- Indian Institute of Technology (ISM), Dhanbad, India
Surface coal mines, when abandoned or closed, pose significant environmental and socioeconomic challenges. Repurposing these sites is crucial for sustainable land use and responsible resource management. This study presents a comprehensive decision framework tailored to the Indian mining context, utilizing a hybrid approach combining the analytic hierarchy process (AHP) and technique for order preference by similarity to an ideal solution (TOPSIS) methodology. The proposed framework assesses and ranks alternative repurposing options by considering a multi-criteria evaluation, including ecological, economic, social, and regulatory factors. AHP is employed to determine the relative importance of these criteria, reflecting the unique priorities and perspectives of stakeholders involved in the repurposing process. TOPSIS then identifies the optimal alternatives based on their overall performance against the established criteria. This hybrid methodology contributes to informed decision-making in the sustainable repurposing of abandoned surface coal mines in India. It aids in identifying the most viable and environmentally responsible alternatives, promoting efficient land use and resource conservation while addressing the challenges associated with abandoned mine sites. The methodology’s applicability extends globally to industries facing similar repurposing challenges, facilitating the transition toward a more sustainable and responsible land reclamation and resource management approach. The methodology is implemented using real mine data and demonstrates the analysis for evaluation among multiple alternatives such as solar parks, fish farming, eco-resorts, forestry, and museums. In our study, eco-resorts show more promise based on the significant potential for local economic development, provision of local employment, long-term revenue generation, potential for upskilling local youth in management, gardening, construction, and animal husbandry, and serving as a site for exhibitions of various arts and crafts.
1 Introduction
The coal mining industry faces various challenges, such as depletion of resources, profitability issues, and safety concerns that may lead to the closure of some mines over time (Laurence, 2006). The closure of coal mines has a significant impact on the social, physical, and environmental aspects of the affected regions and the communities that depend on the mines for their livelihoods (Bainton, N., & Holcombe, 2018). Thus, it is essential to plan and implement closures effectively to ensure sustainability and wellbeing in these areas.
In November 2021, during COP26 (Conference of the Parties) in Glasgow, India announced five key elements for its climate action, referred to as the “Panchamrit” goals (Mishra and Srivastava, 2022):
1. Non-fossil fuel capacity to reach 500 GW by 2030 (currently 179.3 GW).
2. 50% of energy requirements to be met through RE by 2030 (currently 43%).
3. Carbon emissions to be reduced by one billion tonnes by 2030.
4. Emissions intensity of GDP to reduce by 45% by 2030 (vs. 2005).
5. Net-zero emissions by 2070 (Das et al., 2023).
Mine closure supports the goal of moving toward net-zero emissions (Jones, 2023). It also creates an opportunity for regional transformation and economic diversification by releasing land that can be used for alternative purposes (Laurence, 2006). The impact of mine closure on the local community and the environment is a complex and multifaceted issue that requires careful planning and management (Bainton and Holcombe, 2018). The main objectives of mine closure are to ensure the long-term safety and stability of the site, to minimize the environmental and social impacts of mining activities (Botha et al., 2018), and to maximize the opportunities for sustainable development and alternative livelihoods for the nearby community. A good mine closure plan should begin early in the development process and be updated regularly. The company should plan the mine closure in a way that ensures the quality of the affected area is restored or enhanced while maintaining and increasing the benefits generated by the operation (Demirkan et al., 2022). Mine closure is usually applicable to closed, abandoned, or discontinued mines, as shown in Figure 1. Re-operationalization is applicable to a sub-category of mines currently not operational due to global market conditions, technology advancements, and variations in commodity prices (Muldoon, J.A., 2015). The reclamation and rehabilitation type of mine closure is the conversion of wasteland into land suitable for use as a site of habitation or cultivation and the restoration of degraded ecosystems to their natural state (Cui et al., 2020a). Repurposing is an alternative to the reclamation process where non-operational mines can be used for energy storage, renewable energy, water disposal, flood protection, tourism, wildlife habitat, pisciculture, horticulture, etc. (Keenan and Holcombe, 2021).
India has a total estimated coal reserve of 344 billion metric tons as of 1 April 2020, and it ranks fourth among all nations (Agarwal et al., 2024). The opening of the coal sector to foreign direct investment and a significant increase in power demand have led to a surge in coal production in the country (Anitha, 2012). Private players are actively participating in mining operations, contributing to this growth. This has led to the quick abandonment of several coal mines pending reclamation or nearly abandoned in coming years. A pie chart depicted in Figure 2 illustrates the distribution of three types of coal mines in India: open-cast, underground, and mixed-type. (Coal India Limited, 2022-23). Open-cast and underground mines lead the count for mines in India. As of February 2023, 1,610 ha of land in India could be converted into green cover by various means of eco-parks, forests, horticulture, pit lake base tourism parks, etc. Data on the number of non-operational coal mines from various coal companies in India are presented in Figure 3 (Press Information Bureau- Ministry of Coal, 2023). The “Just-transition from coal” committee constituted in July 2021 under the India–US strategic Clean Energy Partnership (Niti Aayog, 2022) aims to make a transition from coal to fuel the power sector (Joshi and Dsouza, 2023). For a clean energy transition and net-zero emissions pledge by 2070, the Ministry of Coal is already working with the World Bank to close mines based on Just-transition principles (Lal et al., 2022; Guliyeva, 2022). Intermediate milestones include peaking coal demand between 2040 and 45, phasing out coal-based electricity by 2050–60 and achieving an ∼80% reduction in coal consumption by 2050, with a complete transition by 2060–70 (Bhushan et al., 2020). The global view now stands that mining itself should be visualized as a temporary land use that can be followed by other uses like agriculture, conservation, recreation, urban development, or renewable energy production (Keenan and Holcombe, 2021). The mining sector and the government are responsible for ensuring that the extraction and processing of mineral resources are done in a sustainable way. This means balancing the economic, environmental, and social impacts of their activities while respecting the rights and interests of affected communities (Lu et al., 2020). Addressing these challenges requires collaboration between the government, mining sector, local communities, academia, international organizations, and other relevant actors to find innovative and effective solutions (Siontorou, 2023).
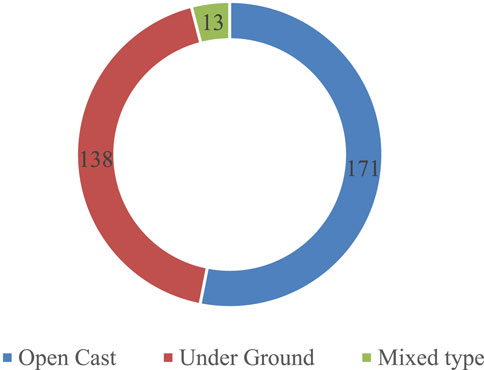
Figure 2. Distribution of all coal mines in India categorized as open-cast, underground, and mixed types.
Numerous examples exist of how abandoned mines can be utilized for beneficial purposes such as creating habitats for wildlife (Lituma et al., 2021), eco-tourism opportunities (Gandah and Atiyat, 2016), recreational parks (Wanhill, 2000; Ballesteros and Ramírez, 2007), solid waste management (Deng et al., 2020; Holcombe and Keenan, 2020), and restoration methods for pit lakes that can be used for aquaculture and sport fishing (Miller, 2008). To mitigate the environmental impact of the open pit acidic lakes, some potential measures are to cover the lakes with alkaline materials such as recycled waste lime and to plant vegetation along the riverbanks (Lund and Blanchette, 2021). These actions could enhance the aquatic biodiversity that is essential for the ecosystem. Other end uses that offer multiple, mutually reinforcing, and lasting benefits are electricity system planning and renewable energy storage (Menéndez et al., 2019).
In a closed lignite mine in Kardia, North Greece, the possibility of energy generation using solar photovoltaic (PV) panels in combination with pumped hydro storage (PHS) technology is explored using time series and neural networks analysis (Louloudis et al., 2022). Other examples of energy storage and generation in closed mines include the potential benefits and limitations of compressed air energy storage (Lutyński, 2017; Schmidt et al., 2020) and using solar and wind energy (Choi, Y., & Song, 2017). According to Bódis et al. (2019), the European Union’s coal areas have a large amount of post-mining landscape space that is suitable for the installation of photovoltaic systems. There are suggestions for using abandoned coal mines as sources of renewable energy and carbon sinks Lyu et al. (2022).
Research on repurposing abandoned mines often only briefly touches on the subject without providing a thorough explanation of how to choose reutilization strategies. Numerous case studies and research methodologies have been discussed and implemented in both China and European countries. In India, some energy-based repurposing alternatives have been studied, such as a hybrid system that combines solar photovoltaic (PV) panels, a grid connection, and a pumped storage hydropower (PSH) system using the abandoned open-cast coal mine as a reservoir (Bhimaraju et al., 2023). However, there has been a lack of mathematical modeling based on Indian conditions for evaluating various alternatives simultaneously. Multi-criteria decision making (MCDM) is one such powerful approach used in various fields to make informed choices when confronted with complex decisions that involve multiple conflicting criteria (Tzeng and Huang, 2011). In MCDM, decision makers consider a range of factors or criteria and assess and compare different alternatives based on these criteria. The goal is to identify the most suitable option that best aligns with the desired outcomes and objectives. Numerous MCDM methods and techniques are available, including the weighted sum model (Odu, 2019), the analytic hierarchy process (AHP) (Saaty, 2008), the technique for order preference by similarity to ideal solution (TOPSIS) (Behzadian et al., 2012), the analytic network process (ANP) (Saaty, 2005), the preference ranking organization method for enrichment evaluations (PROMETHEE) (Brans and Mareschal, 2005), and many more. Using multi-attribute utility theory (MAUT), researchers at the Henderson Mill and Mine (Colorado, United States) determined which alternative best reflects stakeholder preferences and results in the most sustainable outcome out of glass manufacturing of tailings, organic shrimp farming, and hemp production (Demirkan et al., 2022). A mined land suitability analysis (MLSA) by Soltanmohammadi et al. (2010) used a combination of AHP and TOPSIS to rank different land-use alternatives based on multiple criteria for a hypothetical mined land. The concept of cloud theory, a mathematical tool for dealing with uncertainty and fuzziness, was introduced by Cui et al. (2020b), who applied it to the evaluation of mine closure risks for a coal mine in China. A three-dimensional risk model was utilized by Amirshenava and Osanloo (2018) to identify, assess, and prioritize risks based on their likelihood, impact, and detectability. Recently, SWOT-AHP was chosen as the framework for developing strategies for coal mine transformation by Spanidis et al. (2023), where the AHP and its eigenvalue calculation framework are integrated with SWOT analysis. AHP and TOPSIS have been applied to rank states and districts based on criteria such as solar radiation, land availability, grid connectivity, and socioeconomic factors by Sindhu et al. (2017). A comprehensive review of major methods for using multi-criteria decision analysis and risk management in post-coal mining land-use selection can be accessed from (Ronyastra et al., 2023).
The main focus of this study is to develop a well-structured framework for repurposing closed coal mines and identifying the most appropriate repurposing solutions for Indian mining conditions. These efforts aim to effectively address critical issues associated with closed mines, including environmental, social, and economic concerns, with the ultimate goal of benefiting the local community. To derive a preference order of alternatives for the optimum after-use of a hypothetical mined land, especially when influenced by multiple stakeholders, a combination of AHP and TOPSIS procedures is recommended within the proposed approach. AHP helps to break down complex decision problems into a hierarchy of criteria and alternatives, which enables decision makers to systematically analyze and prioritize factors based on their relative importance. At the same time, TOPSIS provides a quantitative method for comparing alternatives against ideal and anti-ideal solutions. It considers the proximity to the ideal and the distance from the anti-ideal while evaluating the alternatives. AHP and TOPSIS are two decision-making methodologies that can be customized to suit various contexts and preferences of decision makers.
The rest of the study is arranged as follows: Section 2 details the methodology used and the selection of alternatives, criteria, and attributes derived from our sustainability indicator concept. Sections 3 extensively discusses the evaluation result obtained, and the article concludes in Section 4.
2 Methodologies
2.1 Research frame
Our proposed decision-making process framework involves integrating expert opinion to identify relevant indicators. Identification of these indicators is discussed in a later sub-section. However, an important aspect of this approach revolves around the active participation of stakeholders and experts in the decision-making process. These stakeholders, which include government agencies, environmental organizations, and local communities and groups of individuals, aptly called decision makers, play an important role in shaping the direction of the efforts (Kuipa, 2023). To facilitate this evaluation, decision makers have been empowered to contribute to a scaling process defined by Saaty based on a 9-point scale where 1 indicates the lowest and 9 is the highest condition of each indicator (Table 1). This scaling mechanism enables decision makers to assign importance and value to various criteria and constraints associated with mine revival. Next, criteria and attributes are meticulously defined to evaluate alternatives, covering environmental, social, and economic aspects. Utilizing AHP, the attribute’s relative importance is established through input from all decision makers, ensuring a consensus on their significance. Subsequently, data are normalized for each attribute to enable the application of the TOPSIS method. Alternatives are then evaluated against the weighted attribute by stakeholders, leading to the application of TOPSIS to rank the options based on their proximity to the ideal solution. This culminates in the generation of a preference order that reflects collective stakeholder preferences and criteria priorities. In cases of divergent rankings, a consensus-building process may be initiated to reconcile differences. By integrating group AHP and TOPSIS results in this manner, the approach ensures the inclusion of multiple stakeholders’ input and preferences, fostering a more robust and equitable decision-making process in determining the optimal use of mined land. The framework and the mutual relationships between individual processes in the study are shown schematically in Figure 4.
2.2 Identification of indicators
Indicators in mine repurposing are specific metrics or criteria used to assess the progress, success, and sustainability of the process of converting an abandoned or exhausted mine site into a new, productive, and often sustainable use (Marnika et al., 2015). These indicators help stakeholders, including government agencies, environmental organizations, and local communities, evaluate the outcomes and impacts of mine repurposing efforts. Table 2 depicts some key indicators commonly used in the assessment of mine repurposing.
2.3 Selection of repurposing alternatives and attributes
The process of selecting repurposing alternatives for closed or abandoned surface coal mines is a critical undertaking that requires a thoughtful and holistic approach. The priorities for selecting these alternatives are established through a thorough review of various repurposing options and extensive consultations with stakeholders who hold a vested interest in the outcome. A comprehensive assessment is conducted to determine the initial repurposing alternatives, taking into account several indicators (Table 2). These indicators encompass an in-depth evaluation of the existing mine conditions, the natural and geological attributes of the site, and the socioeconomic considerations specific to the region in question. It is important to note that the decision-making process is focused not on all closed coal mines in general but specifically on the coalfield under consideration, where two or three coal mines have been closed or abandoned. In the pursuit of a well-balanced and informed decision, a diverse range of re-habitation options is presented to the decision makers. These options span various forms of land use, each with its own merits and implications for the environment, economy, and community.
Repurposing abandoned mines is a complex task influenced by a range of factors, including local conditions, the state of the mine, and natural surroundings. However, when constructing an indicator system for this purpose, it is crucial to adhere to certain guidelines. Specifically, the framework should aim to encompass as many key factors as possible while considering the availability of essential data.
Based on feasible post-mining land uses addressed in the literature and expert consultation, nine distinct categories containing 16 alternatives were selected and are shown in Figure 5. While all 16 repurposing options are technically feasible for closed mines, our focus here is directed toward eight alternatives. The eight repurposing alternatives are solar park, pumped storage hydropower (PSH), fish farming, eco-resort, eco-park, museum, forestry, and warehouse. All these eight alternatives are particularly excelling in terms of their alignment with critical factors, including environmental sustainability, social impact, and economic viability. One additional factor is that these eight alternatives could fit in a closed open-cast mine.
The selection of an optimal repurposing of a closed mine involves various internal and external restricted conditions. It is worth noting that evaluating the reclamation suitability of mining land shares many common economic, social, and environmental factors with the entire life cycle of closed mines. Various factors come into play when analyzing repurposing options. Economic factors like capital cost, maintenance costs, and revenue generation possibilities are crucial. While evolution was taking place, assistance from some government tender documents was also used, such as when rating solar parks and PSH (Pavloudakis et al., 2023; Tender document of Coal India Limited (CIL); Report CER, 2021; Report CSTEP, 2021). Technical factors address constraints; the availability of some repurposing techniques may influence the selection of reutilization modes. Mine-specific factors pertain to the characteristics of the individual mine, including size, depth, and storage volume. Hydrogeological conditions like water quality and permeability ensure the repurposing is fit for the local situation. Natural factors like the availability of sunny days and annual rainfall decide the viability of water-based options and renewable energy alternates. Consideration of social factors, such as the target audience (urban dwellers; villagers); proximity to urban areas, villages, or remote locations; and their positive impact on public acceptance, is essential when evaluating alternative options (Schneider and Greenberg, 2023). In this study, we identify 14 such attributes that fall well within the most viable criteria for a closed mine repurposing in Table 3. In order to facilitate the scoring of attributes in accordance with our AHP-TOPSIS methodology, it is advisable to categorize these attributes. This categorization will provide decision makers with a more streamlined and convenient approach to the scoring process. Attribute definition and classification are presented in Table 4. The above attributes may act as a constraint and can be either permanent or temporary, depending on factors such as the type of mine (e.g., open-cast or underground). As a result, the diverse conditions of different mines may give rise to unique repurposing options beyond the primary indicators. For instance, underground mines are generally unsuitable for repurposing as solar parks, eco-parks, eco-resorts, or for agriculture or fish farming. Permanent constraints may also include variables like the number of sunny days in a year, annual rainfall, and the mine’s location in relation to urban areas, villages, or remote regions. In contrast, some constraints may not be permanent but can still significantly impact the repurposing process. For instance, altering the depth of a mine through landfilling is possible but costly, leading stakeholders to explore alternatives like creating a pit lake through various means (Cui et al., 2020b). Within this framework, criteria are used to define the technical attributes that guide the decision-making process. Each of these criteria plays a pivotal role in influencing the preferences of individual decision makers as they seek repurposing alternatives that best align with the technological requirements associated with these criteria. This methodology ensures that the chosen alternatives not only maximize the potential of the land but also meet the needs and expectations of all involved parties.
2.4 Attribute weighting using AHP
The AHP approach was used to determine the weights of the criteria. The seven decision makers selected included a research scientist from IIT-ISM, Dhanbad, who is an expert in data analysis techniques and methodologies, which is crucial for effectively applying AHP principles in decision-making processes. A member of the legislative assembly from the mine constituency was interviewed. As a representative of the community, they provided a valuable insight into socioeconomic factors, public opinion, and policy implications, which are important considerations in AHP. The third was an environmentalist who was asked to be a part of the panel because their input is essential for evaluating the ecological impact of proposed actions. The mine’s general manager, due to their firsthand experience managing mining projects, can assess the feasibility, risks, and benefits of various mining-related options. An energy expert weighted the criteria based on specialized knowledge of energy technologies, market trends, and regulatory frameworks. A pisciculturist assessed the proposed activities’ impacts on aquatic habitats, biodiversity, and fisheries resources through weighting. Finally, a geologist provided insights into geological formations, mineral resources, and land-use hazards. Together, these diverse experts bring together multidisciplinary perspectives and specialized knowledge essential for conducting a thorough AHP-based decision analysis, ensuring that holistic considerations of environmental, socioeconomic, technical, and geological factors are included in the decision-making process.
The AHP weighting process can be summed up as follows. In the first step, decision makers are asked to compare the importance of one attribute relative to another. Pairwise comparisons are made for all attributes, and a numerical value is assigned to express the preference or importance. The comparisons are typically done on a scale from 1 to 9, with 1 indicating equal importance and 9 indicating extreme importance (Table 1). The comparisons are organized into a pairwise comparison matrix c, where cij represents the importance of attribute i relative to j.
In this matrix, the main diagonal elements (e.g., 1, 1, 1, … , 1) represent the relative importance of an element compared to itself, which is always considered equal (cij = 1). The elements above the main diagonal (cij where i < j) are the values that should be filled by decision makers to represent their preferences. These values represent the preference of element “j” compared to element “i”. Conversely, the elements below the main diagonal (cij where i > j) are derived as the reciprocal values of the elements above the main diagonal to ensure that the matrix is consistent and satisfies the reciprocal relationship, that is, cij * cji = 1 for all i ≠ j.
Second, the pairwise comparison matrix is normalized by dividing each element in a column by the column’s sum. This creates a normalized matrix N. Then, the average of the columns in the normalized matrix is calculated to obtain the weight vector W. The weight vector represents the relative importance or weight of each criterion.
Finally, to ensure the reliability of the judgments made during pairwise comparisons, a consistency check is performed using the consistency index (CI) and the random index (RI).
The CI is calculated by comparing the actual consistency of the matrix to what would be expected by chance. If CI is greater than 0, it indicates some inconsistency. To evaluate this inconsistency, the consistency ratio (CR) is calculated by dividing CI by the RI. If CR is less than 0.10 (a commonly used threshold), the judgments are considered sufficiently consistent.
2.5 Alternative evaluation using TOPSIS
After determining the criteria weights using AHP, we can proceed with evaluating the alternatives using TOPSIS, following these steps of the method:
Step 1: As shown in a general m by n matrix D of alternative An criterion Cn, assign numerical values or scores from the 1–9 scale defined by Saaty (Table 1) to each alternative for each criterion. D shows the decision matrix according to a subjective decision maker.
Step 2: Create the normalized decision matrix to ensure that the elements of the matrix are on a common scale so that different criteria with different measurement units can be compared. The steps involve creating a normalized matrix N whose elements are obtained by using Equations 4, 5.
where N (dij) is the normalized value for the cost attribute Cij. dij is the raw (original) value for the cost attribute for an alternative Ai over criterion Cj. dmaxj is the maximum value of the cost attribute across all alternatives for criterion Cj. dminj is the minimum value of the cost attribute across all alternatives for criterion Cj. In our study, the capital cost and maintenance are cost attributes, while the rest are all benefits.
Step 3: Multiply the normalized scores of each alternative by the corresponding criteria weights determined in the AHP step. This step emphasizes the importance of each criterion. The weighted decision matrix Rij is obtained by Equation (5)
Step 4: Determine the positive ideal solution and the negative ideal solution. For benefit criteria (where higher values are better), R+ is the maximum value in the corresponding column and R− is the minimum value. For cost criteria (where lower values are better), R+ is the minimum value in the corresponding column and R− is the maximum value.
Step 5: Establish the ideal and anti-ideal solutions for each criterion. The best performance for each criterion is represented by the ideal solution, while the lowest performance is represented by the anti-ideal solution. This determination is achieved through calculations utilizing the following equation:
Step 5: Rank the alternative based on the following equation:
3 Result
It is important to highlight that the calculation process should involve a team of decision makers comprising relevant experts. The aggregation of individual judgments can be accomplished through methods such as the weighted geometric mean. Calculating the value of CI for our AHP matrix using Equation 1, we obtain a value of 0.150,049, where
The decision makers were requested to provide ratings for criteria for each alternative in the TOPSIS analysis (Table 6). Subsequently, the criteria weights were applied to the normalized scores within the TOPSIS framework. This multiplication process emphasized the significance of criteria identified as more critical in the AHP analysis. The determination of ideal and non-ideal solutions, along with the ranking of alternatives based on their relative proximity to the ideal solution, is illustrated in Table 7. The order of eight alternatives is also depicted through a graph in Figure 6, which shows that eco-resort was considered the best repurposing alternative for a closed/abandoned surface coal mine in India. This choice is driven by a holistic evaluation of both economic and environmental sustainability, as well as the potential positive impact on the local community.
4 Discussion
An eco-resort was deemed the optimal choice due to its significant potential for local economic development. When a closed mine is repurposed into an eco-resort, the local economy can experience a revitalization, as a resort provides a means to increase income and mitigate unemployment issues stemming from job losses resulting from mine closures. This is achieved by aligning the repurposing efforts with the existing skill sets of the local workforce, such as gardening, construction, culinary arts, and hospitality, which are highly transferrable to the operation of an eco-resort in comparison to solar park or PSH. Furthermore, the versatility of eco-resorts in terms of scalability and budgetary feasibility makes them an attractive option for stakeholders. Eco-resorts have the unique ability to be developed incrementally based on the revenue and income generated from their services. This adaptability ensures that the project can remain financially sustainable, catering to the stakeholders’ financial capacities while gradually expanding and improving over time. While the maintenance of resorts does incur costs, it is notably lower compared to alternative repurposing options like solar parks and pumped storage hydropower (PSH). Additionally, the maintenance services required can be viewed as a valuable source of employment for the local population, further addressing the issue of unemployment and ensuring the financial viability of the eco-resort. Another significant advantage of repurposing mines into eco-resorts is the positive impact on the valuation of surrounding land and properties. This appreciation in property values can yield long-term benefits for local residents, as it enhances their overall asset value and economic prospects. Basu and Mishra (2023) reviewed 112 studies and suggested that tourism-based reclamation techniques are the solutions implemented most often for closed or abandoned mines.
5 Conclusion
In conclusion, this study introduces a comprehensive decision framework tailored to the Indian mining context that offers a novel and practical approach to repurposing abandoned surface coal mines. This approach represents a significant step for India to progress toward achieving net-zero coal mining by facilitating the transition toward sustainable and responsible land reclamation and resource management. It promotes efficient land use, resource conservation, and addresses the multifaceted challenges associated with abandoned mine sites. The hybrid methodology, combining AHP and TOPSIS, enables informed decision-making by assessing and ranking alternative repurposing options. The research highlights the importance of considering a multi-criteria-criteria evaluation, encompassing ecological, economic, social, and regulatory factors, in the decision-making process. AHP is instrumental in determining the relative importance of these criteria, reflecting the diverse perspectives and priorities of stakeholders engaged in the repurposing endeavor. TOPSIS then identifies optimal alternatives, ensuring a comprehensive assessment of their overall performance against the established criteria. The suitability of the mine site for an eco-resort is also an important consideration, offering significant potential for local economic development, employment opportunities, long-term revenue generation, and skill development for local youth. Factors such as the size of the mine and the availability of space for the construction of facilities, including pools or lakes, within the resort, can be pivotal in ensuring a successful transformation. Moreover, if water quality within the mine pit presents a challenge, it can be addressed by converting the water area into a floating solar park, thus enhancing the resort’s sustainability credentials. Working within the resort’s operations, residents can acquire valuable skills and knowledge about sustainable practices, further empowering the community in terms of environmental awareness and job opportunities. Resorts can be located anywhere there is space, whether in remote villages or near urban centres, thus relaxing the constraints on their location. Overall, this study provides a valuable tool for stakeholders involved in repurposing abandoned mine sites and supports sustainable land use and responsible resource management.
Coal India Limited (CIL) is actively engaged in the transformation of its disused mines into ecological parks, which have gained popularity as eco-tourism destinations. These ecological parks and tourist sites are also proving to be a means of sustenance for the local population. Currently, 30 such ecological parks are experiencing a consistent influx of visitors, and there are ongoing plans to establish additional ecological parks and ecological restoration sites within CIL’s mining regions. Glimpses of such habitational work are shown in Figure 7–9. The company has successfully planted over three million saplings in the current financial year. Over the 5 fiscal years ending in FY ‘22, CIL has greened 4,392 ha within the mining lease area, creating a carbon sink potential of 2.2 lakh tonnes per year.
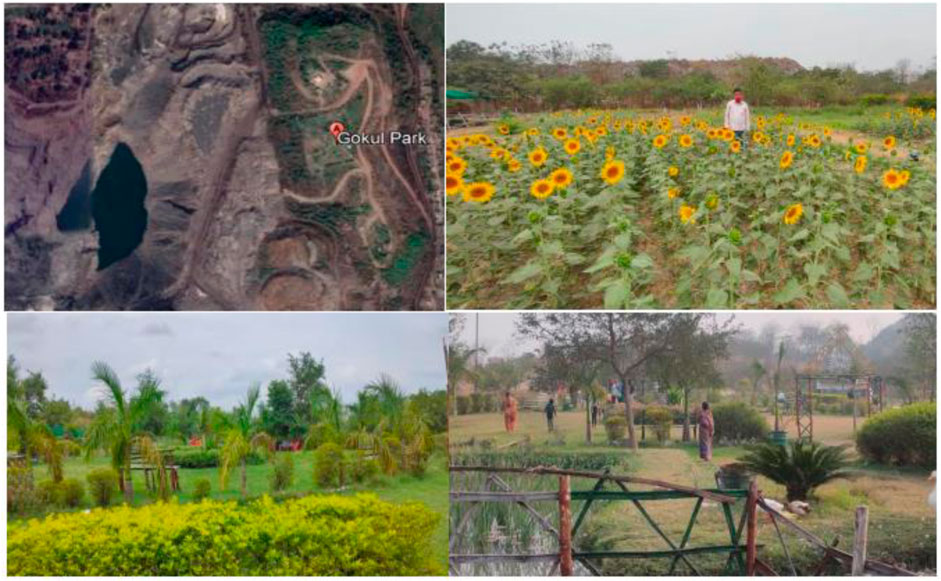
Figure 7. A view of an eco-park built by Bharat Coking Coal Limited (BCCL), over 10 ha of mined-out land in NT-ST-Jeenagora Project of Lodna Area. Source: https://www.bcclweb.in/files/2022/08/ECO-PARKS.pdf.
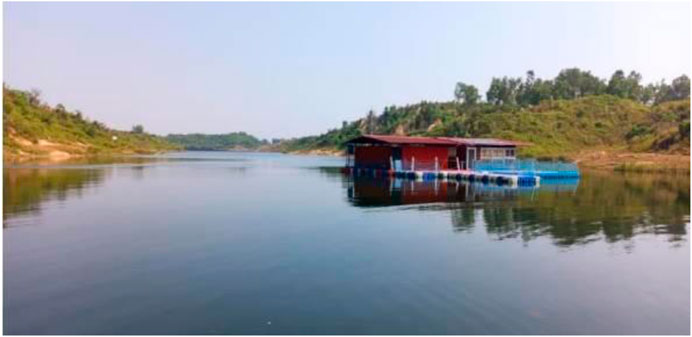
Figure 8. Water sports center and floating restaurant developed at abandoned quarry no. 6 of the Bishrampur OC mine at Kenpara by South Eastern Coalfields Limited. Source: https://www.pib.gov.in/PressReleasePage.aspx?PRID=1900977.
The initiative is particularly applicable in developing countries with high population density. The MCDM techniques can be adapted and implemented in other regions with abandoned mine sites, especially in countries undergoing transitions in their energy sectors.
Data availability statement
The original contributions presented in the study are included in the article/Supplementary Material, further inquiries can be directed to the corresponding author.
Author contributions
AS: methodology and writing–original draft. SA: supervision, visualization, and writing–review and editing. AP: data curation, investigation, validation, and writing–review and editing.
Funding
The author(s) declare that no financial support was received for the research, authorship, and/or publication of this article.
Acknowledgments
We would like to express our sincere gratitude to Coal India Limited (CIL) and its subsidiary for their valuable support and cooperation in providing data and insights that greatly contributed to the success of this research. Their collaboration was instrumental in the completion of this study, and we acknowledge their assistance with appreciation.
Conflict of interest
The authors declare that the research was conducted in the absence of any commercial or financial relationships that could be construed as a potential conflict of interest.
Publisher’s note
All claims expressed in this article are solely those of the authors and do not necessarily represent those of their affiliated organizations, or those of the publisher, the editors, and the reviewers. Any product that may be evaluated in this article, or claim that may be made by its manufacturer, is not guaranteed or endorsed by the publisher.
References
Agrawal, K., Pathak, M., Jana, K., Unni, J., and Shukla, P. (2024). Just transition away from coal: vulnerability analysis of coal districts in India. Energy Res. Soc. Sci. 108, 103355. doi:10.1016/j.erss.2023.103355
Amirshenava, S., and Osanloo, M. (2018). Mine closure risk management: an integration of 3D risk model and MCDM techniques. J. Clean. Prod. 184, 389–401. doi:10.1016/j.jclepro.2018.01.186
Anitha, R. (2012). Foreign direct investment and economic growth in India. Int. J. Mark. Financial Serv. Manag. Res. 1 (8), 108–125.
Bainton, N., and Holcombe, S. (2018). A critical review of the social aspects of mine closure. Resour. Policy 59, 468–478. doi:10.1016/j.resourpol.2018.08.020
Ballesteros, E. R., and Ramirez, M. H. (2007). Identity and community—reflections on the development of mining heritage tourism in Southern Spain. Tour. Manag. 28 (3), 677–687. doi:10.1016/j.tourman.2006.03.001
Basu, D., and Mishra, S. (2023). A comprehensive literature review approach for assessing probable impact of post-reclamation strategies applied to abandoned mines. J. Min. Environ. 14 (3), 871–896. doi:10.22044/jme.2023.13007.2356
Behzadian, M., Otaghsara, S. K., Yazdani, M., and Ignatius, J. (2012). A state-of the-art survey of TOPSIS applications. Expert Syst. Appl. 39 (17), 13051–13069. doi:10.1016/j.eswa.2012.05.056
Bhimaraju, A., Mahesh, A., and Nirbheram, J. S. (2023). Feasibility study of solar photovoltaic/grid-connected hybrid renewable energy system with pumped storage hydropower system using abandoned open cast coal mine: a case study in India. J. Energy Storage 72, 108206. doi:10.1016/j.est.2023.108206
Bhushan, C., Banerjee, S., and Agarwal, S. (2020). Just Transition in India: an inquiry into the challenges and opportunities for a post-coal future. Delhi, India: Sustainability Innovations and Advisories Pvt. Ltd.
Bódis, K., Kougias, I., Taylor, N., and Jäger-Waldau, A. (2019). Solar photovoltaic electricity generation: a lifeline for the European coal regions in transition. Sustainability 11 (13), 3703. doi:10.3390/su11133703
Botha, D., Van der Waldt, G., and Ackermann, M. (2018). Potential socio-economic consequences of mine closure. TD J. Transdiscipl. Res. South. Afr. 14 (1), 1–11. doi:10.4102/td.v14i1.458
Brans, J. P., and Mareschal, B. (2005). “PROMETHEE methods,” in Multiple criteria decision analysis (Springer), 163–186.
Choi, Y., and Song, J. (2017). Review of photovoltaic and wind power systems utilized in the mining industry. Renew. Sustain. Energy Rev. 75, 1386–1391. doi:10.1016/j.rser.2016.11.127
Coal India Limited (2022). Integrated annual reports 2022-23. https://d3u7ubx0okog7j.cloudfront.net/documents/annuareportnsebse.pdf.
Cui, C. Q., Wang, B., Zhao, Y. X., and Xue, L. M. (2020a). Waste mine to emerging wealth: innovative solutions for abandoned underground coal mine reutilization on a waste management level. J. Clean. Prod. 252, 119748. doi:10.1016/j.jclepro.2019.119748
Cui, C. Q., Wang, B., Zhao, Y. X., Zhang, Y. J., and Xue, L. M. (2020b). Risk management for mine closure: a cloud model and hybrid semi-quantitative decision method. Int. J. Minerals, Metallurgy Mater. 27, 1021–1035. doi:10.1007/s12613-020-2002-7
Das, N., Murshed, M., Rej, S., Bandyopadhyay, A., Hossain, Md E., Mahmood, H., et al. (2023). Can clean energy adoption and international trade contribute to the achievement of India’s 2070 carbon neutrality agenda? Evidence using quantile ARDL measures. Int. J. Sustain. Dev. World Ecol. 30 (3), 262–277. doi:10.1080/13504509.2022.2139780
Demirkan, C. P., Smith, N. M., and Duzgun, S. (2022). A quantitative sustainability assessment for mine closure and repurposing alternatives in Colorado, USA. Resources 11 (7), 66. doi:10.3390/resources11070066
Deng, D. Q., Jiang, F. F., Duan, Y., and Peng, L. (2020). Solid waste management in urban mines based on resource reutilization mode of cleaner production. J. Mines, Metals Fuels 68 (2).
Gandah, F., and Atiyat, D. (2016). Re-use of abandoned quarries; case study of eco-tourism and rangers academy—ajloun—Jordan. J. Civ. Environ. Eng. 6, 238. doi:10.4172/2165-784x.1000238
Guliyeva, A. (2022). India’s transformation towards clean energy for a sustainable future: understanding and analyzing the role of intangible cultural heritage.
Holcombe, S., and Keenan, J. (2020). Mining as a temporary land use scoping project: transitions and repurposing; the social aspects of mine closure research consortium; centre for social responsibility in mining. Brisbane, Australia: Sustainable Minerals Institute.
Jones, A. G. (2023). Mining for net zero: the impossible task. Lead. Edge 42 (4), 266–276. doi:10.1190/tle42040266.1
Joshi, M., and Dsouza, S. (2023). 10 international experiences on Just energy transition planning and lessons for India. Role Coal a Sustain. Energy Mix India A Wide-Angle View, 168. doi:10.4324/9781003433088
Keenan, J., and Holcombe, S. (2021). Mining as a temporary land use: a global stocktake of post-mining transitions and repurposing. Extr. Industries Soc. 8 (3), 100924. doi:10.1016/j.exis.2021.100924
Kuipa, E. (2023). Developing a framework for the management and rehabilitation of abandoned mines in South Africa. South Africa: PhD diss., North-West University.
Lal, S. R., Suresh, J. H. G. M., Arjunan, P., and Suryan, A. (2022). Advancements in renewable energy transition in India: a review. Energy Sources, Part A Recovery, Util. Environ. Eff., 1–31. doi:10.1080/15567036.2021.2024921
Laurence, D. (2006). Optimisation of the mine closure process. J. Clean. Prod. 14 (3-4), 285–298. doi:10.1016/j.jclepro.2004.04.011
Lituma, C. M., Cox, J. J., Spear, S. F., Edwards, J. W., De La Cruz, J. L., Muller, L. I., et al. (2021). Terrestrial wildlife in the post-mined Appalachian landscape: status and opportunities. Appalachia's Coal-Mined Landscapes Resour. Communities a New Energy Era, 135–166. doi:10.1007/978-3-030-57780-3_6
Louloudis, G., Roumpos, C., Louloudis, E., Mertiri, E., and Kasfikis, G. (2022). Repurposing of a closed surface coal mine with respect to pit lake development. Water 14 (21), 3558. doi:10.3390/w14213558
Lu, P., Zhou, L., Cheng, S., Zhu, X., Yuan, T., Chen, D., et al. (2020). Main challenges of closed/abandoned coal mine resource utilization in China. Energy Sources, Part A Recovery, Util. Environ. Eff. 42 (22), 2822–2830. doi:10.1080/15567036.2019.1618992
Lund, M. A., and Blanchette, M. (2021). Can saline pit lakes offer biodiversity values at closure. Proceedings of the IMWA—mine water management for future generations; P. Stanley, C. Wolkersdorfer, and K. Wolkersdorfer, Eds, pp.300–306.
Lutyński, M. (2017). “An overview of potential benefits and limitations of Compressed Air Energy Storage in abandoned coal mines,” in IOP conference series: materials science and engineering (Switzerland: Springer Cham) 268, 012006.
Lyu, X., Yang, K., and Fang, J. (2022). Utilization of resources in abandoned coal mines for carbon neutrality. Sci. Total Environ. 822, 153646. doi:10.1016/j.scitotenv.2022.153646
Marnika, E., Christodoulou, E., and Xenidis, A. (2015). Sustainable development indicators for mining sites in protected areas: tool development, ranking and scoring of potential environmental impacts and assessment of management scenarios. J. Clean. Prod. 101, 59–70. doi:10.1016/j.jclepro.2015.03.098
Menéndez, J., Ordóñez, A., Álvarez, R., and Loredo, J. (2019). Energy from closed mines: underground energy storage and geothermal applications. Renew. Sustain. Energy Rev. 108, 498–512. doi:10.1016/j.rser.2019.04.007
Miller, D. (2008). Using aquaculture as a post-mining land use in West Virginia. Mine Water Environ. 27, 122–126. doi:10.1007/s10230-008-0038-4
Mishra, S. N., and Srivastava, R. (2022). “Climate change and responsible business leadership,” in Responsible leadership for sustainability in uncertain times: social, economic and environmental challenges for sustainable organizations (Singapore: Springer Nature Singapore), 193–209.
Muldoon, J. A. (2015). Policy networks: policy change and causal factors, A uranium mining case study. Canada: The University of Regina.
Niti Aayog (2022). Report of the inter-ministerial committee on Just transition from coal under the sustainable growth pillar of the India-us strategic clean energy partnership. https://www.niti.gov.in/sites/default/files/2022-11/Report_Just-Transition-Committee_compressed.pdf (Accessed February 6, 2024).
Odu, G. O. (2019). Weighting methods for multi-criteria decision-making technique. J. Appl. Sci. Environ. Manag. 23 (8), 1449–1457. doi:10.4314/jasem.v23i8.7
Pavloudakis, F., Roumpos, C., and Spanidis, P.-M. (2023). Planning the closure of surface coal mines based on circular economy principles. Circular Econ. Sustain. 4, 75–96. doi:10.1007/s43615-023-00278-x
Press Information Bureau (2023). Coal India ltd converts 30 mined out areas into eco-tourism destinations. 1900977. https://www.pib.gov.in/PressReleseDetailm.aspx?PRID=1900977 (Accessed February 6, 2024).
Report CER (2021). Review of the benchmark capital cost for solar PV, solar thermal and grid interactive rooftop and small solar PV plants to be applicable for FY 2021-22 and onwards till reviewed/revised by the UERC. https://cer.iitk.ac.in/odf_assets/upload_files/CER_IITK_Final_Comments_on_UERC_Benchmark_Capital_Cost_for_FY_2021_22_and_onwards.pdf (Accessed February 6, 2024).
Report CSTEP (2021). Pricing mechanism of pumped-hydro storage in India. https://cstep.in/drupal/sites/default/files/2021-06/Pumped-hydro%20policy%20brief_30June21.pdf (Accessed February 6, 2024).
Ronyastra, I. M., Saw, L. H., and Low, F. S. (2023). A review of methods for integrating risk management and multicriteria decision analysis in financial feasibility for post-coal-mining land usage selection. Resour. Policy 86, 104260. doi:10.1016/j.resourpol.2023.104260
Saaty, T. L. (2005). Theory and applications of the analytic network process: decision making with benefits, opportunities, costs, and risks. Pittsburgh, United States: RWS Publications.
Saaty, T. L. (2008). Decision making with the analytic hierarchy process. Int. J. Serv. Sci. 1 (1), 83–98. doi:10.1504/ijssci.2008.017590
Schmidt, F., Menéndez, J., Konietzky, H., Pascual-Muñoz, P., Castro, J., Loredo, J., et al. (2020). Converting closed mines into giant batteries: effects of cyclic loading on the geomechanical performance of underground compressed air energy storage systems. J. Energy Storage 32, 101882. doi:10.1016/j.est.2020.101882
Schneider, D., and Greenberg, M. R. (2023). Remediating and reusing abandoned mining sites in US metropolitan areas: raising visibility and value. Sustainability 15 (9), 7080. doi:10.3390/su15097080
Sindhu, S., Nehra, V., and Luthra, S. (2017). Investigation of feasibility study of solar farms deployment using hybrid AHP-TOPSIS analysis: case study of India. Renew. Sustain. Energy Rev. 73, 496–511. doi:10.1016/j.rser.2017.01.135
Siontorou, C. G. (2023). Fair development transition of lignite areas: key challenges and sustainability prospects. Sustainability 15 (16), 12323. doi:10.3390/su151612323
Soltanmohammadi, H., Osanloo, M., and Bazzazi, A. A. (2010). An analytical approach with a reliable logic and a ranking policy for post-mining land-use determination. Land use policy 27 (2), 364–372. doi:10.1016/j.landusepol.2009.05.001
Spanidis, P.-M., Roumpos, C., and Pavloudakis, F. (2023). Evaluation of strategies for the sustainable transformation of surface coal mines using a combined SWOT–AHP methodology. Sustainability 15 (10), 7785. doi:10.3390/su15107785
Tender document Providing project management consultancy (PMC) services for Development of Eco-parks at 09 (Nine) different Areas of CCL. https://www.centralcoalfields.in/tenderNW/admin/documents/77092.pdf.
Tzeng, G. H., and Huang, J. J. (2011). Multiple attribute decision making: methods and applications. New York, United States: CRC Press.
Keywords: mine repurposing, AHP-TOPSIS, abandoned surface coal mine, net-zero emission, sustainable mining
Citation: Singh A, Agarwal S and Prabhat A (2024) A multi-criteria decision framework to evaluate sustainable alternatives for repurposing of abandoned or closed surface coal mines. Front. Earth Sci. 12:1330217. doi: 10.3389/feart.2024.1330217
Received: 30 October 2023; Accepted: 19 March 2024;
Published: 22 April 2024.
Edited by:
Elizabeth Steyn, Western University, CanadaReviewed by:
Ying Zhu, Xi’an University of Architecture and Technology, ChinaFrancis Pavloudakis, University of Western Macedonia, Greece
Copyright © 2024 Singh, Agarwal and Prabhat. This is an open-access article distributed under the terms of the Creative Commons Attribution License (CC BY). The use, distribution or reproduction in other forums is permitted, provided the original author(s) and the copyright owner(s) are credited and that the original publication in this journal is cited, in accordance with accepted academic practice. No use, distribution or reproduction is permitted which does not comply with these terms.
*Correspondence: Siddhartha Agarwal, sagarwal@iitism.ac.in