- 1Research Institute of Petroleum Exploration and Development, PetroChina, Beijing, China
- 2Petroleum Exploration and Production Research Institute, SINOPEC, Beijing, China
The Sichuan Basin, covering an area of 180 × 103 km2, has the following advantages in natural gas geology: The sedimentary rocks are 6,000–12,000 m thick with high maturity of source rocks, and nine sets of primary gas source rocks are developed in the basin with a gas–oil ratio of 80:1, and thus it is a gas basin. The remaining recoverable reserves of conventional and unconventional natural gas are up to 13.6404 × 1012 m3. Multiple gas-bearing systems are developed with 25 conventional and tight oil and gas producing layers and 135 discovered gas fields, and the total proved geological reserves and cumulative production of natural gas by the end of 2019 were 5.7966 × 1012 m3 and 648.8 × 109 m3, respectively. The CO2 components and the correlation with relevant parameters for 243 samples from 22 gas fields indicate that CO2 in the Sichuan Basin display the following two characteristics: (1) Relatively low CO2 content of 0.02%–22.90% with an average of 2.96%, which guaranteed the commerciality of natural gas exploration and production; (2) cratonic CO2, which is characterized by low CO2 contents (<5%) and low R/Ra ratios (<0.24). According to the δ13CCO2 values and the relationship with R/Ra, δ13C1, CO2 contents, and wetness coefficient (W) for 263 gas samples, the δ13CCO2 values display three characteristics: (1) The highest δ13CCO2 value (10.4‰) in China is found in the Fuling shale gas field, which extends the interval values from previous −39‰–7‰ to −39‰–10.4‰. (2) The δ13CCO2 values can be applied to identify the CO2 origin of natural gas in the Sichuan Basin: type A, organic origin from thermal decomposition of organic matter, with an average δ13CCO2 value of −12.8‰ and average wetness coefficient of 7.8% for 44 samples; type B, organic origin from thermal cracking of organic matter, with an average δ13CCO2 value of −15.7‰ and average wetness coefficient of 1.30% for 34 samples; type C, inorganic origin from thermal decomposition or organic acid dissolution of carbonate rocks or minerals, with an average δ13CCO2 value of −1.8‰ and average wetness coefficient of 0.85% for 175 samples. (3) δ13CCO2>δ13CCH4. This is a common characteristic shared by all geological age (from Z2dn to J2s) gas reservoirs and various gas types (coal-derived gas, oil-associated gas, and shale gas).
1 Introduction
The Sichuan Basin is a large superimposed basin developed on the basis of craton, with an area of about 180 × 103 km2. The basin has developed sedimentary rocks with a thickness of 6,000–12,000 m. It is a basin with the most developed source rock series in China, especially gas source rocks (nine sets) due to its high thermal maturity (Figure 1), which makes it a basin enriched in both conventional and unconventional gas resources. The remaining recoverable resources of conventional and unconventional natural gas amounted to 13.6404 × 1012 m3 (Li et al., 2019). By the end of 2019, the total proved geological reserves of the basin had reached 5.7966 × 1012 m3. The cumulative gas production is 648.8 × 109 m3, but the cumulative oil production is very low, 7.296 × 106 t, so the gas oil equivalent ratio is up to 80:1 (Dai et al., 2021). The basin has many gas-bearing layers and they overlap to form multiple gas-bearing systems, including 25 conventional and tight oil and gas producing layers (18 marine facies) and two shale gas producing layers (Figure 1). It is the basin with the most industrial oil and gas layers found so far in China (Dai et al., 2018; Dai, 2019). By the end of 2019, 135 gas fields had been discovered in the basin (Figure 2). There are 27 large gas fields with reserves more than 30.0 × 109 m3, among which Anyue gas field is the largest. Anyue gas field is also the largest carbonate gas field in China, with a proved geological reserve of 1.1709 × 1012 m3 and a gas production of 12.013 × 109 m3 by the end of 2019 (Dai et al., 2021). In the 13th century, the Sichuan Basin developed the world’s first gas field—Ziliujing gas field (Meyerhoff, 1970; Dai, 1981). Fryklund and Stark (2020) pointed out that when the cumulative gas production exceeded five billion barrels of oil equivalent (793.166 × 109 m3 gas), sedimentary basins with remaining recoverable resources of at least five billion barrels of oil equivalent were regarded as super basins, which are called tier-one super basins. If it is slightly lower than these two indicators, it is called a tier-two super basin. Accordingly, since the remaining recoverable resources in the Sichuan Basin are 13.6404 × 1012 m3, exceeding the index value of 793.166 × 109 m3, while its cumulative gas production is 656.9 × 109 m3, slightly lower than the index cumulative gas production, it can only be regarded as a tier-two super basin. Recently, Dai et al. (2021) claimed that according to the percentage of oil and gas in the cumulative total production, the oil and gas fields with an oil or gas ratio of 20–80% should be regarded as super oil and gas basins. Most super basins in the world fall into this class. When the proportion of oil is greater than 80%, it is called a super oil basin; when the proportion of gas exceeds 80%, it is called a super gas basin. The proportion of gas in the Sichuan Basin is 98.76% (Dai et al., 2021).
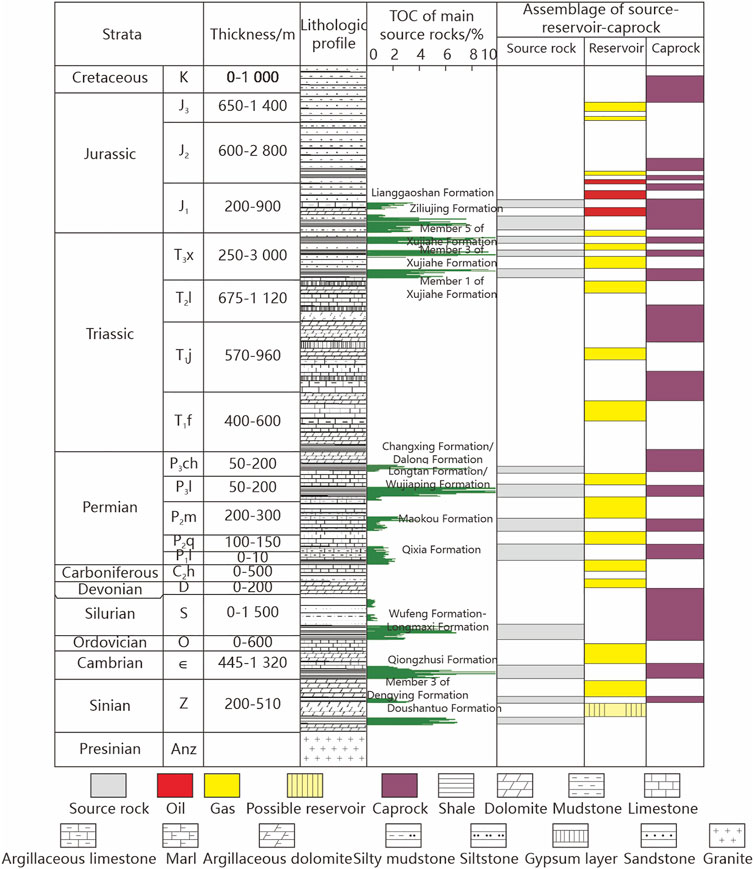
FIGURE 1. Comprehensive histogram of source-reservoir-caprock in Sichuan Basin. T3x-Upper Triassic Xujiahe Formation; T2l-Middle Triassic Leikoupo Formation; T1j-Lower Triassic Jialingjiang Formation; T1f-Lower Triassic Feixianguan Formation; P3ch-Upper Permian Changxing Formation; P2m -Middle Permian Maokou Formation; P2q-Middle Permian Qixia Formation; P1l-Lower Permian Liangshan Formation; C2h-Middle Carboniferous Huanglong Formation.
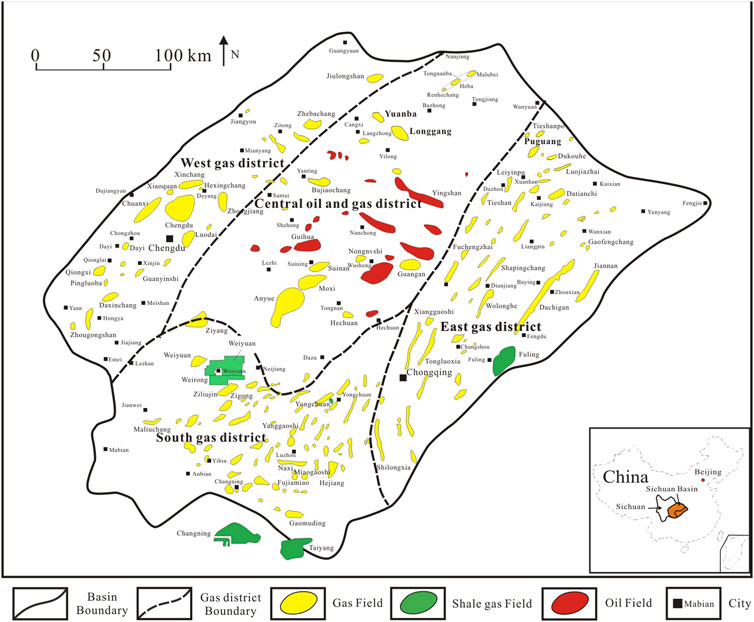
FIGURE 2. Distribution of oil and gas fields in the Sichuan Basin (Modified after Ni et al., 2021).
Natural gas more or less contains CO2; generally, the content of CO2 in natural gas is low. According to the analysis of 1,025 gas samples from 48 large gas fields in nine basins in China, the average CO2 content is 3.58% (Dai, 2016). The CO2 content in natural gas is low and is often widely distributed in cratonic basins with stable structures, such as the Ordos Basin and Sichuan Basin in China (Dai et al., 2017; Wu et al., 2017; Wu et al., 2020). However, there are also some natural gases with a high CO2 content, which are often widely distributed in rift basins with intense tectonic activity, large fault zones, and volcanic activity zones in geological history or modern times (Dai et al., 2000; Dai et al., 2017). For example, the CO2 content of Well Shuishen nine in Sanshui Basin in China reaches 99.55%. The CO2 in natural gas after the volcanic period in the famous young volcanic area of Tengchong is 96.0%–96.9%. CO2 is a greenhouse gas that pollutes the environment, so its high content will reduce the commercial value of natural gas exploration in the area. For example, the CO2 content in natural gas from the exploration well in Lishui sag of the East China Sea extensional basin is high, which is 31%–98%, reducing the commercial interests of exploration (Diao, 2019).
Gas reservoirs can be classified according to the carbon dioxide content in the gas reservoir. Tang (1983) called a gas reservoir with CO2 content of more than 80% to nearly 100% a CO2 gas reservoir. Shen et al. (1991) called a gas reservoir a CO2 gas reservoir when the content of carbon dioxide in the gas reservoir is greater than 85%. Dai et al. (2000) called a gas reservoir with CO2 content of 90% to nearly 100% a CO2 gas reservoir. The gas reservoir with a CO2 content of 60%–90% is called a sub CO2 gas reservoir; the gas reservoir with a CO2 content of 15%–60% is called a high CO2 gas reservoir; A gas reservoir with a CO2 content of trace to 15% is called a CO2-containing gas reservoir. There are many research studies on CO2 gas reservoirs (fields) carried out at home and abroad (Muffler and White, 1968; Qi and Dai, 1981; Tang, 1983; Song, 1991; Dai et al., 2000). The imperial gas field in the Los Angeles Basin of the United States has been producing carbon dioxide since 1934–1954 with accumulated gas production of 18.4 × 106 m3 (Muffler and White, 1968). China’s proved CO2 geological reserves at the end of 2019 amounted to 213 × 109 m3, with a cumulative output of CO2 gas of 12.75 × 109 m3. At least 30 CO2 gas fields (reservoirs) with industrial value have been found in the continental rift basins in eastern China, the continental shelf marginal basins in the East China Sea, and the northern South China Sea (Zhang et al., 2019).
The carbon isotope value of carbon dioxide (δ13CCO2) is an important parameter to identify organic and inorganic carbon dioxide, which has been the research object of many scholars at home and abroad. Shangguan and Zhang (1990) pointed out that CO2 of metamorphic origin has δ13C value similar to that of the sedimentary carbonate rocks, that is, −3‰ ∼ +1‰, while mantle-derived CO2 has δ13C between −8.5‰ and −5‰. Shen et al. (1991) believed that inorganic CO2 has δ13C>−7‰. For the quartz monzodiorite, equigranular granodiorite, and porphyritic granodiorite in granite in Fangshan District, Beijing, the δ13CCO2 values were −3.8‰, −7.4‰, and −7.8‰, respectively (Zhen et al., 1987). Gould et al. (1981) believed that the δ13CCO2 values of magmatic rock origin were generally −7 ± 2‰, although they were variable, while Pankina et al. (1978) believed that the δ13CCO2 value is between −9.1‰ and 4.9‰. Moore et al. (1977) pointed out that the δ13CCO2 value in basalt inclusions in the Middle Pacific ridge is −6.0‰ to −4.5‰. Dai et al. (1989); Dai et al. (1992); Dai et al. (2000) proposed a δ13CCO2−CO2 content identification diagram of organic origin and inorganic origin based on the compilation of 212 gas samples from China and more than 100 samples from Australia, Thailand, New Zealand, the Philippines, Canada, Japan, and the former Soviet Union. At the same time, it is pointed out that organic CO2 has δ13C value lower than −10‰, mainly in the range of −30‰ to −10‰; inorganic CO2 has δ13C value more than −8‰, mainly in the range of −8‰ to +3‰. Among inorganic carbon dioxide, those of carbonate rock metamorphism origin have δ13CCO2 value close to that of the carbonate rock, about 0 ± 3‰; CO2 of volcanic magmatic origin and mantle origin has δ13C values mostly in the range of - 6 ± 2‰. At the same time, the 3He/4He−δ13CCO2 diagram (see Section 3.1) can be used to identify inorganic carbon dioxide of carbonate thermal metamorphic origin or magmatic and mantle origin (Etiope et al., 2011).
The study of CO2 is far behind that of the alkane gas due to the following two reasons: 1. Alkane gas has very high economic values; thus, it attracts much research attention. 2. Alkane gases have similar chemical structure and chemical characteristics, which can provide more scientific information. However, CO2 is an important greenhouse gas; its occurrence in natural gas not only impacts the commercial value of natural gas and potential environmental pollution but also has great significance on the gas origin and gas-source correlation research of the accompanied natural gases. The aim of this study is to investigate the geochemistry characteristics of CO2 in the Sichuan Basin and further explore its formation mechanism, thus establishing a set of chemical and isotopic distinguishing parameters for CO2 of different origins. In this study, we systematically analyzed the chemical and isotopic compositions of CO2 from the Sichuan Basin, and published data of CO2 from different strata and gas fields are also compared. Three different formation mechanisms of CO2 are investigated, their typical carbon isotopic compositions are identified, and their relationship with hydrocarbon gases is discussed. The geochemical study of CO2 has great significance for the research and exploration of natural gas in the Sichuan Basin.
2 Analytical Methods
Stable carbon isotopic compositions were determined on a Thermo Delta V mass spectrometer in the PetroChina Research Institute of Petroleum Exploration and Development. The mass spectrometer was interfaced with a Thermo Trace GC Ultra gas chromatograph (GC). Individual hydrocarbon gas components (C1–C4) and CO2 were separated on a gas chromatograph using a fused silica capillary column (PLOT Q 27.5 m × 0.32 mm × 10 μm), which were then converted into CO2 in a combustion interface, and finally injected into the mass spectrometer. The temperature of the GC oven rises from 33 to 80°C at 8°C/min, then to 250°C at 5°C/min, and the final temperature was maintained for 10 min. Gas samples were analyzed in triplicate, and the stable carbon isotopic values were reported in the δ-notation in per mil (‰) relative to Vienna Peedee Belemnite (VPDB), and the analytical precision is ±0.3‰.
3 Results and Discussion
3.1 Cratonic CO2 With Low Content in Natural Gas
Supplementary Tables S1, S2 show the geochemical parameters of natural gas from 22 gas fields in Sichuan Basin. By analyzing 243 CO2 components and their relationship with relevant parameters, the compositional characteristics of CO2 can be obtained.
The CO2 content of 243 gas fields ranges from 0.02% (Xinchang gas field X21-h, Dachiganjing gas field G31, Weiyuan gas field Wei202, and Fuling gas field JY6-2) to 22.90% (Yuanba gas field YB101), with an average content of 2.96%, which is lower than the average CO2 content of 3.58% (Dai et al., 2016) of 1,025 gas samples from 48 large gas fields developed in China (Supplementary Tables S1, S2, Figure 3). The low CO2 content in Sichuan Basin reduces the risk of natural gas exploration and development.
According to the CO2-R/Ra diagram (Figure 4) (Dai et al., 2017), CO2 in cratonic basin (expressed as cratonic CO2) is characterized by low CO2 content (generally <5%) and small variation of R/Ra ratio (<0.24), while CO2 in rift basin (expressed as rift CO2) is characterized by large variation of CO2 content (0.0n%–>95%) and large variation of R/Ra (0.0n–n). A total of 41 samples with CO2 and R/Ra values from Supplementary Tables S1, S2 all fall into C1 (Ordos Basin) and C2 (Sichuan Basin) cratonic areas, indicating that the CO2 from Supplementary Tables S1, S2 belongs to cratonic CO2. The rest 202 samples in Supplementary Tables S1, S2 have CO2 values but no R/Ra values. According to the research work by Ni et al. (2014), the average value of R/Ra in the Sichuan Basin is only 0.016, so the rest 202 samples without R/Ra data are also cratonic CO2.
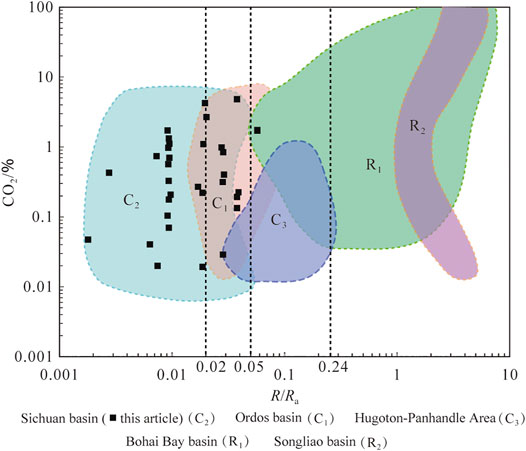
FIGURE 4. Comparison of CO2-R/Ra between cratonic basin and rift basin (Modified after Dai et al., 2017).
3.2 Characteristics of Carbon Isotope of Carbon Dioxide (δ13CCO2)
3.2.1 Heaviest Carbon Isotope of Carbon Dioxide in China
The interval value of δ13CCO2 of natural gas in the Sichuan Basin ranges from −25.4‰ (Yuanba gas field, Y11 well) to +10.4‰ (Fuling gas field, JY47-3 well), and the main frequency peak is between −6‰ and +2‰ (Supplementary Tables S1, S2, Figure 5). 30 years ago, Dai et al. (1992) pointed out that the δ13CCO2 value of natural gas in China ranged from −39‰ to +7‰. In the past 30 years, the author analyzed δ13CCO2 values of 102 samples. Combined with 508 published δ13CCO2 values by other researchers (He, 1995; Fu et al., 2004; Liao et al., 2012; Liu D et al., 2016; Liu et al., 2018; Deng et al., 2018; Zhang et al., 2018a; Xu et al., 2018; Zhang et al., 2018b; Zhang S. et al., 2018; Li, et al., 2018; Diao, 2019; She et al., 2021; Wei et al., 2021), about 610 samples in total are investigated, which are distributed in Songliao, Bohai Bay, Sanshui, Ordos, Sichuan, Tarim, East China Sea, and Yinggehai–Qiongnan basins. Among them, only five samples have δ13CCO2 values higher than 7‰, ranging from 7.8‰ to 8.9‰ (Xu et al., 2018). Therefore, at present, the δ13CCO2 value of 10.4‰ in Well JY47-3 in this study should be the highest in China. Thus, in China, the variation range of δ13CCO2 value should be −39‰ to +10.4‰, which is lower than that of the world whose interval value of the δ13CCO2 ranges from −42‰ to +27‰ (Barker, 1983). Therefore, the δ13CCO2 interval value of China, both high and low, still has the potentiality of extension.
3.2.2 Carbon Isotopic Identification Parameters of CO2
The δ13C values have usually been used to identify the CO2 origins such as organic versus inorganic and also sub-categories of them (Table 1). Parameters such as δ13CCO2−CO2 (Dai et al., 1992) (Figure 6), δ13CCO2−R/Ra (Etiope et al., 2011), and δ13CCO2−δ13C1 (Milkov and Etiope, 2018) have been widely used. It can be seen from Table 1 and Figure 6 that the δ13CCO2 value of inorganic CO2 is higher than that of organic CO2. This is because the original δ13C of organic CO2 is relatively low, and the original δ13C of inorganic CO2 is relatively high (Table 2). Due to the carbon isotopic inheritance, the carbon isotopic composition of organic and inorganic CO2 gases is mainly affected by the carbon isotopic value of their precursors.
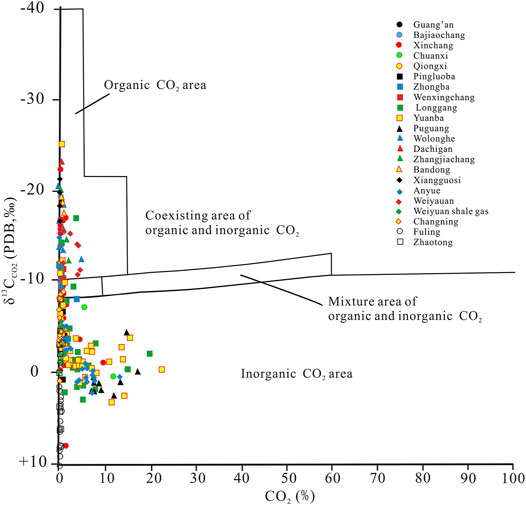
FIGURE 6. Identification of CO2 origin of natural gas in the Sichuan Basin (Dai et al., 1996).
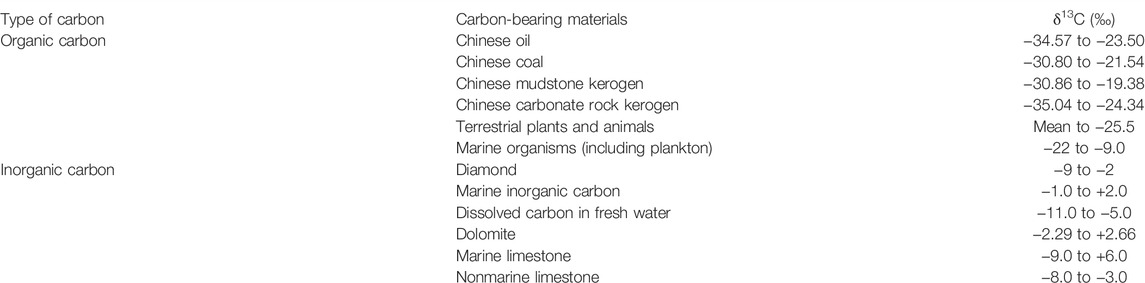
TABLE 2. δ13C values of various carbon-bearing materials (Dai et al., 2000).
When the δ13CCO2 value < −10‰ (a few < −8‰), the carbon dioxide belongs to organic origin, including gas samples from Guang’an, Bajiaochang, Zhongba, Wenxingchang, Wolonghe, Dachiganjing, Zhangjiachang, Bandong, Xiangguosi, and Weiyuan gas fields, and Xujiahe Formation gas reservoir of Longgang gas field, Jurassic Formation gas reservoir of Xinchang gas field, and Triassic Formation gas reservoir of Anyue gas field (Supplementary Table S1, Table 1, and Figure 6). CO2 of organic origin can be divided into several sub-categories (Supplementary Table S1, Table 1 and Figure 7). Among the abovementioned 13 gas fields, gases in the Guang’an, Bajiaochang, and Zhongba gas fields, Jurassic Formation gas reservoir in Xinchang gas field, and Triassic Formation gas reservoir in Anyue gas field are thermogenic wet gas (oil-associated thermogenic gas, OA). The wetness (W) of 37 gas samples varies between 3.2% and 17.7%, with an average value of 7.8%, and the δ13CCO2 values of 44 gas samples range from − 6.2‰ to −22.6‰, with an average of −12.8‰. The thermal maturity Ro% value of gas source rocks of Xujiahe Formation in Guang’an gas field, Zhongba gas field, and Bajiaochang gas field is between 0.88% and 1.15% (Dai et al., 2016), which also proves that the carbon dioxide in these gas fields is of thermogenic origin. Among them, there are individual wells showing a δ13CCO2 value of inorganic origin, that is, Well Jiao49 has a δ13CCO2 value of −6.2‰, which is inorganic CO2 formed by dissolution of carbonates through organic acid, such as Well Pu1 in Ordos Basin, whose δ13CCO2 value of −6.39‰ results from the dissolution of carbonates by organic acid (Dai et al., 1992).
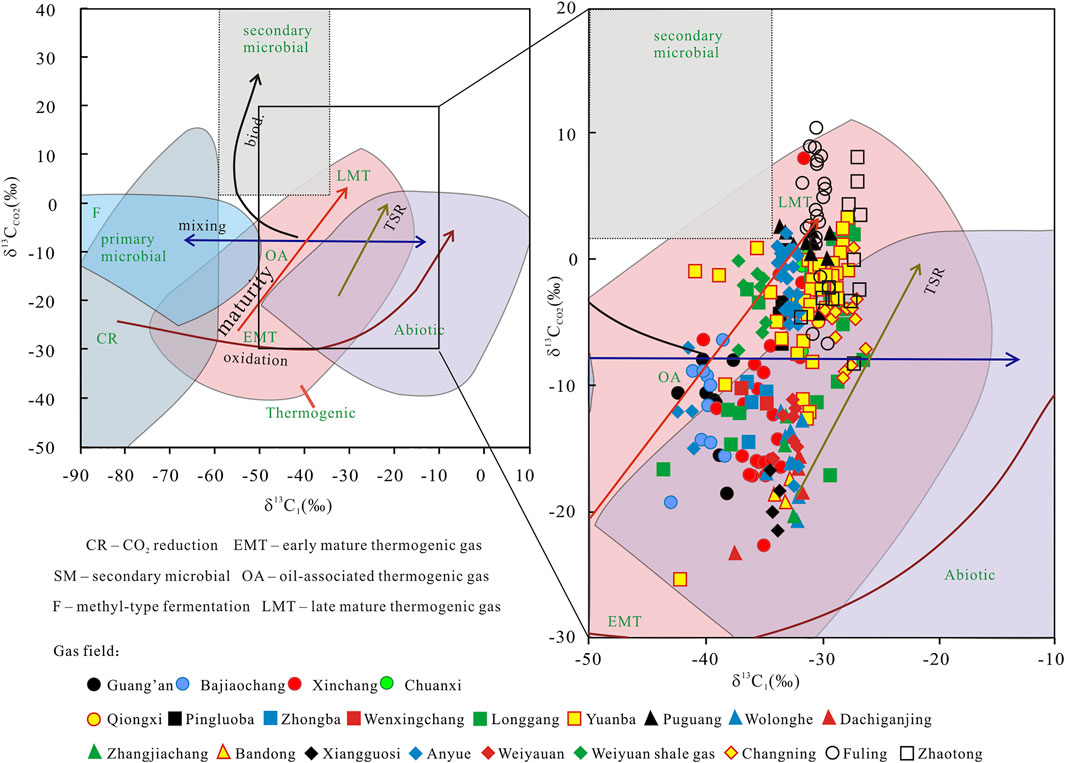
FIGURE 7. Plot of δ13C1−δ13CCO2 of natural gases from gas fields in the Sichuan Basin (Modified after Milkov and Etiope, 2018).
CO2 in Wenxingchang gas field, Xujiahe Formation gas reservoir of Longgang gas field, Wolonghe gas field, Dachiganjing gas field, Zhangjiachang gas field, Bandong gas field, Xiangguosi gas field, and Weiyuan gas field are of cracking origin. The δ13CCO2 value of 34 gas samples fall between −23.4 and −10.3‰, with an average of −15.7‰. The wetness of 33 gas samples ranges from 0.08% to 7.04%, with an average of 1.30%. Alkane gas accompanied with CO2 of thermogenic origin is often dry gas, which also proves that CO2 is of thermogenic origin. It can be seen from Figure 7 that there are only thermogenic CO2 (in the area of OA) and cracking CO2 (in the area of LMT) among the biogenic gas in the Sichuan Basin, and no microbial degradation type CO2 (EMT).
In addition to the abovementioned thermal decomposition and cracking CO2, in Supplementary Table S1, among Xujiahe Formation gas reservoir and Leikoupo Formation gas reservoir of Xinchang gas field, Western Sichuan gas field, Qiongxi gas field, Pingluoba gas field, Leikoupo Formation gas reservoir of Longgang gas field, Feixianguan Formation gas reservoir, Changxing Formation gas reservoir, and Permian gas reservoir of Yuanba gas field, Feixianguan Formation gas reservoir of Puguang gas field, and Longwangmiao Formation gas reservoir and Dengying Formation gas reservoir of Anyue gas field, the majority is dry gas. Based on the analysis of 120 gas samples, the δ13CCO2 value ranges from 8.1 to 17.2‰, with an average of 2.4‰. According to the analysis of 118 gas samples, the gas wetness is 0.02%–11.5%, with an average value of 1.02%. Supplementary Table S2 shows the δ13CCO2 values of shale gas from Weiyuan, Changning, Fuling, and Zhaotong shale gas fields. According to the analysis of 55 gas samples, the δ13CCO2 value ranges from −9.2‰ (well N211) to 10.4‰ (well JY47-3), with an average value of 0.42‰. According to the analysis of 54 gas samples, the gas wetness ranges from 0.28% to 0.79% with an average of 0.47%. It can be seen from Table 1 and Figure 6 that CO2 in the abovementioned gas fields is of inorganic origin.
According to the genetic type, inorganic CO2 can be subdivided into upper mantle degassing, volcanic magmatic source, and thermal metamorphism or organic acid dissolution of carbonate rocks (minerals) (Table 1). The shale gas is characterized by δ13C1>δ13C2>δ13C3, belonging to the secondary negative carbon isotope series (Dai et al., 2016). For the Marcellus shale gas, which has the largest annual production, when the gas wetness (W) is less than 1.49%–1.57%, the secondary negative carbon isotope series appear (Jenden et al., 1993). The gas wetness of the four shale gas fields in Supplementary Table S2 is between 0.28% (well NH3-6) and 0.79% (well JY12-2), so they all have negative carbon isotope series. The negative carbon isotope series only occur in the thermogenic gas in the over-mature area, and the RO% of the gas source rocks is greater than 2%. As shown in Supplementary Table S2, the RO% value of Wufeng–Longmaxi shale in Changning, Zhaotong, and Fuling shale gas fields fall in the range of 2.1%–3.85% (Dai et al., 2014; Guo and Zeng, 2015; Dai et al., 2016; Liu S et al., 2016; Feng et al., 2020). Since the shale of Wufeng–Longmaxi formations is rich in carbonate minerals (Dai et al., 2014; Dai et al., 2016; Feng et al., 2020) and is at the over-mature stage, these two factors together led to inorganic CO2 from thermal metamorphism of carbonate minerals. Figure 8 clearly shows that the CO2 from the four shale gas fields is of inorganic origin related to carbonate minerals. However, the δ13CCO2 values of the four shale gas fields in Figure 7 mainly fall in the thermogenic area (LMT), so the δ13CCO2 value in Figure 7 is in the range of −8‰ to 10‰, which should be classified as inorganic CO2 from thermal metamorphism of carbonate minerals.
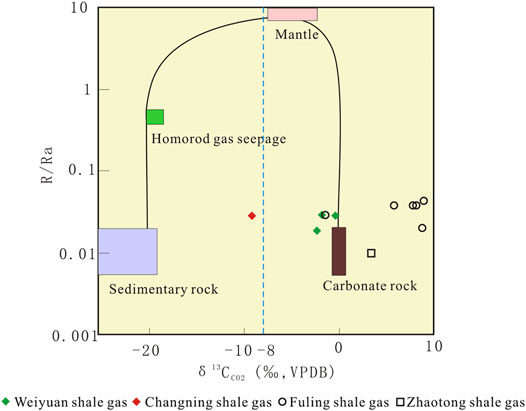
FIGURE 8. Comparison diagram of natural gas R/Ra−δ13CCO2 in the Sichuan Basin (Modified after Etiope et al., 2011).
Calcareous sandstone is widely distributed in the fourth member of Xujiahe Formation (T3x4) in Western Sichuan depression, and carbonate rock debris accounts for more than 50% of calcium debris (Lin et al., 2007; Lin et al., 2012). Calcareous sandstone is also developed in the third member of Xujiahe Formation (T3x3) in the Yuanba area. Carbonate debris which is dissolved by organic acid is discharged during the compaction of mudstone in the third member of Xujiahe Formation in the late diagenetic stage of Xujiahe Formation (Ma, 2012), forming organic acid dissolved CO2 in inorganic carbonate rocks of Xujiahe Formation in Yuanba gas field (Dai et al., 2013). However, according to Supplementary Table S1, Xujiahe Formation gas reservoir of Yuanba gas field is characterized by dry gas, with gas wetness mainly between 0.39% and 1.51%, and δ13CCO2 value between −7.5‰ and 0.5‰, so CO2 in Xujiahe Formation gas reservoir of Yuanba gas field should also include CO2 derived from the thermal metamorphism of carbonate mineral. The Xujiahe Formation gas reservoirs of Pingluoba gas field and Qiongxi gas field are similar to Yuanba gas reservoir with dry natural gas, so the CO2 in these gas fields should also originate from thermal metamorphism of carbonate minerals.
In Supplementary Table S1, the Leikoupo Formation gas reservoir of Xinchang gas field, Leikoupo Formation gas reservoir of Longgang gas field, Feixianguan Formation gas reservoir, Changxing Formation gas reservoir, and Leikoupo Formation gas reservoir of Yuanba gas field, Feixianguan Formation gas reservoir and Changxing Formation gas reservoir of Puguang gas field, and Longwangmiao and Dengying (Z2dn) formations gas reservoirs of Anyue gas field are characterized by carbonate rock reservoir and dry natural gas. Therefore, they should also produce carbonate mineral thermal metamorphism type of CO2.
3.2.3 δ13CCO2>δ13C1
Figure 9 shows the carbon isotope of coexisting carbon dioxide and methane from the 22 gas fields, which shows a wide range of δ13CCO2 (35.8‰) but a relatively narrow range of δ13C1 (17.2‰). They are characterized by δ13CCO2>δ13C1, which is found in all geological age (from Z2dn to J2s) gas reservoirs and various gas types (coal-derived gas, oil-associated gas, and shale gas). The difference between δ13CCO2 and δ13C1 (δ13CCO2-CH4) for individual gas samples varies between 11.4‰ (Wolonghe gas field) and 40.9‰ (Fuling shale gas field), with an average of 27.0‰ (n = 261).
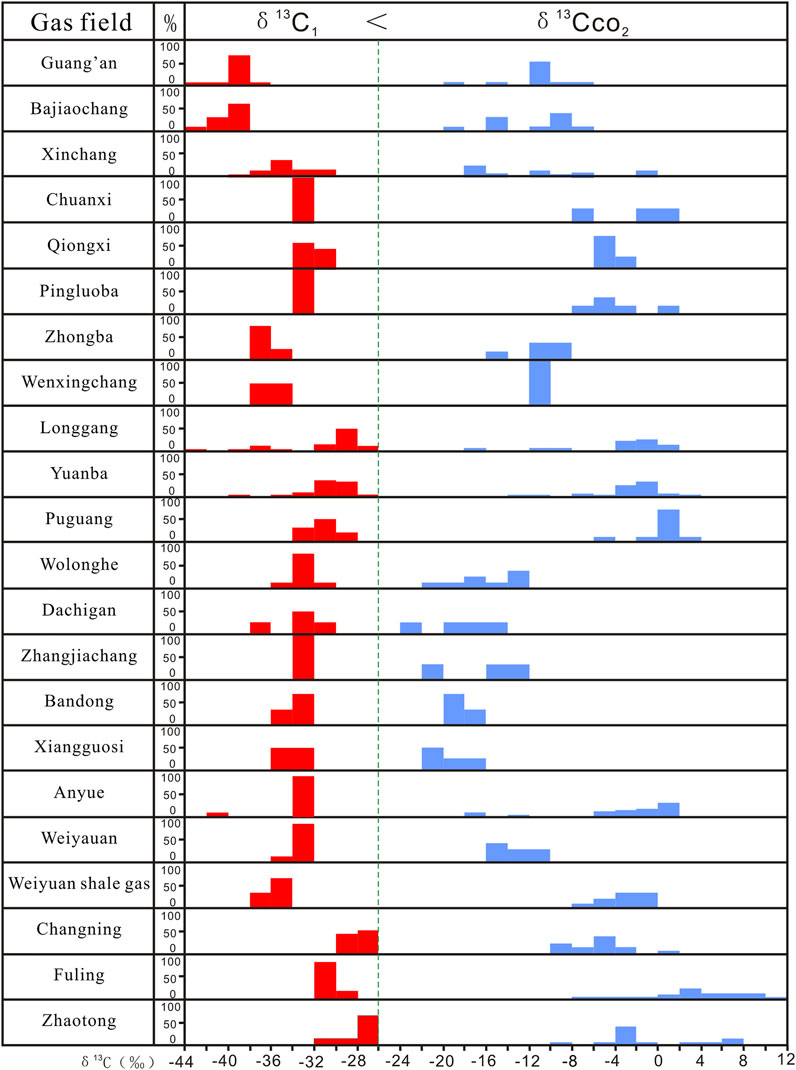
FIGURE 9. Comparison of carbon isotopic value of methane and CO2 in natural gas from the Sichuan Basin.
Carbon isotopic changes of CH4 and CO2 mainly result from the different sources. Sources of methane mainly include bacterial, thermogenic, and inorganic. Bacterial methane is normally generated at low temperature and depleted in 13C (δ13C < −50‰), thermogenic methane is formed at elevated temperatures by decomposition or cracking of organic matter and generally characterized by −50‰<δ13C < −30‰, and inorganic methane derived from mantle degassing or reactions at high temperatures is enriched in 13C (δ13C > −30‰). As discussed previously, sources of carbon dioxide mainly include thermogenic and inorganic. CO2 formed through the decomposition or cracking of organic matter is relatively depleted in 13C (<−10‰, mainly of −10‰ to −30‰), while CO2 derived from mantle degassing, volcanic magmatic source, and thermal metamorphism or organic acid dissolution of carbonate rocks (minerals) are much more enriched in 13C (>−8‰, mainly of −8‰∼+3‰).
As shown in Figure 10, δ13CCO2 varies between −25.4‰ and 10.4‰, with an average of −5.8‰ (n = 263), while δ13C1 varies between −43.5‰ and −26.3‰, with an average of −32.8‰ (n = 261). The variation range of CO2 (35.8‰) is nearly twice that of CH4 (17.2‰). According to the δ13C values of methane, alkane gases from these 22 gas fields all belong to thermogenic gas. In contrast, sources of CO2 include both organic and inorganic. Organic CO2 commonly has carbon isotopic composition lower than −10‰, and the lowest δ13CCO2 value of −25.4‰ is found in the Yuanba gas field. Since thermogenic methane has δ13C1<δ13CCO2, the carbon isotopic difference between thermogenic methane and organic CO2 (δ13CCO2-CH4) is relatively small, and the smallest carbon isotopic difference of 11.4‰ is found in the Wolonghe gas field. Since inorganic CO2 is characterized by much heavier carbon isotopic compositions (δ13CCO2>−8‰), the carbon isotopic difference between thermogenic methane and inorganic CO2 (δ13CCO2-CH4) will be bigger, and the biggest carbon isotopic difference of 40.9‰ is found in the Fuling shale gas field. CO2 generated from the thermos-metamorphic process of carbonates is enriched in 13C. As in the CO2–calcite system, carbon isotope fractionation will cause the enrichment of 13C in CO2 at high temperature; therefore, CO2 produced by decarbonation reactions will be more enriched in 13C than that in the original carbonates (Giustini et al., 2013). δ13C of Phanerozoic seawater is generally stable, and the Phanerozoic low magnesium calcite shells have δ13C values of −2 to +6‰ (Veizer et al., 1999; Dong et al., 2021). δ13C values of carbonate cement of sandstone from the Silurian Formation in southeast Sichuan vary from −1.90‰ to 4.78‰ with an average value of 1.42‰ (n = 14) (An et al., 2015). However, positive carbon isotopic excursion of both shales and limestones has been found in the Late Ordovician Hirnantian stage in North America (Orth et al., 1986; BergstrÖM et al., 2006), Europe (Brenchley et al., 1994; Marshall et al., 1997), and China (Wang et al., 1997; Fan et al., 2009). The positive carbon isotopic excursion can be up to 5–7‰ in the Hirnantian limestones (Qing and Veizer, 1994; Marshall et al., 1997). A recent study found that diffusive migration of shale gas occurs in the southern Sichuan Basin (Ni et al., 2021). Therefore, if assuming an infinite reservoir of C compared with CO2 generated by decarbonation and the CO2 decarbonated does not isotopically fractionate on its way to the surface in the absence of water, metamorphic reactions between carbonate and silicate occur at 600 °C (Muffler and White, 1968), and the produced CO2 will be enriched in 13C by about +2.6‰ compared with that of CaCO3 (Ohmoto and Rye, 1979). Then, it will produce a gas with δ13C around 4‰, and if considering the carbon isotopic excursion, it will be around 10‰ (Giustini et al., 2013). While in the presence of water, metamorphic reactions between carbonate and silicate begin with T > 200°C (Muffler and White, 1968), and the produced CO2 will be enriched in 13C by 1.3‰ at a temperature around 250°C (Ohmoto and Rye, 1979). Then, it will produce a gas with δ13C around 2.7‰, and if considering the carbon isotopic excursion, it will be around 9‰ (Giustini et al., 2013).
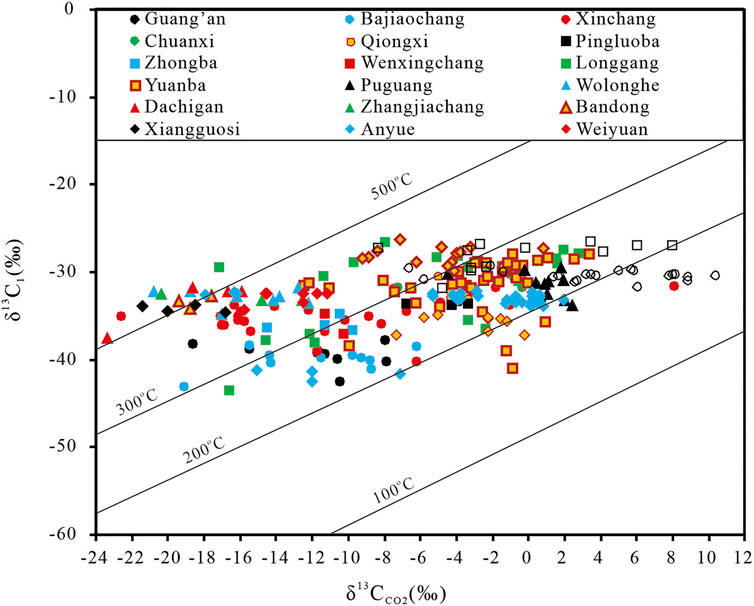
FIGURE 10. Plot of δ13C values of CO2 and coexisting CH4. Isotherms for hypothetical isotopic equilibrium are from Richet et al. (1977).
4 Geological Implications
The three types of CO2 gases are characterized by different geochemical characteristics and different reservoir types, and distributed in different sedimentary basins. Type A organic CO2, generated from the thermal decomposition of organic matter, was mainly formed in the craton basin, where organic matter was controlled by thermal evolution. The CO2 content and carbon isotope composition were different at different stages of thermal evolution, but the CO2 abundance was generally relatively low, such as the Upper Paleozoic gas reservoir in Ordos Basin. Type B organic CO2 was formed through the cracking of organic matter or hydrocarbons at higher thermal maturity. For example, CO2 in the over-mature coal-derived gas from the Kuqa depression in the Tarim basin was mainly formed through the cracking of organic matter. Thermal cracking of crude oil in the marine gas reservoirs can also form cracking CO2 of organic origin such as the CO2 in the Tazhong and Tabei deep natural gas. Type C inorganic CO2 has complex sources and pathways, including the thermal metamorphism or thermal decomposition of deep carbonates, organic acid dissolution, TSR, and mantle degassing. High temperature is required for the thermal metamorphism or thermal decomposition of deep carbonates such as the CO2-rich gas reservoirs in Yinggehai Basin. CO2 formed through the organic acid dissolution is mainly distributed in the gas reservoirs where TSR occurs such as the Ordovician marine facies Jianbian gas field in the Ordos Basin and the marine gas reservoirs in Sichuan Basin. Inorganic mantle-derived CO2 is mainly controlled by deep faults such as Fangshen two and Songnan gas reservoirs in Songliao Basin and Huangqiao gas reservoir in Subei Basin. The content of inorganic CO2 varies widely. Generally, the content of deep mantle-derived CO2 is more than 60%, while the content of carbonate decomposition and organic acid dissolution depends on gas reservoir temperature and source supply. In short, different geological backgrounds and evolutionary histories will form different types of CO2, and their content is also very different.
5 Conclusion
The Sichuan Basin is a large superimposed basin developed on the basis of craton, with an area of about 180 × 103 km2. It has excellent geological conditions for natural gas development: ① The thickness of sedimentary rocks is 6,000–12,000 m, the maturity of source rocks is high, there are nine sets of main gas source rocks, and the equivalent ratio of gas to oil production is 80:1, so it is a gas basin. ② It is rich in conventional and unconventional gas resources, and the remaining recoverable resources of conventional and unconventional natural gas amounts to 13.6404 × 1012 m3. The total proved geological reserves of natural gas was 5.7966 × 1012 m3, and the cumulative gas production of the basin was 648.8 × 109 m3 by the end of 2019. ③ There are many gas-bearing formations and systems, including 25 conventional and tight oil and gas producing formations (18 marine facies) and two shale gas–producing formations. ④ A total of 135 gas fields had been discovered by the end of 2019. Anyue gas field, the largest gas field and the largest carbonate gas field in China, had proved geological reserves of 1.1709 × 1012 m3, with an annual output of 120 × 108 m3 in 2019.
Carbon dioxide composition of CO2 in Sichuan Basin is characterized by two features: ① The content of carbon dioxide is low. Based on 243 CO2 components collected from 22 gas fields, the content ranges from 0.02% to 22.90%, with an average value of 2.96%, which is lower than the average value of 3.58% of 1025 CO2 components in 48 large gas fields developed in China. ② Carbon dioxide in cratonic basins is featured with a combination of a low CO2 content (generally <5%) and a low R/Ra ratio (<0.24), while carbon dioxide in rift basins is typically characterized by large variation of the CO2 content (0.0n% – > 95%) and large variation of the R/Ra ratio (0.0n – n).
Based on the δ13CCO2 values of 263 samples in Sichuan Basin and their correlation with R/Ra, δ13C1, CO2 content, and gas wetness, it is observed that δ13CCO2 has three characteristics: ① δ13CCO2 (10.4‰) in Fuling shale gas field was found to be the highest in China, making the interval value of δ13CCO2 of China expand from −39‰–7‰ to −39–10.4‰. ② According to the δ13CCO2 value, three types of CO2 were identified: A. organic CO2 formed by the thermal decomposition of organic matter. The δ13CCO2 values of 44 samples range from −6.2 to −22.6‰, with an average value of −12.8‰, and the gas wetness of 37 samples ranges from 3.2% to 17.7%, with an average of 7.8%; B. organic CO2 formed by the cracking of organic matter. The δ13CCO2 values of 34 samples range from −10.3‰ to −23.4‰, with an average value of −15.7‰, and gas wetness of 33 samples ranges from 0.08% to 7.04%, with an average value of 1.30%; C. inorganic CO2 formed by the dissolution of carbonates through metamorphism or organic acid. δ13CCO2 values of 175 samples range from −17.2 to 10.4‰, with an average value of −1.8‰, and gas wetness of 172 samples ranges from 0.02% to 11.5%, with an average value of 0.85%; ③ δ13CCO2>δ13C1, which is a characteristic shared by all geological age (from Z2dn to J2s) gas reservoirs and various gas types (coal-derived gas, oil-associated gas, and shale gas).
Data Availability Statement
The original contributions presented in the study are included in the article/Supplementary Material, further inquiries can be directed to the corresponding author.
Author Contributions
JD: manuscript writing and design, data collection. YN: manuscript writing and revision, sample analyses. QL: manuscript revision and data collection. XW, CY and DG: manuscript revision. FH, YZ and ZY: manuscript preparation.
Funding
This study is funded by the National Key Research and Development Projects of China (Grant No. 2019YFC1805505), the PetroChina Scientific Research and Technology Development Project (2017D-5008-08), and the National Natural Science Foundation of China (Grant Nos.: U20B6001, 42172149, and 42141021).
Conflict of Interest
Authors JD, YN, CY, DG, FH, YZ, and ZY were employed by the company PetroChina. Authors QL and XW were employed by the company SINOPEC.
The authors declare that the research was conducted in the absence of any commercial or financial relationships that could be construed as a potential conflict of interest.
Publisher’s Note
All claims expressed in this article are solely those of the authors and do not necessarily represent those of their affiliated organizations, or those of the publisher, the editors, and the reviewers. Any product that may be evaluated in this article, or claim that may be made by its manufacturer, is not guaranteed or endorsed by the publisher.
Supplementary Material
The Supplementary Material for this article can be found online at: https://www.frontiersin.org/articles/10.3389/feart.2022.857876/full#supplementary-material
References
An, Q., Huang, D., and Zhu, Z. (2015). Carbon and Oxygen Isotope Geochemistry for Carbonate Cement of the Lower Silurian Xiaoheba Formation Sandstone in Southeast Sichuan-West Hunan. Acta Geologica Sichuan 35 (1), 157–160.
Barker, C. (1983). Petroleum Generation and Occurrence for Exploration Geologists. Testbook of OGCI.
BergstrÖM, S. M., Saltzman, M. M., and Schmitz, B. (2006). First Record of the Hirnantian (Upper Ordovician) δ13C Excursion in the North American Midcontinent and its Regional Implications. Geol. Mag. 43 (5), 657–678.
Brenchley, P. J., Marshall, J. D., Carden, G. A. F., Robertson, D. B. R., Long, D. G. F., Meidla, T., et al. (1994). Bathymetric and Isotopic Evidence for a Short-Lived Late Ordovician Glaciation in a Greenhouse Period. Geology 22 (4), 295–298. doi:10.1130/0091-7613(1994)022<0295:baiefa>2.3.co;2
Cao, B., Mz, C., Lla, B., Ywb, D., Zla, B., Dab, L., et al. (2020). Tracing the Sources and Evolution Processes of Shale Gas by Coupling sTable (C, H) and noble Gas Isotopic Compositions: Cases from Weiyuan and Changning in Sichuan Basin, China. J. Nat. Gas Sci. Eng. 78. doi:10.1016/j.jngse.2020.103304
Chen, Z., Chen, L., Wang, G., Zou, C., Jiang, S., Si, Z., et al. (2020). Applying Isotopic Geochemical Proxy for Gas Content Prediction of Longmaxi Shale in the Sichuan Basin, China. Mar. Pet. Geology. 116 (C8), 104329. doi:10.1016/j.marpetgeo.2020.104329
Cornides, I. (1993). Magmatic Carbon Dioxide at the Crust's Surface in the Carpathian Basin. Geochem. J. 27, 241–249. doi:10.2343/geochemj.27.241
Dai, J., Chen, J., Zhong, N., Pang, X., and Qin, S. (2003). Large Gas fields and Their Gas Sources in China. Beijing: Science Press, 37–44.
Dai, J. (1981). Geographical Distribution of Oil and Gas Discovered in Ancient China. Oil & Gas Geology. 2 (3), 292–299.
Dai, J. (2016). Giant Coal-Derived Gas fields and Their Gas Sources in China. Beijing: Science Press, 180–186. 210-214, 241-254.
Dai, J., Liao, F., and Ni, Y. (2013). Discussions on the gas source of the Triassic Xujiahe Formation tight sandstone gas reservoirs in Yuanba and Tongnanba, Sichuan Basin: An answer to Yinfeng et al. Pet. Exploration Dev. 40 (2), 250–256. doi:10.1016/s1876-3804(13)60033-6
Dai, J., Ni, Y., Huang, S., Gong, D., Liu, D., Feng, Z., et al. (2016). Secondary Origin of Negative Carbon Isotopic Series in Natural Gas. J. Nat. Gas Geosci. 1 (1), 1–7. doi:10.1016/j.jnggs.2016.02.002
Dai, J., Ni, Y., Liu, Q., Wu, X., Gong, D., Hong, F., et al. (2021). Sichuan Super Gas basin in Southwest China. Pet. Exploration Dev. 48 (6), 1–8. doi:10.1016/s1876-3804(21)60284-7
Dai, J., Ni, Y., Qin, S., Huang, S., Gong, D., Liu, D., et al. (2017). Geochemical Characteristics of He and CO 2 from the Ordos (Cratonic) and Bohaibay (Rift) Basins in China. Chem. Geology. 469, 192–213. doi:10.1016/j.chemgeo.2017.02.011
Dai, J., Ni, Y., Qin, S., Huang, S., Peng, W., and Han, W. (2018). Geochemical Characteristics of Ultra-deep Natural Gas in the Sichuan Basin, SW China. Pet. Exploration Dev. 45 (4), 619–628. doi:10.1016/s1876-3804(18)30067-3
Dai, J., Pei, X., and Qi, H. (1992). Natural Gas Geology of China·Volume 1. Beijing: Petroleum Industry Press, 46–50.
Dai, J., Qi, H., and Hao, S. (1989). Survey of Natural Gas Geology. Beijing: Petroleum Industry Press, 30–42.
Dai, J., Song, Y., and Dai, C. (2000). Conditions Governing the Formation of Abiogenic Gas and Gas Pools in Eastern China. Beijing, New York. 1-4, 19-23, 42-60, 73-200.
Dai, J., Song, Y., and Dai, C. (1996). Geochemistry and Accumulation of Carbon Dioxide Gases in China. AAPG Bull. 80 (10), 1615–1626. doi:10.1306/64eda0d2-1724-11d7-8645000102c1865d
Dai, J., Zou, C., Liao, S., Dong, D., Ni, Y., Huang, J., et al. (2014). Geochemistry of the Extremely High thermal Maturity Longmaxi Shale Gas, Southern Sichuan Basin. Org. Geochem. 74, 3–12. doi:10.1016/j.orggeochem.2014.01.018
Deng, Y., Hu, G., and Zhao, C. (2018). Geochemical Characteristics and Origin of Natural Gas in Changxing-Feixianguan Formtions from Longgang Gas Field in the Sichuan Basin, China. Nat. Gas Geosci. 29 (6), 892–907.
Diao, H. (2019). Sources of Natural Gas and Carbon Dioxide in Lishui Sag, East China Sea Basin. Shanghai Land Resour. 40 (4), 101–105.
Dong, Q., Hu, Z., and Chen, S. (2021). Isotope Geochemical Responses and Their Geological Significance of Changxing-Feixianguan Formation Carbonates, Northeastern Sichuan Basin. Oil Gas Geology. 42 (6), 1307–1320.
Etiope, G., Baciu, C. L., and Schoell, M. (2011). Extreme Methane Deuterium, Nitrogen and Helium Enrichment in Natural Gas from the Homorod Seep (Romania). Chem. Geology. 280, 89–96. doi:10.1016/j.chemgeo.2010.10.019
Fan, J. X., Peng, P. A., and Melchin, M. J. (2009). Carbon Isotopes and Event Stratigraphy Near the Ordovician–Silurian Boundary, Yichang, South China. Palaeogeogr. Palaeoclimatol. Palaeoecol. 276 (1), 160–169. doi:10.1016/j.palaeo.2009.03.007
Fan, R., Zhou, H., and Cai, K. (2005). Carbon Istopic Geochemistry and Origin of Natural Gas in Southern Part of the Western Sichuan Depression. Acta Geoscientica Sinica 26 (2), 157–162.
Feng, Z., Hao, F., Dong, D., Zhou, S., and Li, Z. (2020). Geochemical Anomalies in the Lower Silurian Shale Gas from the Sichuan Basin, China: Iosights from a Rayleigh-type Fractionation Model. Org. Geochem. 142. doi:10.1016/j.orggeochem.2020.103981
Fryklund, B., and Stark, P. (2020). Super Basins-New Paradigm for Oil and Gas Supply. Bulletin 104 (12), 2507–2519. doi:10.1306/09182017314
Fu, X., Li, C., Wang, X., and Hao, J. (2004). Forming Conditions of CO2 Gas Reservoir in Sanshui Basin. Nat. Gas Geosci. 15 (4), 428–431.
Giustini, F., Blessing, M., Brilli, M., Lombardi, S., Voltattorni, N., and Widory, D. (2013). Determining the Origin of Carbon Dioxide and Methane in the Gaseous Emissions of the San Vittorino plain (Central Italy) by Means of Stable Isotopes and noble Gas Analysis. Appl. Geochem. 34, 90–101. doi:10.1016/j.apgeochem.2013.02.015
Gould, K. W., Hart, G. N., and Smith, J. W. (1981). Technical Note: Carbon Dioxide in the Southern Coalfields N.S.W.—A Factor in the Evolution of Natural Gas Potential. Proceeding Australas. Inst. Mining Metall. 279, 41–42.
Guo, T., and Zeng, P. (2015). The Structural and Preservation Conditions for Shale Gas Enrichment and High Productivity in the Wufeng-Longmaxi Formation, Southeastern Sichuan Basin. Energy Exploration & Exploitation 33 (3), 259–276. doi:10.1260/0144-5987.33.3.259
Guo, X., and Guo, T. (2012). Theory and Exploration Practice of Puguang and Yuanba Giant Gas Field in Platform Margin. Beijing: Science Press.
He, J. (1995). Preliminary Study on CO2 Natural Gas in Yinggehai Basin. Nat. Gas Geosciences 29 (6), 1–12.
Hoefs, J. (1978). Some Peculiarities in the Carbon Isotope Composition of “Juvenile Carbon”: sTable Isotopes in the Earth Science. DSIR Bull. 200, 181–184.
Hu, G., Yu, C., Gong, D., Tian, X., and Wu, W. (2014). The Origin of Natural Gas and Influence on Hydrogen Isotope of Methane by TSR in the Upper Permian Changxing and the Lower Triassic Feixianguan Formations in Northern Sichuan Basin, SW China. Energy Exploration & Exploitation 32, 139–158. doi:10.1260/0144-5987.32.1.139
Javoy, M., Pineau, F., and Iiyama, I. (1978). Experimental Determination of the Isotopic Fractionation between Gaseous CO2 and Carbon Dissolved in Tholeiitic Magma. Contr. Mineral. Petrol. 67, 35–39. doi:10.1007/bf00371631
Jenden, P. D., Drazan, D. J., and Kaplan, I. R. (1993). Mixing of Thermogenic Natural Gas in Northern Appalachian Basin. AAPG Bull. 77 (6), 980–998. doi:10.1306/bdff8dbc-1718-11d7-8645000102c1865d
Li, D., Li, W., and Wang, Z. (2007). The Natural Gas Genesis Type and Gas-Source at Analysis of Guang'an Gas Field in the Middle of Sichuan Basin. Geology. China 34 (5), 829–836.
Li, J., Li, J., Li, Z., Zhang, C., Cui, H., and Zhu, Z. (2018). Characteristics and Genetic Types of the Lower Paleozoic Natural Gas, Ordos Basin. Marine & Petroleum Geology, 89106–89119.
Li, J., Zheng, M., Guo, Q., and Wang, S. (2019). Forth Assessment for Oil and Gas Resource. Beijing: Petroleum Industry Press, 203–270.
Li, P., Hao, F., Guo, X., Zou, H., Yu, X., and Wang, G. (2015). Processes Involved in the Origin and Accumulation of Hydrocarbon Gases in the Yuanba Gas Field, Sichuan Basin, Southwest China. Mar. Pet. Geology. 59, 150–165. doi:10.1016/j.marpetgeo.2014.08.003
Liao, F., Wu, X., and Huang, S. (2012). Geochemical Characteristics of CO2 Gases in Eastern China and the Distribution Patterns of Their Accumulations. Acta Petrologica Sinica 28 (3), 939–948.
Lin, X., Liu, L., and Wei, L. (2007). Prediction of Calcarenaceous sandstone Gas-Bearing Reservoir in the 4th Member, Xujiahe Formation in Fenggu Area, West Sichuan basin. J. Southwest Pet. Univ. 29 (4), 82–84.
Lin, Y., Wu, S., Xu, Z., and Ni, Y. (2012). Controlling Factors for T3x4 Calcarenaceous sandstone in Fenggu Structure, Western Sichuan Basin. Nat. Gas Geosci. 23 (4), 691–699.
Liu, D., Zhang, W., Kong, Q., Feng, Z., Fang, C., and Peng, W. (2016). Lower Paleozoic Source Rocks and Natural Gas Origins in Ordos Basin, NW China. Pet. Exploration Dev. 43 (4), 540–549. doi:10.1016/s1876-3804(16)30069-6
Liu, Q., Jin, Z., Li, H., Wu, X., Tao, X., Zhu, D., et al. (2018). Geochemistry Characteristics and Genetic Types of Natural Gas in central Part of the Tarim Basin, NW China. Mar. Pet. Geology. 89, 91–105. doi:10.1016/j.marpetgeo.2017.05.002
Liu, S., Lu, X., Hong, F., Fu, X., San, X., Wei, L., et al. (2016). Accumulation Mechanisms and Distribution Patterns of CO2-containing Natural Gas Reservoirs in the Songliao Basin. Beijing: Science Press. 6-12、41-47.
Ma, R. (2012). Main Controlling Factors of Gas Accumulation in the Calcarenaceous sandstone Reservoirs in the 3rd Member of the Xujiahe Formation in the YB Area. Nat. Gas Industry 38 (8), 56–62.
Marshall, J. D., Brenchley, P. J., Mason, P., Wolff, G. A., Astini, R. A., Hints, L., et al. (1997). Global Carbon Isotopic Events Associated with Mass Extinction and Glaciation in the Late Ordovician. Palaeogeogr. Palaeoclimatol. Palaeoecol. 132 (1), 195–210. doi:10.1016/s0031-0182(97)00063-1
Meyerhoff, A. A. (19701969). Developments in Mainland China. AAPG Bull. 54 (8), 1949. doi:10.1306/5d25cbd1-16c1-11d7-8645000102c1865d
Milkov, A. V., and Etiope, G. (2018). Revised Genetic Diagrams for Natural Gases Based on a Global Dataset of >20,000 Samples. Org. Geochem. 125, 109–120. doi:10.1016/j.orggeochem.2018.09.002
Moore, J. G., Backelder, N., and Cunningham, C. G. (1977). CO2 Filled Vesicle in Mid-ocean basalt. J.Valcano. Gestherm. Res. 2, 309. doi:10.1016/0377-0273(77)90018-x
Muffler, F. J. P., and White, D. E. (1968). Origin of CO2 in the Salton Sea Geothermal System, southeastern California. U.S.A. XXIII International Geol. Congress 17, 185–194.
Ni, Y., Dai, J., Tao, S., Wu, X., Liao, F., Wu, W., et al. (2014). Helium Signatures of Gases from the Sichuan Basin, China. Org. Geochem. 74, 33–43. doi:10.1016/j.orggeochem.2014.03.007
Ni, Y., Yao, L., and Liao, F. (2021). Geochemical Comparison of the Deep Gases from the Sichuan and Tarim Basins, China. Front. Earth Sci. 9, 1–22. doi:10.3389/feart.2021.634921
Ohmoto, H., and Rye, R. O. (1979). “Isotope of Sulfur and Carbon,” in Geochemistry of Hydrothermal Deposits. Editor H. L. Barnes (New York: John Wiley & Sons), 509–567.
Orth, C. J., Gilmore, J. S., Quintana, L. R., and Sheehan, P. M. (1986). Terminal Ordovician Extinction: Geochemical Analysis of the Ordovician/Silurian Boundary, Anticosti Island, Quebec. Geol 14 (5), 433–436. doi:10.1130/0091-7613(1986)14<433:toegao>2.0.co;2
Pankina, R. G., Mekhtiyeva, V. L., and Guriyeva, S. M. (1978). Origin of CO2 in Petroleum Gases (From the Isotopic Composition of Carbon). Int. Geology. Rev. 21 (5), 535–539.
Qi, H., and Dai, J. (1981). Discussion on Distribution and Origin of the Gas Pools Containing High Percentage of Carbon Dioxide of China. Pet. Exploration Dev. 2, 34–42.
Qin, S., Yang, Y., Lu, F., Zhou, H., and Li, Y. (2016). The Gas Origin in Changxi-Feixianguan Gas Pools of Longgang Gas Field in Sichuan Basin. Nat. Gas Geosci. 27 (1), 41–49.
Qing, H., and Veizer, J. (1994). Oxygen and Carbon Isotopic Composition of Ordovician Brachiopods: Implications for Coeval Seawater. Geochimica et Cosmochimica Acta 58 (20), 4429–4442. doi:10.1016/0016-7037(94)90345-x
Richet, P., Bottinga, Y., and Javoy, M. (1977). A Review of Hydrogen, Carbon, Nitrogen, Oxygen, Sulphur, and Chlorine Stable Isotope Fractionation Among Gaseous Molecules. Annu. Rev. Earth Planet. Sci. 5, 65–110. doi:10.1146/annurev.ea.05.050177.000433
Sano, Y., Urabe, A., Wakita, H., Chiba, H., and Sakai, H. (2008). Chemical and Isotopic Compositions of Gases in Geothermal Fluids in Iceland. Geochemical J. GJ 19 (3), 135–148.
Shangguan, Z., and Zhang, P. (1990). Active Faults in Northwestern Yunnan Province. Beijing: Seismology Press, 162–164.
She, J., Li, K., Zhang, H., Shabbiri, K., Hu, Q., and Zhang, C. (2021). The Geochemical Characteristics, Origin, Migration and Accumulation Modes of Deep Coal-Measure Gas in the West of Linxing Block at the Eastern Margin of Ordos Basin. Nat. Gas Sci. Engi. doi:10.1016/j.jngse.2021.103965
Shen, P., Xu, Y., Wang, X., Liu, D., Shen, Q., and Liu, W. (1991). Studies on Geochemical Characteristics of Gas Source Rocks and Natural Gas and Mechanism of Genesis of Gas. Lan Zhou. Gansu Sci. TechnologyPress, 120–121.
Song, Y. (1991). Origin of the Natural Gas in Wanjinta Reservoir of the Songliao Basin. Nat. Gas Industry 11 (1), 17–21.
Tang, Z. (1983). Geologic Characteristics of Natural Carbon Dioxide Gas Pool and its Utilization. Nat. Gas Industry 3 (3), 22–26.
Tao, S., Zou, C., Tao, X., Huang, C., Zhang, X., Gao, X., et al. (2009). Study on Fluid Inclusion and Gas Accumulation Mechanism of Xujiahe Formation of Upper Triassic in the Central Sichuan Basin. Bull. Mineralogy, Pet. Geochem. 28 (1), 2–11.
Veizer, J., Ala, D., and Azmy, K. (1999). 87Sr/86Sr, δ13C and δ18O Evolution of Phanerozoic Seawater. Chem. Geology. 161 (1), 59–88. doi:10.1016/s0009-2541(99)00081-9
Wang, K., Chatterton, B. D. E., and Wang, Y. (1997). An Organic Carbon Isotope Record of Late Ordovician to Early Silurian marine Sedimentary Rocks, Yangtze Sea, South China: Implications for CO2 Changes during the Hirnantian Glaciation. Palaeogeogr. Palaeoclimatol. Palaeoecol. 132 (1), 147–158. doi:10.1016/s0031-0182(97)00046-1
Wei, G., Xie, Z., Song, J., Yang, W., Wang, Z., Li, J., et al. (2015). Features and Origin of Natural Gas in the Sinian–Cambrian of central Sichuan Paleo-Uplift, Sichuan Basin, SW China. Pet. Exploration Dev. 42 (6), 702–711. doi:10.1016/s1876-3804(15)30073-2
Wei, J., Wang, Y., Wang, G., Wei, Z., and He, W. (2021). Geochemistry and Shale Gas Potential of the Lower Permian marine-continental Transitional Shales in the Eastern Ordos Basin. Energy Exploration & Exploitation 39 (3), 738–760. doi:10.1177/0144598720979242
Wu, X., Liu, Q., Chen, Y., Zhai, C., Ni, C., and Yang, J. (2020). Constraints of Molecular and Stable Isotopic Compositions on the Origin of Natural Gas from Middle Triassic Reservoirs in the Chuanxi Large Gas Field, Sichuan Basin, SW China. J. Asian Earth Sci. 204. doi:10.1016/j.jseaes.2020.104589
Wu, X., Liu, Q., Liu, G., and Ni, C. (2019). Genetic Types of Natural Gas and Gas-Source Correlation in Different Strata of the Yuanba Gas Field, Sichuan Basin, SW China. J. Asian Earth Sci. 181, 103906. doi:10.1016/j.jseaes.2019.103906
Wu, X., Liu, Q., Liu, G., Wang, P., Li, H., Meng, Q., Chen, Y., and Zeng, H. (2017). Geochemical characteristics and genetic types of Natural Gas in the Xinchang Gas Field, Sichuan Basin, SW China. Acta Geologica Sinica - English Edition 91 (6), 2200–2213. doi:10.1111/1755-6724.13458
Xu, H., Zhou, W., Cao, Q., Xiao, C., Zhou, Q., Zhang, H., et al. (2018). Differential Fluid Migration Behaviour and Tectonic Movement in Lower Silurian and Lower Cambrian Shale Gas Systems in China Using Isotope Geochemistry. Mar. Pet. Geology. 89, 47–57. doi:10.1016/j.marpetgeo.2017.03.027
Yin, F., Liu, R., and Qin, H. (2013). About Origin of Tight sandstone Gas: To Discuss with Academician Dai Jinxing. Pet. Exploration Dev. 40 (1), 125–128. doi:10.1016/s1876-3804(13)60016-6
Zhang, M., Tang, Q., Cao, C., Lv, Z., Zhang, T., Zhang, D., et al. (2018b). Molecular and Carbon Isotopic Variation in 3.5 Years Shale Gas Production from Longmaxi Formation in Sichuan Basin, China. Mar. Pet. Geology. 89, 27–37. doi:10.1016/j.marpetgeo.2017.01.023
Zhang, M., Tang, Q., and Cao, C. (2018a). Oxygen Hydrogen and Carbon Isotope Studies for Fangshan Granitic Intrusion. Acta Petrologica Sinica 3 (3), 13–22.
Zhang, S., He, K., Hu, G., Mi, J., Ma, Q., Liu, K., et al. (2018c). Unique Chemical and Isotopic Characteristics and Origins of Natural Gases in the Paleozoic marine Formations in the Sichuan Basin , SW China: Isotope Fractionation of Deep an High Mature Carbonate Reservoir Gases. Mar. Pet. Geology. 89, 68–82.
Zhang, S., Hu, G., and Liu, S. (2019). Chinese Natural Gas Formation and Distribution. BeijingPetroleum Industry Press, 143–146.
Zhen, S., Huang, F., Jiang, C., and Zheng, S. (1987). Oxygen Hydrogen and Cabon Istope Studies for Fangshan Granitic Intrusion. Acta Petrologica Sinica 3 (3), 13–22.
Keywords: Sichuan Basin, carbon dioxide, δ 13 CCO2, origin, geochemical characteristics
Citation: Dai J, Ni Y, Liu Q, Wu X, Yu C, Gong D, Hong F, Zhang Y and Yan Z (2022) Carbon Dioxide and its Carbon Isotopic Composition of Natural Gas in the Sichuan Basin, SW China. Front. Earth Sci. 10:857876. doi: 10.3389/feart.2022.857876
Received: 19 January 2022; Accepted: 10 March 2022;
Published: 26 April 2022.
Edited by:
Fang-Zhen Teng, University of Washington, United StatesReviewed by:
Ziqi Feng, China University of Petroleum (East China), ChinaShikha Sharma, West Virginia University, United States
Copyright © 2022 Dai, Ni, Liu, Wu, Yu, Gong, Hong, Zhang and Yan. This is an open-access article distributed under the terms of the Creative Commons Attribution License (CC BY). The use, distribution or reproduction in other forums is permitted, provided the original author(s) and the copyright owner(s) are credited and that the original publication in this journal is cited, in accordance with accepted academic practice. No use, distribution or reproduction is permitted which does not comply with these terms.
*Correspondence: Yunyan Ni, bml5eUBwZXRyb2NoaW5hLmNvbS5jbg==; Quanyou Liu, cXlsaXVAc29odS5jb20=