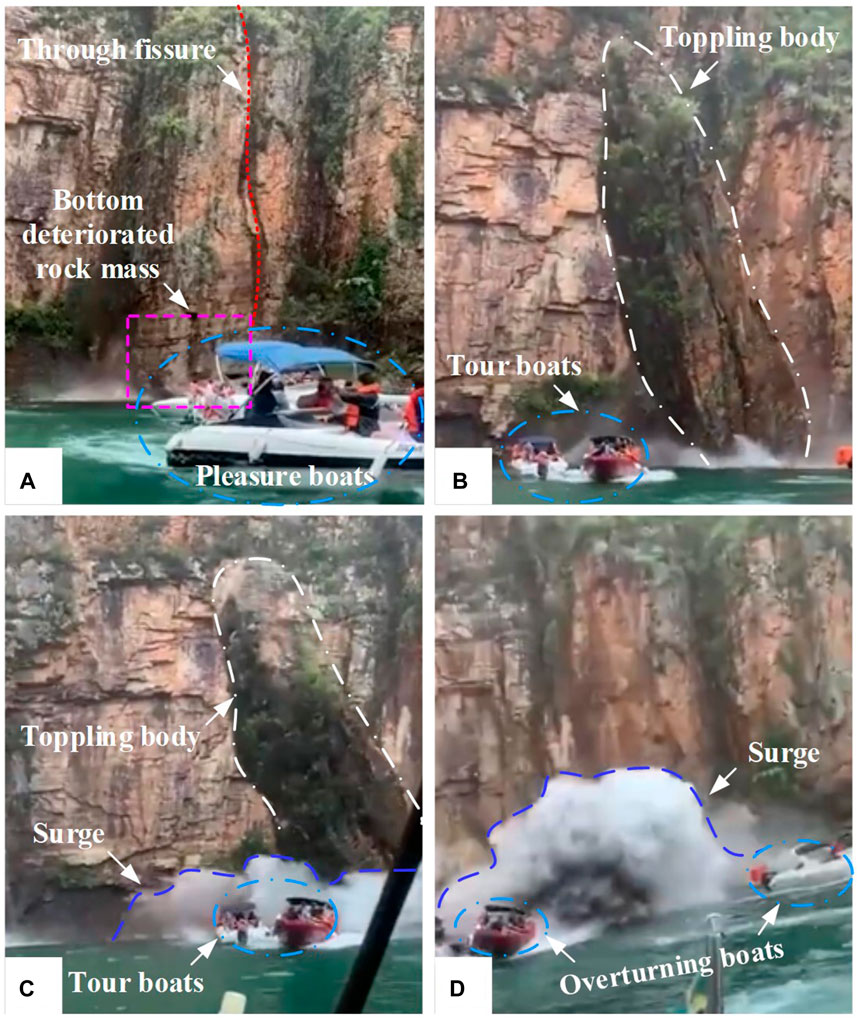
Figure 2. On January 8, 2022, a massive rock slab detached from a cliff and fell onto leisure boaters floating near a waterfall at Furnas Lake, Brazil. The incident involved the following sequence of events: (A) the rock had evidently experienced deterioration, forming a weak area at the base of the slope prior to the collapse; (B) the moment the failure was initiated; (C) the rockfall generated a powerful surge in the water; and (D) the resulting surges led to the boat capsizing.
Conventionally, the geohazards are generally recognized by field mapping, geophysical survey or engineering investigation, and the requirements of manpower, resources, professional experience and abilities in line with the objectives of good accuracy limit their practical application. Now the mainstream methods for the identifications of reservoir geohazards include the remote sensing and photogrammetric techniques, the former mainly includes the terrestrial laser scanning, airborne laser scanning, infrared thermography, ground based synthetic aperture radar, and differential interferometric synthetic aperture radar, and the latter mainly include the aerial photogrammetry and close range photogrammetry. These techniques are capable of obtaining the information on the temporal and spatial distributions, kinematics of potential geohazards (Hervás et al., 2003; Yin et al., 2010; Tofani et al., 2013; Wasowski and Bovenga, 2014; Sun et al., 2015; Dong et al., 2018; Carlà et al., 2019; Macciotta and Hendry, 2021), and their implementations usually need a carrier such as aircraft, spacecraft, terrestrial-based platform, and so on. By utilizing these techniques, even the three dimensional large-scale terrain mappings of the unsafe, inaccessible, or vulnerable areas for investigators can be achieved efficiently and quickly (Oppikofer et al., 2009; Abellán et al., 2014). Due to the revisit period of satellites and the high requirement of professional skills in processing the acquired data, the applicability of these techniques for early recognition of geohazards may be limited. The advantages and disadvantages of these techniques are briefly clarified in the Table 1 (Hervás et al., 2003).
As to the practical application of these techniques in recognition and monitoring of reservoir geohazards, some valuable academic fruits have been achieved through several scholar’s attempts (Huang et al., 2017; Zhao et al., 2018; Wu et al., 2022; Zhao et al., 2022). For the remote sensing images acquired by most of these techniques, the spatial and temporal resolution are not sufficient to monitor most slope deformation, and the usage of remote sensing still faces challenges in monitoring the steep mountainous slopes whose gradients are greater than 40° (Huang et al., 2017; Dong et al., 2022). For instance, when the direct georeferencing is adapted, large vertical errors often manifest in datasets with vertically oriented objects and extensive vertical landscape features (Nesbit et al., 2022). Owing to the low accuracy and resolution of the remote sensing images for the high steep slopes, the situations of false and missing identifications of potential reservoir geohazards could occur quite often in practice. Additionally, few attentions have been paid to the reservoir geohazards induced by RMD, not to mention the early recognition of this kind of geohazards.
In this paper, the bank slopes from Zigui County to Badong County in the TGRA were selected as the study objects to establish the generalized evolution modes of typical RMD-induced geohazards. By integrating the orthophotographic imaging, three-dimensional multi-angle oblique imaging, and high-precision digital elevation model (DEM), a new unmanned aerial vehicle (UAV) photographic approach was proposed for early identification of RMD-induced geohazards in the reservoir bank slopes. Its practical application in the field survey indicated the good reliability of the presented method.
2 Materials and methods
2.1 Main types of RMD in HFB in the three gorges reservoir area
After water storage in the Three Gorges Reservoir, the rock masses of HFBs on both banks of the main Yangtze River channel all show different degrees of deterioration. For the HFBs from Zigui County to Badong County, the rock masses of bank slopes with different lithology and structures significantly present different deterioration features under water-rock interaction, water erosion, periodic stress, and environmental load. Through field investigation and data analysis of actual cases depicted in the Figure 3, the RMD of HFBs with carbonate and clastic rocks in the study area mainly includes seven types, i.e., dissolution-suffosion, crack development-extension, mechanical erosion, loosening-spalling, erosion-abrasion, collapsing-block falling near structural planes, and weak strip interlayer denudation, referring to previous related research (Huang et al., 2019; Huang et al., 2020b; Yan et al., 2021).
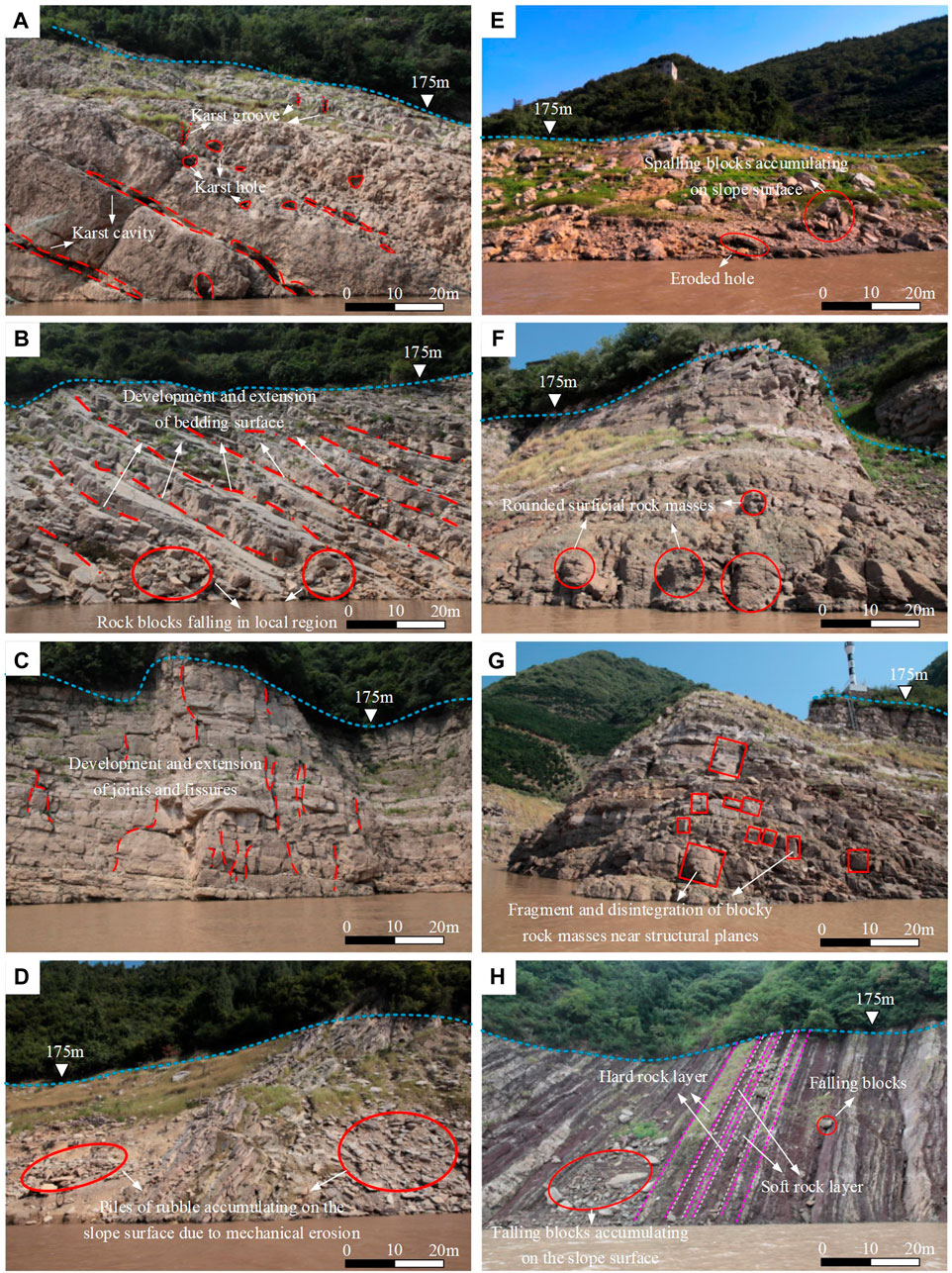
Figure 3. Typical cases of RMD occurred in the HFBs of the TGRA: (A) dissolution-suffosion (B, C) crack development-extension, (D) mechanical erosion, (E) loosening-spalling, (F) erosion-abrasion, (G) collapsing-block falling near structural planes, and (H) weak strip interlayer denudation.
2.1.1 RMD of carbonate rocks in HFB
2.1.1.1 Dissolution-suffosion
Starting at the beginning of water storage in the Three Gorges Reservoir, the reservoir water level has been fluctuating for a long period of time. The maximum single-day fluctuation amplitudes of the water level of the TGRA from 1 September 2008 to 1 September 2020 can be found in the relevant literature (Dai et al., 2022). The surface water and groundwater near the HFBs of the bank slopes are extremely active, which may lead to subsurface corrosion of the shallow carbonate rocks with joints and fissures. In a large number of gently-dipping stratified carbonate slopes, there are many karst holes, cavities, and grooves along the joints, fissures and bedding planes of rock masses in the HFBs. For example, due to corroded groves widely developed along the bank slopes of Zhenjiang Temple, the residual rock barriers between such corroded grooves are as thin as blades, as illustrated in the Figure 3A. The dissolution is a common karst phenomenon reflecting the process of chemical erosion of soluble rocks by water, which can reduce the integrity and quality of the surficial rock masses in the HFBs of the bank slopes. The solubility of rock, the permeability of rock, the corrosion ability of water, and the cyclic alternation of water level are the basic conditions for the development of karst (Wang and Yu, 2018; Huang et al., 2019; Yin et al., 2021). For the most of carbonate HFBs, the near-surface water has the characteristics of weak corrosion due to the dissolution of CO2 and acidic rainwater, and strong flowability due to the periodic adjustment of reservoir water level, which meets the aforementioned basic conditions required for dissolution. The dissolution process readily occurs in the karst areas of the HFBs of the bank slopes, especially in the structural planes with a little calcium cementation. When the calcareous cement or cementation layer filled in the structural planes and original karst holes is dissolved, the loose particles formed are easily carried away by flowing water, resulting in subsurface suffosion. Thus, this type of RMD can be regarded as the process of dissolution-suffosion.
2.1.1.2 Crack development-extension
For the bank slopes mainly composed of thin to medium layered limestone and dolomite in the study area, the structural planes of rock masses in the HFBs significantly develop, and the surficial bedding planes, joints and fissures gradually emerge and expand due to the periodic change in the reservoir water level, resulting in the deterioration phenomena that the shallow rock masses detach from the host rock by fracturing or falling. Through a detailed investigation on the development characteristics of structural planes within the HFBs, the consequent and oblique bedding slopes are characterized by the development and extension of bedding planes (Figure 3B), whereas the reverse and transverse bank slopes are mainly characterized by the development and extension of joints and fissures (Figure 3C). In addition, compared with the rock mass above the HFBs, the number of fissures within the HFB increases, and the crack width and trace length generally grow, indicating significant deterioration characteristics. The complicated mechanism for the crack development-expansion of rock masses is closely related to the environmental change of surface water and groundwater, the temperature stress, and the hydro-mechanical coupling effect. The jointed rock masses experience stress-corrosion cracking under the combined action of a high ground stress field and slightly corrosive water flow. That is, rock bridges or closed structural surfaces crack or break because their strengths decrease under the long-term stress effect and chemical corrosion. The stress-corrosion cracking generally enables the rock mass to break under a low-medium stress (compared to the normal failure stress state), which is a chemo-mechanical coupling effect.
2.1.1.3 Mechanical erosion
In the study area, small cavities emerge in the local region of weak layers or fissure belts when the fillings of discontinuities within the HFBs of carbonate bank slopes are eroded away by water flow. Then, under the continuous erosion of flowing water, the original joints and fissures in the rock masses further develop and extend towards the cavity. In addition, the mechanical erosion caused by water flow, rainfall, groundwater, and sand-carrying water may also alter the morphology of karst bank slopes (Figure 3D). The mechanical erosion is generally dominated by the impact force and drag force imposed on the surficial loose cataclastic rock masses by water flow, and the degree of erosion can be characterized by the annual erosion amount or erosion rate. In the HFBs of the carbonate bank slopes in the TGRA, the mechanical erosion enables many grooves and cavities to emerge within the rock masses, and eventually leads to a significant change in the original structure of shallow rock masses.
2.1.2 RMD of clastic rocks in HFB
2.1.2.1 Loosening-spalling
The clastic rocks in the HFBs of the bank slopes within the study area mainly include argillaceous sandstone and argillaceous shale. They are easy to soften and loosen in contact with water, and then peel off under the erosion of water flow and the gravity, causing the rock masses in the HFBs of bank slopes to loosen and spall. With this type of RMD, the bedrock outcrops peel off, and some rock fragments of various sizes, mainly red purple sandstone, remain on the bank slopes. Besides, there are visible eroded holes and Quaternary deposits in some parts, as illustrated in the Figure 3E. In summer, the temperature of the surficial rock masses in the HFBs can exceed 60°C, and the temperature difference in a day may be more than 25°C. Additionally, the periodic water level adjustment enables the rock masses to experience an immersion and air-drying cycle for a long period of time. The temperature stress generated on the surface and interior of rocks, and the expansion and contraction resulting from the water saturation-dehydration cycling make the surficial rock masses lamellar, sill-like, small block-shaped or scaly so that they crack, loosen, and peel off from the bank slopes.
2.1.2.2 Erosion-abrasion
There are many bank slopes with sandstone-argillaceous shale interbeddings in the study area. These rocks are easy to soften in contact with water, causing rock fragments to fall off and round under the action of water flow. Erosion-abrasion is characterized by the relatively clean slope surface with less debris, the rounded shallow rock masses dominated by red purple sandstone, the visible corroded holes and the Quaternary deposits in the local region (Figure 3F). The process of erosion-abrasion mainly refers to the combined action of impact and abrasion between the debris carried by water flows or waves and the bedrock surface. Therefore, when the rock masses in the HFBs have undergone erosion-abrasion, the slope surface is very clean, and parts of the shallow rock masses are rounded.
2.1.2.3 Collapsing-block falling near structural planes
The HFBs of sand-shale interbeddings within the study area readily hollows out under the action of water flow, resulting in the fragment and disintegration of medium layered sandstone along the original structural planes. As to this type of RMD, a large number of unloading fissures emerge on the bank slope surface, mainly consist of two group of primary structural planes. One group of structural planes are roughly parallel with the bank slope surface, while the other group is nearly perpendicular to the bank slope surface. Most of the rocks are red purple sandstone, and many of them hang on the slope surface, forming a “tofu-like” shape, and readily fall off (Figure 3G). During the process of collapsing-block falling near structural planes, after the shallow rock masses in the HFBs become loose and corroded, the soft rock layers are hollowed out, and the local concentrated stress in the rock masses causes the fissures to expand and further results in the cracking and disintegration of rock blocks.
2.1.2.4 Weak strip interlayer denudation
Many bank slopes in the study area are dominated by bedding structures, and the alternating soft and hard rock layers are exposed in the HFBs. In particular, the hard rocks are mainly brittle rocks, such as marl, calcareous sandstone, and feldspathic quartz sandstone. The soft rocks mainly include mudstone, siltstone, and shale that have a low strength. For these consequent bedding slopes, the slopes are generally steep, and red purple rock masses often alternate with grey green ones. The exposed rock masses in the HFBs are mainly the sandstone-shale interbeddings. As to the type of weak strip interlayer denudation, the shale as the interlayer of hard rocks firstly softens in water, then it is hollowed out and denuded under the erosion of water flow, causing the thin to medium-thick sandstone or limestone to fall off along the bedding planes. This type of RMD is characterized by the relatively clean slope surface with less debris, and much shale has become soil or soil-like (Figure 3H). The red-purple sandstone readily falls from the host rock when it is struck by a geological hammer. Generally, this type of RMD shows different degrees of erosion; the soft rocks tend to be loosened and eroded, while the hard rocks always experience cracking and disintegration.
2.2 Evolution modes for typical RMD-induced geohazards
According to field investigation and existing research fruits (Zhang P et al., 2021), there are several kinds of typical RMD-induced potential reservoir geohazards, including base-crushing, toe-emptying induced toppling, bedding sliding in carbonate bank slopes, and soft-hard interbedded rock collapse, apparent-dip wedge sliding, bedding sliding and reverse toppling in clastic bank slopes, as illustrated in the Figure 4. In combination with the previous descriptions on the types of RMD in the HFBs, the generalized evolution modes (with seven subcategories) for the potential RMD-induced reservoir geohazards in the TGRA can be established, as depicted in the Figure 5.
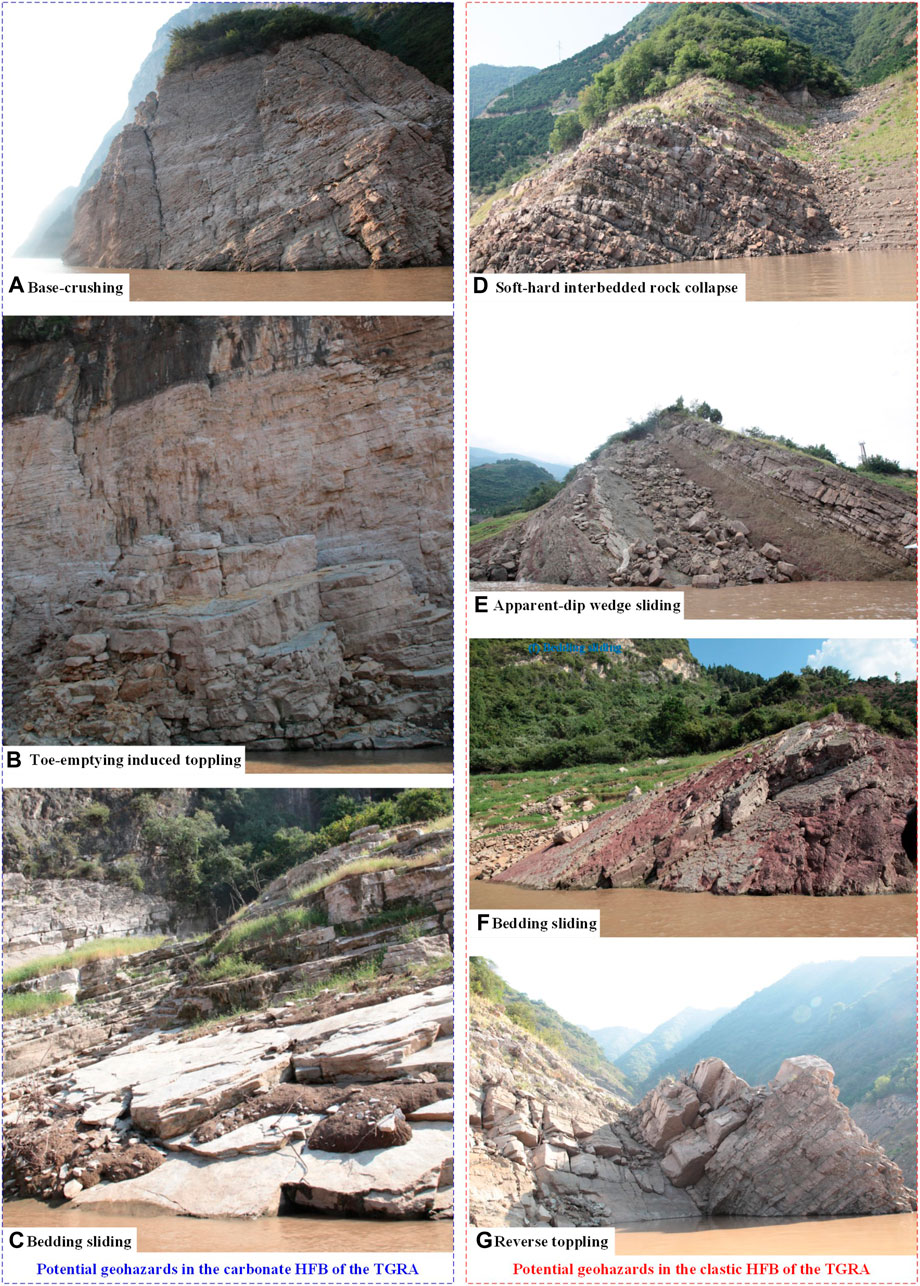
Figure 4. Typical cases of potential RMD-induced geohazards in the TGRA: (A) base-crushing; (B) toe-emptying induced toppling; (C) bedding sliding; (D) soft-hard interbedded rock collapse; (E) apparent-dip wedge sliding; (F) bedding sliding; (G) reverse topping.
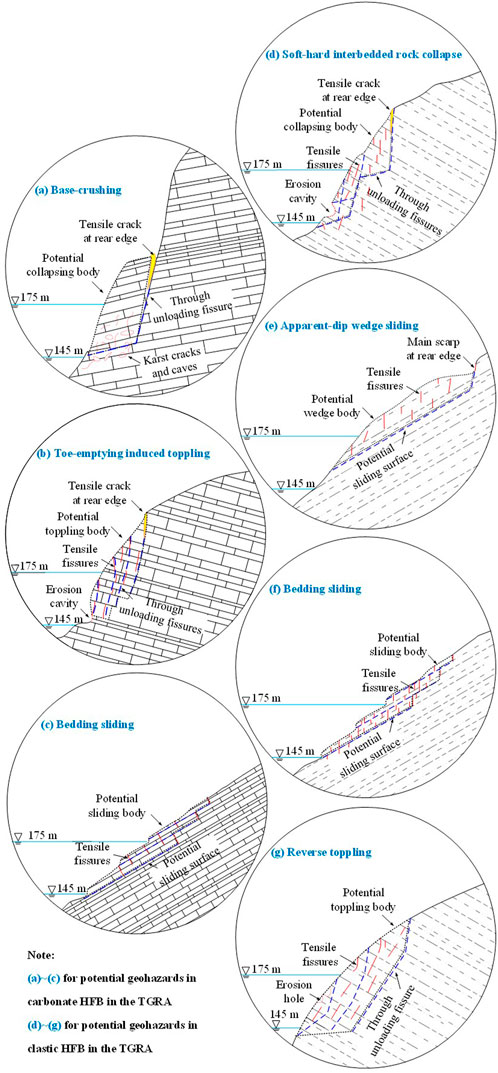
Figure 5. Generalized evolution modes for potential RMD-induced geohazards in the TGRA: (A–C) for potential geohazards in carbonate HFB in the TGRA; (D–G) for potential geohazards in clastic HFB in the TGRA.
2.2.1 Evolution modes for RMD-induced geohazards in carbonate bank slopes
2.2.1.1 Base-crushing
Bank slopes composed of carbonate rocks are susceptible to karst geological processes, such as the dissolution and suffosion. Under the long-term hydrodynamic environment and the periodic fluctuation of water level, the HFB of the carbonate bank slope, namely, the potential base of unfavorable geological body, can develop many karst ditches, grooves, cracks, and caves. When subjected to intense rainfall or other external forces, the upper rock masses continue to squeeze the base and gradually disintegrate along the corrosion cracks and unloading fissures, eventually resulting in the occurrence of geological disasters such as collapses or landslides. Figure 5A illustrates the schematic of this potential evolution mode, which usually emerges in the columnar dangerous rock masses with generally small development scales.
2.2.1.2 Toe-emptying induced toppling
Under the action of long-term mechanical erosion, such as the erosion and denudation of reservoir water, the relatively weak rock masses in the HFBs of counter-tilt carbonate bank slopes are exposed to a continuous process of drying-wetting cycling, softening, argillization and disintegration. The process contributes to the formation of erosion cracks and cavities with different shapes in the HFBs, which gradually empties the toe of the bank slope. Without the sufficient support, the unloading-induced tensile fractures begin to appear, toppling failure of the upper rock masses finally occurs. Figure 5B depicts the schematic for the potential evolution mode of toe-emptying induced toppling. This failure mode mainly exists in unstable counter-tilt bank slope and slightly occurs in dangerous rock mass, which is usually small-scaled and mostly dustpan-shaped.
2.2.1.3 Bedding sliding
Consequent and oblique bedding carbonate bank slopes are widely distributed in the study area. Under the long-term fluctuation of water level, the rock masses in the HFBs continually degrades owing to the erosion, scouring, dissolution and suffosion. The infillings of discontinuities are gradually washed away, and a great number of joint fissures perpendicular to the bedding surfaces emerge continuously in the HFBs due to stress adjustment, which finally cut the massive intact rock into discrete small blocks. Subsequently, the small rock blocks become unstable owing to their gravities and the wave erosion, and begin to slip towards the reservoir along bedding surfaces. The schematic diagram for the bedding sliding mode is provided in the Figure 5C.
2.2.2 Evolution modes for RMD-induced geohazards in clastic bank slopes
2.2.2.1 Soft-hard interbedded rock collapse
In the study area, there exists many bank slopes with interbeddings of soft and hard rocks, such as the sandstone interbedded with mudstone or shale. Under the action of long-term water-level fluctuation, the interlayered hard rocks fracture and fragment due to the softening and unloading of soft rocks in the HFBs. Subsequently, the joints and fissures continually extend, and finally form the persistent unloading fissure perpendicular to bedding planes. Once the deformation and instability of rock masses separated by highly developed fissures occur in a local steep area, the instable rock masses begin to collapse and slip towards the reservoir. This evolution mode is mainly found in steep zone of counter-tilt bank slopes with interbeddings of soft and hard rocks, and the Figure 5D depicts the corresponding schematic diagram.
2.2.2.2 Apparent-dip wedge sliding
Owing to the long-term hydro-fluctuation, the rock masses (siltstone and mudstone) in the HFBs of apparent-dip bank slopes deteriorate dramatically in the alternating dry-wet environment. After RMD of the anti-slide body at the front edge of the bank slope, a wedge-shaped sliding surface gradually appears in the rear edge, and then extends through the bank slope, which finally leads to the overall wedge sliding of potential unstable rock masses. This kind of rock slide is usually characterized by larger scale, higher starting speed and considerable run-out distance. For instance, the Kamenziwan landslide occurred in Xietan Town, Zigui County, China, on 10 December 2019, is in an apparent-dip wedge sliding mode (Yin et al., 2020a). The schematic diagram of this evolution mode is presented in the Figure 5E.
2.2.2.3 Bedding sliding
Due to the scouring, erosion and denudation effects of water on the HFBs of clastic bank slopes, the superficial completely and intensely weathered rock masses of consequent bedding slopes are washed away by water flow, causing the eroded and denudated bank slopes to retreat slowly. The shear strengths of interlayered or superficial weak layers decrease progressively, resulting that the shallow rock masses of HFBs become unstable and then slips towards reservoir along bedding surfaces. In this failure mode, the local and shallow rock masses slide firstly, and then cut the slope toe to form free faces, which might finally lead to a large-scale bedding sliding of upper rock masses. This is a typical evolution mode of newly formed clastic geohazard in the study area. Its schematic is given in the Figure 5F.
2.2.2.4 Reverse toppling
As a result of long-term water-level fluctuation, the clastic rock masses in the HFBs of reverse bank slopes are subjected to severe deterioration, and the persistent unloading fissures perpendicular to the bedding surface gradually develops, which splits intact rock into discrete blocks. Once the deformation and failure of discrete rock blocks emerges in a local steep area, the local unstable rock masses collapse and topple towards the reservoir. The reverse toppling mode mainly occurs in steep clastic bank slopes, as depicted in the Figure 5G.
2.3 Indices and method for identifying RMD-induced geohazards
2.3.1 Identifying indices for RMD-induced potential geohazards in HFB
This study focuses on the potential geohazards triggered by the RMD of carbonate and clastic HFBs. The proposed evolution modes of geohazards in HFBs are not only influenced by the types of RMD, but also related to other external and internal factors. Thus, the determination of interpretation indices for different evolution modes of RMD-induced geohazards is crucial to identifying potential hazardous sites. With regard to the establishment of the indices of RMD, there are currently no relevant international or national standards available, because the research topic of the RMD of HFBs in the TGRA is somewhat controversial and still in the ascendant. Thus, this paper proposes the qualitative indices of RMD through analysing and summarising the results of the field investigation with reference to the existing research fruits (Huang et al., 2019; Huang et al., 2020a; Huang et al., 2020b; Huang et al., 2021; Yan et al., 2020). The identification process is a technical mean to obtain relevant information on potential geohazards using the human-machine interactive visual interpretation. The principle of this method is to recognize differences in color tone, shape, shadow, and texture between potential geohazard sites and their background geological bodies. Meanwhile, the types and characteristics of the RMD in the HFBs are also fully considered in the above identification process. In general, interpretation indices refer to the image characteristics contributing to the recognition of the existence, attribute and mutual relationship of the target objects or phenomena, which can be divided into direct and indirect indices. For example, the type of RMD and the development feature of structural plane are regarded as direct interpretation indices. Indirect interpretation indices reflect the properties of a certain object or natural phenomenon, which are internal relations, e.g., the landslide landform, the structure of bank slope, the type of rock strata, the vegetation development, etc. Herein, the interpretation indices of RMD-induced reservoir geohazards are selected based on the consideration of the extrinsic factors triggering the instability, the intrinsic factors controlling the failure mode, and the visible signs reflecting the evolution process of geohazards. Specifically, the triggering factor of reservoir geohazards is water-induced RMD, which can be indirectly reflected by the development of vegetation and directly recognized by the enhancement of macroscopic defects of rock masses. The controlling factors mainly consist of the geomorphological feature, the structure of bank slope, the type of RMD related to the lithology and the structure of rock masses, and the feature of structural plane. The visible signs for the evolution state of potential reservoir geohazards which can be captured by the photographic method are boundary characteristics. The specific interpretation indices for RMD-induced reservoir geohazards in the HFBs and the corresponding descriptions are given in the Supplementary Table SA1.
2.3.2 Identification method of RMD-induced potential geohazards
In order to identify the RMD-induced reservoir geohazards by photographic approach, the three-dimensional high-precision DEM of the HFBs is firstly established based on the aerial images obtained by the UAV photography. The raw image data is imported into the Pix4Dmapper to check out the integrity and the light-shade difference of successive images, and whether the overlapping area of the adjacent images is sufficient. The report for the fast processing of the raw data can be used to verify its reliability and high quality. After the raw data meet the quality requirement, the three-dimensional data set can be obtained by aerial triangulation. Then, the original aerial photos are imported into the software smart3D to reproduce the position of every shots according to the longitude, latitude, height and angle of every photos. The relationships among points are established via the smart3D, and the recognized points are included in the intensive computation to generate the greyscale point cloud. By utilizing the internal algorithm for vector function in the smart3D, the point cloud data is transformed into the irregular triangulation network, and further to build the three-dimensional model composed of points, line, and surfaces. The depth maps with different perspectives are matched in a same coordinate system to generate the complete geometric model of the HFBs by image fusion. Later, the mapping relationship between the depth maps and the texture maps is determined, the whole texture mapping map is obtained by texture fusion based on a mixed weight. Finally, the real high-precision three-dimensional model of the HFBs is constructed by texture mapping. After that, the RMD-induced potential geohazards can be identified according to the established evolution modes considering main interpretation indices. The identification methodology is outlined in the Figure 6.
Specifically, in combination with ArcGIS geographic information system software, ENVI remote sensing software is utilized to process and analyze geospatial imagery obtained by the UAV photography. This image processing consists mostly of geometric correction, ortho-rectification, and image mosaic. At the same time, the DEM is used to classify the inclinations of bank slopes to improve the reliability of interpretation, and to recognize the interpretation indices of potential geohazards according to topography, geomorphology and image characteristic. The above process yields the identification and interpretation results, which includes basic characteristics of potential geohazards, such as morphology, scale, range and distribution.
2.4 Recognition of potential RMD-induced geohazards and corresponding evolution modes
For the RMD-induce geohazards in the TGRA, the phenomena of RMD within the HFBs are very common, and the geomorphological characteristic of bank slopes and valleys along the Yangtze River are basically the same. Thus, the interpretation indices of the lithology, rock mass structure, RMD’s type, bank slope structure, feature of structural plane, and boundary characteristics given in the Supplementary Table SA1 are sufficient to establish a discrimination system for recognition of the potential RMD-induced reservoir geohazards with different evolution modes, as depicted in the Figure 7. Specifically, for the identification of RMD-induced geohazards in the investigated area, the determination of the type of RMD according to the lithology of the HFB is the first step. Then, it is necessary to judge whether the bank slope has a trend of overall deformation or displacement by comprehensively considering the degree of RMD, bank slope structure, rock mass structure, feature of structural plane and boundary characteristics. Eventually, the potential evolution mode of the identified RMD-induced geohazard also can be ascertained based on the generalized models in the Figure 5.
2.4.1 Discrimination conditions for potential RMD-induced geohazards in carbonate bank slopes
As depicted in the Figure 7, the discrimination conditions for three typical evolution modes of potential RMD-induced reservoir geohazards in carbonate HFBs are as follows.
2.4.1.1 Base-crushing
The dissolution-suffosion and crack development-extension are the main types of RMD. The large or controlling structural planes have extended into the boundaries of the potential geohazard. The bank slope structures are mainly the reverse or gentle stratified slopes, and the lithology is carbonate rock, e.g., limestone, dolomitic limestone, and dolomite. Most rock masses are characterized by the columnar shape of intact blocky structure, layered structure, fractured structure, loose structure, or soft-hard interbedded structure. There is little or no vegetation coverage, and the boundaries of potential dangerous rock masses are preliminarily formed.
2.4.1.2 Toe-emptying induced toppling
The crack development-extension and mechanical erosion are the main types of RMD. The large, steeply out-dipping or controlling structural planes are developed. Most slopes are in reverse or gentle stratified structures, and the lithology is hard carbonate rock, such as limestone, dolomitic limestone and dolomite. Most deteriorated rock masses of HFBs are in fractured or loose structures, and the overlying rock masses are mostly in blocky or layered structures. There is little or no vegetation, and the boundaries of potential dangerous rock masses have preliminarily taken shape.
2.4.1.3 Bedding sliding
The dissolution-suffosion, crack development-extension and mechanical erosion are the main types of RMD. Most bank slopes are in consequent or oblique dipping structures, and the lithology is hard carbonate rock, such as limestone, dolomitic limestone and dolomite. Most rock masses are in intact blocky structure, layered structure or fractured structure. The bedding planes, soft interlayers or relatively soft layers are quite developed. There is little or no vegetation, and the boundaries of potential landslide hazards have preliminarily taken shape.
2.4.2 Discrimination conditions for potential RMD-induced geohazards in clastic bank slopes
Similarly, the conditions for discriminating four typical evolution modes of potential RMD-induced reservoir geohazards in clastic HFBs are as follows (see the Figure 7):
2.4.2.1 Soft-hard interbedded rock collapse
The weak strip interlayer denudation is the major type of RMD in the HFBs, and random structural planes are developed. The slopes are mainly in consequent bedding, oblique bedding or reverse structures, and the lithology is clastic rock, such as interbedded sandstone-mudstone and sandstone interbedded with mud shale. Most of rock masses are in soft-hard interbedded layered structure. There is little or no vegetation, and the boundaries of potential landslide hazards have preliminarily taken shape.
2.4.2.2 Apparent-dip wedge sliding
The erosion-abrasion and collapsing-block falling near structural planes are the main types of RMD. The large or controlling structural planes are developed. The slopes were mainly in consequent bedding and oblique bedding structures, and the lithology is clastic rock, such as sandstone, mudstone, shale, interbedded sandstone-mudstone or sandstone interbedded with mud shale. Most of rock masses are in layered structure with soft interlayers or mudstone layers occasionally. The vegetation is not developed well, and there are preliminary boundaries of the potentially landslide hazards.
2.4.2.3 Bedding sliding
The main types of RMD, development situation of large or controlling structural planes, structure of bank slopes and the lithology for bedding slide mode are the same as those for the apparent-dip wedge sliding mode. As to the structure of rock masses, layered structure with soft interlayers are fairly common. Additionally, the vegetation is not developed well, and the boundaries of potential landslide hazards have started to form.
2.4.2.4 Reverse toppling
The weak strip interlayer denudation, loosening-spalling and collapsing-block falling near structural planes are the main types of RMD. The large and medium-sized structural planes, or controlling structural planes are developed. Most bank slopes are of the reverse structure, and the lithology is clastic rock, such as sandstone, mudstone, shale, interbedded sandstone-mudstone or sandstone interbedded with mud shale. Most rock masses are in layered or fractured structures. The vegetation is not developed well, and the boundaries of potentially landslide hazards have started to take shape.
3 Results
3.1 Potential geohazards in the study area by the proposed method
Using the presented photographic interpretation method, the spatial coordinates of potential RMD-induced geohazards emerged in the HFBs of the study area were determined according to the UAV aerial images. It should be clarified that the determination of the RMD’s type and range was a prerequisite for the recognition of potential RMD-induced reservoir geohazards in this study. As the detailed field survey indicated that the RMD of same type usually emerged with a certain range, the lines with different colors were selected to divide the HFBs into small segments, whose lengths represented the ranges of the RMD with a specific type. Besides, the ranges of soil bank slopes and treated bank slopes were also delineated in the figures of interpretation results. The long-term effect of water level changes on the soil bank slopes might also lead to reservoir geohazards, but this kind of geohazards were not the concern of this study. As illustrated in the Figure 8, a total of 116 geohazards were identified, including 64 sites on the left bank and 52 sites on the right bank, respectively. And the development characteristics of each reservoir geohazard site were listed in the Supplementary Table SB1.
Additionally, the remote sensing software ENVI 5.3 and the geographic information system software ArcGIS 10.2 were combined to process and analyze the high-precision UAV orthophotographic images, and the boundaries of potential reservoir geohazards were obtained through the human-machine interactive visual interpretation, as depicted in the Figure 9. The development scale and area were also given in the Supplementary Table SB1.
3.2 Field verification of interpretation results
In an effort to assess the reliability, quality, and confidence of human-machine interactive visual interpretation outcomes, a field survey was conducted. The survey involved three primary tasks: verifying interpretation indices for various geohazards, conducting field surveys in key areas with unclear attributes, and performing field verifications for randomly selected geohazard sites to evaluate interpretation reliability. The survey methodology entailed route-based observations and fixed-point inspections, focusing on geohazards in key areas, main towns, and along major traffic arteries. Observations were recorded through detailed descriptions and photographic documentation, followed by a comparison with preliminary interpretation results.
The survey findings revealed that the identified potential geohazards on both banks were consistent with existing geohazard sites, demonstrating the effectiveness of the proposed photographic method in avoiding false and missing recognitions of potential RMD-induced reservoir geohazards, given the current state of knowledge and technology. Furthermore, the interpretation results pertaining to landslide types, scales, and morphologies were found to be reasonable. A comprehensive overview of the verification results can be found in Supplementary Table SB1.
3.3 Case study of field verified RMD-induced geohazard
Among those verified RMD-induced reservoir geohazards in the Supplementary Table SB1, the Yangjiaping landslide (Figure 10) is a typical soft-hard interbedded rock collapse. The Yangjiaping landslide lies in the Xietan Town, Zuigui County, Hubei Province, China, located on the north bank of the Yangtze River and the left bank of the Xietan River, is about 55 km away from the Three Gorge Dam. This landslide has a dustpan shape on the plane, as shown in the aerial picture (Figure 10). The front and back widths of the landslide are approximately 130 m and 135 m, respectively. The elevations of the front and rear edges of the landslide are 145 m and 194 m, respectively. The area and volume of the landslide are about 2.6 × 104 m2 and 40 × 104 m3, respectively. The neighbouring landforms are mainly the reverse bank slopes generally inclined towards the north direction, the gradients of natural bank slopes vary between 15° and 25°, and the attitude of stratum is 50°∠35°. The Yangjiaping landslide and its surrounding gentle slopes are mostly covered by the landslide accumulation layer (Qdel). The bedrock outcrops are common on the scarps, and the bedrock is mainly the clastic rock of the Upper Triassic Shazhenxi Formation (T3s) and the Lower Jurassic Xiangxi Formation (J1x). The rock masses of the Yangjiaping landslide are soft-hard interbedded, and mainly consist of hard sandstone and soft silty mudstone, as depicted in the Figure 11.
After the impoundment of the Three Gorge Reservoir, the HFB of the Yangjiaping landslide has been undergoing the long-term effect of water level change. Under the combined action of the water level fluctuation and wetting-drying cycles, the porosity or pores of soaked rocks increase and the dissolution of hydrophilic mineral components enhances, which results in the softening and disintegration of rocks. Then, the surficial fragmental rocks and the filling materials of discontinuities are washed away by the scour and erosion of flowing water. The interlayered soft silty mudstone are no longer able to support the overlying rock masses, and the overlying stratified hard sandstone begin to bend downwards under the unloading and gravity. The stress concentration near the slope surface results in the formation of tension fracture and the intensive extension of unloading fissures. Accompanying with the fracture and fragment of layered rock masses, the surficial rock masses lose the original structural integrity and collapse to form a zone of loose rock fragments with a certain depth. Currently, due to repetition of the previous process, a through step-shaped potential sliding surface has formed along the unloading fissures, and the tension crack at the rear edge of the Yangjiaping landslide is filled with the rock fragments (Figure 11). It should also be noted that the local failure mode of the Yangjiaping landslide could be the planar rupture on the polygonal sliding plane, which can be formed by the tension cracks and unloading fissures.
4 Discussions
In order to clearly clarify the gaps between the existing remote sensing methods and the proposed photographic method in this study for recognition of reservoir geohazards, the interferomertric synthetic aperture radar, as one of the typical remote sensing techniques, was selected for the comparative analysis with the presented photographic approach. The detailed comparative chart is depicted in the Figure 12. On the whole, these two methods both need to establish the high-precision three-dimensional terrain models of the study areas by integrating the DEM and the collected image data, and adopt the deformation or displacement indices of ground surface to identify potential reservoir geohazards. The main difference between these two methods is that the presented photographic method needs to zone the investigated area according to the types of RMD, and further to identify the potential RMD-induced reservoir geohazards according to the generalized evolution modes of typical cases. Moreover, the photographic approach developed in this study is objective-based, its usage is limited to the refined identification of RMD-induced reservoir geohazards.
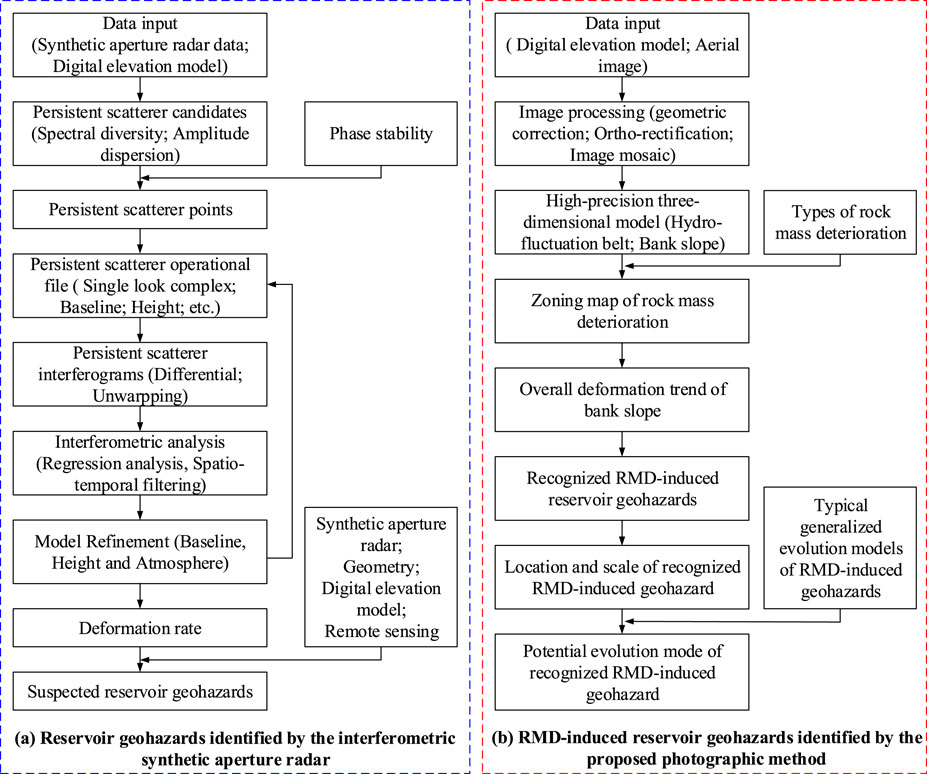
Figure 12. Comparative chart for the interferometric synthetic aperture radar and the proposed method.
Essentially, the RMD of HFBs in reservoir bank slopes is a long-term water-rock interaction process, which results in the continuous degradation of rock mass quality, namely, the loss of structural integrity and the decline of strength indices. Though the mechanisms of rock mass degradation under the periodic water-level fluctuation, such as one of the hydraulic, mechanical, thermal, and chemical effect, or the coupling of several aforementioned effects (Liu et al., 2008; Liu et al., 2009; Liu et al., 2018; Deng et al., 2012a; Deng et al., 2012b; Sun and Zhang, 2019; Liu et al., 2018; Yao et al., 2020; Wang et al., 2021; Zhou et al., 2021; Gu et al., 2021), are complicated and somewhat controversial, the consequences could be described by the degree of RMD, e.g., the rock mass degradation variable (Huang et al., 2021) or the damage deterioration variable (Yin et al., 2020a), and ultimately the potential geohazards induced by RMD proposed in this study (Figure 5). Thus, the evolution of the RMD-induced geohazard could be regarded as a dynamic catastrophic process, which is not only relevant to the type of RMD, but also highly depends on the degree of RMD. Though the novel method in this paper had an excellent performance in recognition of the RMD-induced geohazards in reservoir bank slopes, the accurate identification is far from sufficient to control the risk effectively. Since the established discrimination system only considers the type of RMD, the current state and development trend of the identified potential geohazards relied on the qualitative description of the type of RMD are not very clear, not to mention the risk of the identified potential geohazards. Hence, it is necessary to simultaneously consider the type and degree of RMD on the basis of the proposed method in future. For instance, by defining an index to quantitatively describe the degree of RMD, the critical threshold of this index suggesting the transition from stability to instability of rock masses can be useful to judge whether a bank slope with RMD is in the risk of failure. Further, the aforementioned index also can be used in the risk assessment of RMD-induced geohazards adopting the conventional risk based framework, which begins with analysis of hazard and consequence, continues with risk estimation, and ends by risk evaluation. As to the risk analysis phase, after the identification of the instability mechanism of RMD-induced geohazards according to the generalized evolution modes presented in this study, the spatial and temporal occurrence (in term of probability) of RMD-induced geohazards and their intensity can be quantified based on the kinetic analysis of potential unstable rock masses, which accounts for the effects of RMD on critical influencing factors (such as the structure, strength and deformation parameters of rock masses) via the quantitative relationships between the index of the degree of RMD and other critical parameters. Since the predisposing factors, such as the degree of RMD and RMD-related indices, change over time, the time-dependent susceptibility or risk of RMD-induced reservoir geohazards also can be determined. For the administration departments related with disaster mitigation and prevention, the above work will contribute to determining the order of priority when the risk control measures or treatments of some potential geohazards, such as multi-row stabilizing piles (Pei et al., 2022; Zhang et al., 2022; Zhang et al., 2023), are about to be implemented.
To guarantee the reliability of the proposed photographic approach in recognizing the potential RMD-induced geohazards, the sufficient precision of original images of reservoir bank slopes acquired by the UAV photography is crucial. Considering the complicated and volatile topography and landforms of alpine valley regions, preplans for the emergency situations such as miserable weather and signal interference should be prepared in advance when performing the UAV photography. In addition, the trial flights are essential to determining the preferable flight line, flight altitude, flight sorties, the degrees of forward overlap and side overlap. Herein, the software pix4Dmapper can be used to check out the integrity and the light-shade difference of successive images, and whether the overlapping area of the adjacent flights is sufficient. After the fast processing of three-dimensional point cloud data obtained by the UAV photography, the reliability of the data can be confirmed through the quality report yielded by the previous software. Meanwhile, the three-dimensional point cloud data is imported into the software pix4d to generate the data sets, and then to obtain the stereo data of three-dimensional model established by the software smart3D. To satisfy the precision requirement of orthophoto, the method of distinct block processing is selected to correct aerial photograph in the software pix4d. However, due to the extremely high pixels of synthetic image, the ordinary equipment is unable to handle the great number of primitive data without the help of particular serves. Therefore, the primitive data in this study was divided into suitable groups to generate the orthophoto by group, in which the number of the blocks ranged from 10 to 20 owing to the different data sizes of the blocks. The above procedure for generations of orthophoto and oblique real scene three-dimensional model is usually time-consuming when meticulous geological mappings of potential reservoir geohazards are required. Thus, in terms of practicability of the proposed photographic method, future research on the technology for improving the data processing efficiency or the strategy for balancing the efficiency and the precision remains to be carried out.
5 Conclusion
In this study, a photographic method for recognition of the RMD-induced potential geohazards in reservoir bank slopes was presented and verified. The following conclusions can be drawn:
(1) Typical evolution modes of the RMD-induced potential geohazards in the HFBs were proposed based on field surveys. The evolution modes in carbonate HFBs include base-crushing, toe-emptying induced toppling and bedding sliding, while the evolution modes in clastic HFBs include soft-hard interbedded rock collapse, apparent-dip wedge sliding, bedding sliding and reverse toppling.
(2) In terms of the carbonate HFBs, base-crushing mode mainly occurs in dangerous rock masses; toe-emptying induced toppling mode generally emerges in unstable bank slopes and slightly distributes in dangerous rock masses, most of which are small-scaled and dustpan-shaped. Additionally, unstable consequent bedding slopes can easily evolve into rock slides with bedding sliding mode. As to the clastic HFBs, the development scales of most RMD-induced potential geohazards are relatively small. Soft-hard interbedded rock collapse mode is usually manifested by the slump of potential unstable clastic slopes. Most of geohazards with apparent-dip wedge sliding, bedding sliding and reverse toppling modes mainly appear in the form of rock slide and collapse.
(3) A total of 116 potential RMD-induced reservoir geohazards as well as their boundaries, areas, and scales have been identified by the proposed photographic method. The field survey results have confirmed the effectiveness of the proposed photographic method in avoiding the false and missing recognitions of the potential RMD-induced reservoir geohazards at current levels of knowledge and technology.
We expect that this paper will contribute a reliable and efficient approach to the areas of geohazard identification and reservoir management.
Data availability statement
The original contributions presented in the study are included in the article/Supplementary Material, further inquiries can be directed to the corresponding author.
Author contributions
ZD: Writing–original draft, Writing–review and editing, Writing–original draft, Writing–review and editing, Writing–original draft, Writing–review and editing, YZ: Formal Analysis, Investigation, Methodology, Writing–review and editing, Formal Analysis, Investigation, Methodology, Writing–review and editing, Formal Analysis, Investigation, Methodology, Writing–review and editing, CZ: Conceptualization, Investigation, Methodology, Writing–original draft, Writing–review and editing, Conceptualization, Investigation, Methodology, Writing–original draft, Writing–review and editing, Conceptualization, Investigation, Methodology, Writing–original draft, Writing–review and editing, XF: Conceptualization, Investigation, Methodology, Writing–review and editing, Conceptualization, Investigation, Methodology, Writing–review and editing, Conceptualization, Investigation, Methodology, Writing–review and editing, PZ: Data curation, Formal Analysis, Methodology, Validation, Writing–original draft, Data curation, Formal Analysis, Methodology, Validation, Writing–original draft, Data curation, Formal Analysis, Methodology, Validation, Writing–original draft, RY: Data curation, Investigation, Methodology, Software, Supervision, Writing–original draft, Data curation, Investigation, Methodology, Software, Supervision, Writing–original draft, Data curation, Investigation, Methodology, Software, Supervision, Writing–original draft.
Funding
The author(s) declare financial support was received for the research, authorship, and/or publication of this article. This work was supported by a follow-up of the Geological Disaster Prevention and Control Project in the Three Gorges area (Grant Nos 000121 2024C C60 001 and 000121 2023C C60 001), Qianlong Plan Top Talent Project of Wuhan Center of China Geological Survey (Grant No. QL 2022-06).
Conflict of interest
The authors declare that the research was conducted in the absence of any commercial or financial relationships that could be construed as a potential conflict of interest.
The reviewer FG declared a shared affiliation with the author PZ to the handling editor at time of review.
Publisher’s note
All claims expressed in this article are solely those of the authors and do not necessarily represent those of their affiliated organizations, or those of the publisher, the editors and the reviewers. Any product that may be evaluated in this article, or claim that may be made by its manufacturer, is not guaranteed or endorsed by the publisher.
Supplementary material
The Supplementary Material for this article can be found online at: https://www.frontiersin.org/articles/10.3389/feart.2024.1365272/full#supplementary-material
References
Abellán, A., Oppikofer, T., Jaboyedoff, M., Rosser, N. J., Lim, M., and Lato, M. J. (2014). Terrestrial laser scanning of rock slope instabilities. Earth Surf. Proc. Land 39 (1), 80–97. doi:10.1002/esp.3493
Carlà, T., Intrieri, E., Raspini, F., Bardi, F., Farina, P., Ferretti, A., et al. (2019). Perspectives on the prediction of catastrophic slope failures from satellite InSAR. Sci. Rep. 9, 14137. doi:10.1038/s41598-019-50792-y
Dai, Z. W., Zhang, Y. J., Zhang, C. Y., Luo, J. H., and Wang, Y. (2022). Interpreting the influence of reservoir water level fluctuation on the seepage and stability of an ancient landslide in the three gorges reservoir area: a case study of the outang landslide. Geotech. Geol. Eng. 40, 4551–4561. doi:10.1007/s10706-022-02170-1
Deng, H. F., Li, J. L., Liu, J., Zhu, M., Luo, J., and Yuan, X. F. (2012a). Influence of immersion-air dry circulation function on deformation and fracture features of sandstone (in Chinese). Chin. J. Geotech. Eng. 34, 66–72.
Deng, H. F., Li, J. L., Zhu, M., Wang, K. W., Wang, Y. H., and Deng, C. J. (2012b). Experimental research on strength deterioration rules of sandstone under “saturation-air dry” circulation function (in Chinese). Rock Soil Mech. 33, 3306–3312.
Dong, J., Zhang, L., Tang, M. G., Liao, M. S., Xu, Q., Gong, J. Y., et al. (2018). Mapping landslide surface displacements with time series SAR interferometry by combining persistent and distributed scatterers: a case study of Jiaju landslide in Danba, China. Remote Sens. Environ. 205, 180–198. doi:10.1016/j.rse.2017.11.022
Dong, X. J., Yin, T., Dai, K. R., Pirasteh, S., Zhuo, G. C., Li, Z. Y., et al. (2022). Identifying potential landslides on giant niexia slope (China) based on integrated multi-remote sensing technologies. Remote Sens. 14 (24), 6328. doi:10.3390/rs14246328
Gu, D. M., Liu, H. L., Gao, X. C., Huang, D., and Zhang, W. G. (2021). Influence of cyclic wetting–drying on the shear strength of limestone with a soft interlayer. Rock Mech. Rock Eng. 54, 4369–4378. doi:10.1007/s00603-021-02502-2
Hervás, J., Barredo, J. I., Rosin, P. L., Pasuto, A., Mantovani, F., and Silvano, S. (2003). Monitoring landslides from optical remotely sensed imagery: the case history of Tessina landslide, Italy. Italy. Geomorphol. 54 (1/2), 63–75. doi:10.1016/s0169-555x(03)00056-4
Huang, B. L., Wang, J., Yin, Y. P., Zhu, S. N., and Dai, Z. W. (2020a). Spatial distribution analysis of degraded karst rock mass in fluctuation zone based on fracture network (in Chinese). Chin. J. Undergr. Sp. Eng. 16, 1901–1908.
Huang, B. L., Yin, Y. P., Li, B., Qin, Z., and Zhang, P. (2020b). Rock mass deterioration and its catastrophic effect of karst bank slope in Three Gorges project reservoir area (in Chinese). Hydrogeol. Eng. Geol. 47, 11. doi:10.16030/j.cnki.issn.1000-3665.202003055
Huang, B. L., Yin, Y. P., Yan, G. Q., Li, B., Qin, Z., and Wang, J. (2021). A study on in situ measurements of carbonate rock mass degradation in the water-level fluctuation zone of the Three Gorges Reservoir, China. Bull. Eng. Geol. Environ. 80, 1091–1101. doi:10.1007/s10064-020-01990-w
Huang, B. L., Yin, Y. P., Zhang, Z. H., Wang, J., Qin, Z., and Yan, G. Q. (2019). Study on deterioration characteristics of shallow rock mass in water the level fluctuation zone of karst bank slopes in Three Gorges Reservoir area (in Chinese). Chin. J. Rock Mech. Eng. 38 (09), 1786–1796. doi:10.13722/j.cnki.jrme.2018.1535
Huang, D., Gu, D. M., Song, Y. X., Cen, D. F., and Zeng, B. (2018). Towards a complete understanding of the triggering mechanism of a large reactivated landslide in the Three Gorges Reservoir. Eng. Geol. 238, 36–51. doi:10.1016/j.enggeo.2018.03.008
Huang, H. F., Long, J. J., Lin, H. Y., Zhang, L., Wu, Y., and Lei, B. J. (2017). Unmanned aerial vehicle based remote sensing method for monitoring a steep mountainous slope in the Three Gorges Reservoir, China. Earth Sci. Inf. 10, 287–301. doi:10.1007/s12145-017-0291-9
Kang, J. T., Wu, Q., Tang, H. M., Hu, X. L., Fan, L. L., Zhang, S., et al. (2019). Strength degradation mechanism of soft and hard interbedded rock masses of Badong formation caused by rock/discontinuity degradation (in Chinese). Earth Sci. 44, 3950–3960. doi:10.3799/dqkx.2019.110
Liu, X. R., Fu, Y., Wang, Y. X., Huang, L. W., and Qin, X. Y. (2008). Deterioration rules of shear strength of sand rock under water-rock interaction of reservoir (in Chinese). Chin. J. Geotech. Eng. 30, 1298–1302.
Liu, X. R., Fu, Y., Wang, Y. X., Huang, L. W., and Qin, X. Y. (2009). Stability of reservoir bank slope under water-rock interaction (in Chinese). Rock Soil Mech. 30, 613–616.
Liu, X. R., Jin, M. H., Li, D. L., and Zhang, L. (2018). Strength deterioration of a Shaly sandstone under dry-wet cycles: a case study from the Three Gorges Reservoir in China. Bull. Eng. Geol. Environ. 77, 1607–1621. doi:10.1007/s10064-017-1107-3
Liu, X. R., Jing, R., Miao, L. L., Han, Y. F., Deng, Z. Y., and Xiong, C. (2020). Reconstruction models and typical case analysis of the fluctuation belt of reservoir bank slopes in Wushan (in Chinese). Chin. J. Rock Mech. Eng. 39, 1321–1332. doi:10.13722/j.cnki.jrme.2019.0887
Macciotta, R., and Hendry, M. T. (2021). Remote sensing applications for landslide monitoring and investigation in western Canada. Remote Sens. 13, 366. doi:10.3390/rs13030366
Nesbit, P. R., Hubbard, S. M., and Hugenholtz, C. H. (2022). Direct georeferencing UAV-SfM in high-relief topography: accuracy assessment and alternative ground control strategies along steep inaccessible rock slopes. Remote Sens. 14 (3), 490. doi:10.3390/rs14030490
Oppikofer, T., Jaboyedoff, M., Blikra, L., Derron, M. H., and Metzger R, R. (2009). Characterization and monitoring of the åknes rockslide using terrestrial laser scanning. Nat. Hazards Earth Syst. Sci. 9 (3), 1003–1019. doi:10.5194/nhess-9-1003-2009
Pei, Z. W., Zhang, Y. J., Nian, T. K., Song, X. L., and Zhao, W. (2022). Performance investigation of micropile groups in stabilizing unstable talus slopes via centrifuge model tests. Can. Geotech. J. 60, 351–365. doi:10.1139/cgj-2021-0681
Sun, Q., Zhang, L., Ding, X. L., Hu, J., Li, Z. W., and Zhu, J. J. (2015). Slope deformation prior to Zhouqu, China landslide from InSAR time series analysis. Remote Sens. Environ. 156, 45–57. doi:10.1016/j.rse.2014.09.029
Sun, Q., and Zhang, Y. L. (2019). Combined effects of salt, cyclic wetting and drying cycles on the physical and mechanical properties of sandstone. Eng. Geol. 248, 70–79. doi:10.1016/j.enggeo.2018.11.009
Tang, H. M., Wasowski, J., and Juang, C. H. (2019). Geohazards in the three Gorges Reservoir Area, China-Lessons learned from decades of research. Eng. Geol. 261, 105267. doi:10.1016/j.enggeo.2019.105267
Thirugnanam, H., Uhlemann, S., Reghunadh, R., Ramesh, M. V., and Rangan, P. V. (2022). Review of landslide monitoring techniques with IoT integration opportunities. IEEE J. Sel. Top. Appl. Earth Observations Remote Sens. 15, 5317–5338. doi:10.1109/JSTARS.2022.3183684
Tofani, V., Segoni, S., Agostini, A., Catani, F., and Casagli, N. (2013). Technical Note: use of remote sensing for landslide studies in Europe. Nat. Hazards Earth Syst. Sci. 13 (2), 299–309. doi:10.5194/nhess-13-299-2013
Wang, J. X., and Yu, Q. C. (2018). Experimental investigations of the process of carbonate fracture dissolution enlargement under reservoir temperature and pressure conditions. Geofluids 2018, 1–19. doi:10.1155/2018/5971421
Wang, L. Q., Yin, Y. P., Huang, B. L., and Dai, Z. W. (2020a). Damage evolution and stability analysis of the Jianchuandong dangerous rock mass in the three gorges reservoir area. Eng. Geol. 265, 105439. doi:10.1016/j.enggeo.2019.105439
Wang, L. Q., Yin, Y. P., Zhou, C. Y., Huang, B. L., and Wang, W. P. (2020b). Damage evolution of hydraulically coupled Jianchuandong dangerous rock mass. Landslides 17, 1083–1090. doi:10.1007/s10346-020-01350-5
Wang, Q., Hu, X. L., Zheng, W. B., Li, L. X., Zhou, C., Ying, C. Y., et al. (2021). Mechanical properties and permeability evolution of red sandstone subjected to hydro-mechanical coupling: experiment and discrete element modelling. Rock Mech. Rock Eng. 54, 2405–2423. doi:10.1007/s00603-021-02396-0
Wasowski, J., and Bovenga, F. (2014). Investigating landslides and unstable slopes with satellite Multi Temporal Interferometry: current issues and future perspectives. Eng. Geol. 174, 103–138. doi:10.1016/j.enggeo.2014.03.003
Wu, S. S., Hu, X. L., Zheng, W., Zhang, G., Liu, C., Xu, C., et al. (2022). Displacement behaviour and potential impulse waves of the Gapa landslide subjected to the Jinping Reservoir fluctuations in Southwest China. Geomorphology 397, 108013. doi:10.1016/j.geomorph.2021.108013
Yan, G. Q., Huang, B. L., Dai, Z. W., and Qin, Z. (2020). A study of the deterioration effect of limestone bank slope rock mass at the Wuxia section of the Three Gorges Reservoir area (in Chinese). Hydrogeol. Eng. Geol. 47, 62–72. doi:10.16030/j.cnki.issn.1000-3665.202003064
Yan, G. Q., Huang, B. L., Qin, Z., Dai, Z. W., and Zhang, Q. (2021). Rock mass deterioration model of bank slope based on high-precision 3D multiperiod point clouds in the Three Gorges Reservoir, China. Q. J. Eng. Geol. Hydroge 55 (1), qjegh2020–100. doi:10.1144/qjegh2020-100
Yao, W. M., Li, C. D., Zhan, H. B., Zhou, J. Q., Criss, R. E., Xiong, S., et al. (2020). Multiscale study of physical and mechanical properties of sandstone in three gorges reservoir region subjected to cyclic wetting-drying of Yangtze River water. Rock Mech. Rock Eng. 53, 2215–2231. doi:10.1007/s00603-019-02037-7
Yin, Y. P., Huang, B. L., Li, B., Zhang, Z. H., Yan, G. Q., and Zheng, J. H. (2021). Research on the deterioration index of karst rock mass in the fluctuating water level zone of Three Gorges Reservoir area (in Chinese). Acta Geol. Sin. 95 (08), 2590–2600. doi:10.19762/j.cnki.dizhixuebao.2021198
Yin, Y. P., Huang, B. L., Liu, G. G., and Wang, S. C. (2015). Potential risk analysis on a Jianchuandong dangerous rockmass-generated impulse wave in the Three Gorges Reservoir, China. Environ. Earth Sci. 74, 2595–2607. doi:10.1007/s12665-015-4278-x
Yin, Y. P., Huang, B. L., Wang, W. P., Wei, Y. J., Ma, X. H., Ma, F., et al. (2016). Reservoir-induced landslides and risk control in three gorges project on Yangtze River, China. J. Rock Mech. Geotech. Eng. 8, 577–595. doi:10.1016/j.jrmge.2016.08.001
Yin, Y. P., Huang, B. L., Zhang, Q., Yan, G. Q., and Dai, Z. W. (2020a). Research on recently occurred reservoir-induced kamenziwan rockslide in three gorges reservoir, China. Landslides 17, 1935–1949. doi:10.1007/s10346-020-01394-7
Yin, Y. P., Wang, L. Q., Zhang, W. G., and Dai, Z. W. (2022). Research on the collapse process of a thick-layer dangerous rock on the reservoir bank. Bull. Eng. Geol. Environ. 2022, 109. doi:10.1007/s10064-022-02618-x
Yin, Y. P., Yan, G. Q., Huang, B. L., Dai, Z. W., and Qin, Z. (2020b). Geological strength index of the slope rock mass deterioration process of the hydro-fluctuation belt in the Three Gorges Reservoir, China (in Chinese). J. Hydraul. Eng. 51, 883–896. doi:10.13243/j.cnki.slxb.20200280
Yin, Y. P., Zheng, W. M., Liu, Y. P., Zhang, J. Y., and Li, X. C. (2010). Integration of GPS with InSAR to monitoring of the jiaju landslide in sichuan, China. Landslides 7, 359–365. doi:10.1007/s10346-010-0225-9
Zhang, C. Y., Dai, Z. W., Tan, W. J., Yang, Y. T., and Zhang, L. H. (2022). Multiscale study of the deterioration of sandstone in the three gorges reservoir area subjected to cyclic wetting–cooling and drying–heating. Rock Mech. Rock Eng. 55 (9), 5619–5637. doi:10.1007/s00603-022-02929-1
Zhang, C. Y., Yin, Y. P., Yan, H., Li, H. X., Dai, Z. W., and Zhang, N. (2021). Reactivation characteristics and hydrological inducing factors of a massive ancient landslide in the three Gorges Reservoir, China. Eng. Geol. 292, 106273. doi:10.1016/j.enggeo.2021.106273
Zhang, C. Y., Yin, Y. P., Yan, H., Zhu, S. N., Zhang, M., and Wang, L. Q. (2023). Centrifuge modeling of unreinforced and multi-row stabilizing piles reinforced landslides subjected to reservoir water level fluctuation. J. Rock Mech. Geotechnical Eng. doi:10.1016/j.jrmge.2023.09.025
Zhang P, P., Zhang, S. L., Huang, B. L., Wang, X., Guo, J., Dai, Z. W., et al. (2021). Study on the evolution model of neogenic landslide (collapse) hazards in rock mass of hydro-fluctuation belt (in Chinese). J. Eng. Geol. 1. –13. doi:10.13544/j.cnki.jeg.2021-0164
Zhao, C., Kang, Y., Zhang, Q., Lu, Z., and Li, B. (2018). Landslide identification and monitoring along the jinsha river catchment (wudongde reservoir area), China, using the InSAR method. Remote Sens. 10, 993. doi:10.3390/rs10070993
Zhao, S., Zeng, R., Zhang, H., Meng, X., Zhang, Z., Meng, X., et al. (2022). Impact of water level fluctuations on landslide deformation at longyangxia reservoir, qinghai Province, China. Remote Sens. 14, 212. doi:10.3390/rs14010212
Keywords: reservoir bank slope, rock mass deterioration, geohazard recognition, UAV photography, image interpretation
Citation: Dai Z, Zhang Y, Zhang C, Fu X, Zhang P and Ye R (2024) A photographic method to identify reservoir geohazards induced by rock mass deterioration of hydro-fluctuation belt. Front. Earth Sci. 12:1365272. doi: 10.3389/feart.2024.1365272
Received: 04 January 2024; Accepted: 07 March 2024;
Published: 25 March 2024.
Edited by:
Tao Wen, Yangtze University, ChinaReviewed by:
Shuangshuang Wu, Hohai University, ChinaFei Guo, China Three Gorges University, China
Zhang Wengang, Chongqing University, China
Copyright © 2024 Dai, Zhang, Zhang, Fu, Zhang and Ye. This is an open-access article distributed under the terms of the Creative Commons Attribution License (CC BY). The use, distribution or reproduction in other forums is permitted, provided the original author(s) and the copyright owner(s) are credited and that the original publication in this journal is cited, in accordance with accepted academic practice. No use, distribution or reproduction is permitted which does not comply with these terms.
*Correspondence: Chenyang Zhang, chenyangzhang1993@126.com