- 1Shaanxi Experimental Center of Geological Survey, Shaanxi Institute of Geological Survey, Xi’an, China
- 2State Key Laboratory of Loess and Quaternary Geology, Institute of Earth Environment, Chinese Academy of Science, Xi’an, China
- 3Institute of Global Environmental Change, Xi’an Jiaotong University, Xi’an, China
- 4Shaanxi Hygrogeology Engineering Geology and Environment Geology Survey Center, Shaanxi Institute of Geological Survey, Xi’an, China
- 5Shaanxi Mineral Resources and Geological Survey, Shaanxi Institute of Geological Survey, Xi’an, China
- 6Shaanxi Geological Science and Technology Center, Shaanxi Institute of Geological Survey, Xi’an, China
- 7State Key Laboratory of Continental Dynamics, Department of Geology, Northwest University, Xi’an, China
- 8Department of Earth Sciences, University of Minnesota, Minneapolis, MN, United States
- 9Shaanxi Institute of Geological Survey, Xi’an, China
- 10Research Center of Intelligent Geological Survey, Northwestern Polytechnical University, Xi’an, China
- 11Key Laboratory of Mine Geological Hazards Mechanism and Control, Ministry of Natural Resources, Xi’an, China
The reconstruction of Asian summer monsoon (ASM) changes during the last glacial period is of great significance for better understanding monsoon dynamics. The phase relationship between the Indian summer monsoon (ISM) and East Asian summer monsoon (EASM) subsystems on different timescales is still unclear. The comparative analysis of speleothem records in the ISM region, EASM region, and central China helps to clarify the relationship between the ISM and EASM. Based on the well-dated isotope records of stalagmite DDH-B15 from the Didonghe (DDH) Cave in Hanzhong, Shaanxi, we reconstructed ASM changes during the past 34–13 thousand years before the present (kyr BP). The small average error (61 years) of 18 uranium-series ages enables a precise comparison of the stalagmite δ18O record with other well-dated records from the orbital to the millennial timescales. The δ18O signal of the DDH-B15 stalagmite is controlled by changes of the low latitude northern hemisphere summer insolation (NHSI) on the orbital timescale. It records cold Heinrich Stadial (HS) and Dansgaard–Oeschger (DO) cycles which are originated from the northern high latitude on the millennial time scale. The δ18O changes of stalagmites from the three regions are similar on the millennial and centennial timescales. But on the orbital-suborbital timescale, stalagmite δ18O changes during the last glacial cycle have different characteristics. The stalagmite δ18O values in eastern China became gradually negative, and the stalagmite δ18O values in the Indian monsoon domain showed a increasing trend, but the stalagmite δ18O values in Central China adopted an intermediate state between the EASM and ISM. Then we argued that the δ18O value of stalagmites in Central China is a mixed signal of the ISM and EASM, which indicates a change of the water vapor source as an important influence on the Chinese stalagmite δ18O record.
1 Introduction
The last glacial period is the most recent ice age of the Quaternary. Its internal climate exhibits huge fluctuations on orbital to millennial timescales (Johnsen et al., 1992; Rasmussen et al., 2014). A series of abrupt climate variations are observed on a millennial timescale, such as Heinrich Stadial (HS) and Dansgaard–Oeschger (DO) cycles. These events are characterized by rapid climate changes within decades and subsequent stability for hundreds to thousands of years (Heinrich, 1988; Alley et al., 1993; Dansgaard et al., 1993). This pattern of abrupt climate change has significance for future climate prediction (Corrick et al., 2020; Tierney et al., 2020).
The ASM includes two subsystems, the ISM and the EASM. The ASM brings abundant rainfall to the Asian continent during summer, whereas during winter, drier and cold conditions result from the Asian winter monsoon. This climate seasonality and its inter-annual variability affect the well-being of mankind in Asia which is a densely populated region (An, 2000; Wang et al., 2001a; Wang and Ding, 2008; Cheng et al., 2012; Wang et al., 2014). It is thus very important to figure out the rhythm of ASM changes. However, short-term modern meteorological observations limit our understanding of ASM dynamics, making it difficult to accurately predict long-term changes in the system. Reconstruction of the past evolutionary history of the ASM is of great significance to future monsoon predictions in the context of global warming. With the development of the uranium–thorium dating method (Edwards et al., 1987; Cheng et al., 2013), cave speleothems have become a favorable archive for tracking ASM changes through time on different timescales based on reconstructing oxygen isotope (δ18O) records (Wang et al., 2001b; Yuan et al., 2004; Cheng et al., 2016).
For example, on the glacial–interglacial timescale, dramatical sea level changes would have an important impact on the water vapor transport distance, resulting in stalagmite δ18O in the ISM region that follow glacial–interglacial cycles, while speleothem δ18O records in eastern coastal areas show smaller differences between glacial and interglacial periods, offsetting the ice volume cycle (Cai et al., 2015; Xue et al., 2019). On the precessional and millennial timescales, the signals of stalagmite δ18O in the ASM region are more consistent (Zhang et al., 2021), which roughly reflects well-integrated precipitation on the Asian continent (Cheng et al., 2016; Cheng et al., 2021), or large-scale monsoon circulation, and/or the meridional migration of monsoon circulation (Hu et al., 2019). On the centennial–decadal–interannual timescales, stalagmite δ18O is likely to represent changes in monsoon circulation, affected by the “circulation effect” (Tan, 2014), which is closely related to ENSO (Lu et al., 2021) or the position of the subtropical high (Zhao et al., 2019), and cannot be explained as changes in local rainfall. However, some stalagmites δ18O can be used for reconstruction of local rainfall on the decadal-centennial timescales, with corrections using instrumental climate data (Tan et al., 2009; Tan et al., 2018).
It is also worth noting that the paleoclimate significance of speleothem (stalagmite) δ18O requires a distinction on timescales and space scales. Stalagmite δ18O records from the Hulu Cave in the EASM region reveal that the EASM experiences significant fluctuations on a millennial timescale and is coupled with climate changes in the northern high latitudes (Wang et al., 2001b). This finding has been subsequently supported by a large number of δ18O records of Asian speleothems (Cai et al., 2006; Chen et al., 2016; Kathayat et al., 2016). Recent comparative studies on stalagmites across the globe with ice core records from Greenland also show abrupt climatic events in the ISM region, EASM region, and the South American monsoon region that responded synchronously or with lag times within the reported dating errors to climate changes in northern latitudes (Corrick et al., 2020). In addition, on the precessional timescale, the δ18O value of stalagmites in the ISM and Central/eastern China changes in phase, and 23 kyr (and 19 kyr) precessional cycles are the main signals (Cai et al., 2015; Kathayat et al., 2016; Liu et al., 2020). On the glacial–interglacial timescale, most stalagmite δ18O records in China lack an obvious 100 kyr cycle (Wang et al., 2008; Cheng et al., 2016), but monsoon proxies from the Chinese Loess Plateau do possess that cycle (Ding et al., 1995; Guo et al., 2000). Recent stalagmite δ18O studies in the ISM region show that the ISM has a significant glacial–interglacial cycle besides the precessional cycle (Cai et al., 2015; Liu et al., 2020). However, on the orbital timescale, what drives the difference between the ISM and the EASM is still controversial (Cai et al., 2015; Cheng et al., 2021).
From a spatial perspective, although most records could capture signals of precessional and millennial events, the differences in the Chinese stalagmite δ18O records from different regions are becoming apparent with the increasing number of stalagmite records. Stalagmite δ18O changes in southern and northern China are basically consistent on the millennial timescale (Wang et al., 2001b; Duan et al., 2016; Dong et al., 2018) and the precessional timescale (Cheng et al., 2016; Li et al., 2020). However, stalagmite δ18O in southern China may have 100 kyr cycles but not in northern China (Liu et al., 2022), and as Zhang et al. (2022) argues, during the MIS 3, a distinct precessional cycle is found in stalagmite δ18O of the Yangzi Cave (Wu et al., 2020) and Yongxing Cave (Chen et al., 2016) but not from the Hulu Cave (Wang et al., 2001b), which may be caused by the increased contribution from spring rainfall to the stalagmite δ18O in southeast China, and there are regional differences in stalagmite δ18O on the centennial timescale (Tan et al., 2009). During the Holocene, there are asynchronous changes of stalagmite δ18O in different caves when the Holocene Optimum terminated, early in the low latitude and late in the higher latitude (Cai et al., 2010). Therefore, timescales and regional differences should be considered when interpreting stalagmite δ18O. To understand this aspect, it is important to compare stalagmites δ18O recording different endmember signals (the ISM region and EASM region), as well as samples from the region affected by both monsoon regimes, and analyze how they vary on different timescales.
We analyzed the δ18O signal in a U-Th dated speleothem from the DDH Cave located in the Shaanxi province to understand the variability of monsoon changes during the 34–13 kyr BP. The DDH Cave is located in the region influenced by the two monsoon subsystems, recording valuable information of the complexity of the full ASM system during part of the last glacial cycle. We compared our results with other paleoclimate records on the orbital-suborbital time scales and identified the millennial timescale events in this period. We further analyzed the structure of those millennial events and discussed the variability of the monsoon during the LGM. Our study aimed to explore the controls of the ASM variability on the orbital to the millennial timescales and investigated the regional differences in speleothem δ18O records.
2 Cave Location Settings and Sample
Didonghe (DDH) Cave (32°44′14″N, 106°24′26″E, 1,262 m), located in Ningqiang County, Shaanxi province, China (Figure 1), is a karst cave of the Chanjiayan Tiankeng (sinkhole) system. The DDH Cave formed in the Permian Wujiaping limestone formation. It was overlain by the Permian Dalong shale and underlain by the Permian Wangpo shale (Luo et al., 2019). The stalagmite sample DDH-B15 was collected from the inner chamber of the cave, 2 km from the cave entrance. The inner chamber is closed, with high humidity and a stable sedimentation environment. Dripping water can be seen in the cave, and a large number of stalagmites, stalactites, flow stones, and other speleothems are developed there.
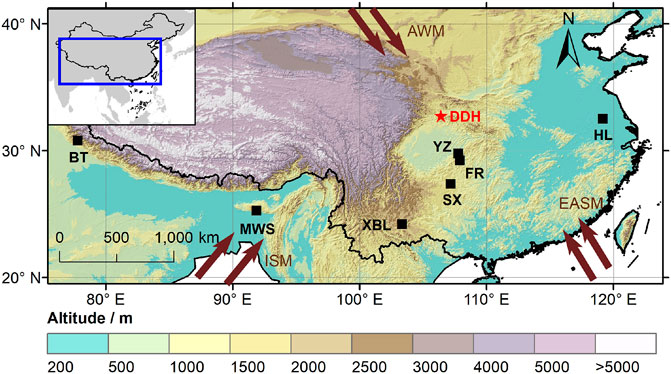
FIGURE 1. Locations of speleothem records during the Last Glacial in the Asian monsoon region. (DDH, this study; YZ, Yangzi Cave, Wu et al., 2020; FR, Furon Cave, Li et al., 2021; SX, Sanxing Cave, Jiang et al., 2014; XBL, Xiaobailong Cave, Cai et al., 2015; NWS, Mawmluh Cave, Dutt et al., 2015; BT, Bitto Cave, Kathayat et al., 2016; HL, Hulu Cave, Wang et al., 2001b). The blown arrows indicate the Indian summer monsoon (ISM), the East Asian summer monsoon (EASM), and the Asian winter monsoon (AWM).
The study site is located in the southern Qinling Mountains, in Central China, where both the southwest ISM and EASM affect the climate (Figure 1). The climate is rainy and hot at the same time. In summer, southerly winds bring warm monsoon precipitation from low latitudes; in winter, northerly winds provide cold and dry air mass. According to meteorological monitoring records of the Ningqiang County Meteorological Station from 1957 to 2007, the average annual temperature in the Ningqiang area is 13°C, the average annual rainfall is 1,104 mm, and precipitation falls mainly during the summer monsoon season from June to September (Hu et al., 2010).
The sample used in this study has been briefly described in the works of Chen et al. (2020). The stalagmite was collected from the Yulong chamber at the deepest part of Cave B in the DDH Cave system. The speleothems were scattered on the ground during collection, possibly due to human activity or earthquake/cave collapse events. The DDH-B15 sample is 158 mm long, the bottom diameter is 64 mm at the widest part, and the top is about 30 mm in diameter. After cutting the stalagmite by using a diamond wire saw, the center of the sample was observed to be yellowish-brown, and the two sides were yellowish-white, with some cracks. In this study, we mainly considered the yellowish-brown central part to avoid the possible influence of pinch-out rocks on both sides on the climatic information. The growth axis of the stalagmite persists steadily, with only a few minor changes (Figure 2A). The stalagmite begins to gradually shift to the right about 40 mm from the top; the stalagmite grows vertically during the 40–120 mm portion, indicating a stable drip position; from 120 to 150 mm, the stalagmite growth shifts to the right likely due to a change in the karst seepage system.
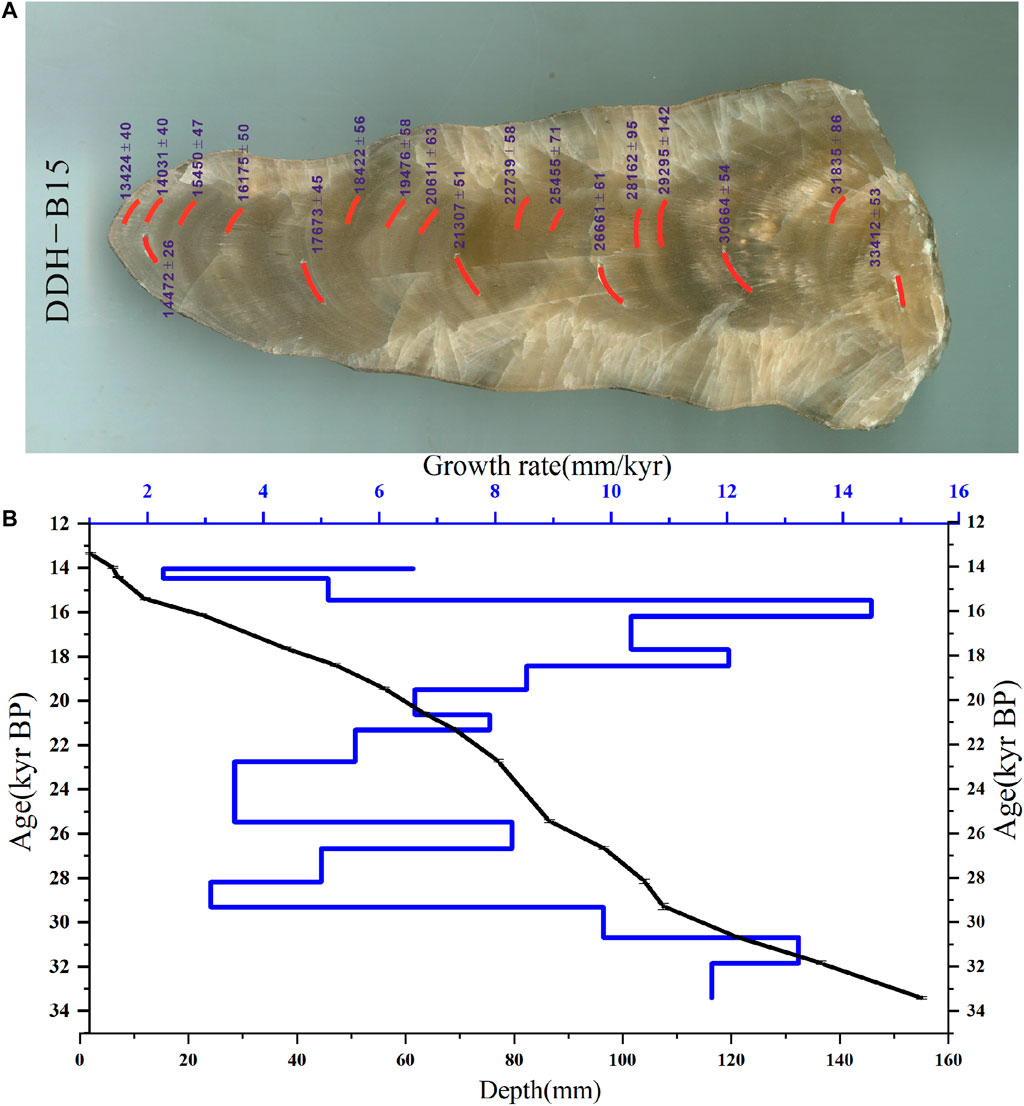
FIGURE 2. DDH-B15 stalagmite and age model. (A), the polished section of the DDH-B15 stalagmite and U-series ages (dark blue numbers) are presented beside the drilling positions (red). (B), age model of DDH-B15 with linear interpolation (black) and the growth rate of the stalagmite (blue).
3 Methods
A dental burr was used to obtain 30–60 mg powder samples along the growth axis of DDH-B15. A total of 18 powdered subsamples were used to perform 230Th-234U-238U age tests using a multi-cup inductively coupled plasma mass spectrometer (MC-ICP-MS) at the Isotope Laboratory, Xi’an Jiaotong University. We used a standard chemical pretreatment protocol (Edwards et al., 1987; Shen et al., 2002) before the test. A triple spike (229Th-233U-236U) with known isotope concentrations was employed to determine the U-Th isotope ratio in the samples. We referred to Cheng et al. (2013) for a detailed description about the instrument, standardization, and half-lives. The U-Th ages are corrected according to 230Th/232Th = 4.4 ± 2.2 × 10−6 (atomic ratio) to deduct the influence of initial thorium, and all the dating errors are given at the 2σ level. Among them, the two top and bottom U-Th ages have been reported in Chen et al. (2020). In this study, 16 new U-Th ages have been tested and are listed as follows (Table 1).
Along the growth axis of DDH-B15, 322 sets of powder subsamples for carbon and oxygen isotope (δ13C and δ18O) analyses were drilled by a dental burr at an even interval of 0.5 mm. All δ13C and δ18O measurements were carried out at the Institute of Earth Environment, Chinese Academy of Sciences, and the test instrument was an Isoprime100 gas source stable isotope ratio mass spectrometer equipped with a MultiPrep system. Generally, a laboratory internal standard TB1 is placed between every 15 samples to monitor the stability of the instrument, and one sample out of every 10–20 samples is randomly selected to verify its repeatability. The δ13C and δ18O test results are given according to the VPDB standard. The precision for δ18O and δ13C measurements of standard materials is better than 0.15‰ and 0.12‰ (2σ).
4 Results
4.1 U-Th Age Model
Including the top and bottom ages (14.5 kyr BP at 7 mm and 33.3 kyr BP at 155 mm) reported by Chen et al. (2020), 18 U-Th high-precision ages were obtained (Table 1). The uranium concentration of the DDH-B15 stalagmite ranges from 757.4 to 1,245.8 parts per billion (ppb), with an average of 1,007.8 ppb, which is a relatively high uranium content for calcite stalagmites. The content of Th depends mainly on the 232Th content. The range of 232Th is 74-3917 parts per trillion (ppt), and the average value is 514ppt. The range of 230Th/232Th is 3302-258162 (atomic ratio), and its average value is 111828. Compared with the initial 230Th/232Th value of 4.4 ± 2.2 (atomic ratio), the correction error caused by the initial thorium does not exceed 1‰, which can be ignored. δ234UInitial varies between 4,434‰ and 4,744‰, with an average value of 4,575‰. The obtained 18 U-Th ages are between 33.4 kyr BP and 13.4 kyr BP, with errors between 26 and 142 years, and the average error being 61 years (the average relative error is 2.76‰). All ages are in stratigraphic order, and linear interpolation was used to establish a chronological model. The age model results show that the growth period of the stalagmite is from 33.97 to 13.12 kyr BP (Figure 2B). The growth rate of DDH-B15 is relatively stable (2.3–14.5 mm/kyr), and the average growth rate is 7.89 mm/kyr. The stalagmite grew fast during 34–29 kyr BP and 22–16 kyr BP but slowly in other periods (Figure 2B).
4.2 DDH-B15 δ18O and δ13C Time Series
Using the chronological scale established earlier, a δ18O time series was constructed for the DDH-B15 stalagmite (Figure 3). The average temporal resolution of δ18O series is 64.8 years during 33.97–13.12 kyr BP, which can be used to study climate variability on centennial–millennial–suborbital timescales. DDH-B15 δ18O values vary from −11‰ to −5.6‰, with an overall increasing trend from 34 kyr BP to 16 kyr BP, and rapidly shift to negative values after 15 kyr BP. The entire δ18O sequence is interrupted by three significant positive events, roughly at 16 kyr BP, 24 kyr BP, and 29.5 kyr BP. In addition, among several significant positive events, the δ18O values of 34–30 kyr BP, 29–26 kyr BP, and 21–17 kyr BP were relatively negative. Among them, the δ18O values during the 34–30 kyr BP period is the most negative, which are ∼5‰ more negative than the most positive value observed at 16 kyr BP. Furthermore, during 21–17 kyr BP, δ18O series values grasp an obvious negative event, which is also reflected in records from other stalagmites in the ASM region (Wang et al., 2001b; Cai et al., 2010; Jiang et al., 2014).
DDH-B15 δ13C values fluctuate between −7.73‰ and −1.16‰. While we observe a significant long-term increasing trend of δ18O, the δ13C values show only a slight positive trend (Figure 3) on the orbital timescale. On the millennial timescale, δ13C and δ18O show similar characteristics at some excursions of enriched isotope events but also with some differences. For example, during 21–17 kyr BP, δ13C does not have a significant depleted value as seen in δ18O; while during 17–15 kyr BP, δ13C exhibits large fluctuations and tends to be negative but not positive. Subsequent to 15 kyr BP, the positive trend of δ13C is gradually reestablished. However, during the period of 34–31 kyr BP, δ18O only shows a modest (∼1‰) oscillation, while δ13C shifts up to 4.5‰. δ18O has a long-term trend, with an overall variation range of about 5.5‰, while δ13C has no obvious trend on the suborbital timescale, and its millennial signal varies by as much as 6.5‰. This suggests that δ13C is more sensitive to climate change than δ18O on the millennial timescale.
5 Discussion
5.1 Interpretation of Stalagmite δ18O and δ13C of the DDH Cave
Before interpreting the climatic significance of stalagmite δ18O of the DDH Cave, it is necessary to judge whether the stalagmite calcite was deposited under an isotopic equilibrium. Recently, the most widely used equilibrium fractionation test is the replication of δ18O records from different stalagmites in the same cave or from different caves in the same climate region (Dorale et al., 1998; Wang et al., 2001b; Dorale and Liu 2009; Li et al., 2019). Comparing the δ18O records of the DDH stalagmite with other contemporaneous stalagmite records in the ASM region, it is observed that the DDH stalagmite δ18O record is consistent with that of the Hulu Cave (Wang et al., 2001b) and Yangzi Cave (Wu et al., 2020) and that major millennial timescale events are well recorded, such as HS1 at 16 kyr BP, HS2 at 24 kyr BP, HS3 at 29.5 kyr BP, and seven DO events (Figure 4). The correlation coefficient between the DDH stalagmite δ18O and Hulu stalagmite δ18O is 0.76 (N = 349 and p < 0.001), and the correlation coefficient of the Yangzi stalagmite δ18O is 0.83 (N = 349 and p < 0.001). Therefore, we have reason to assume that the DDH-B15 stalagmite was deposited under (near-)equilibrium fractionation conditions, and the influence of kinetic fractionation is negligible.
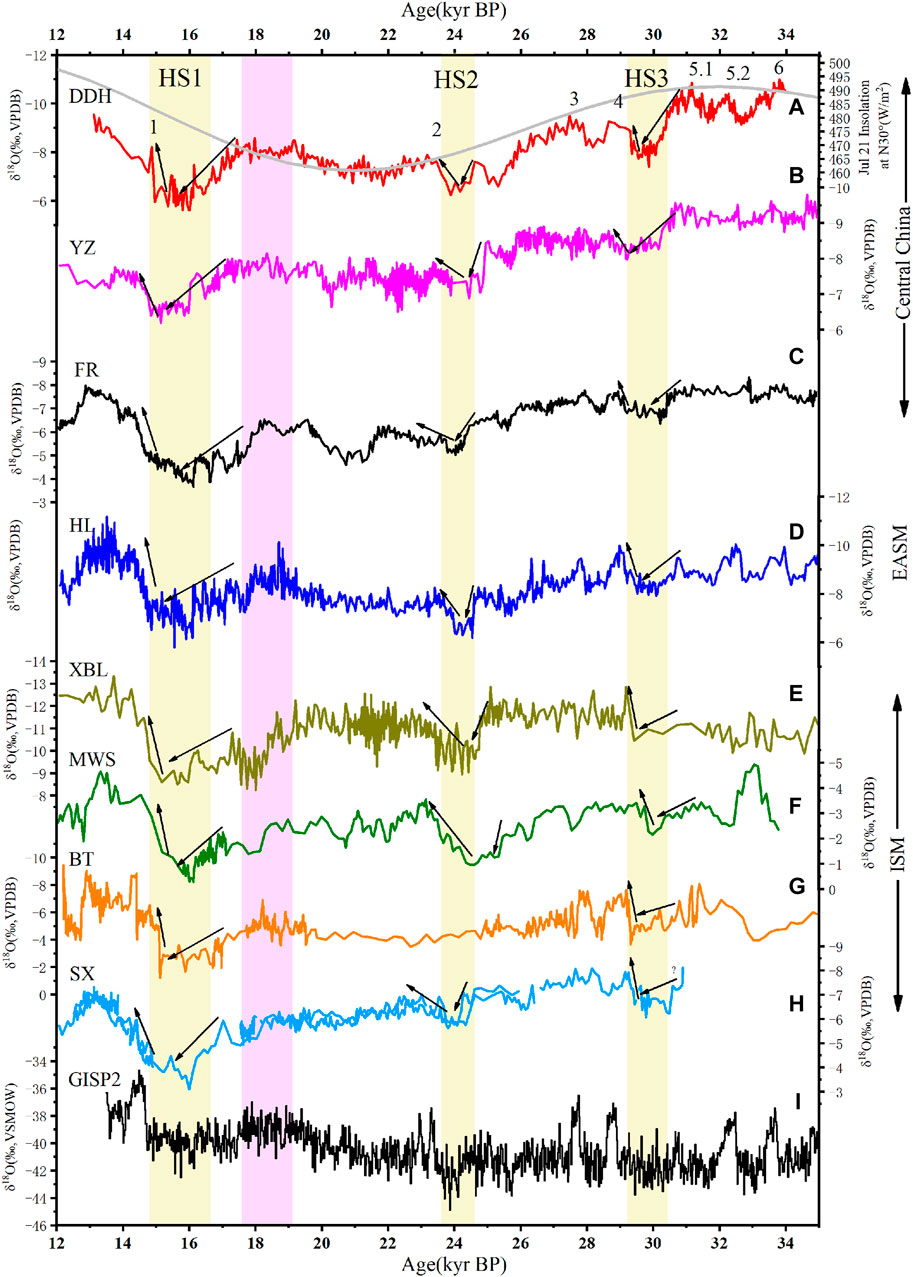
FIGURE 4. Comparison of DDH speleothem δ18O records (Central China) with other paleoclimate records (EASM and ISM regions). a, DDH Cave, this study; b, Yangzi Cave (Wu et al., 2020); c, Furon Cave (Li et al., 2021); d, Hulu Cave (Wang et al., 2001b);e, Xiaobailong Cave (Cai et al., 2015); f, Mawmluh Cave (Dutt et al., 2015); g, Bitto Cave (Kathayat et al., 2016);h, Sanxing Cave (Jiang et al., 2014); and k, Greenland ice core (Rasmussen et al., 2014). Shaded in pink are monsoon intensification events during the LGM, and the yellow bars are three HS events.
The stalagmite δ18O is mainly controlled by the cave temperature and drip δ18O changes (Lachniet, 2009). The DDH-B15 δ18O variation is 5.5‰. The δ18O fractionation coefficient (−0.23‰/°C, O'Neil et al., 1969) caused by the temperature is very small. The 34–13 kyr BP belongs to the MIS3-2 stage, when the global average temperature did not change in the region by more than 4°C (Peterse et al., 2014). Indeed, an 8°C change between glacial and interglacial cycles in the Loess Plateau (Peterse et al., 2014) only implies a maximum temperature contribution around 1.8‰. Therefore, we can interpret the DDH-B15 stalagmite δ18O values as indicators of precipitation δ18O.
The climatic significance of the Chinese stalagmite δ18O is controversial (Maher, 2008; Pausata et al., 2011; Liu et al., 2020). In the ASM region, especially in eastern China, the stalagmite δ18O values are variably affected by many factors. Early studies had shown that the amount of rainfall was not significantly negatively correlated with δ18O values (Wang et al., 2001b). At the same time, Cai et al. (2001) had proposed that different moisture sources may affect stalagmite δ18O values. Wang et al. (2001a) used the ratio of summer/winter rainfall to explain the δ18O of stalagmites and introduced the concept of “monsoon intensity.” Cheng et al. (2009, 2016, 2021) followed and extended the concept of “monsoon intensity” and believed that the lower stalagmite δ18O mainly represented the higher spatially integrated monsoon rainfall between the tropical monsoon sources and reflected “wind” characteristics of the monsoon. Yuan et al. (2004) believed that the stalagmite δ18O record was dominated by Rayleigh distillation processes associated with the water vapor transport, that is, the “rainout effect” or “continental effect”. Subsequent work on paleoclimate records and simulations also basically supported these two interpretations (Hu et al., 2008; Pausata et al., 2011; Liu et al., 2014; Hu et al., 2019). The contribution of the rainout effect and the proportion of summer rainfall remain uncertain (Orland et al., 2009; Liang et al., 2020). Chinese stalagmite δ18O records are very consistent on precessional-millennial timescales (Wang et al., 2001b; Cheng et al., 2009). At present, δ18O is still the most effective stalagmite paleoclimate proxy. Most researchers approve that δ18O can be an indicator of monsoon rainfall in northern China or the Asian continent.
The DDH cave is located on the southern slope of the Qinling Mountains, about 500 km away from the Sanbao Cave and Linzhu Cave (Cheng et al., 2016). The DDH stalagmite δ18O is very similar to the compositive stalagmite δ18O-based records from the Sanbao–Dongge–Hulu–Linzhu Cave (Figure 4), and the long-scale changes of the DDH stalagmite δ18O are consistent with summer insolation changes in the northern hemisphere. Recent EASM reconstruction by the loess microcodium δ18O presented an opportunity to reconcile the paradox between speleothems and loess, which showed that the dominated signal of the EASM was precession but the ice volume influenced the amplitude. The loess microcodium δ18O consisted of speleothem δ18O on precessional and millennial scales (Zhang et al., 2022). This also reveals that the speleothem δ18O can be used as a monsoon tensity index. In addition, the stalagmite δ18O in the Xianglong Cave, 20 km away from the DDH Cave, was consistent with the local rainfall on the decadal timescale (Tan et al., 2015, 2018). The DDH stalagmite δ18O should show similar disciplinary. Considering the aforementioned data, the “monsoon intensity” concept is followed (Cheng et al., 2016); we argue that the δ18O of the DDH stalagmite reflects monsoon intensity changes and integrated rainfall changes on a large spatial scale. When the stalagmite δ18O is negative, the ASM rain belt is pushed northward, the rainfall in north China increases, and the rainfall in the south may be different (Cheng et al., 2016, 2019). On a shorter timescale, it is affected by local hydrology and climate. There may be some differences between the stalagmite δ18O and rainfall index. δ13C may represent changes of the local hydroclimate because when precipitation increases, the proportion of C3 vegetation increases, vegetation coverage becomes larger, and the water–rock interaction (WRI) time becomes shorter, which will make δ13C more negative (Dorale et al., 1992; Baker et al., 1997). The relationship between δ13C and δ18O on different timescales may represent the relationship between local precipitation and Asian monsoon intensity. They are not identical on the orbital scale but have certain similarities on the millennial scale (Figure 4). But stalagmite δ13C is also affected by temperature, cave ventilation, prior calcite precipitation (PCP) process, atmospheric CO2, and other factors (Fairchild et al., 2006; Genty et al., 2006). Therefore, it is necessary to further study the DDH stalagmite δ13C combined with other hydrological proxies, but it is not discussed here. In the following sections, we focused on the changes of our DDH stalagmite δ18O on multiple timescales and regional differences.
5.2 Characteristics of Orbital-Suborbital and Abrupt Events Recorded by the DDH Stalagmite δ18O During the Last Glacial
On the orbital-suborbital timescales, the δ18O of the DDH stalagmite is consistent with other caves and is mainly controlled by summer insolation in the northern hemisphere (Figure 4). The overall trend of the DDH stalagmite δ18O is similar to the Yangzi Cave (Wu et al., 2020) and the Hulu Cave (Wang et al., 2001b) stalagmite δ18O; however, during the period of 34–30 kyr BP, the Chinese cave records also showed some differences. The DDH δ18O changes from a negative value to a positive value, while the Hulu Cave stalagmite δ18O is relatively stable, with no significantly persistent positive trends during 34–18 kyr BP. In addition, the Hulu cave δ18O values during the 19–17 kyr BP period are nearly as negative as those observed in the 34–30 kyr BP period (Figure 4). The stalagmite δ18O values from the Xiaobailong Cave in Yunnan (Cai et al., 2015) were relatively stable or even gradually negative during the 34–20 kyr BP period. Mawmluh Cave (Dutt et al., 2015) and Bitto Cave (Kathayat et al., 2016) from the ISM region also share similar characteristics, which may be influenced by the low time resolution of the record, or be a characteristic in the ISM region. Therefore, further research is needed for verification. The significant negative δ18O values of the DDH Cave and the Yangzi Cave during the 34–30 kyr BP period may be related to a stronger summer insolation. However, the Hulu Cave stalagmite δ18O values are not closely related to insolation during this period.
On the millennial timescale, the DDH stalagmite δ18O record shows seven warm DO cycles (Figure 4A). The DO1 event, which is the Bolling event, occurred at 15–14 kyr BP, DO2 occurred at 23.5–23 kyr BP, DO3 occurred at 28–27.5 kyr BP, DO4 occurred at 29.2–28.6 kyr BP, DO5.1 occurred at 31.5–30.5 kyr BP, DO5.2 occurred at 32.5–32 kyr BP, and DO6 occurred at 34–33.5 kyr BP. The seven DO events in our records are temporally consistent with the Greenland ice core, but there are some differences in the structure. The onsets of these DO warm events are not very rapid, which are similar to other stalagmites in the Asian monsoon region, but not as rapid as the warming shift recorded by the arctic ice core, which may be influenced by the climate of the southern hemisphere (Cai et al., 2006; Rohling et al., 2009). In addition, the DDH stalagmite δ18O and other stalagmites δ18O records from the ASM region also record three significant HS cold events on the millennial timescale (Figure 4). HS1 occurred at 17–15 kyr BP, HS2 occurred at 24.5–23.5 kyr BP, and HS3 occurred at 30.5–29.2 kyr BP. As described by Li et al. (2019), two phases of weak monsoon occurred in the Central China at the onset of the HS1 cold event. The stalagmite records in the ISM area also exhibit a two-phase structure (Figure 4). In addition, at the end of HS1, the DDH record and the Hulu Cave, Xiaobailong Cave, Sanxing Cave, and others also showed a two-stage process of monsoon enhancement. In general, this two-stage enhancement process can be regarded as a multi-cycle slow enhancement process. Four internal turbulent events at the onset of HS1 can be found in the Greenland ice core, Hulu Cave, and DDH records. The onset and termination of HS2 and HS3 events from high latitudes may also have multi-stage structures, but the performance is not obvious in DDH and other stalagmite records, which may be attributed to duration or time resolution limitations (Figure 4).
Comparing the DDH stalagmite δ18O record with other stalagmite δ18O records, it is found that the three HS events do not have exactly the same structural characteristics. Generally speaking, HS1 and HS3 events are characterized by slow entry and rapid termination. This mode has similar characteristics to the glacial–interglacial cycle, which may be related to the “binge-purge” dynamic mechanism of ice sheet evolution (MacAyeal, 1993), while HS2 and Holocene millennial events (such as 4.2 and 8.2 kyr) mainly enter quickly and terminate slowly (Liu et al., 2018). This implies that the HS1 and HS3 events of the glacial period are different from the Bond events of the interglacial period (Liu et al., 2018), and the structure of the HS2 event is different from the other two HS events.
Considering the aforementioned characteristics together, we found that precessional and millennial signals are the main portrait of the ASM, and low latitude summer insolation is the driving force of the ASM which is coupled with high-latitude climate changes on the millennial scale. However, there are some differences in the stalagmite δ18O of different caves on the suborbital scale, and there are also some differences in the structure of different HS events.
5.3 Periodic Characteristics of DDH Stalagmite δ18O Changes
In order to assess the dominant period of the DDH stalagmite δ18O, we first used REDFIT software (Schulz and Mudelsee, 2002) to analyze the δ18O signal. It is found that the DDH stalagmite δ18O has significant periods of 555, 206, and 142 years (Figure 5), consistent with the main periods observed in the Holocene Dongge Cave δ18O record (Wang et al., 2005; Dykoski et al., 2005). These periodicities are close to significant periods (512, 206, and 148 years) of the △14C record (Stuiver and Braziunas, 1993). Therefore, 555, 206, and 142 years are the main periods associated with solar activity. It is observed that 206 years is the Suess period of solar activity and 555 years may be the significant 500-year period of the ASM (Xu et al., 2019), Greenland temperature (Stuiver et al., 1995), and ENSO variability (Zhu et al., 2017). These periods support the idea that solar activities are responsible for changes of glacial ASM intensity.
For distinguishing the variability of the DDH stalagmite δ18O on different timescales, the EEMD method (Wu and Huang, 2009) is employed to decompose it. At the same time, we also compared the variability of the stalagmite δ18O from the ISM region and the EASM region on different timescales. The Hulu Cave and XBL Cave with high resolution δ18O and an accurate age model are selected as representatives of the EASM and ISM. Interpolating the three records to a resolution of 30 years, we used the EEMD method to decompose the DDH stalagmite δ18O into nine components, including the long trend. These nine components extend the period from orbital to suborbital, millennial, and centennial time scales (Figure 6). IMF C7-C9 (Figure 6B) are reconstructed to form a new component of the orbital-suborbital timescale, which should be dominated by precessional signals (Yuan et al., 2004; Wang et al., 2008); IMF C4-6 (Figure 6C) are reconstructed to form a millennial time scale component, including cycles of 5,000, 2000, and 1,000 years, which are consistent with the northern high latitude temperature changes (Bond et al., 1999; Wang et al., 2008); IMF C1-3 (Figure 6D) are reconstructed to obtain cycles below the millennial time scale, which are mainly on the centennial time scale, corresponding to 200- and 500-year cycles of solar activity.
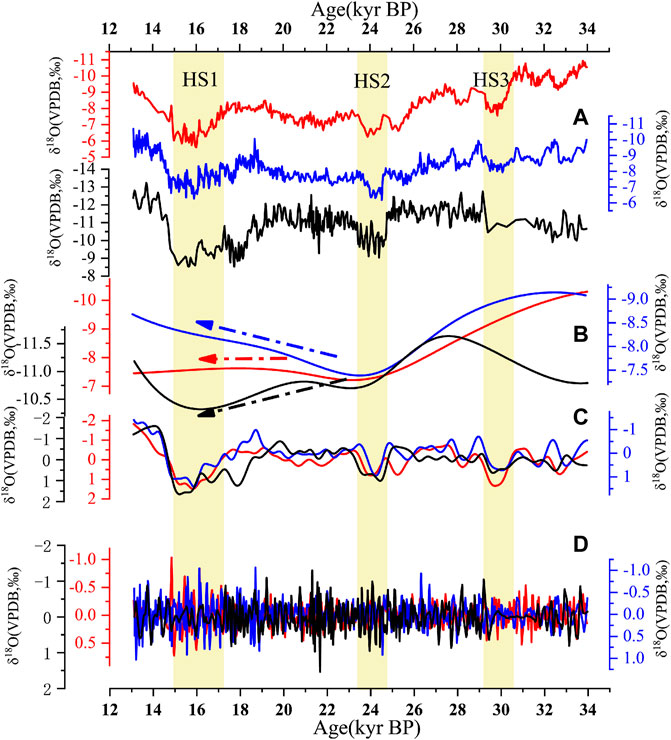
FIGURE 6. a, Speleothem δ18O of the DDH Cave (red, Central China), Hulu Cave (blue, EASM), and Xiaobailong Cave (black, ISM); b, IMF 7-9 component of the speleothem δ18O in the DDH Cave, Hulu Cave, and Xiaobailong Cave, which are mainly the cycles of suborbital-orbital periods; c, IMF C4-6 component of speleothem δ18O in the DDH Cave, Hulu Cave, and Xiaobailong Cave, which are mainly millennial cycles; d, IMF C1-3 component of the speleothem δ18O in the DDH Cave, Hulu Cave, and Xiaobailong Cave, which are mainly centennial cycles.
On the three different timescales, the stalagmite δ18O records of the three regions have similarities and differences. On the centennial (Figure 6D) and millennial (Figure 6C) timescales, stalagmite δ18O changes in the three regions are almost the same, and their amplitude and frequency are similar. On the millennial scale, three HS events can be clearly identified. All these show that the climate of the ASM region (EASM, ISM, and Central China) changes contemporaneously, within the age error range on the centennial and the millennial timescale. In addition, millennial and centennial variability both show an increasing trend since 19 kyr BP. This may be associated with the abnormally dry and cold HS1 event or deglaciation on the millennial–centennial time scales, which could be verified by long high-resolution records. On the suborbital time scale (Figure 6B), stalagmite δ18O records in the three regions show three trends during the 24–16 kyr BP period. The Hulu Cave stalagmite δ18O values in eastern China become gradually negative, consistent with the more positive nature of HS2, as compared to HS1. The Xiaobailong Cave δ18O values from the ISM region gradually become more positive, while the DDH stalagmite δ18O in Central China adopts an intermediate state between the EASM and ISM, further supporting the idea that the precipitation in Central China during the 24–19 kyr BP period was a mixture of water vapor from the Indian Ocean and the Pacific Ocean. This is probably caused by a decline in the sea level during the glacial period, while the ISM region was mainly influenced by ice volume (Cai et al., 2015; Xue et al., 2019). This difference on the orbital to sub-orbital timescale indicates that ASM rainfall isotope data also have regional differences, although precession is the main driver.
6 Conclusion
The DDH stalagmite δ18O records from Shaanxi established by high-precision uranium dating have been used to reconstruct the Asian summer monsoon evolution history of 34–13 kyr BP. It is found that DDH stalagmite δ18O records and those from other ASM regions are broadly consistent on orbital and millennial timescales. The DDH stalagmite δ18O is controlled by low latitude summer insolation changes in the northern hemisphere on orbital timescale and responds to millennial climate changes from the northern high latitudes. Several cold HS events and DO climate cycles have been recorded. Spectrum analysis shows that the δ18O of the DDH stalagmite has characteristic cycles of 500, 206, and 142 years, indicating that the ASM during the last glaical period is regulated in part by solar activity. Multi-scale EEMD decomposition shows that the stalagmite δ18O in the three regions (ISM, EASM and Central China) changes consistently on millennial and centenary timescales, but there are also three characteristics for each region during the glacial period on the suborbital timescale, which is likely to be caused by changes in regional sea level differences. This underscores the important influence of water vapor sources on the Chinese stalagmite δ18O.
Data Availability Statement
The raw data supporting the conclusion of this article will be made available by the authors, without undue reservation.
Author Contributions
ZH supervised and managed the project. XC, YC and QC designed the research and experiments. XC, QC, GX, YC, QL, JZ, LT, YH, JR, PW, YW and YZ participated in field work and collected samples. XC, GX, HC and RE performed the experiments. XC, QC, YC, GX and JZ carried out data analysis. QC, XC and YC wrote and reviewed the manuscript.
Funding
This research was supported by the National Natural Science Foundation of China grants (41901096), the Strategic Priority Research Program of Chinese Academy of Sciences (Grant No. XDB 40010200), the Key R & D project of the Science and Technology Department of Shaanxi Province (2017ZDXM-SF-27-1), the Natural Science Foundation of Shaanxi Province general project (2020JQ-975), the Opening Fund of State Key Laboratory of Loess and Quaternary Geology (SKLLQG 1704 and SKLLQG 1803), the commonweal geological survey project of Shaanxi Province (202008, 201902, and 201916), and the National Natural Science Foundation of China grants (41888101).
Conflict of Interest
The authors declare that the research was conducted in the absence of any commercial or financial relationships that could be construed as a potential conflict of interest.
Publisher’s Note
All claims expressed in this article are solely those of the authors and do not necessarily represent those of their affiliated organizations, or those of the publisher, the editors, and the reviewers. Any product that may be evaluated in this article, or claim that may be made by its manufacturer, is not guaranteed or endorsed by the publisher.
Acknowledgments
We acknowledge Le Ma for helping with the stable isotope measurements, Yanbin Lu and David Domínguez-Villar for reviewing the manuscript, George S. Burr for improving our English, and Yingying Wei and Jinhu Ren for the field work.
References
Alley, R. B., Meese, D. A., Shuman, C. A., Gow, A. J., Taylor, K. C., Grootes, P. M., White, J. W. C., Ram, M., Waddington, E. D., Mayewski, P. A., and Zielinski, G. A. (1993). Abrupt Increase in Greenland Snow Accumulation at the End of the Younger Dryas Event. Nature 362 (6420), 527–529. doi:10.1038/362527a0
An, Z. (2000). The History and Variability of the East Asian Paleomonsoon Climate. Quat. Sci. Rev. 19 (1-5), 171–187. doi:10.1016/s0277-3791(99)00060-8
Baker, A., Ito, E., Smart, P. L., and McEwan, R. F. (1997). Elevated and Variable Values of 13C in Speleothems in a British Cave System. Chem. Geology. 136 (3-4), 263–270. doi:10.1016/S0009-2541(96)00129-5
Bond, G. C., Showers, W., Elliot, M., Evans, M., Lotti, R., Hajdas, I., et al. (1999). The North Atlantic's 1-2 Kyr Climate Rhythm: Relation to Heinrich Events, Dansgaard/Oeschger Cycles and the Little Ice Age. Geophys. Monograph-American Geophys. Union 112, 35–58. doi:10.1029/gm112p0035
Cai, Y., An, Z., Cheng, H., Edwards, R. L., Kelly, M. J., Liu, W., et al. (2006). High-resolution Absolute-Dated Indian Monsoon Record between 53 and 36 Ka from Xiaobailong Cave, Southwestern China. Geol 34 (8), 621–624. doi:10.1130/G22567.1
Cai, Y., Fung, I. Y., Edwards, R. L., An, Z., Cheng, H., Lee, J.-E., et al. (2015). Variability of Stalagmite-Inferred Indian Monsoon Precipitation over the Past 252,000 Y. Proc. Natl. Acad. Sci. U.S.A. 112 (10), 2954–2959. doi:10.1073/pnas.1424035112
Cai, Y., Tan, L., Cheng, H., An, Z., Edwards, R. L., Kelly, M. J., et al. (2010). The Variation of Summer Monsoon Precipitation in central China since the Last Deglaciation. Earth Planet. Sci. Lett. 291 (1-4), 21–31. doi:10.1016/j.epsl.2009.12.039
Cai, Y., Zhang, M., Peng, Z., Lin, Y., An, Z., Zhang, Z., et al. (2001). The δ18O Variation of a Stalagmite from Qixing Cave, Guizhou Province and Indicated Climate Change during the Holocene. Chin.Sci.Bull. 46 (22), 1904–1908. doi:10.1007/bf02901169
Chen, Q., Hong, Z., Luo, Q., Tang, L., Wang, P., Hu, Y., et al. (2020). Last Glacial Paleoclimate Record of Stalagmite Trace Elements from Didonghe Cave in Hanzhong. J. Xi’an Univ. (Natural Sci. Edition) 23 (3), 92–102.
Chen, S., Wang, Y., Cheng, H., Edwards, R. L., Wang, X., Kong, X., et al. (2016). Strong Coupling of Asian Monsoon and Antarctic Climates on Sub-orbital Timescales. Sci. Rep. 6 (1), 1–7. doi:10.1038/srep32995
Cheng, H., Edwards, R. L., Broecker, W. S., Denton, G. H., Kong, X., Wang, Y., et al. (2009). Ice Age Terminations. science 326 (5950), 248–252. doi:10.1126/science.1177840
Cheng, H., Edwards, R. L., Sinha, A., Spötl, C., Yi, L., Chen, S., et al. (2016). The Asian Monsoon over the Past 640,000 Years and Ice Age Terminations. nature 534 (7609), 640–646. doi:10.1038/nature18591
Cheng, H., Lawrence Edwards, R., Shen, C.-C., Polyak, V. J., Asmerom, Y., Woodhead, J., et al. (2013). Improvements in 230Th Dating, 230Th and 234U Half-Life Values, and U-Th Isotopic Measurements by Multi-Collector Inductively Coupled Plasma Mass Spectrometry. Earth Planet. Sci. Lett. 371-372, 82–91. doi:10.1016/j.epsl.2013.04.006
Cheng, H., Sinha, A., Wang, X., Cruz, F. W., and Edwards, R. L. (2012). The Global Paleomonsoon as Seen through Speleothem Records from Asia and the Americas. Clim. Dyn. 39 (5), 1045–1062. doi:10.1007/s00382-012-1363-7
Cheng, H., Zhang, H., Cai, Y., Shi, Z., Yi, L., Deng, C., et al. (2021). Orbital-scale Asian Summer Monsoon Variations: Paradox and Exploration. Sci. China Earth Sci. 64, 529–544. doi:10.1007/s11430-020-9720-y
Corrick, E. C., Drysdale, R. N., Hellstrom, J. C., Capron, E., Rasmussen, S. O., Zhang, X., et al. (2020). Synchronous Timing of Abrupt Climate Changes during the Last Glacial Period. Science 369 (6506), 963–969. doi:10.1126/science.aay5538
Dansgaard, W., Johnsen, S. J., Clausen, H. B., Dahl-Jensen, D., Gundestrup, N. S., Hammer, C. U., et al. (1993). Evidence for General Instability of Past Climate from a 250-kyr Ice-Core Record. nature 364 (6434), 218–220. doi:10.1038/364218a0
Ding, Z., Liu, T., Rutter, N. W., Yu, Z., Guo, Z., and Zhu, R. (1995). Ice-volume Forcing of East Asian winter Monsoon Variations in the Past 800,000 Years. Quat. Res. 44 (2), 149–159. doi:10.1006/qres.1995.1059
Dong, J., Shen, C.-C., Kong, X., Wang, Y., and Duan, F. (2018). Asian Monsoon Dynamics at Dansgaard/Oeschger Events 14-8 and Heinrich Events 5-4 in Northern China. Quat. Geochronol. 47, 72–80. doi:10.1016/j.quageo.2018.05.012
Dorale, J. A., Edwards, R., Ito, E., and González, L. (1998). Climate and Vegetation History of the Midcontinent from 75 to 25 ka: A Speleothem Record from Crevice Cave, Missouri, USA. Science 282 (5395), 1871–1874. doi:10.1126/science.282.5395.1871
Dorale, J. A., González, L. A., Reagan, M. K., Pickett, D. A., Murrell, M. T., and Baker, R. G. (1992). A High-Resolution Record of Holocene Climate Change in Speleothem Calcite from Cold Water Cave, Northeast Iowa. Science 258 (5088), 1626–1630. doi:10.1126/science.258.5088.1626
Dorale, J., and Liu, Z. (2009). Limitations of Hendy Test Criteria in Judging the Paleoclimatic Suitability of Speleothems and the Need for Replication. J. cave karst Stud. 71 (1), 73–80.
Duan, W., Cheng, H., Tan, M., and Edwards, R. L. (2016). Onset and Duration of Transitions into Greenland Interstadials 15.2 and 14 in Northern China Constrained by an Annually Laminated Stalagmite. Sci. Rep. 6 (1), 1–6. doi:10.1038/srep20844
Dutt, S., Gupta, A. K., Clemens, S. C., Cheng, H., Singh, R. K., Kathayat, G., et al. (2015). Abrupt Changes in Indian Summer Monsoon Strength during 33,800 to 5500 Years B.P. Geophys. Res. Lett. 42 (13), 5526–5532. doi:10.1002/2015GL064015
Dykoski, C. A., Edwards, R. L., Cheng, H., Yuan, D., Cai, Y., Zhang, M., et al. (2005). A High-Resolution, Absolute-Dated Holocene and Deglacial Asian Monsoon Record From Dongge Cave, China. Earth & Planet. Sci. Lett. 233 (1–2), 71–86. doi:10.1016/j.epsl.2005.01.036
Edwards, R. L., Chen, J. H., and Wasserburg, G. J. (1987). 238U-234U-230Th-232Th Systematics and the Precise Measurement of Time over the Past 500,000 Years. Earth Planet. Sci. Lett. 81 (2-3), 175–192. doi:10.1016/0012-821x(87)90154-3
Fairchild, I. J., Smith, C. L., Baker, A., Fuller, L., Spötl, C., Mattey, D., et al. (2006). Modification and Preservation of Environmental Signals in Speleothems. Earth-Science Rev. 75 (1-4), 105–153. doi:10.1016/j.earscirev.2005.08.003
Genty, D., Blamart, D., Ghaleb, B., Plagnes, V., Causse, C., Bakalowicz, M., et al. (2006). Timing and Dynamics of the Last Deglaciation From European and North African δ13C Stalagmite Profiles-Comparison with Chinese and South Hemisphere Stalagmites. Quat. Sci. Rev. 25 (17–18), 2118–2142. doi:10.1016/j.quascirev.2006.01.030
Guo, Z., Biscaye, P., Wei, L., Chen, X., Peng, S., and Liu, T. (2000). Summer Monsoon Variations over the Last 1.2 Ma from the Weathering of Loess-Soil Sequences in China. Geophys. Res. Lett. 27 (12), 1751–1754. doi:10.1029/1999GL008419
Heinrich, H. (1988). Origin and Consequences of Cyclic Ice Rafting in the Northeast Atlantic Ocean during the Past 130,000 Years. Quat. Res. 29 (2), 142–152. doi:10.1016/0033-5894(88)90057-9
Hu, C., Henderson, G. M., Huang, J., Xie, S., Sun, Y., and Johnson, K. R. (2008). Quantification of Holocene Asian Monsoon Rainfall from Spatially Separated Cave Records. Earth Planet. Sci. Lett. 266 (3-4), 221–232. doi:10.1016/j.epsl.2007.10.015
Hu, J., Emile‐Geay, J., Tabor, C., Nusbaumer, J., and Partin, J. (2019). Deciphering Oxygen Isotope Records from Chinese Speleothems with an Isotope‐Enabled Climate Model. Paleoceanography and Paleoclimatology 34 (12), 2098–2112. doi:10.1029/2019PA003741
Hu, J., Lu, X., and Su, J. (2010). Analysis on Climatic Characteristics of Ningqiang County in Recent 51 Years. J. Shaanxi Meteorology 3, 18–21.
Jaffey, A. H., Flynn, K. F., Glendenin, L. E., Bentley, W. C., and Essling, A. M. (1971). Precision Measurement of Half-Lives and Specific Activities ofU235andU238. Phys. Rev. C 4 (5), 1889–1906. doi:10.1103/PhysRevC.4.1889
Jiang, X., He, Y., Shen, C.-C., Lee, S.-Y., Yang, B., Lin, K., et al. (2014). Decoupling of the East Asian Summer Monsoon and Indian Summer Monsoon between 20 and 17 Ka. Quat. Res. 82 (1), 146–153. doi:10.1016/j.yqres.2014.05.001
Johnsen, S. J., Clausen, H. B., Dansgaard, W., Fuhrer, K., Gundestrup, N., Hammer, C. U., et al. (1992). Irregular Glacial Interstadials Recorded in a New Greenland Ice Core. Nature 359 (6393), 311–313. doi:10.1038/359311a0
Kathayat, G., Cheng, H., Sinha, A., Spötl, C., Edwards, R. L., Zhang, H., et al. (2016). Indian Monsoon Variability on Millennial-Orbital Timescales. Sci. Rep. 6 (1), 1–7. doi:10.1038/srep24374
Lachniet, M. S. (2009). Climatic and Environmental Controls on Speleothem Oxygen-Isotope Values. Quat. Sci. Rev. 28 (5-6), 412–432. doi:10.1016/j.quascirev.2008.10.021
Li, D., Tan, L., Cai, Y., Jiang, X., Ma, L., Cheng, H., et al. (2019). Is Chinese Stalagmite δ18O Solely Controlled by the Indian Summer Monsoon? Clim. Dyn. 53 (5), 2969–2983. doi:10.1007/s00382-019-04671-x
Li, T.-Y., Wu, Y., Shen, C.-C., Li, J.-Y., Chiang, H.-W., Lin, K., et al. (2021). High Precise Dating on the Variation of the Asian Summer Monsoon since 37 Ka BP. Sci. Rep. 11 (1), 1–14. doi:10.1038/s41598-021-88597-7
Li, Y., Rao, Z., Xu, Q., Zhang, S., Liu, X., Wang, Z., et al. (2020). Inter-relationship and Environmental Significance of Stalagmite δ13C and δ18O Records from Zhenzhu Cave, north China, over the Last 130 Ka. Earth Planet. Sci. Lett. 536, 116149. doi:10.1016/j.epsl.2020.116149
Liang, Y., Zhao, K., Edwards, R. L., Wang, Y., Shao, Q., Zhang, Z., et al. (2020). East Asian Monsoon Changes Early in the Last Deglaciation and Insights into the Interpretation of Oxygen Isotope Changes in the Chinese Stalagmite Record. Quat. Sci. Rev. 250, 106699. doi:10.1016/j.quascirev.2020.106699
Liu, D., Wang, Y., Cheng, H., Edwards, R. L., Kong, X., Chen, S., et al. (2018). Contrasting Patterns in Abrupt Asian Summer Monsoon Changes in the Last Glacial Period and the Holocene. Paleoceanography and Paleoclimatology 33 (2), 214–226. doi:10.1002/2017PA003294
Liu, G., Li, X., Chiang, H.-W., Cheng, H., Yuan, S., Chawchai, S., et al. (2020). On the Glacial-Interglacial Variability of the Asian Monsoon in Speleothem δ18O Records. Sci. Adv. 6 (7), eaay8189. doi:10.1126/sciadv.aay8189
Liu, S., Liu, D., Wang, Y., Zou, L., and Gao, H. (2022). Spatio-temporal Expressions of Precessional-Scale Stalagmite δ18O Variations from the Asian Monsoon Area. Palaeogeogr. Palaeoclimatol. Palaeoecol. 585, 110720. doi:10.1016/j.palaeo.2021.110720
Liu, Z., Wen, X., Brady, E. C., Otto-Bliesner, B., Yu, G., Lu, H., ., , Cheng, H., Wang, Y., Zheng, W., Ding, Y., Edwards, R. L., Cheng, J., Liu, W., and Yang, H. (2014). Chinese Cave Records and the East Asia Summer Monsoon. Quat. Sci. Rev. 83, 115–128. doi:10.1016/j.quascirev.2013.10.021
Lu, J., Zhang, H., Li, H., Sha, L., Zhao, J., Li, Y., et al. (2021). A 120-year Seasonally Resolved Speleothem Record of Precipitation Seasonality from southeastern China. Quat. Sci. Rev. 264, 107023. doi:10.1016/j.quascirev.2021.107023
Luo, Q., Zhang, J., Li, Y., Yin, Z., Tang, L., Wang, P., et al. (2019). Geologic Conditions and Distribution Features of Tiankeng Groups in Hanzhong, Shaanxi Province. CARSOLOGICA SINICA 38 (2), 281–291. doi:10.11932/karst20190214
MacAyeal, D. R. (1993). Binge/purge Oscillations of the Laurentide Ice Sheet as a Cause of the North Atlantic's Heinrich Events. Paleoceanography 8 (6), 775–784. doi:10.1029/93PA02200
Maher, B. A. (2008). Holocene Variability of the East Asian Summer Monsoon from Chinese Cave Records: a Re-assessment. The Holocene 18 (6), 861–866. doi:10.1177/0959683608095569
O'Neil, J. R., Clayton, R. N., and Mayeda, T. K. (1969). Oxygen Isotope Fractionation in Divalent Metal Carbonates. J. Chem. Phys. 51 (12), 5547–5558. doi:10.1063/1.1671982
Orland, I. J., Bar-Matthews, M., Kita, N. T., Ayalon, A., Matthews, A., and Valley, J. W. (2009). Climate Deterioration in the Eastern Mediterranean as Revealed by Ion Microprobe Analysis of a Speleothem that Grew from 2.2 to 0.9 Ka in Soreq Cave, Israel. Quat. Res. 71 (1), 27–35. doi:10.1016/j.yqres.2008.08.005
Pausata, F. S. R., Battisti, D. S., Nisancioglu, K. H., and Bitz, C. M. (2011). Chinese Stalagmite δ18O Controlled by Changes in the Indian Monsoon during a Simulated Heinrich Event. Nat. Geosci 4 (7), 474–480. doi:10.1038/ngeo1169
Peterse, F., Martínez-García, A., Zhou, B., Beets, C. J., Prins, M. A., Zheng, H., et al. (2014). Molecular Records of continental Air Temperature and Monsoon Precipitation Variability in East Asia Spanning the Past 130,000 Years. Quat. Sci. Rev. 83, 76–82. doi:10.1016/j.quascirev.2013.11.001
Rasmussen, S. O., Bigler, M., Blockley, S. P., Blunier, T., Buchardt, S. L., Clausen, H. B., ., , Cvijanovic, I., Dahl-Jensen, D., Johnsen, S. J., Fischer, H., Gkinis, V., Guillevic, M., Hoek, W. Z., Lowe, J. J., Pedro, J. B., Popp, T., Seierstad, I. K., Steffensen, J. P., Svensson, A. M., Vallelonga, P., Vinther, B. M., Walker, M. J. C., Wheatley, J. J., and Winstrup, M. (2014). A Stratigraphic Framework for Abrupt Climatic Changes during the Last Glacial Period Based on Three Synchronized Greenland Ice-Core Records: Refining and Extending the INTIMATE Event Stratigraphy. Quat. Sci. Rev. 106, 14–28. doi:10.1016/j.quascirev.2014.09.007
Rohling, E., Liu, Q., Roberts, A., Stanford, J., Rasmussen, S., Langen, P., et al. (2009). Controls on the East Asian Monsoon during the Last Glacial Cycle, Based on Comparison between Hulu Cave and Polar Ice-Core Records. Quat. Sci. Rev. 28 (27-28), 3291–3302. doi:10.1016/j.quascirev.2009.09.007
Schulz, M., and Mudelsee, M. (2002). REDFIT: Estimating Red-Noise Spectra Directly from Unevenly Spaced Paleoclimatic Time Series. Comput. Geosciences 28 (3), 421–426. doi:10.1016/S0098-3004(01)00044-9
Shen, C., Edwards, R., Cheng, H., Dorale, J., Thomas, R., Moran, S., ., , and Edmonds, H. N. (2002). Uranium and Thorium Isotopic and Concentration Measurements by Magnetic Sector Inductively Coupled Plasma Mass Spectrometry. Chem. Geology. 185 (3-4), 165–178. doi:10.1016/S0009-2541(01)00404-1
Stuiver, M., and Braziunas, T. F. (1993). Sun, Ocean, Climate and Atmospheric 14CO2: an Evaluation of Causal and Spectral Relationships. The Holocene 3 (4), 289–305. doi:10.1177/095968369300300401
Stuiver, M., Grootes, P. M., and Braziunas, T. F. (1995). The GISP2 δ18O Climate Record of the Past 16,500 Years and the Role of the Sun, Ocean, and Volcanoes. Quat. Res. 44 (3), 341–354. doi:10.1006/qres.1995.1079
Tan, L., Cai, Y., Cheng, H., Edwards, L. R., Gao, Y., Xu, H., et al. (2018). Centennial-to Decadal-Scale Monsoon Precipitation Variations in the Upper Hanjiang River Region, China over the Past 6650 Years. Earth Planet. Sci. Lett. 482, 580–590. doi:10.1016/j.epsl.2017.11.044
Tan, L., Cai, Y., Cheng, H., Edwards, R. L., Shen, C. C., Gao, Y., et al. (2015). Climate Significance of Speleothem δ18O from central China on Decadal Timescale. J. Asian Earth Sci. 106, 150–155. doi:10.1016/j.jseaes.2015.03.008
Tan, L., Cai, Y., Cheng, H., An, Z., and Edwards, R. L. (2009). Summer Monsoon Precipitation Variations in central China over the Past 750 Years Derived from a High-Resolution Absolute-Dated Stalagmite. Palaeogeogr. Palaeoclimatol. Palaeoecol. 280 (3-4), 432–439. doi:10.1016/j.palaeo.2009.06.030
Tan, M. (2014). Circulation Effect: Response of Precipitation δ18O to the ENSO Cycle in Monsoon Regions of China. Clim. Dyn. 42 (3-4), 1067–1077. doi:10.1007/s00382-013-1732-x
Tierney, J. E., Poulsen, C. J., Montañez, I. P., Bhattacharya, T., Feng, R., Ford, H. L., ., , Hönisch, B., Inglis, G. N., Petersen, S. V., Sagoo, N., Tabor, C. R., Thirumalai, K., Zhu, J., Burls, N. J., Foster, G. L., Goddéris, Y., Huber, B. T., Ivany, L. C., Kirtland Turner, S., Lunt, D. J., McElwain, J. C., Mills, B. J. W., Otto-Bliesner, B. L., Ridgwell, A., and Zhang, Y. G. (2020). Past Climates Inform Our Future. Science 370 (6517). doi:10.1126/science.aay3701
Wang, B., and Ding, Q. (2008). Global Monsoon: Dominant Mode of Annual Variation in the Tropics. Dyn. Atmospheres Oceans 44 (3-4), 165–183. doi:10.1016/j.dynatmoce.2007.05.002
Wang, B., Wu, R., and Lau, K.-M. (2001a). Interannual Variability of the Asian Summer Monsoon: Contrasts between the Indian and the Western North Pacific-East Asian Monsoons*. J. Clim. 14 (20), 4073–4090. doi:10.1175/1520-0442(2001)014<4073:ivotas>2.0.co;2
Wang, P. X., Wang, B., Cheng, H., Fasullo, J., Guo, Z. T., Kiefer, T., et al. (2014). The Global Monsoon across Timescales: Coherent Variability of Regional Monsoons. Clim. Past 10 (6), 2007–2052. doi:10.5194/cp-10-2007-2014
Wang, Y., Cheng, H., Edwards, R. L., He, Y., Kong, X., An, Z., et al. (2005). The Holocene Asian Monsoon: Links to Solar Changes and North Atlantic Climate. Science 308 (5723), 854–857. doi:10.1126/science.1106296
Wang, Y., Cheng, H., Edwards, R. L., Kong, X., Shao, X., Chen, S., et al. (2008). Millennial- and Orbital-Scale Changes in the East Asian Monsoon over the Past 224,000 Years. Nature 451 (7182), 1090–1093. doi:10.1038/nature06692
Wang, Y. J., Cheng, H., Edwards, R. L., An, Z. S., Wu, J. Y., Shen, C.-C., et al. (2001b). A High-Resolution Absolute-Dated Late Pleistocene Monsoon Record from Hulu Cave, China. Science 294 (5550), 2345–2348. doi:10.1126/science.1064618
Wu, Y., Li, T.-Y., Yu, T.-L., Shen, C.-C., Chen, C.-J., Zhang, J., et al. (2020). Variation of the Asian Summer Monsoon since the Last Glacial-Interglacial Recorded in a Stalagmite from Southwest China. Quat. Sci. Rev. 234, 106261. doi:10.1016/j.quascirev.2020.106261
Wu, Z., and Huang, N. E. (2009). Ensemble Empirical Mode Decomposition: a Noise-Assisted Data Analysis Method. Adv. Adapt. Data Anal. 01 (01), 1–41. doi:10.1142/S1793536909000047
Xu, D., Lu, H., Chu, G., Liu, L., Shen, C., Li, F., et al. (2019). Synchronous 500-year Oscillations of Monsoon Climate and Human Activity in Northeast Asia. Nat. Commun. 10 (1), 1–10. doi:10.1038/s41467-019-12138-0
Xue, G., Cai, Y., Ma, L., Cheng, X., Cheng, H., Edwards, R. L., et al. (2019). A New Speleothem Record of the Penultimate Deglacial: Insights into Spatial Variability and Centennial-Scale Instabilities of East Asian Monsoon. Quat. Sci. Rev. 210, 113–124. doi:10.1016/j.quascirev.2019.02.023
Yuan, D., Cheng, H., Edwards, R. L., Dykoski, C. A., Kelly, M. J., Zhang, M., et al. (2004). Timing, Duration, and Transitions of the Last Interglacial Asian Monsoon. Science 304 (5670), 575–578. doi:10.1126/science.1091220
Zhang, H., Zhang, X., Cai, Y., Sinha, A., Spötl, C., Baker, J., et al. (2021). A Data-Model Comparison Pinpoints Holocene Spatiotemporal Pattern of East Asian Summer Monsoon. Quat. Sci. Rev. 261, 106911. doi:10.1016/j.quascirev.2021.106911
Zhang, Z., Li, G., Cai, Y., Cheng, X., Sun, Y., Zhao, J., et al. (2022). Millennial‐Scale Monsoon Variability Modulated by Low‐Latitude Insolation during the Last Glaciation. Geophys. Res. Lett. 49, e2021GL096773. doi:10.1029/2021GL096773
Zhao, J., Cheng, H., Yang, Y., Tan, L., Spötl, C., Ning, Y., et al. (2019). Reconstructing the Western Boundary Variability of the Western Pacific Subtropical High over the Past 200 Years via Chinese Cave Oxygen Isotope Records. Clim. Dyn. 52 (5), 3741–3757. doi:10.1007/s00382-018-4456-0
Keywords: speleothem, last glacial, Indian summer monsoon, East Asian summer monsoon, time scales, Chinese stalagmite δ18O
Citation: Chen Q, Cheng X, Cai Y, Luo Q, Zhang J, Tang L, Hu Y, Ren J, Wang P, Wang Y, Zhang Y, Xue G, Zhou J, Cheng H, Edwards RL and Hong Z (2022) Asian Summer Monsoon Changes Inferred From a Stalagmite δ18O Record in Central China During the Last Glacial Period. Front. Earth Sci. 10:863829. doi: 10.3389/feart.2022.863829
Received: 27 January 2022; Accepted: 16 March 2022;
Published: 14 April 2022.
Edited by:
Yu Li, Lanzhou University, ChinaReviewed by:
Selvaraj Kandasamy, Xiamen University, ChinaAmzad Hussain Laskar, Physical Research Laboratory, India
Copyright © 2022 Chen, Cheng, Cai, Luo, Zhang, Tang, Hu, Ren, Wang, Wang, Zhang, Xue, Zhou, Cheng, Edwards and Hong. This is an open-access article distributed under the terms of the Creative Commons Attribution License (CC BY). The use, distribution or reproduction in other forums is permitted, provided the original author(s) and the copyright owner(s) are credited and that the original publication in this journal is cited, in accordance with accepted academic practice. No use, distribution or reproduction is permitted which does not comply with these terms.
*Correspondence: Zenglin Hong, bGhxaHpsQDE2My5jb20=
†These authors have contributed equally to this work and share first authorship