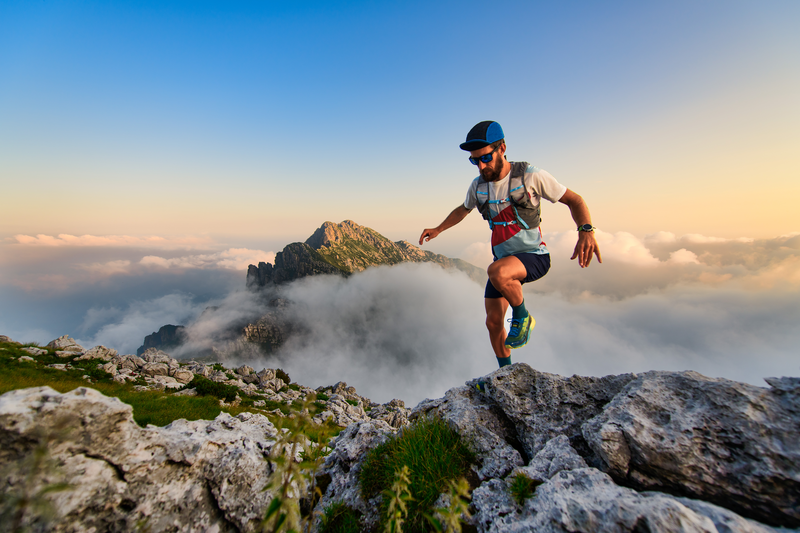
94% of researchers rate our articles as excellent or good
Learn more about the work of our research integrity team to safeguard the quality of each article we publish.
Find out more
ORIGINAL RESEARCH article
Front. Earth Sci. , 24 February 2023
Sec. Economic Geology
Volume 11 - 2023 | https://doi.org/10.3389/feart.2023.1136695
This article is part of the Research Topic Mechanics and Seepage Behaviors of Deep Reservoir Rocks View all 7 articles
With the increasing demand for natural gas, the emergency peak shaving capacity of gas storage is facing great challenges. The operation of gas storage converted from depleted oil and gas reservoir has the characteristics of strong injection and production, and alternate injection and production. However, the sand production of the reservoir makes the major gas storages face the dilemma that the injection and production capacity cannot be enhanced. In this study, the impact factors of sand production in injection-production wells of gas storage converted from depleted oil and gas reservoir were analyzed, and the sand production simulation device of gas storage was developed. Aiming at the two main controlling factors of alternating load and flow, the experimental study of quantitative sand production was conducted. The experimental results show that the alternating load has a significant weakening effect on the reservoir, and the sand production is positively correlated with the number, size and span of the alternating load exerted on the reservoir. By the method of multiple regression analysis, the parameters affecting the sand production degree of the core were obtained as follows: the upper and lower limit pressure of the alternating load, the flow rate and the number of alternating loads. The sand production map was obtained by combining the sand production amount of the core and the upper limit pressure of the alternating load to evaluate the sand production degree of the injection-production wells. In addition, at the same flow rate, the ratio of sand yield of two alternating loads stages is approximately equal to the constant, which provides a reference for the prediction of sand production in gas storage. The indoor simulation experiment of sand production in gas storage provides technical ideas and theoretical basis for the reconstruction of gas storage in Nanpu 1–29 block, and guides the selection of sand control completion methods in gas storage.
In recent years, natural gas consumption has continued to increase at a rapid rate. However, its supply and demand relationship is particularly uneven in time and space. Therefore, as a method of peak shaving and strategic reserve, gas storage is in urgent need of construction (Zheng et al., 2018; Yuan et al., 2020). By 2020, 27 gas storages putting into peak shaving operation have formed an annual peak shaving capacity of 13 billion cubic meters, and it is planned to build six gas storage centers in Northeast, North, Midwest, Northwest, Southwest and Middle East to ensure stable gas supply (Bo et al., 2003; Donghou et al., 2015; Ding and Huan, 2020). The gas storage has the characteristics of large capacity, low cost, safety and environmental protection, but compared with oil and gas production wells, sand production in injection-production wells of depleted oil and gas storage is more common (Wang et al., 2019; Wang et al., 2022a; Zhang et al., 2022). The main reason is that the operation characteristics of the gas storage of strong injection and strong production, alternate injection and production make it easier for injection-production wells to reach the sand production conditions (Liu et al., 2021; Wu et al., 2021; Yuan, 2021; Ma et al., 2022), and the sand bridge is always in a dynamic cycle of creation and destruction during the operation of gas storage. The slight sand production of injection-production wells in gas storage can enhance the reservoir production capacity, while the severe sand production may result in production reduction, abrasion of equipment, and reservoir collapse in serious cases (Wang et al., 1998; Fan et al., 2004; Yan, 2005; Dong, 2009; Zhu et al., 2011; Yang et al., 2012; Dong et al., 2022).
LUO (Luo et al., 2011) believes that formation rock failure may cause sand production in wellbore. The failure mechanism of rock can be divided into shear failure, tensile failure and bond failure. SUI (Sui et al., 2019) analyzed the experimental data of alternating load damage, it is found that the core will produce plastic and brittle deformation under alternating load, and the damage amount of the core increases with the increase of loading frequency and times. The increase of damage amount directly leads to the increase of core sand production. By analyzing the permeability variation of Shuang 6 gas storage reservoir core under different displacement pressure difference and gas water saturation, WEN (Wen, 2018) judged the amount of sand production, and concluded that both production pressure difference and reservoir water cut would increase sand production of injection-production wells. Based on the analysis of sand production from the geomechanical aspects, P.J. McLellan (McLellan et al., 2000) believed that the geomechanical aspects of sand production include the damage of bottomhole pressure to rock, the change of reservoir pressure to in-situ stress, the promoting effect of temperature on fracture development, and the pressure instability of compressible and non-Darcy fluid near wellbore. LIAO (Liao, 2021) uses the method of laboratory experiment combined with the actual production conditions of H gas storage to analyze a variety of external conditions that lead to sand production in gas storage, including injection-production flow, production pressure difference, formation pressure drop, permeability and water content. The experimental results show that the production pressure difference and permeability have a great influence on the sand production. In addition to the factors mentioned above, WANG (Wang et al., 2022b) believes that well completion methods also have a greater impact on wellbore sand production, including deviation parameters, perforation parameters and wellbore size.
According to the exsiting research, the main controlling factors of sand production, namely alternating load and injection-production flow, are still not fully understood. The existing experimental devices cannot meet the experimental conditions of high pressure and large displacement. Aiming at this issue, a simulation device for sand production in gas storage is designed and developed. Based on this device, the sand production law of cores under alternating load and flow rate is analyzed. This study draws conclusions regarding the controlling factors of sand production that provide guidance for the selection of sand control completion methods in field gas storage.
There are two main controlling factors of sand production in injection-production wells of depleted gas storage. The one is alternating load. Due to fatigue effect, the reservoir rock under alternating load is more likely to produce stress concentration causing sand production. The other is sufficiently large fluid velocity. Under the action of fluid carrying, some sand particles enter the wellbore through cracks. Therefore, it is crucial to analyze the effect of alternating load and flow rate on sand production of cores.
According to the operation characteristics of the gas storage in the period, the formation pressure increases and the bedrock stress decreases with the injection of natural gas. In the process of gas production, the formation pressure decreases and the bedrock force increases. When the rock reaches its critical failure pressure during gas production, the sand production in reservoir may occur. Therefore, we need to focus on the selection and optimization of sand control completion mode when the alternating load amplitude exceeds the critical failure pressure of the rock. The alternating load range selected in this study is lower than the critical failure pressure of the core. The experiment is designed according to the general rule of formation pressure change during the operation of the gas storage and the properties of the core.
The experimental device of sand production in gas storage shown in Figure 1 can be used to simulate the sand production in the high-temperature and high-pressure environment of depleted oil and gas reservoirs during multiple injection and production. This device can study the sand production of injection-production wells in depleted oil and gas reservoirs at different temperatures and pressures, and can realize the simulation of large displacement conditions. The device is mainly composed of gas supply system, core pressure and temperature control system, core holder, valve, sand container and electronic balance. The gas supply system consists of 1–4, for gas pressure adjustment and provide gas source; the core pressure and temperature control system is composed of 5–8, which is used to adjust the confining pressure, axial pressure and temperature of the core. The switching state of positive and negative drive controller determines whether the gas can enter the core holder; the core holder is used for fixing the core; the sand container and the electronic balance are used to collect and measure the core sand.
FIGURE 1. Diagram of sand production simulation device for gas storage (Ma et al., 2022).
The fluid flows axially from the air inlet of the core holder along the core shown in Figure 2, and the flow rate increases with the increase of inlet pressure. The core fixed in the gripper will be subjected to the confining pressure and axial pressure generated by the external liquid of the rubber sleeve under the action of the manual pressure regulating valve. The confining pressure and axial pressure can simulate the change of formation pressure during the injection and production process of the gas storage, and its value will be set according to the upper and lower operating pressures during the injection and production of the gas storage. The liquid outside the rubber sleeve is always separated from the core under the action of the sealing ring, and the core remains dry during the experiment. The sand particles carried by the gas enter the sand collecting container through the gas/sand outlet, and the sand production of each measuring point can be obtained by weighing.
As the first domestic self-developed and designed sand production experiment device of gas storage, it can show the simulation results intuitively through the method of indoor experiment. Because the upper limit pressure of the gas storage is generally high, the pressure bearing capacity of most experimental devices cannot reach the operating conditions of the gas storage. The safe working pressure and temperature of the device are 55 MPa and 120°C, respectively, which can realize the simulation of the formation environment of most gas storages. In addition to the characteristics of high-pressure and high-temperature resistance, this equipment can also simulate the sand production under the injection and production conditions of the reservoir. The device has a manual valve with confining pressure and axial pressure, which can adjust the pressure according to the upper and lower limits of the gas storage operation, and the temperature can be increased or decreased by adjusting the control knob.
In order to simulate the sand production of formation rock under alternating load during the operation of gas storage, the experimental cores are taken from Well N77 in Block Nanpu1-29 and Well G1 in Block Pugu2. The conditions of these two wells are shown in Table 1. The experimental method of thick wall cylinder was adopted, that is, drilling 10 mm through hole along the central axis of the cylindrical core sample, so that the stress distribution of the treated core is close to the reservoir fracture.
The coring well and coring depth of cores are summarized according to the experimental requirements. The length and diameter of the core are shown in Table 2. In the experiment, the thick walled cylinder method was adopted, that is, drilling 10 mm through-hole along the central axis of the cylinder core sample, so that the stress distribution of the treated core is close to that of the reservoir fracture.
Due to the strong heterogeneity of cores, it is not conducive to reveal the sand production rule to study the difference of sand production of different cores under the same experimental condition. In the following experimental scheme, the heterogeneity of the core was taken into consideration, and the sand production is considered as the reference factor for setting the experimental conditions, and it is progressiveness to set the experimental conditions from the combination of qualitative and quantitative aspects.
P0 (micro sand production pressure) is defined as the corresponding confining pressure when the cumulative sand production reaches 0.01 g; P1 (medium sand production pressure) is the corresponding confining pressure when the cumulative sand production reaches 0.03 g and the core is not damaged; The formation pressure is PP. In the experiment, we assume that the change range of the alternating load during each injection production cycle of the gas storage is half of PP, and the alternating load range imposed by the core is different as shown in Figure 3. The range of section A is Pa-Pb, and the alternating load range does not reach P0; the range of section B is Pc-P1, and the alternating load exceeds P0; Section C is a long-span alternating load with a range of 10-P1.
P0 and P1 were measured in a step-by-step pressurized manner, with the inlet pressure maintained at 1 MPa, and the confining pressure and axial pressure increased by 1–2 MPa for 2 min each time, and then the sand mass in the sand container was measured until the collected sand mass reached 0.03 g. Multi-stage small-span alternating loads are applied to core 1, 3 and 5, and single-stage large-span alternating loads are applied to core 2, 4 and 6. According to P0 and P1 values in Table 3, the application of core alternating loads is presented in Figure 4.
The cores (core 1, 3 and 5) with multi-segment and small-span alternating loads were subjected to the alternating load of section A for 30 times when the inlet pressure was 0 MPa, and then the inlet pressure was increased to 1 MPa, 1.5 MPa, 2 MPa and 2.5 MPa for 2 min, respectively. The sand production at different inlet pressure was measured and marked as measuring points 1–4. Continuing to apply B section alternating load 30 times also maintain inlet pressure at 0 MPa, and the change of inlet pressure is the same as above, again measured at different inlet pressure of sand production, marked as measuring point 5–8.
The cores (core 2, 4 and 6) of single-segment large-span alternating load is applied to the C-segment alternating load for 30 times when the inlet pressure is 0 MPa. The inlet pressure is also maintained for 2 min at the pressure of 1 MPa, 1.5 MPa, 2 MPa and 2.5 MPa. The sand production at different inlet pressures is measured and marked as measuring points 1–4.
Because of the strong heterogeneity of the core, the stress fatigue state of the core under alternating load varies greatly. The increase degree of sand production of each core under the action of alternating load varies greatly. The sand production of core 1–4 increases with the increase of external load conditions and flow, as shown in Figure 5. It can be seen that the core is more likely to produce sand under the action of alternating load, and the reservoir rock will be subjected to cyclic loading in the injection and production process of the gas storage, which causes the borehole to produce sand more easily. Compared with injection-production wells in gas storage, oil and gas production wells only have the process of fluid production. With the development, the formation pressure gradually decreases, without the influence of cyclic loading, so the possibility of sand production in the wellbore is relatively low.
Although the alternating load of section A applied by core 1 and 3 for 30 times is lower than P0, the sand production will still increase steadily with the increase of inlet pressure. Compared with the measuring point 4 without alternating load of section B, the measuring point 5 after applying the alternating load of section B for 30 times has an obvious increase in sand production. Compared with the alternating load of section A, the upper and lower limit pressures of the alternating load of section B are higher than that of section A, and the number of alternating loads has also changed from 30 to 60. It can be seen that the sand production of loose cores will increase with the increase of the number and size of alternating loads. When the alternating load is slightly lower than P0, it will also promote the sand production of the reservoir. Because the gas consumption is affected by many factors, such as climate, the gas storage in China has to carry out injection and production cycles at least twice a year. With the increase of injection and production cycles, the damage of the reservoir is getting worse and worse, thus exacerbating the sand production of the reservoir. In addition, in the design phase of the gas storage, the sand production in the wellbore can be reduced by optimizing the injection and production process of the gas storage and reducing the alternating load of the reservoir.
From the sand production curve, it can be seen that the sand production of core 2 and 4 is higher than that of 1–4 measuring points of core 1 and 3 after the single section long-span alternating load is applied, which also verifies that the sand production is positively related to the alternating load. In the process of core 5 and 6 experiments, even if the upper limit of alternating load is increased to the critical pressure value of 55 MPa of the device, no sand production is found. At this time, increasing the inlet pressure will not promote sand production.
It can be seen that the flow rate will promote sand production when the external stress reaches P0. Although the alternating load and flow rate have influence on sand production, the alternating load is the precondition of sand production. Because the limit pressure value of the test equipment is far less than P0 of core 5 and 6, the damage to the core is small, and it is difficult to reach the sand production conditions.
As shown in Figure 6, the sand production experiment results show that the damage of the core through-hole is obviously greater than that of other positions. The software simulation can also obtain the damage factor of the core under alternating load. The setting of the elastic parameters and plastic parameters of the core is shown in Table 4. After 60 times of 0–35 MPa alternating load, the damage cloud diagram of the core is obtained, as shown in Figure 7. In Figure 7, colorbar represents damage factor, the damage factor is a dimensionless quantity, and the damage factor from 0 to 1 represents no damage and complete damage.
The simulation results of the software and the results of the sand production experiment can be seen that the inner hole damage of the core is obviously greater than that of other positions. The inner hole wall of the core and the fracture section of the formation are both produced under the action of stress concentration. The experimental results directly illustrate the role of reservoir fracture section development in promoting sand production. In addition, the upper limit pressure of the alternating load applied by core 1 during the experiment is P1, and the value of P1 is less than the critical failure pressure under the initial state of the core, but the core 1 skeleton produces obvious cracks after the experiment. It can be explained that the strength of the core is continuously weakened during the alternating load process until it is lower than P1 to produce skeleton cracks.
The percentage of sand production at each measuring point in the total sand production (the sum of sand production at eight measuring points) is shown in Figure 8 by recording the sand production at eight measuring points under the multi section small span alternating load of core 1, 3 and 5. It can be clearly seen from the data in Figure 7 that with the increase of external load and inlet pressure, the proportion of sand production of each measuring point increases.
The inlet pressure conditions of measuring point X and measuring point Y of core 1 and 3 are the same compared with each other, but the application of alternating load is different (measuring point X applied alternating load of section A for 30 times, and measuring point Y applied alternating load of section A and B for 30 times respectively). Through calculation, it is found that the ratio of sand production in process X to that in process Y is close to a certain constant as shown in Table 5. The analysis results show that the ratio of the damage amount of 30 times of alternating load of section A at measuring points 1–4 to the damage amount of 30 times of alternating load of sections A and B at measuring points 5–8 is fixed, so the ratio of sand production at the same inlet pressure is the same.
Therefore, in order to facilitate the experimental operation, only the sand production of measuring points 1–5 can be measured, and the sand production of measuring points 6–8 can be calculated by formula (1). In addition, in the actual production process, when the heterogeneity of the formation is assumed to be poor, the sand production under a certain working condition can also be roughly predicted.
In the formula: GX1 is the sand production amount of measuring point X1, g; GY1 is the sand production amount of measuring point Y1, g; GYN is the sand production amount of measuring point YN, g; GXN is the sand production amount of XN at the measuring point to be calculated, g; β is a constant.
In order to discuss the influence of various experimental conditions on sand production in the sand production experiment, the method of multiple regression of data analysis software can be used to explain the correlation between the dependent variable (sand production volume) and the independent variable (upper limit pressure of alternating load, lower limit pressure of alternating load, times of alternating load, flow rate), as shown in Table 6. R is a multiple correlation coefficient, which is mainly used to judge the linear relationship between independent variables and dependent variables. The closer R2 is to 1, the better the fitting degree is. When the VIF value is greater than or equal to 10, we believe that there is a serious collinearity between variables. When the VIF value is less than 10, we believe that the data basically conforms to the assumption of multiple linear analysis; When the significance is less than 0.05, it is considered acceptable; The absolute value of the standardized coefficient indicates the influence of the independent variable on the dependent variable. It can be seen from the data that the sequence of parameters affecting the sand production degree of core is: upper and lower limit pressure of alternating load, lower limit pressure of alternating load, flow rate and times of alternating load.
By analyzing the influence of experimental conditions on sand production, it can be seen that the upper limit pressure of alternating load has the greatest influence on sand production of the core. According to the upper limit pressure of alternating load, the sand production of core is divided into four grades: no sand production range, slight sand production range, medium sand production range and large amount of sand removal range. The sand production simulation experiment of gas storage combines the alternating load upper limit pressure with the sand production volume, and the sand production range atlas is shown in Figure 9. The sand production range atlas can be used to evaluate the sand production situation on the site, providing a basis for the selection of sand control completion.
The gas reservoir of the gas storage in Block Nanpu1-29 belongs to loose sandstone, and sand production is common during the development process. Under the production mode of the gas storage, the gas injection and production flow are large and the flow rate is high, and the erosion and denudation of the sand body are more serious. The wellbore stress bears alternating loads, and the formation is more prone to produce sand and even collapse. Gravel packing sand control technology has high sand control strength and can reinforce well wall and protect screen pipe. The investigation shows that open hole gravel filling is the optimal sand control completion mode for horizontal wells in gas storage.
According to the grain size analysis data of clastic rock, the median grain size of the target layer is 199.46 μm. Particle size analysis data of sand production experiment, the median particle size of the target layer is 204.3 μm. According to the clastic rock particle size analysis data and formula (2), the gravel size is calculated. The variation range of the packed gravel size is 0.798–1.197 mm. According to the industrial gravel selection standard, the size range of the packed gravel is determined to be 20–40 mesh.
In the formula: D50 is the median grain size of filled gravel, μm; d50 is the median grain size of filled gravel, μm.
In the gravel packing sand control process, the mechanical screen pipe is used to block the gravel layer. It is required to block 100% gravel, and the accuracy is slightly less than the minimum gravel size. According to Table 7, the recommended selection range of screen pipe precision is 150–200 μm. It is found that the sand control requirements could be met during the trial injection and trial production of gas storage.
(1) Under the action of alternating load, the core is easier to produce sand, and the sand production has a positive correlation with the number and span of the alternating load. The alternating load has a significant weakening effect on the core strength, so that the alternating load slightly below P0 can also promote the sand production of the core, and the core may be damaged under the alternating load condition though the load is below critical failure pressure. When the applied stress reaches P0, the flow rate can significantly promote the sand production of the core.
(2) At the same flow rate, the ratio of sand yield of two alternating loads stages is approximately equal to the constant, which provides a reference for the prediction of sand production of gas storage.
(3) By the multiple regression analysis of the experimental data, the sequence of four experimental variables that affect the sand production degree of the core were obtained: the upper limit pressure of the alternating load, the lower limit pressure of the alternating load, the flow rate and the times of the alternating load. According to the upper limit pressure of alternating load and the sand production volume of core, the sand production atlas is drawn, which can be used to evaluate the sand production degree in the field.
(4) For the construction of gas storage in Block Nanpu1-29, the sand production of injection-production wells needs to be considered and the proper sand control measures also need to be developed. Considering the construction difficulty, sand control effect, project cost and other factors, the gravel packing is the most suitable option as the sand control method for Block Nanpu1-29. For the design of gas storage operation parameters, the effects of large flow and alternating load on the efficient and safe operation of the gas storage should be emphatically considered.
The raw data supporting the conclusions of this article will be made available by the authors, without undue reservation.
All authors listed have made a substantial, direct, and intellectual contribution to the work and approved it for publication.
Authors YZ and GY were employed by CNPC Engineering Technology R&D Company Limited. Author YQ was employed by PetroChina Jidong Oilfield Company.
The remaining authors declare that the research was conducted in the absence of any commercial or financial relationships that could be construed as a potential conflict of interest.
All claims expressed in this article are solely those of the authors and do not necessarily represent those of their affiliated organizations, or those of the publisher, the editors and the reviewers. Any product that may be evaluated in this article, or claim that may be made by its manufacturer, is not guaranteed or endorsed by the publisher.
Ding, G., and Huan, W. (2020). Review on 20 years’ UGS construction in China and the prospect[J]. Oil Gas Storage Transp. doi:10.6047/j.issn.1000-8241.2020.01.004
Dong, C. (2009). Sand control technology for oil and gas wells[M]. Beijing: China Petrochemical Press.
Dong, C., Shen, C., and Wang, L. (2022). Present progress of sanding mechanism and sand control technology in oil and gas reservoir-type underground gas storage and the future prospect[J/OL]. Oil Drill. Prod. Technol., 1–14. [2022-05-19] Available at: http://kns.cnki.net/kcms/detail/13.1072.TE.20220316.1218.002.html.
Fan, X., Li, X., and Guan, W. (2004). Review on mechanism of sand removal in China[J]. Drill. Prod. Technol. (03), 63–64+76+4. 1006–768X(2004) 03–0057–02
Li, B., Chen X., X., and Q., Yang Q. Y. (2003). The fundamentality of construction underground gas storage to solve the imbalance of gas consumption[J]. Oil Gas Storage Transp. (02), 7–10. 60-61. doi:10.3969/j.issn.1000-8241-D.2003.02.002
Liao, Wei (2021). Experimental simulation on the influencing factors of sand production in H gas storage, xinjiang, China. ACS omega 6 (47), 31727–31737. doi:10.1021/acsomega.1c04419
Liu, W., Yan, X., and Lin, H. (2021). Experimental investigation of sand production in moderately consolidated sandstones[J]. Petroleum Sci. Bull. 6 (01), 67–78. doi:10.1021/acsomega.1c04419
Luo, T., Ma, H., and Ainiwal, (2011). The numerical analysis of reasonable producing pressure drop in hutubi gas storage [J]. Sino-Global Energy 16 (06), 43–46. CNKI:SUN:SYZW.0.2011-06-010
Ma, L., Dong, J., and Wang, J. (2022). “Sand production prediction of depleted-reservoir underground gas storage - an experimental study,” in 56th US rock mechanics/geomechanics symposium (Santa Fe, United States.
McLellan, P. J., Hawkes, C. D., and Read, R. S. (2000). Sand production prediction for horizontal wells in gas storage reservoirs[C]//SPE/CIM International Conference on Horizontal Well Technology. OnePetro.
Sui, Y., Lin, T., and Liu, X. (2019). Effect of alternating load on sand production of injection-production wells in gas storage[J]. Oil Gas Storage Transp. 38 (03), 303–307. doi:10.6047/j.issn.1000-8241.2019.03.010
Wang, Q., Chao, C., and Sayiti, H. (2022). Early warning model for critical sand production in horizontal wells based on pressure monitoring: A case of H gas storage in xinjiang[J]. Xinjiang Pet. Geol. 43 (02), 214–220. doi:10.7657/XJPG20220213
Wang, Y., Gu, H., and Zhang, X. (1998). Review on sand production mechanism and sand control methods[J]. Special Oil Gas Reservoirs (04), 65–68.
Wang, Y., Jiang, H., and Yang, Y. (2022). Study on reservoir damage mechanism and protection technology of gas storage[J]. China Petroleum Chem. Stand. Qual. 42 (07), 185–187. doi:10.7657/XJPG20220213
Wang, Z., Li, W., and Liu, J. (2019). A review on state-of-the-art of underground gas storage and causes of typical accidents[J]. Hazard Control Tunn. Undergr. Eng. 1 (02), 49–58. CNKI:SUN:SDZH.0.2019-02-006
Wei, D., Dong, S., and Liang, W. (2015). Applied research of integrity management system and related technologies of underground gas storage[J]. Oil Gas Storage Transp. 34 (02), 115–121. doi:10.6047/j.issn.1000-8241.2015.02.001
Wen, H. (2018). Analysis of sand production law in the development of sandstone-type underground gas storage[J]. Petroleum Geol. Eng. 32 (04), 116–118+121+126. CNKI:SUN:SYHN.0.2018-04-032
Wu, N., Li, Y., Chen, Q., Liu, C., Jin, Y., and Tan, M. (2021). Sand production management during marine natural gas hydrate exploitation: Review and an innovative solution. Energy & Fuels 35 (6), 4617–4632. doi:10.1021/acs.energyfuels.0c03822
Yan., R.S. (2005). Research and application of high pressure pre-packed sand filling sand control technology[J]. Pet. Plan. Des. 2005 (03), 30–31+47.
Yang, X., Yan, C., and Li, F. (2012). Sand production management of gas well in loose sandstone gas reservoir[J]. Oil-Gas Field Surf. Eng. 31 (010), 86–87. doi:10.3969/j.issn.1006-6896.2012.10.045
Yuan, G. (2021). Drilling and production engineering of gas storage[M]. Beijing: Petroleum Industry Press.
Yuan, G., Yan, X., and Jin, G. (2020). Current status and development suggestions of drilling and completion technology of underground gas storage in China[J]. Pet. Drill. Tech. 48 (03), 1–7. doi:10.11911/syztjs.201704002
Zhang, Z., Chang, X. F., and Zhao, Y. (2022). Experience and enlightenment of major accidents in underground GasStorages rebuilt from depleted gas reservoir abroad [J]. J. Southwest Petroleum Univ. Technol. Ed. 44 (06), 114–120. doi:10.11885/.issn.1674-5086.2022.05.26.03
Zheng, D., Zhang, G., and Huan, W. (2018). Countermeasures and suggestions on natural gas peak shaving and supply guarantee in China[J]. Nat. Gas. Ind. 38 (4), 153–160. doi:10.3787/j.issn.1000-0976.2018.04.018
Keywords: depleted reservoir gas storage, sanding from gas storage, injection-production wells, alternating load, sand control completion
Citation: Zhang Y, Yuan G, Dong J, Liu T, Qiu Y, Wu Q, Zang C and Ding J (2023) Experimental study on sand production of depleted oil and gas reservoirs. Front. Earth Sci. 11:1136695. doi: 10.3389/feart.2023.1136695
Received: 03 January 2023; Accepted: 09 February 2023;
Published: 24 February 2023.
Edited by:
Jun Lu, Shenzhen University, ChinaReviewed by:
Jiajia Gao, Southwest Petroleum University, ChinaCopyright © 2023 Zhang, Yuan, Dong, Liu, Qiu, Wu, Zang and Ding. This is an open-access article distributed under the terms of the Creative Commons Attribution License (CC BY). The use, distribution or reproduction in other forums is permitted, provided the original author(s) and the copyright owner(s) are credited and that the original publication in this journal is cited, in accordance with accepted academic practice. No use, distribution or reproduction is permitted which does not comply with these terms.
*Correspondence: Yuda Zhang, enlkMTc3MTg0ODY5MzlAMTYzLmNvbQ==; Jingnan Dong, enlkX2RyX2NucGNAMTYzLmNvbQ==
Disclaimer: All claims expressed in this article are solely those of the authors and do not necessarily represent those of their affiliated organizations, or those of the publisher, the editors and the reviewers. Any product that may be evaluated in this article or claim that may be made by its manufacturer is not guaranteed or endorsed by the publisher.
Research integrity at Frontiers
Learn more about the work of our research integrity team to safeguard the quality of each article we publish.