- 1Institue of Geology, Chinese Academy of Geological Sciences (CAGS), Beijing, China
- 2Key Laboratory of Deep-Earth Dynamics of Ministry of Natural Resources, Beijing, China
- 3School of Earth Sciences and Engineering, Sun Yat-sen University, Zhuhai Campus, Zhuhai, China
- 4School of Earth Sciences and Resources, China University of Geosciences, Beijing, China
- 5Department of Mathematical Sciences, Tsinghua University, Beijing, China
- 6School of Geophysics and Measurement-Control Technology, East China University of Technology, Nanchang, China
The Kexueshan Basin in the western Ordos Block is characteristic of typical regional superimposed folds in the Jurassic strata, documenting the main tectonic shortening since the Mesozoic. Field structural investigation, fault kinematic analysis, together with regional structural analysis, we reconstruct two-phase tectonic stress fields related to crustal shortening. Synthesizing the regional structural analysis and geochronology, two stages of tectonic evolution are proposed in the Kexueshan Basin since the late Mesozoic. In the mid-late Middle Jurassic (J2), controlled by NW-SE compaction, NW-SE structures formed in the Kexueshan Basin on the western margin of the Ordos Block, marked by NE-to NNE-trending folds and thrust faults. Growth strata found in the study area constrain the starting time of the NW-SE structures. This shortening is likely related to a combination of the southward convergence of the Siberian plate and the northwestward subduction of the Pacific plate. In the end Cenozoic (N12-N2), the tectonic stress field was transformed to NE-SW compression, resulting in the development of NW- to NNW-trending fold structures, which were superimposed on early NE-to NNE-trending structures to produce regional superimposed folds. The tectonic shortening coincided with the northeastward growth of the Tibetan Plateau.
Introduction
As an important tectonic boundary in the western part of the North China Craton (NCC), the western marginal structural belt of the Ordos Block (OB) has attracted the attention of many researchers for a long time (Figure 1). In previous studies, this belt has been named the “Longxi System” and “the spine of the Qilian-Lvliang-Helan mountain-shaped structure” (Li, 1954), the Helan-Liupan fold belt (Ma et al., 1961), and the Ordos platform fold belt (Huang, 1983). Some later studies indicated that this region was a plate suture zone formed in the early Paleozoic, a Meso-Neoproterozoic rift trough (Zhang, 1983; Zhang, 1989), or a “thrust structural belt on the western edge of Ordos” (Chen, 1986; Tang et al., 1988; Liu et al., 2000). Based on the basin-mountain coupling mechanism, combined with geodynamic analysis, Liu et al. (2000) proposed that the southwestern edge of the Ordos Basin was a peripheral foreland basin formed by Late Triassic-Cretaceous arc-continent collision and continental collision orogeny. U–Pb and Hf isotope studies of Middle-Late Devonian detrital zircons in the southeastern part of the Alxa Block and paleomagnetic analysis show that the Indosinian movement caused the Alxa Block to rotate 32° counterclockwise relative to the North China Block, followed by convergence and amalgamation to form a Triassic continental collision zone (Yang et al., 2014; Yuan and Yang, 2015). Detailed structural geology and sedimentological analysis showed that the northern part of the western margin of the Ordos Block formed a rift basin in the Late Triassic, while the southern part developed a foreland basin controlled by thrust faults (Liu et al., 2013). Liu et al. (1997) proposed an unrestricted lateral extrusion structure model through the north-south structural difference in the western margin, which was reasonably explained from the perspective of structural geology and dynamic mechanism (Liu and Yang, 1996; Liu and Yang., 1996; Liu and Yang, 1997; Liu et al., 2013).
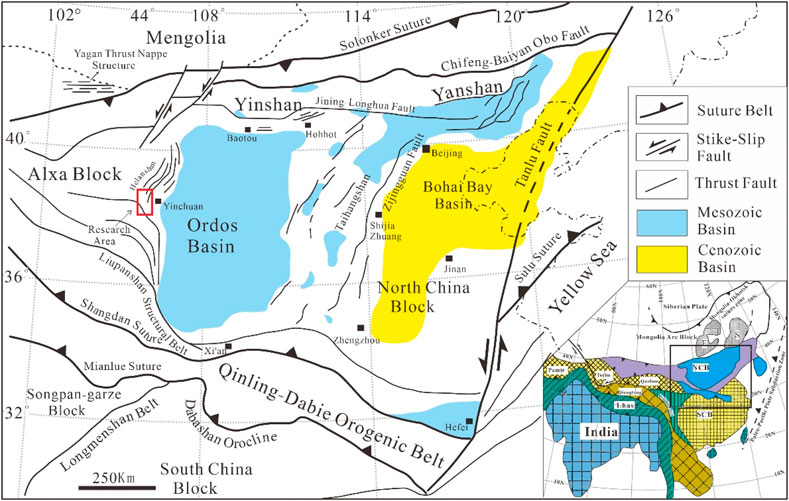
FIGURE 1. Regional structural map of the North China Plate (modified after Liu et al., 2013).
Sedimentary analysis shows that the western margin of the Ordos Block belonged to a residual inner cratonic basin in the Mesozoic; the foreland basins began to form in the Late Jurassic and further developed in the Early Cretaceous, and the current foreland basin pattern was basically formed by the middle of the Cenozoic (Zhao, 2003; Liu et al., 2005; Liu et al., 2006a; Wang et al., 2006). Darby and Ritts (2002) proposed that the Helan Mountains and Zhuozishan areas on the western margin of the Ordos Block represent an intracontinental deformation zone, in which deformation and structural shortening of at least 30% occurred from the Middle-Early Jurassic to the Late Jurassic. Its tectonic shorting and deformation mechanism may have been related to the subduction of the Paleo-Pacific plate. Zhang et al. (2008) concluded that the northern part of the western margin of the Ordos Block is a large-scale continental margin thrust nappe structural system through regional structural investigation and analysis; this system consists of a Mesozoic sedimentary strata and an early Paleozoic continental margin sedimentary cap layer. The main development stage of the thrust structure was the Jurassic, and the cumulative displacement can reach 60–80 km. A recent analysis of the superimposed deformation of the Helan Mountains shows that the western margin of the Ordos Block experienced two main phases of intracontinental shortening and deformation during the end of Triassic in the NNE-SSW direction and during the Middle Jurassic in the NW-SE direction, forming a regional superimposed fold, which is typical of an intracontinental tectonic deformation zone (Cheng et al., 2019; Huang et al., 2015). These studies show that the western margin of the Ordos Block has experienced complicated intracontinental deformation since the Mesozoic, and the tectonic evolution process remains very controversial. In addition to the controversy over the structures in the western margin of the Ordos Block, the kinematic model and the geodynamic origin of this area still remain unclear due to a lack of detailed structural investigations and the fact that there is no comprehensive understanding of regional structures within the western of the Ordos Block, This paper selects the late Mesozoic basin in the Kexueshan basin on the western margin of the Ordos Block (Figure 1), presents a detailed structural analysis, proposes the main tectonic evolution processes since the Mesozoic, and provides a basis for determining the late Mesozoic-Cenozoic tectonic framework of the western margin of the Ordos Block.
Geological Setting
The Kexueshan Basin (KB) is located on the western edge of the Ordos Block and adjacent to the Alxa Block in the northwest; the KB developed as a late Mesozoic basin extending in the N-S direction. It is bounded by Tujingzi to the north, includes the Dazhanchang to the west where it is bounded by Qianguchengzi, extends to the Xinjing coal mine to the south, and is limited by the Liumugao fault to the east. The central part of the KB is flat and surrounded by mountains. The Mesozoic sedimentary characteristics and stratigraphic contact relationships in the basin are consistent with those in the Ordos Block (Wang et al., 2006; Zhao et al., 2006). Ordovician, Devonian, Jurassic, Cretaceous and a few Cenozoic strata are exposed in the study area (Figure 1; Geological Bureau and Mineral Resources of Ningxia Hui Autonomous Region, 1983; Region, 1990; Li et al., 1999). The Mesozoic stratigraphic distribution is characterized by the Jurassic strata exposed in the central part of the KB (Ningxia Hui Autonomous Region Geological Survey Institute, 2017), including the Yan’an Formation (J1-2y), Zhiluo Formation (J2z), and Anding Formation (J3a); the eastern margin is bounded by the Early Cretaceous Miaoshanhu Formation (K1ms), and older strata are exposed south, west and north of the KB.
The Jurassic Yan’an Formation (J1-2y) is mainly distributed in the Wanbulegou, Tujuan, Shuangjuan, Dazhanchang, Heigeda, and Weigou areas; the lithology is characterized by purple, purple-brown, gray-yellow moderately calcareous breccia and conglomerate at the bottom of the Yan’an Formation, with purple-brown calcareous feldspar quartz sandstone, quartz sandstone, gravel-bearing coarse sandstone, and a coal-bearing seam in the middle of the Yan’an Formation. The Yan’an Formation (J1-2y) is the most important coal-bearing layer in the Ordos Basin. In the KB, it mainly comprises sandstones and mudstones intercalated with coal beds (Figure 2). The Xinjing coal mine produces coal mainly from the Yan’an Formation, and the Yan’an Formation can be further divided into five members representing five sedimentary cycles (Liu et al., 2006b). The results of previous studies show that the Yan’an Formation (J1-2y) records the complete evolution of a continental lake from its occurrence and development to its shrinkage and extinction, and the middle three members represent the phases of lake expansion (Li et al., 2015). During the deposition of the last member, along with the evident uplift of the Ordos Basin, the lake shrank considerably, and fluvial facies dominated the western margin of the Ordos Block. There are many feldspar quartz sandstones in the Yan’an Formation, indicative of recycled sedimentation. Then, a short-lived sedimentary hiatus occurred, and the upper part of the Yan’an Formation was exhumed and eroded to varying degrees (Liu et al., 2006a).
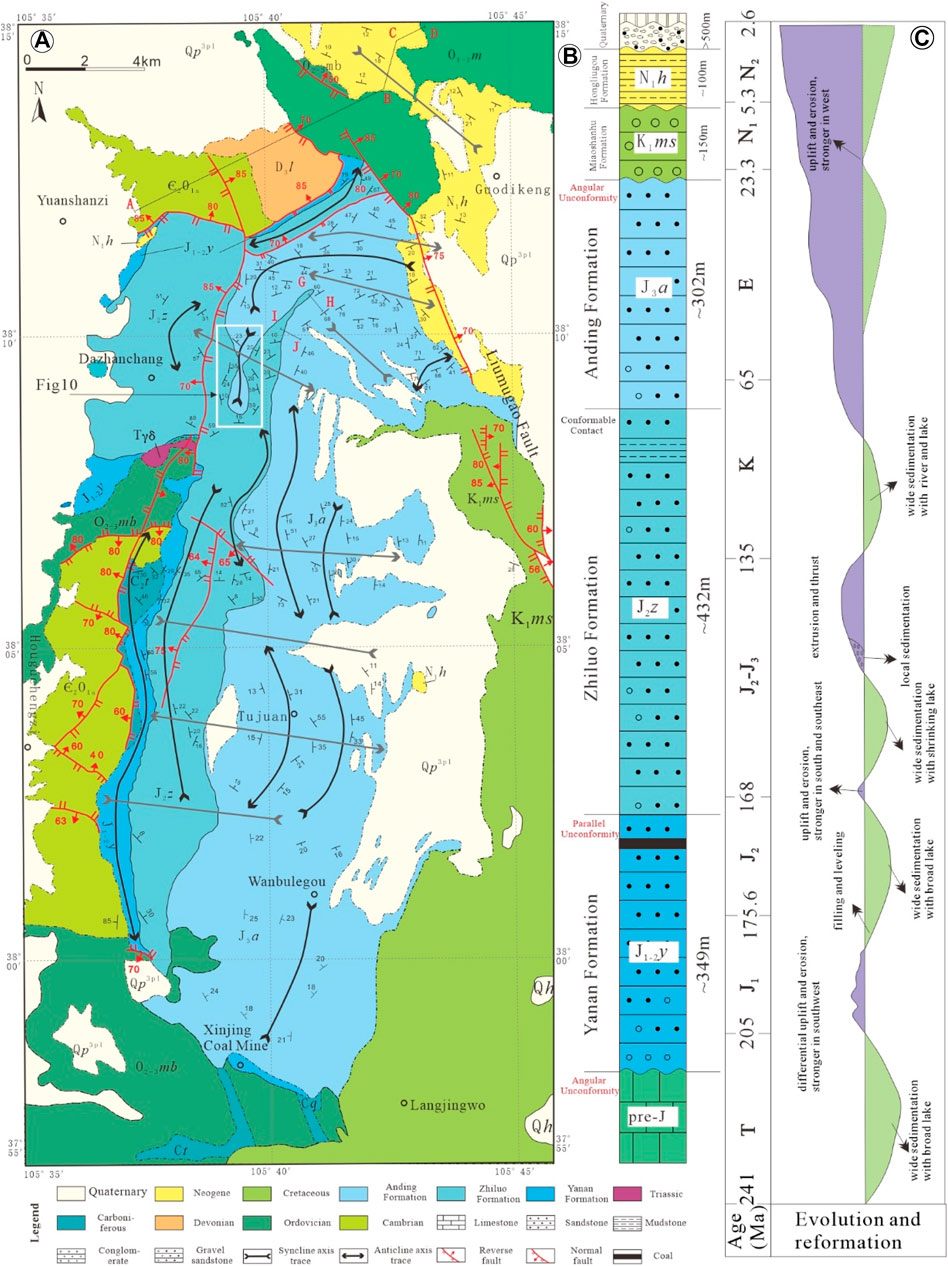
FIGURE 2. (A) Regional geological map of the Kexueshan basin, western margin of the Ordos (KB; adapted from 1/250000 geological map of China (China Geological Survey,2008)). Cross sections of A-B-C-D, G-H and I-J. See Figure 1 for location of this map. (B) Schematic stratigraphy of the Jurassic strata in the Kexueshan Basin. (C) Simplified schematic figure of evolutional history of the Ordos Basin from Triassic to Neogene (adapted after Liu et al., 2006).
The Zhiluo Formation (J2z) is widely distributed in the study area and is found from north of the Xinjing coal mine to the Dazhanchang. The Zhiluo Formation (J2z) and the underlying Yan’an Formation (J1-2y) are in parallel unconformity contact, and the Zhiluo Formation (J2z) and the Anding Formation (J3a) are in conformable contact.
The Zhiluo Formation is a significant uranium-bearing layer in the Ordos Basin (Liu et al., 2006b). Its lithology is comparatively uniform and mainly comprises sandstones, with a small proportion of mudstones. Conglomeratic sandstone is developed locally in the Zhiluo Formation (Figure 2). Braided rivers occurred in the early phase, and meandering and anastomosed rivers then dominated in the later phase. At its base, a unit of thick-bedded conglomerate was deposited, which can be correlated across the whole basin (Zhao et al., 2006). Many large lithic fragments are found in the Zhiluo Formation sandstones, including extrusive rocks and quartzite grains. Compared with the Yan’an Formation, the coarser sediments with more lithic fragments in the Zhiluo Formation may indicate tectonic disturbance in the source areas.
The Anding Formation (J3a) has a wider distribution range than the Yan’an Formation (J1-2y) and Zhiluo Formation (J2z), with obvious lithological and lithofacies differentiation from south to north. The sedimentary rocks south of Tujuan are relatively coarse in grain size, and the proportion of coarse clastic rocks is higher than that in the northern sedimentary rocks. The lithologies are mainly purple and gray-brown conglomerate and sandstone, with interposed gray-purple feldspar quartz sandstone. The upper part often contains purple-red calcareous siltstone, argillaceous siltstone and a small number of glutenite lenses, and the sedimentary rocks are mainly purple-red. During its deposition, the color of the sediments changed from gray and grayish green to aubergine and olive, possibly indicating a change in climate (Zhao et al., 2006). The main sedimentary characteristics are fluvial facies and bank-flood floodplain sedimentary facies. The sediments of the Anding Formation are much finer than those of the Zhiluo Formation.
The Anding Formation (J3a) is in conformable contact with the underlying Zhiluo Formation (J2z), and the top is in unconformable contact with the clastic rocks of the Lower Cretaceous Miaoshanhu Formation (K1ms). The eastern mountain is dominated by Early Cretaceous Miaoshanhu Formation (K1ms) strata comprising a set of sedimentary rocks dominated by clastic rocks, with an overall upward thinning sequence structure belonging to alluvial fan-lacustrine deposits. The lower part of the Miaoshanhu Formation (K1ms) has brown-red, massive medium-coarse conglomerates and coarse boulders, intercalated conglomerate sandstone lenses and a small amount of silty mudstone. The upper part is a light brown-red and light gray-green thick layer of coarse boulders, glutenite and interbedded gray-green mudstone, siltstone, and sandy limestone. The southern and western mountains are composed of the Miboshan Formation (O2-3mb) limestone and Abuqiehai Formation (Є2-O1a) moderately thin microcrystalline limestone with argillaceous bands. Triassic granodiorite intrusions (Tγδ) in the western part of the study area are produced in the form of stock (Bai and Lu, 2009). The pluton is in intrusive contact with the surrounding limestone of the Abuqiehai Formation (Є2-O1a) and the feldspar quartz sandstone of the Jurassic Yan’an Formation (J1-2y). The northern part of the study area is dominated by the Ordovician Majiagou Formation (O1-2m) with gray-yellow fragmented crystalline limestone and the Devonian Laojunshan Formation (D3l) with gray-purple and gray-green calcareous siltstone. The lower part of this formation is dominated by purple-red medium-thick calcareous conglomerate, and the upper part is gray-purple calcareous siltstone with a small amount of glutenite. The lithological combination is characterized by piedmont accumulation-fluvial lacustrine deposits.
Methodology
Stress Inversion of Fault-Slip Vectors
The reconstruction of the paleotectonic stress field is one of the important topics in the study of structural deformation, providing a basis for regional structural evolution and structural reconstruction (Zhang et al., 2003a; 2003b). Interlayer sliding and fold-related fault kinematics, as an important method for the restoration of the paleotectonic stress field in a fold deformation zone, are gradually applied in structural analysis to study the stress mechanism of crustal shortening (Shi et al., 2012; Shi et al., 2013a; Shi et al., 2013b; Shi et al., 2015b).
Mesoscale shear faults, notably slickensides, are widespread in geological terrains that are affected by brittle deformation. Shear faults permit the acquisition of so-called fault-slip vector in the field. These data consist of orientations of faults and slip sense or direction from kinematic indicators such as slickensides. By now, fault-slip vectors are almost routinely acquired in structural, mostly neo-tectonic, field studies (Shi et al., 2015b). In this paper, we using the Fault software from Géophysique et Géodynamique Laboratore, Université de Paris-Sud, France (Carey, 1979; Angelier, 1984), yielding three principal stress axes (σ1, σ2, σ3). The reliability and regional significance of a stress field have to be considered, including data collection, separation, calculation so on (see Mercier et al. (1991), and Sperner and Zweigel (2010) for the details). The usage of such data sets for regional tectonic analyses is motivated by the availability of software packages enabling rapid processing of strain increments or states of paleo-stress from fault-slip data, known as fault-slip inversions. To define the formation mechanism of the superposed folds, we reconstructed their paleo-stress fields in this work based on the syn-folding fault kinematic methodology. Better definition of a paleo-stress field can be achieved by calculating fault slip vectors comprising dip directions and angles of fault planes, pitch directions and angles of striations, and strike-slip directions.
In this paper, we dominantly introduce two approaches to determine a paleo-stress direction regarding syn-tectonic shortening by measuring folding-related striations based on the previous studies (Shi et al., 2007; Shi et al., 2015a). One of the approaches is to measure the striations on bedding (S0) that are largely perpendicular to the fold hinge as the result of the mutual slipping along bedding planes within sediments during folding deformation in the study area. Another method is to measure the striations presenting on fault planes and coevally parallel to bedding, which generally indicate the orientation of initial strike-slip movements on fault planes, coincident with the shortening of the original units (see Shi et al., 2015b for the details).
Structural Analysis in the Kexueshan Basin
Structural Deformation and Fault Kinematic Analysis
Relevant structural analysis was carried out in the KB on the western margin of the Ordos Block. The results of regional geological mapping showed that two sets of fold structures have developed in this area, namely, fold structures extending in the NW-SE and NE-SW directions, forming superimposed folds (Figure 2). Based on the structural maps and field observations, the main superimposed fold structure styles in this area are “T-shaped”, “crescent-shaped”, “dome-shaped”, etc. (Figure 2; Ramsay, 1987). The edge of the KB is mainly controlled by thrust faults. The faults mostly cut the late Mesozoic-Cenozoic strata and locally cut the Cambrian-Ordovician strata, indicating that the fold-thrust deformation has mainly developed since the late Mesozoic. Regional structural analysis shows that most NE-SW-trending thrust faults are cut by NW-SE-trending faults, which roughly indicates that the NE-SW-trending faults in this area were active earlier than the NW-SE-trending faults. The KB is preliminarily confirmed to have experienced two main strong tectonic shortening and deformation events since the late Mesozoic. In this research work, some typical structures in the field were investigated, and structural analysis was carried out from three aspects, namely, NE-SW-oriented structures, NW-SE-oriented structures and superimposed structures, to obtain the two-phase structural shortening and deformation events in the KB.
NW-SE Structural Shortening Deformation
A large Jurassic stratum is exposed in the study area, which was subjected to strong tectonic compressive stress, forming a series of NE-SW-trending anticline and syncline structures with various fold styles. According to the size of the limb angle, open folds (Figure 3E, Figure 4A), medium-normal folds, and closed folds are found in the field; according to the differences in fold axis morphology, there are arc folds, box-shaped folds, and flexural structures (Song et al., 2013). The strata of the southwestern Anding Formation (J3a) at Guodikeng in the northern part of the study area are strongly deformed, some strata are nearly vertical or even overturned, and some folds retain a series of simultaneous thrust faults and associated secondary faults (Figure 4B).
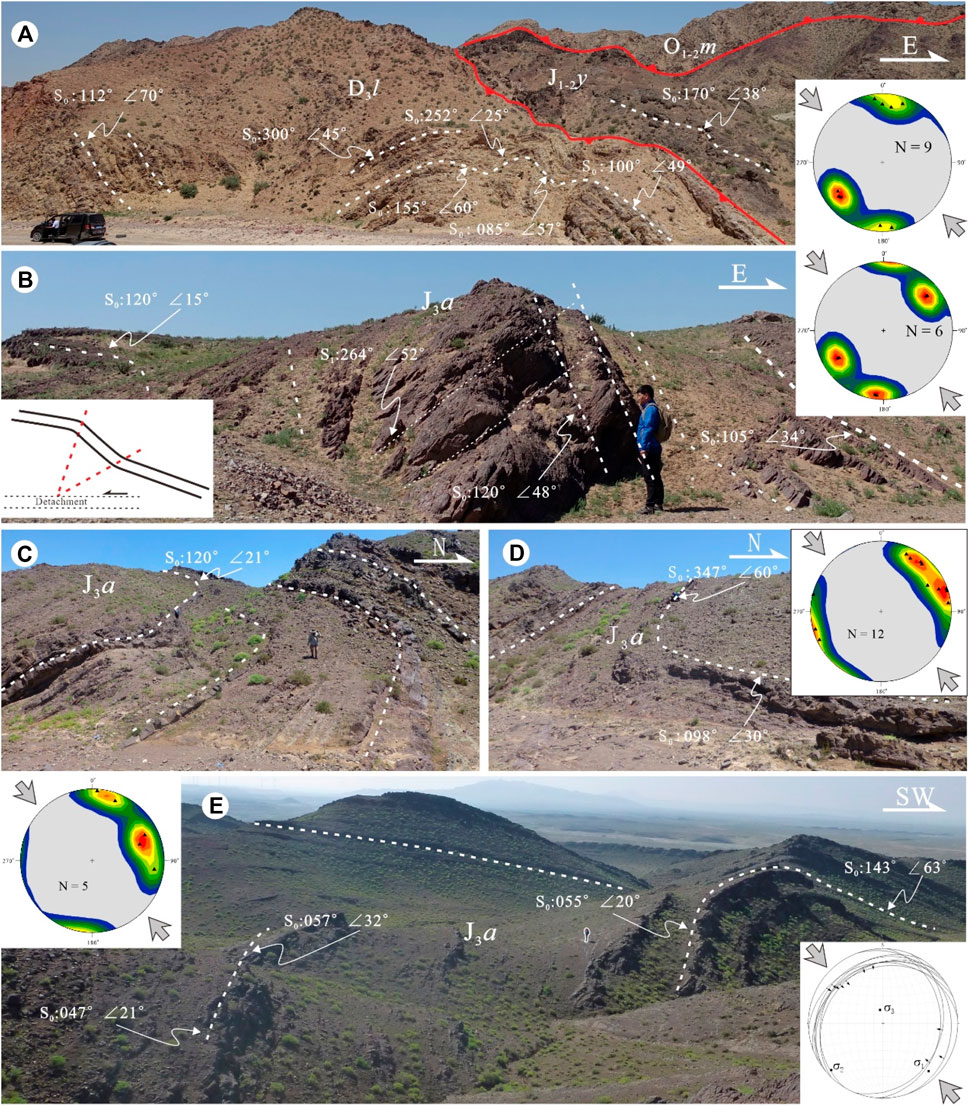
FIGURE 3. Deformation of the NW-SE shortened structure in Kexueshan basin (Results of slip vectors and fold hinges). (A) Composite fold of outcrop-scale and lower hemisphere, equal-area stereographic diagram plotted from bedding planes of the fold indicating NW-SE shortening; (B) Flexure, a flexural structure has developed in the Anding Formation indicating NW-SE shortening; (C,D) Box fold indicating NW-SE compressive stress; (E) Wide anticline indicating NW-SE shortening. Lower hemisphere, equal-area stereographic diagram of fold hinges largely shows NW–SE shortening.
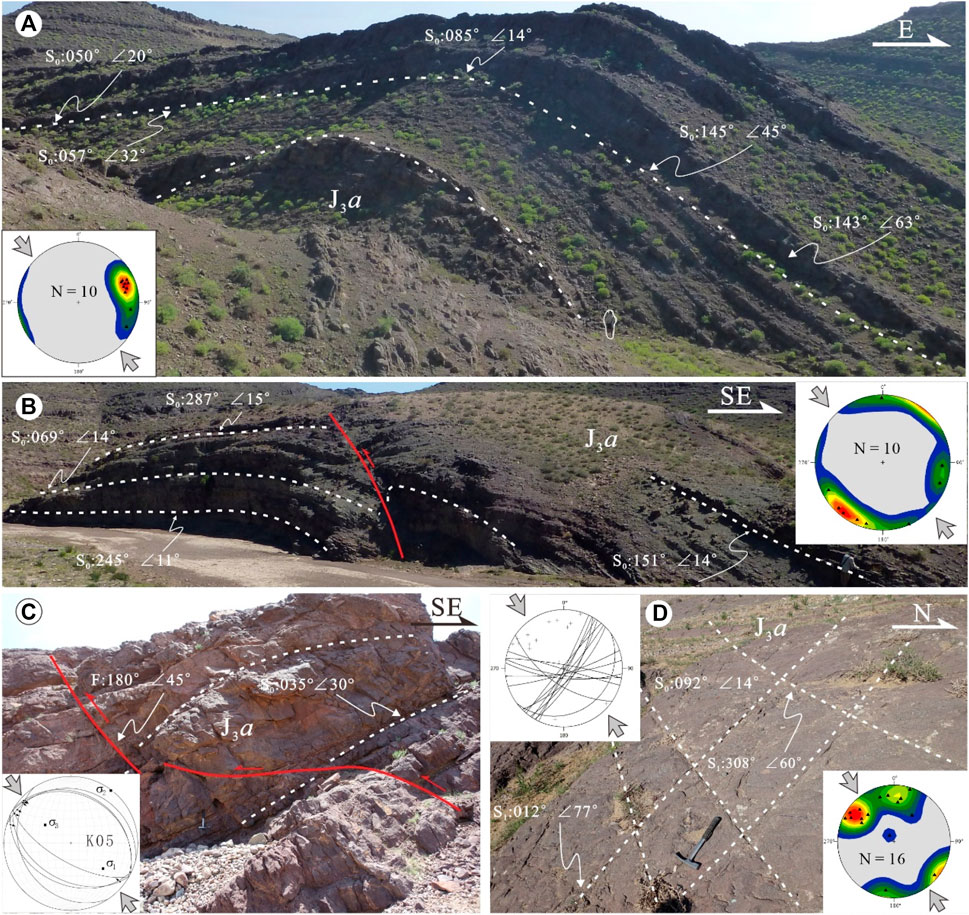
FIGURE 4. Deformation of the NW-SE shortened structure in Kexueshan basin (Results of slip vectors and fold hubs) (A) Broad anticline of outcrop-scale and lower hemisphere, equal-area stereographic diagram plotted from bedding planes of the fold indicating NW-SE shortening; (B) Breaking fold indicating the existence of a NW-SE compressive paleostress field; (C) Thrust fault and fault slip vectors indicate the effect of NW-SE compression; (D) “X-shaped” conjugate shear joints in Anding Formation show that joints are controlled by the compressive stress in the NW-SE direction.
At the northern margin of the KB, the Laojunshan Formation (D3l) is unconformably thrust over the gray-green sandstone of the Yan’an Formation (J1-2y); the limestone of the Majiagou Formation (O1-2m) is thrust over the Yan’an Formation (J1-2y) (Figure 3A), and the structure has been shortened and strongly deformed in this area. The Laojunshan Formation (D3l) has developed a fan-shaped compound anticline. The left limb of the fold is an “S”-shaped fold, and the right limb is a “Z”-shaped fold. The fold axis extends in the NE-SW direction. The results of statistical analysis of the fold hinge roughly indicate the effect of NW-SE compression (Figure 3A). Near Tujuan in the middle of the KB, a flexural structure has developed in the Anding Formation (J3a) (observation point K06 in Figure 3B). The gentle strata suddenly became steep, the fold surface is bent into a knee shape, and the rock surface attitudes are 120°∠15°, 120°∠48°, and 105°∠34°. The stereographic projection method is used to calculate the data of the fold hinge, and the statistics of the hinge indicate the occurrence of shortening deformation in the NW-SE direction (Figure 3B). At observation point K60, an outcrop-scale box fold has developed in the Anding Formation (Figures 3C,D). The fold limb has a steep dip, the turning end is flat and wide, and the fold hinge extension position statistics show that it is close to NE-SW (Figure 3D), which indicates that it formed by NW-SE compressive stress. There are open folds in the Anding Formation (J3a) west of Guodikeng (Figure 3E, observation points K55 and K57). The angle between the limbs of the anticline is 70°–120°, and the fold measurement and statistical analysis show compressive deformation in the NW-SE direction. At this point, the sliding phenomenon between the folds can be observed, and calcite has developed growth lines. The analytical results for the fault-slip vectors indicate the effect of NW-SE compressive stress (Figure 3E).
North of Dazhanchang (Figures 4A,B; observation points 55, 67), the fold limb angles developed in the Anding Formation (J3a) are 70°–120°, which mark open folds. The field measurement of limb attitudes yield 9 pivot data, and the polar density maps of the lower hemisphere equal-area stereographic projection are arranged in the NE-SW direction (Figures 4A,B), indicating the existence of a NW-SE compressive paleostress field. Due to the differences in lithology in various regions and the diverse compressive deformation coefficients of similar rocks, a thrust fault has developed on the right flank of the fold (Figure 4B) with a fault throw of approximately 1 m. Observation point K73 in the Anding Formation (J3a) has developed a recumbent fold with two limbs spaced less than 10 m apart. The inversion results for the fold hinge are scattered in the NE-SW direction also indicates the occurrence of shortening stress in the NW-SE direction. In summary, the analysis of folds with different shapes shows that this area is controlled by compressive stress in the NW-SE direction and is mainly characterized by the development of NE-SW-trending fold structures.
At observation point K05 east of Dazhanchang, the moderately thick sandstone of the Anding Formation (J3a) has developed an outcrop-scale thrust fault (Figure 4C), and fault slip has produced obvious calcite lineations on the fault plane (Figure 4C). The statistical result for the slip vector indicates the effect of NW-SE compression (Figure 4C). In the sandstone of the Anding Formation (J3a) near Tujuan (observation point K04), “X-shaped” conjugate shear joints have developed (Figure 4D). The purple-red pebbly sandstone is cut into a diamond-shaped checkerboard, and the two sets of joints are arranged nearly equidistantly; part of the gravel is cut off, the joint surfaces are straight and clean, and the data projection results for the joint surfaces show that joints are controlled by the compressive stress in the NW-SE direction.
In recent years, some researchers have combined growth strata with tectonic chronology to further reveal the time limits of regional tectonic events and constrain the dynamics and kinematics of the basin (Wang et al., 2017a; Liu et al., 2018) and have made good research progress. There are two types of growth strata: fold growth strata and thrust fault growth strata. Growth strata are often found in orogenic foreland basins. In the process of structural deformation, newly deposited strata from nearby provenances form growth folds and growth strata related to thrusting. At present, scholars recognize two main types of deformation mechanism models of growth strata (Figure 5): a. Hinge migration and b. Limb rotation. The characteristics of growth strata controlled by the two different deformation mechanisms are quite distinct. The characteristics of growth strata formed by hinge migration are as follows: the limb inclination angle of the growth strata remains unchanged, and the fold axis of the growth strata continues to change with structural deformation. Obvious growth triangles can be observed on the vertical section or on the seismic section. In growth strata controlled by the rotation of the limbs, the strata on the side of the rotation are subject to continuous changes in the mechanical direction, resulting in the continuous active structural deformation of the axial plane with tectonic activity (Figure 5).
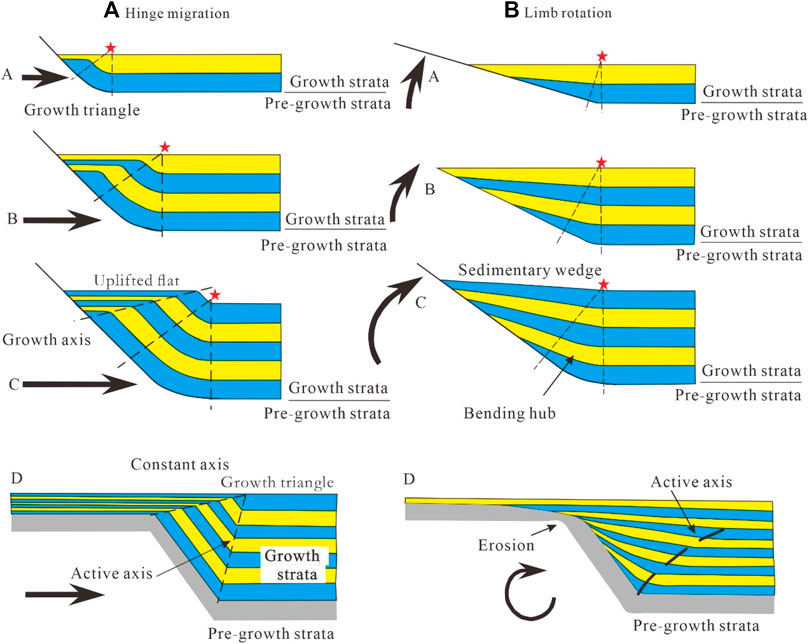
FIGURE 5. Two modes of growth strata (modified after Ford et al., 1997). (A) Model of hinge migration; (B) Model of limb rotation; (A–C) represent the growth strata development process and the fold axis change; (D) is the final form of the growth strata, in which the growth axis under the pivot migration mode is always in a constant state, and the growth triangle can be clearly identified, In the wing rotation mode, the growth axis is active and migrates to the front edge, and the growth triangle is not obvious.
The KB is located in the northern part of the thrust tectonic belt on the western margin of the Ordos Block. On the eastern side of Dazhanchang in the KB, synsedimentary structural growth strata developed in the Jurassic Zhiluo Formation (J2z) and Anding Formation (J3a) at Songjigou. Two profiles were measured in the field, section G-H (Figure 7) and section I-J (Figure 6). The locations are shown in Figure 2. The two measured sections record the dynamic process of shortening and deformation of the Middle-Late Jurassic structures and the related thrust faults and thrust fold structural styles. Through the analysis of growth strata, the time limits of structural deformation can be well defined (Ford et al., 1997; Zhang P. Z et al., 2006; Liu et al., 2018). Growth strata are a special style of synsedimentary structural deformation, and their bottom age can be used as the starting age of growth folds to constrain the time limit of structural deformation. The growth strata in the study area (Figures 6, 7) mark the time limit of the early NW-SE compression in the KB that caused shortening and structural deformation, although sedimentary strata that can be sampled for dating have not yet been found in the Zhiluo Formation (J2z) and Anding Formation (J3a) in the study area. However, new progress has been made regarding the chronological data of growth strata in the Middle Jurassic Changhangou Formation (J2c) in Daqingshan on the northern margin of the Ordos Block. Scholars have obtained the U–Pb age of zircons in the middle-lower volcanic ash interlayers of the growing strata and restricted the onset of this period of tectonic compression to the late Middle Jurassic (∼170 Ma) (Wang et al., 2017b). The dynamics of this tectonic shortening event are generally attributed to control by the East Asian multidirectional convergence system during the Middle-Late Jurassic (∼165 Ma) (Zhang Y. Q et al., 2006; Dong et al., 2008), which may have been related to the combined effect of the southward convergence of the Siberian plate and the NW subduction of the Pacific plate.
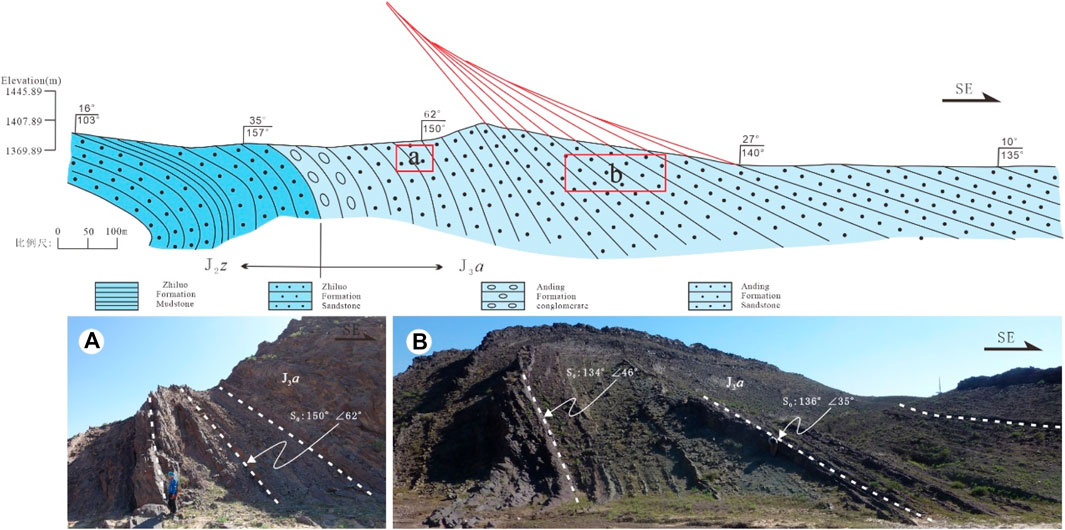
FIGURE 6. Structural section of Growth strata in Songjigou (I–J) (Point K38, The position is shown in Figure 2) (A,B) are typical photos of the section, reflecting the characteristics of the rapid changes in the occurrence of the sedimentary strata and the rapid thickness after a short distance.
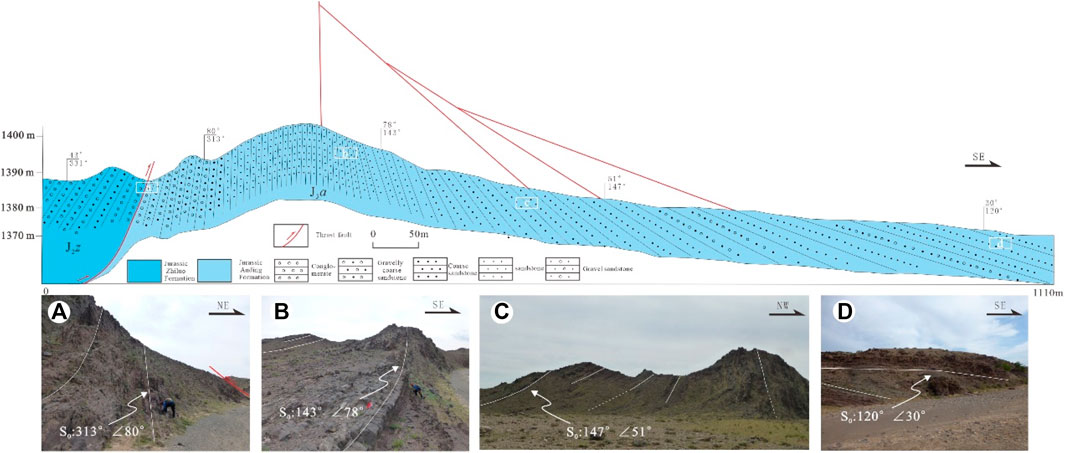
FIGURE 7. Structural section of Growth strata (G-H) (Point K38, the position is shown in Figure 2) Figure (A–D) are typical photos of the section, reflecting the characteristics of the rapid changes in the occurrence of the sedimentary strata and the rapid thickness after a short distance.
NW-SE Structural Shortening Deformation and its Tectonic Stress Field
A series of NW-SE-striking folds and thrust structures are also developed in the Jurassic strata in the study area. This paper analyzes some typical outcrop-scale structures. The moderately thin sandstone of the Anding Formation (J3a) from east of Dazhanchang in the northern part of the KB to Guodikeng is strongly deformed, and large-scale vertical folds can be seen in many places, which may indicate superimposed deformation. Observation point K58 (Figure 8A) has developed superimposed folds. The inclination angle of the fold pivot is between 14° and 21°, and the axial inclination angle is in the range of 47°–70°. According to the classification criteria of the fold position, the fold is described as a plunging and inclined fold (Song et al., 2013). The stereographic projection results for the fold hinge indicate that it was controlled by the stress field of NW-SE compression. The results of the interlayer sliding vector analysis reveal the existence of the NE-SW compression stress field, indicating that the vertical fold was controlled by a two-phase compressive stress (Figure 8A).

FIGURE 8. Structural section on the northern margin of the Kexueshan basin (The section position is shown in Figure 2).
An outcrop-scale vertically dipping fold (Figure 8B) can be seen in the sandstone of the Anding Formation (J3a) at observation point K91. The lower hemisphere equal-area stereographic projection of the fold hinge data indicates that it is controlled by a NE-SW-directed compressive stress field. At the top of the northern mountain in Dazhanchang (observation point K95), the Anding Formation (J3a) exposes a broad and gentle syncline (Figure 8C). The results for the interlayer slip vector and the stereographic projection of the fold hinge all indicate this NE-SW direction of tectonic compressive stress. Well-developed “X”-shaped conjugate shear joints are present in the Anding Formation (J3a) near Tujuan in the KB (Figure 8D). The joint surfaces are straight and smooth, with large inclination angles and long extent. The joint spacing is small and equidistant. The projection results for the joint surfaces show that they are affected by the NE-SW-directed tectonic stress field. At observation point K01 of the Xinjing coal mine in the north, the Ordovician Miboshan Formation (O2-3mb) has been thrust onto the Yan’an Formation (J1-2y) sandstone; the fault plane has developed fine rough grooves, and the sliding vector projection results indicate that the thrust extrusion in this period was controlled by the NE-SW extrusion stress (Figure 8E). These structural analyses confirm that strong NE-SW crustal shortening occurred in the KB.
Analysis of Superimposed Deformation
Typical small regional superimposed folds have developed near Dazhanchang in the middle of the KB, showing a relatively complete dome-basin structure (Figure 2, Figure 9A). The latest strata affected by this fold deformation are the Zhiluo Formation (J2z) and Anding Formation sandstone (J3a).
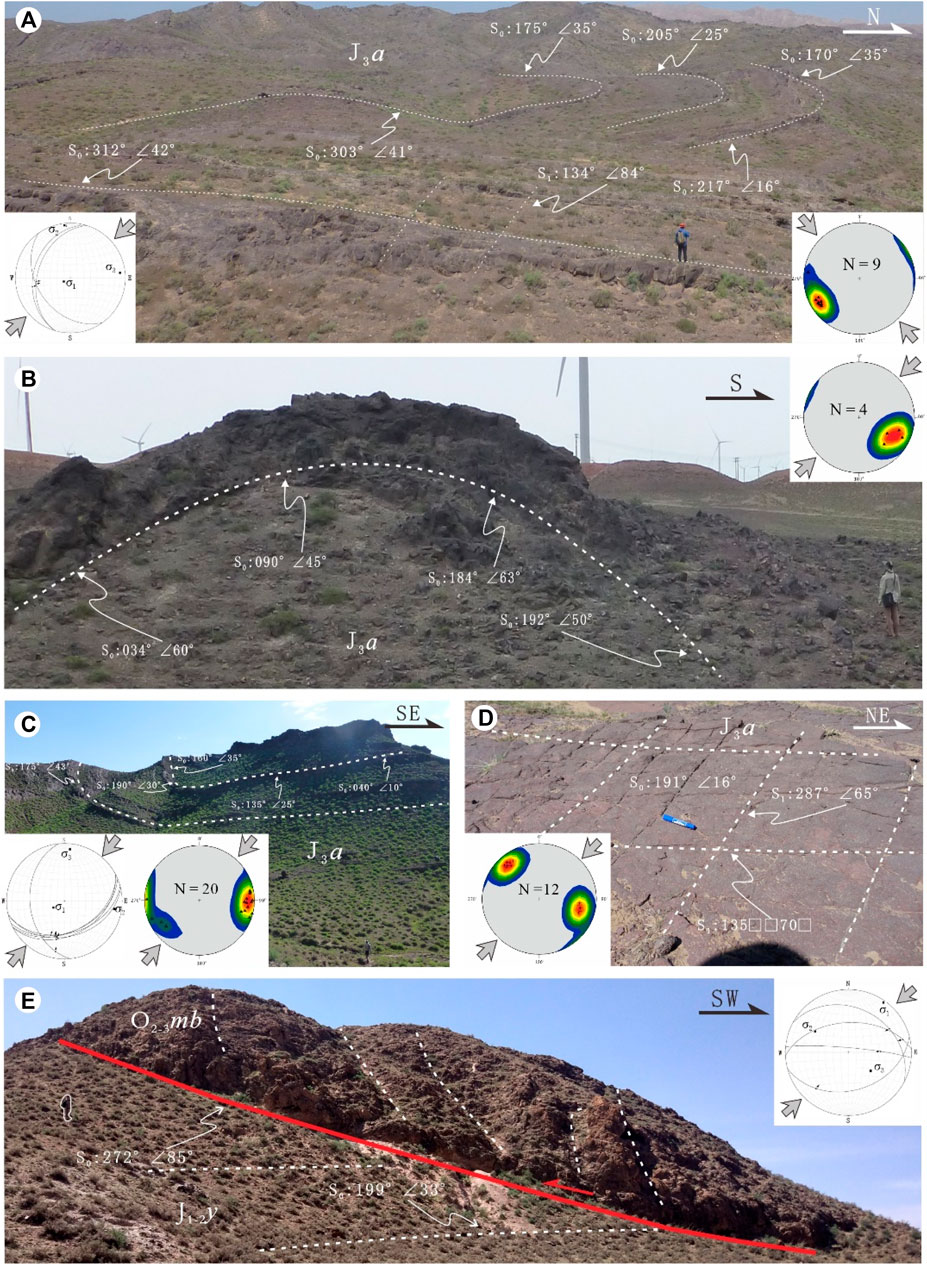
FIGURE 9. Deformation of the NE-SW shortened structure in Kexueshan basin. (A) large-scale vertical folds can be seen in Anding formation, the stereographic projection results for the fold hinge indicate that it was controlled by the stress field of NW-SE compression. The results of the interlayer sliding vector analysis reveal the existence of the NE-SW compression stress field; (B) An outcrop-scale vertically dipping fold can be seen in the sandstone of the Anding Formation; (C) The results of slip vectors and poles of fold indicate NE-SW compressive stress; (D) “X”-shaped conjugate joint in Anding formation indicate the effect of NE-SW compression; (E) the Ordovician Miboshan Formation (O2-3mb) has been thrust to overlie the Yan’an Formation (J1-2y) sandstone, Thrust fault structure and the result of slip vectors confirming the strong NE-SW crustal shortening occurred in Kexueshan basin.
At observation point K13 (Figure 9B), small, superimposed syncline basins can be seen spreading in the north-south direction. The graphical interpretation of remote sensing data and the analysis of stratigraphic attitudes show that the basin is caused by shortening in the NW-SE and NE-SW directions, and the topography of superimposed folds resembles a “dumbbell shape” with a clear structure and is called an effective superimposed fold (Ramsay, 1987). NW-SE compression controls the syncline structure oriented in the north-south direction in the basin. Due to shortening in the NE-SW direction, the anticline crosses and overlaps, the dorsal hinge is tilted, and the syncline hinge has risen, forming a saddle structure (Song et al., 2013). At observation point K15, the Zhiluo Formation (J2z) thick sandstone is nearly horizontal; two dense and nearly orthogonal vertical cleavages have developed, and partial replacement has occurred. The bedding attitudes are is 033°<70° and 120°<70° (Figures 9B,C), and the bedding attitude is 335°<13°, which indicates that the basin was controlled by crustal shortening in both the NW-SE and NE-SW directions.
At observation points K14 and K16 on the western edge of the basin (Figures 9D,E), a nearly upright cleavage has developed in the Anding Formation (000°<15°), and the attitudes of cleavage are 250°<85° and 248°<70°, indicating the existence of the NE-SW-directed extrusion stress field. Another stage of cleavage developed at observation point K20 (Figure 9I) in the central part of the superimposed deformed basin, and the attitudes of cleavage are 301°<42° and 330°<59°, indicating that the cleavage in this period was formed by NW-SE structural extrusion. Two sets of diamond-shaped intersecting cleavages have developed at observation points K17, K18, and K19 in the Anding Formation (J3a) in the northern part of the basin (Figures 9F–H); the stratum attitude is 110°<25°, and cleavage planes and stratum planes intersect obliquely, NW-SE compression-related interlayer cleavage attitudes are 301°<42°, 330°<59°, and 332°<60°, and the attitudes of interlayer cleavage related to NE-SW structural shortening are 237°<54°, 240°<60°, and 242°<58°. The two sets of cleavages at observation point K19 (Figure 9H) have an obvious crosscutting relationship. The cleavage controlled by NW-SE compression is cut by the cleavage controlled by NE-SW crustal shortening, which shows that the structural extrusion deformation in the NE-SW direction occurred later than that in the NW-SE direction. In addition, there are slip vectors at observation points K21 and K23 at the northern and southern ends of the superimposed deformation basin. The inversion results for the slip vectors on the northern margin indicate NW-SE structural compression (Figure 9J), and the results for the slip vectors on the southern margin indicate NE-SW structural compression (Figure 9K). A small-scale superimposed deformation syncline has developed at K22 in the Anding Formation (J3a) at the northern observation point of the small basin (Figure 9L). In the early stage, the basic outline of synclinal folds was formed due to extrusion in the NW-SE direction. Later, due to extrusion in the NE-SW direction, the syncline hinge was bent into a wide and gentle “Z” shape, which produced a superimposed fold at the outcrop scale. This shows that the NW-SE tectonic stress field occurred earlier than the NE-SW tectonic stress field.
In summary, the analysis of the deformation of superimposed folds and the crosscutting relationship between the cleavages associated with tectonic compression show that since the Middle-Late Jurassic, the KB has experienced two main periods of structural shortening and deformation oriented NW-SE and NE-SW, and the NW-SE crustal shortening and deformation occurred earlier than the NE-SW deformation; the later NE-SW crustal shortening event basically shaped the tectonic pattern of the KB.
Superimposed Deformation Tectonic Stress Field
Regarding the tectonic stress field in the superimposed fold area, it is necessary to consider the deformation relationship between the earlier and the later fold deformation and fold-related faults. According to the field measurement data, a structure screen is applied to extract the structural deformation patterns at different stages, including the interlayer slip vector, to measure and process tectonic elements at different stages and to restore the tectonic stress field in the superimposed fold area (Shi et al., 2007; Shi et al., 2012; Shi et al., 2015b). In accordance with the abovementioned ideas and methods, this paper restores the paleotectonic stress field in the superimposed fold deformation zone of the Kexueshan Basin. This work mainly involved fold and fault kinematic analysis of the Mesozoic and Cenozoic strata in the KB, combined with the two phases of structural deformation analysis, and restored the two phases of the tectonic stress field (Figures 10A,B; Table 1).
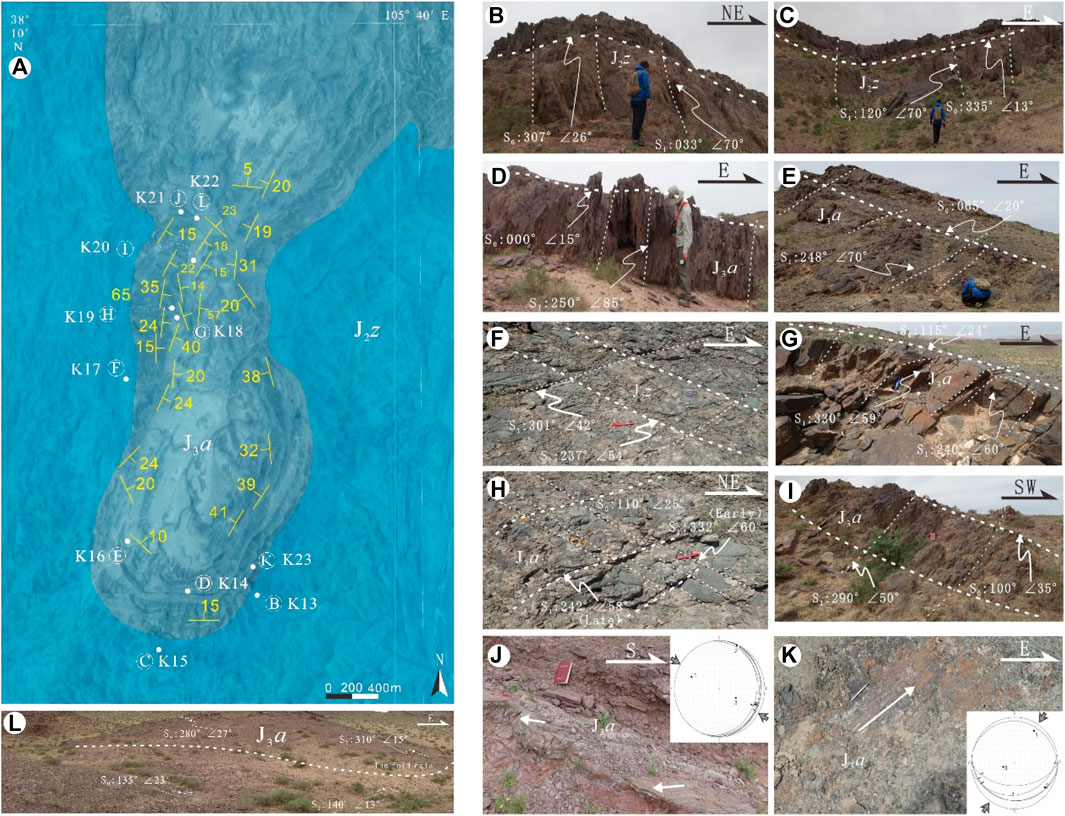
FIGURE 10. Characteristics of superposed deformation and typical slip vectors in Kexueshan basin. (A) Remote sensing map of syncline basin; (B–E,I) Cleavage of bedding around the syncline basin; (F–H) Cutting relationship of two phase cleavage; (J,K) Two-stage compression, NW-SE and NE-SW, in the superposed syncline basin; (L) Superimposed deformation syncline.
Observation point K38 at the northern edge of the study area (Figures 10A,B; Figure 11) is located in the superimposed deformation zone of the Anding Formation in southwestern Guodikeng. The stratum attitude is 145°<25°, and the stratum has not been overturned. There are many secondary calcite stretching lineations developed in the rock layer. The calcite layer is approximately 1–2 cm thick and retains the sliding vector of the shortening deformation that produced the two-stage structure. The lower hemisphere equal-area stereographic projection of the sliding vector retained by the bottom calcite indicates NW-SE tectonic compression, and the overlying late calcite retains the sliding vector formed by NE-SW compression.
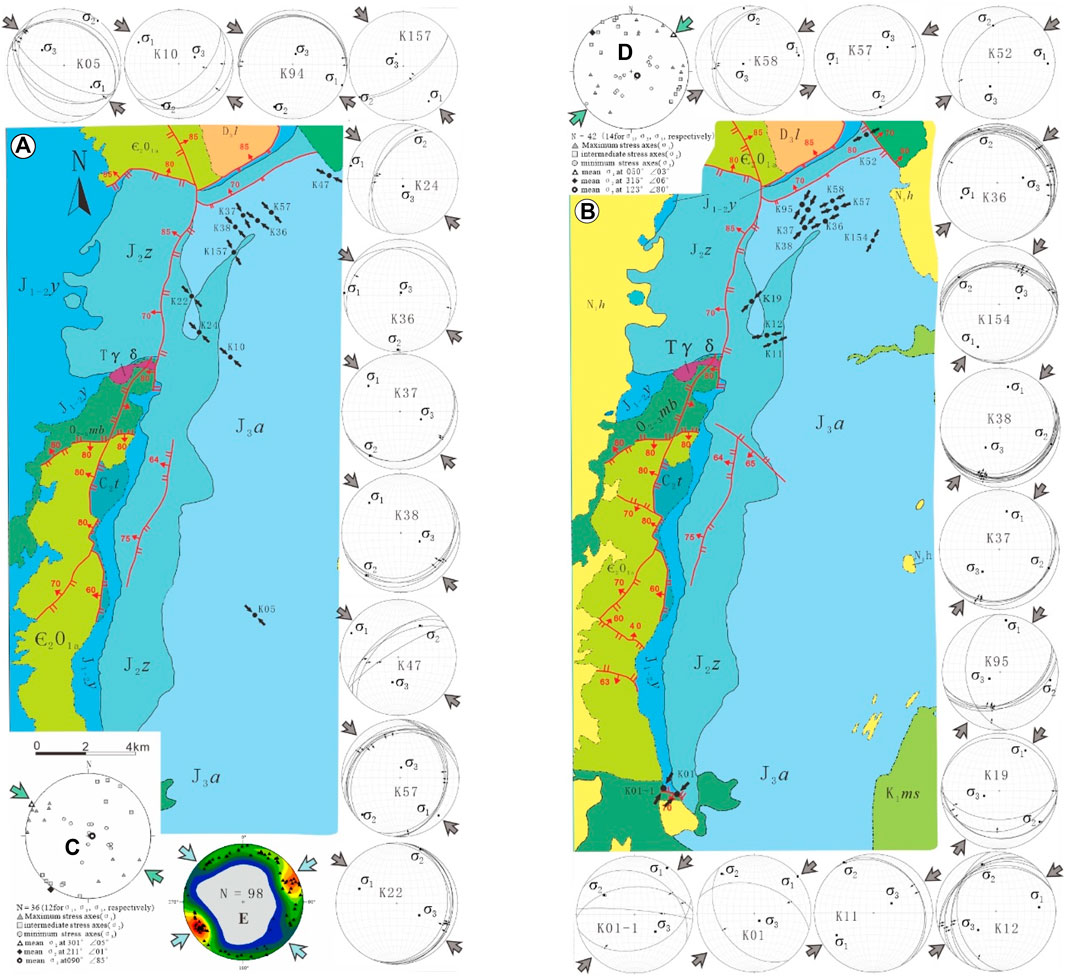
FIGURE 11. The two tectonic stress fields resulted from bedding plane and fault slip vectors observed in the Kexueshan basin. (A) NW-SE compressive stress regime in Kexueshan superposed folds derived from the analysis of the synfolding slip vectors; (B) NE-SW compressive stress regime in Kexueshan superposed folds derived from the analysis of the synfolding slip vectors; (C) the result of the NW-SE compressive structural stress field; (D) the result of the NE-SW compressive structural stress field; (E) Through statistical analysis of all fold hinges in the study area, the stereographic projection results also indicate the existence of the two-phase paleotectonic stress field. Same legends as Figure 2.
Although the early slip vector was destroyed by late tectonic action, it can still be clearly distinguished. According to the crosscutting relationship of the scratch slip vector, the stress field of the NW-SE compression structure in the early stage and the compression structure stress field in the NE-SW direction in the late stage are shown.
Early near-NW-SE compression is found in the Ordovician, Devonian, and Jurassic strata in the study area (Table 1; Figure 10A). The study area has large and wide folds, and due to the uneven local forces on the right limbs of the folds, right limb thrust fault folds developed at Dazhanchang in the Anding Formation (Figure 4B). Under the control of the early NW-SE-trending paleotectonic stress field, various structural styles, such as flexural folds and box folds, formed in the study area (Figure 3). Interlayer cleavage and “X”-shaped conjugate shear joints developed in some areas. The abovementioned various structural styles are the result of the NW-SE compressive structural stress field (Figure 10C). Both the Jurassic Yan’an Formation (J1-2y) and Zhiluo Formation (J2z) reveal compressive tectonic stress during this period (Table 1; Figure 10A). In summary, the above studies indicate that the starting time of the NW-SE compression paleotectonic stress field was at least after the Middle Jurassic or Late Jurassic. At the same time, the analysis of the statistical results for the slip vector between the folds in the field shows that the NE-SW compression tectonic stress field is well reflected in the strata from the Middle Jurassic Zhiluo Formation (J2z) to the end of the Jurassic (Table 1; Figure 10B), forming NW-SE-striking folds, thrust faults, “X”-type conjugate joints, and interlayer cleavage and reforming the early folds to produce cross- or diagonal-span superimposed folds. Most importantly, contemporaneous folds developed in the Hongliugou (N1h) and Anding Formation (J3a) strata (Figure 2), and the pivot direction is oriented NW-SE. Syncline folds are developed in the Hongliugou Formation (N1h) northwest of Guodikeng, with the fold axes in the NW-SE direction (Geological Bureau and Mineral Resources of Ningxia Hui Autonomous Region, 1983, 1990). This is consistent with fold deformation controlled by the NE-SW-trending paleotectonic stress field (Figure 10D) in the study area, indicating that NE-SW-trending tectonic compression occurred after the deposition of the Hongliugou (N1h) strata in the later period. The Liumugao fault has been an active fault controlled mainly by the NE-SW compressional tectonic stress field since the late Cenozoic (Chen et al., 2013; Gong et al., 2016). After analyzing the two-stage sliding vector lineations in the Jurassic Zhiluo Formation (J2z) sedimentary strata (Figure 10) and the structural results for the Dazhanchang superimposed deformation basin (Figure 9), the above two lines of evidence support the interpretation that the NW-SE compressional tectonic deformation event occurred earlier than the NE-SW compressional tectonic deformation event. Through statistical analysis of all fold hinges in the study area, the stereographic projection results also indicate the existence of the two-phase paleotectonic stress field (Figure 10E). Therefore, the above analytical results imply that there have been at least two important tectonic compression and deformation events since the Mesozoic in the KB: the early tectonic stress field was dominated by NW-SE compression (Figure 10C), and the late tectonic stress field was dominated by NE-SW compression (Figure 10D).
Discussion: Tectonic Evolution Process
In summary, the KB on the western margin of the Ordos Block has experienced two main periods of structural shortening events oriented NW-SE and NE-SW. Combining regional structural analysis and deformation-affected stratigraphic characteristics, the two periods of deformation events can be determined to have occurred since the late Mesozoic. This paper proposes a tectonic evolution model of the western margin of the Ordos Block since the late Mesozoic (Figure 12). The specific analysis is as follows:
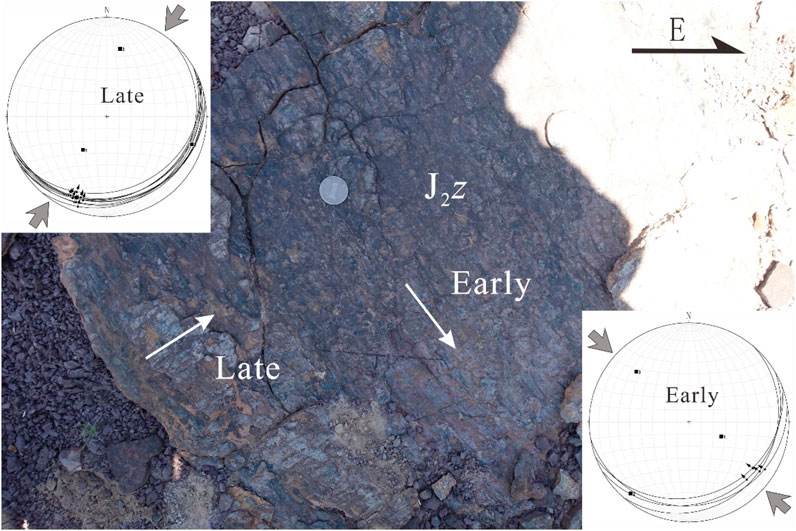
FIGURE 12. Two sets of striations grown in the sandstone of the same Anding formation, early-stage stiations showing NW-SE striking compression and late-stage striations indicating NE-SW striking compression.
The Jurassic strata in the Ordos Basin can be divided into five formations, from lower to upper, the Lower Jurassic Fuxian (∼201–175.6 Ma), the Middle Jurassic Yan’an (∼175.6–168 Ma), Zhiluo and Anding (∼168–161.2 Ma), and the Upper Jurassic Fenfanghe formations (∼161.2–135 Ma), Influenced by the late Triassic tectonic crust shorting events, KB was in a relatively high relief, Fuxian Formation was not deposited (Liu et al., 2006a). Although there existed no sedimentary hiatus between the Zhiluo and Anding formations, U–Pb ages of detrital zircons, lithology, and sedimentary facies show great difference between these two formations (Guo et al., 2017), the sediment colour of the Anding Formation is mainly aubergine and olive and that of the underlying Yan’an and Zhiluo formations is mainly greyish green, the climate of the Anding Formation period was interpreted as cold and dry and that of the Yan’an and Zhiluo formations was warm and humid (Li et al., 2015). All of those indicate that there was a tectonic deformation event in the study area during the Middle Jurassic (Figure 2C).
The above structural analysis shows that the early NW-SE-trending tectonic compression affected all the Mesozoic Jurassic strata, forming a regional NE-SW-trending fold in the KB and the basin edge thrust structure involved in the base (Figure 2; Figure 10A). Existing research data indicate that the western margin of the Ordos Block was compressed and uplifted during the Middle-Late Jurassic. The analysis of regional tectonic geology shows that the Ordos Basin and its periphery were compressed in multiple directions under stress field control during the Middle-Late Jurassic (Zhang Y. Q et al., 2006; Zhang Y et al., 2007). The closure of the Paleo-Tethys Ocean in the southern Ordos Basin resulted in strong intracontinental deformation and a strong northward compressive force. The Mongolia-Okhotsk tectonic belt to the north was in a closure stage, and the Siberian plate was thrust southward, forming a southward compressive force. The eastern circum-Pacific belt, as an active continental margin, formed a strong NW-trending compressive force. Sedimentary analysis shows that the paleocurrent direction on the western margin of the Ordos Basin changed during the Middle Jurassic (J2), indicating a strong intracontinental deformation event (Darby and Ritts, 2002). These studies suggest that the shortening time of the NW-SE-trending structure in the KB should have been in the Middle-Late Jurassic. This period of tectonic shortening and deformation events resulted in a regional angular unconformity contact between the Late Jurassic and Early Cretaceous strata in this area, and thick bottom conglomerates developed (Zhao, 2003; Zhang Y. Q et al., 2006; Zhang Y. Q et al., 2007). The Helan Mountains at the northern margin of the KB experienced superimposed deformation during the Middle-Late Jurassic; that is, the shortening associated with the NW-SE-trending structure was superimposed on the early NW-trending structure (Huang et al., 2015). These studies indicate that the KB also experienced a NW-SE tectonic compression event during this period (Figure 10A). New progress has been made in the chronological data of the growth strata of the Middle Jurassic Changhangou Formation (J2c) in the Daqingshan area on the northern margin of the Ordos Block. The zircon U–Pb ages of the middle-lower volcanic ash interlayers in the growth strata have been obtained, and the structure of this period formed by compression. The onset of compression is limited to the late Middle Jurassic (∼170 Ma) (Wang et al., 2017b). The dynamics of this tectonic shortening event are generally attributed to the control of the Middle-Late Jurassic (∼165 Ma) East Asian multidirectional convergence system (Zhang Y. Q et al., 2006; Dong et al., 2008), which may have been related to the joint effect of southward convergence of the Siberian plate and northwestern subduction of the Pacific plate.
Another strong NE-SW-trending tectonic shortening event is identified in the KB, which led to the widespread development of fold structures and thrust faults in the Jurassic strata, and the main structures are NW-SE-trending folds. This period of deformation strongly reshaped the structure related to the early NW-SE compressive stress. In the area, superimposed structural deformation described as “folding”, “T-shaped”, “crescent” and “dome-shaped” formed, and recumbent folds and overturned folds developed in local areas (Figure 10B). This tectonic shortening event also affected the Paleogene-Neogene strata on the eastern margin of the study area. Previous studies have shown that a set of stable red clastic sediments in the Eocene-Miocene lake environment developed around the Haiyuan fault zone on the western margin of the Ordos Basin. This indicates that the crust was relatively stable during this period, and the northeastward expansion of the Qinghai-Tibet Plateau did not affect the Haiyuan fault zone on the western margin of the Ordos Basin (Shi et al., 2013c). A large amount of research shows that since the late Miocene, strong tectonic uplift has occurred on the western margin of the Ordos Block (Zhao et al., 2006; Wang et al., 2011). Low-temperature thermochronological data also show that a strong NE-SW-trending tectonic shortening event occurred on the western margin of the Ordos Block in the late Miocene (10–8 Ma) (Zhang Y. Q et al., 2006; Bai and Lu, 2009). From the analysis of the Cenozoic structures on the western margin of the Ordos Block, NE-SW tectonic compression can be seen to have controlled the deposition of the Ganhegou Formation, which unconformably overlies the Qingshuiying Formation (Zhang et al., 2005; Shi et al., 2012); as a result, the sedimentary facies of this group change from underlying river-lake facies to fluvial facies (Wang et al., 2011), and regional basin-mountain arc structures formed (Shi et al., 2013a; Shi et al., 2013b; Chen et al., 2015; Shi et al., 2015a). The latest strata involved in the deformation are the late Miocene-Pliocene Ganhegou Formation (Figure 13), and the structural deformation age may be 16.7–5.4 Ma (Wang et al., 2011). Since the end of the Pliocene, against the background of northeastward extrusion of Qinghai-Tibet Plateau material, the Ordos Block has rotated counterclockwise (Zhang et al., 1998). The tectonic stress field in the study area has been mainly transformed to NE compression (Chen et al., 2015). A recent Cenozoic paleomagnetic study on the western margin of the Ordos Block determined the age of the Ganhegou Formation to be 9.5–2.7 Ma (Liu et al., 2019), which indicates that the NE-SW-trending structure shortened from the late Miocene to the end of the Pliocene.
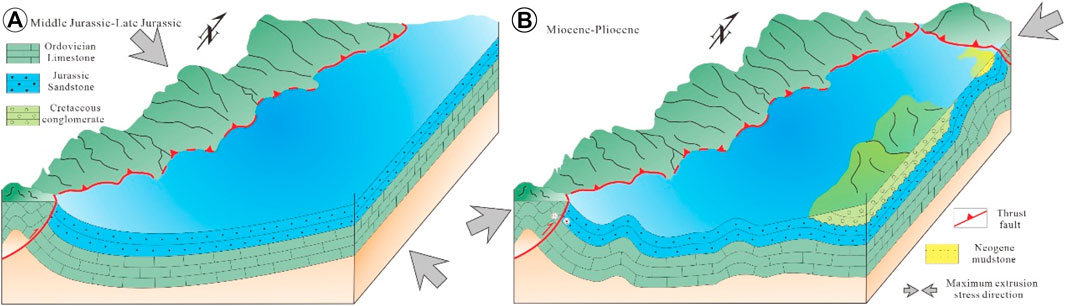
FIGURE 13. Tectonic evolution models of the Kexueshan basin. (A): Mid-late Jurassic NW-SE structure shortening deformation. (B): Short-term deformation of NE-SW to the Late Miocene—late Pliocene.
In summary, the KB on the western margin of the Ordos Block has experienced two main crustal shortening events. The early NW-SE shortening deformation occurred in the Middle-Late Jurassic, and the late NE-SW shortening deformation occurred from the late Miocene to the end of the Pliocene. The abovementioned two tectonic deformation events jointly shaped the superimposed fold structure and geomorphic characteristics of the KB. The late dynamic background should be related to the uplift and northeastward material expansion of the Qinghai-Tibet Plateau since the late Cenozoic (Shi et al., 2015a; Shi et al., 2015a).
Conclusion
In summary, through analyzing the structural deformation of the KB on the western margin of the Ordos Block and combining this information with the regional structural analysis, the following preliminary interpretations have been obtained:
1) Growth strata were discovered in the Jurassic Zhiluo Formation (J2z) and Anding Formation (J3a) in the KB; these growth strata indicate that the initial period of early NW-SE structural shortening occurred in the late Middle Jurassic-Late Jurassic, and the structural shortening and deformation during this period controlled the deposition of the Late Jurassic Anding Formation.
2) Since the late Mesozoic Era, the KB has experienced two main phases of intracontinental shortening and deformation. The early period (J2) was compressed in the NW-SE direction, resulting in strong NE-SW-directed fold shortening; the late period (N12-N2) was controlled by a NE-SW-trending structural compressive stress field, and a series of NW-SE-directed fold structures developed, strongly transforming the early NE-SW structure to form a regional superimposed structure. These results show that the western margin of the Ordos Block is a Meso-Cenozoic intracontinental deformation zone.
3) In terms of the dynamic background of the two phases of crustal shortening and deformation in the KB, the early intracontinental shortening and deformation may have been related to the combined effect of the southward convergence of the Siberian plate and the northwestward subduction of the Pacific plate. The late intracontinental deformation was mainly due to the strong northeastward extrusion of the Qinghai-Tibet Plateau.
Data Availability Statement
The original contributions presented in the study are included in the article/Supplementary material, further inquiries can be directed to the corresponding author.
Author Contributions
YC: Data curation, Methodology, Investigation, Formal analysis, Writing- Original draft preparation, Visualization. RG: Conceptualization, Methodology, Investigation, Writing -Review, Editing, Supervision, Project administration, Funding acquisition. ZL and WL: Supervision, Project administration, Writing- Original draft preparation, Visualization. HS, RH, and HC: Software, Formal analysis, Investigation.
Funding
This study is supported by the Second Tibetan Plateau Scientific Expedition and Research Program (STEP), (Grant No. 2019QZKK0701) and Geological Survey Project of China (DD20190001).
Conflict of Interest
The authors declare that the research was conducted in the absence of any commercial or financial relationships that could be construed as a potential conflict of interest.
Acknowledgments
We thank two reviewers for their constructive comments which helped to improve the manuscript. We greatly thank all the coworkers for their efforts on the fieldwork of the survey project, including Tianyu Wang, Zixian Zhao, and Xiang Qin. We appreciate the sincere discussion and methodological guidance with Prof. Wei Shi.
Publisher’s Note
All claims expressed in this article are solely those of the authors and do not necessarily represent those of their affiliated organizations, or those of the publisher, the editors and the reviewers. Any product that may be evaluated in this article, or claim that may be made by its manufacturer, is not guaranteed or endorsed by the publisher.
References
Angelier, J. (1984). Tectonic Analysis of Fault Slip Data Sets. J. Geophys. Res. 89 (B7), 5835–5848. doi:10.1029/JB089iB07p05835
Bai, S. M., and Lu, C. G. (2009). The Characteristics and Age of South Section of Helanshan Dazhanchang Granodiorite. Ningxia Engingeering Tech. 8 (3), 282–286. (in Chinese).
Carey, E. (1979). Recherche des directions principales de contraintes associées au jeud'une population de failles. Rev. Geogr. Phys. Geol. Dyn., 21.
Chen, F. J. (1986). Types, Tectonic Evolution and Hydrocarbon Occurrence of Petroliferous Basins of China. Earth Sci. 11 (3), 7–16. (in Chinese).
Chen, H., Hu, J., Gong, W., Kang, R., and Li, L. (2015). Characteristics and Transition Mechanism of Late Cenozoic Structural Deformation within the Niushoushan-Luoshan Fault Zone at the Northeastern Margin of the Tibetan Plateau. J. Asian Earth Sci. 114, 73–88. doi:10.1016/j.jseaes.2015.06.034
Chen, H., Hu, J. M., Gong, W. B., and Li, L. b. (2013). Cenozoic Deformation and Evolution of the Niushoushan-Luoshan Fault Zone in the Northeast Margin of the Tibet Plateau. Earth Sci. Front. 20 (4), 18–35. (in Chinese).
Cheng, Y. Z., Shi, W., Zhao, G. C., Wang, T. Y., Yang, Q., Qin, X., et al. (2019). Superimposed Deformation Analysis in Kexueshan Area, the Western Periphery of Ordos Block, China. Environ. Earth Sci. 41 (2), 209–224. (in Chinese).
Darby, B. J., and Ritts, B. D. (2002). Mesozoic Contractional Deformation in the Middle of the Asian Tectonic Collage: The Intraplate Western Ordos Fold-Thrust Belt, China. Earth Planet. Sci. Lett. 205 (1/2), 13–24. doi:10.1016/s0012-821x(02)01026-9
Dong, S. W., Zhang, Y. Q., Long, C. X., Yang, Z. Y., Ji, Q., Wang, T., et al. (2008). Jurassic Tectonic Revolution in China and New Interpretation of the “Yanshan Movement”. Acta Geologica Sinica (English Edition) 81 (11), 1449–1461.
Ford, M., Williams, E. A., Artoni, A., Vergés, J., and Hardy, S. (1997). Progressive evolution of a fault-related fold pair from growth strata geometries, Sant Llorenç de Morunys, SE Pyrenees. Journal of Structural Geology, 1997:413–441.
Gong, W. B., Shi, W., Chen, H., Qiu, S. D., Yin, Y. G., and Zhao, Y. (2016). Quaternary Active Characteristics of the Liumugao Fault in the Northern Segment of the Niushoushan-Luoshan Fault. J. Geomechanics 22 (4), 1004–1014. (in Chinese).
Huang, J. Q. (1983). Problems on the Tectonics of China. Pet. Geology. Exp. 5 (3), 165–169. (in Chinese).
Huang, X., Shi, W., Chen, P., and Li, H. (2015). Superposed Deformation in the Helanshan Structural Belt: Implications for Mesozoic Intracontinental Deformation of the North China Plate. J. Asian Earth Sci. 114, 140–154. doi:10.1016/j.jseaes.2015.05.027
Hui, N. (2008). “Autonomous Region Geological Environment Monitoring Station,” in Geological Map Manual of Yinchuan City (1:250 000) (Yinchuan: Ningxia Hui Autonomous Region Geological Environment Monitoring Station). (in Chinese).
Li, Q. H., Guo, S. N., and Lu, D. H. (1999). Deep Structure and Structure of the Western and Southwestern Margins of Ordos. Beijing: Seismological Press. (in Chinese).
Li, S. G. (1954). Vortex Structure and Other Problems Relating to the Compounding of Geotectonic Systems of Northwestern China. Acta Geologica Sinica 34 (4), 339–410. (in Chinese).
Li, Z. H., Dong, S. W., Feng, S. B., and Qu, H. J. (2015). Sedimentary Response to Middle-Late Jurassic Tectonic Events in the Ordos Basin. Acta Geoscientica Sinica 36, 22–30. (in Chinese).
Liu, C. Y., Zhao, H. G., Gui, X. J., Yue, L. P., Zhao, J. F., and Wang, J. Q. (2006a). Space‐time Coordinate of the Evolution and Reformation and Mineralization Response in Ordos Basin. Acta Geologica Sinica 80, 617–638. (in Chinese).
Liu, C. Y., Zhao, H. G., Gui, X. J., Yue, L. P., Zhao, J. F., and Wang, J. Q. (2006b). Space-time Coordinate of the Evolution and Reformation and Mineralization Response in Ordos Basin. Acta Geologica Sinica 80 (5), 617–638. (in Chinese).
Liu, C. Y., Zhao, H. G., Wang, F., and Chen, H. (2005). Attributes of the Mesozoic Structure on the West Margin of the Ordos Basin. Acta Geologica Sinica 79 (6), 737–747. (in Chinese).
Liu, H. F., Wang, Z. C., Xiong, B. X., Li, Y. L., Liu, L. Q., and Zhang, J. Z. (2000). Coupling Analysis of Mesozoic-Cenozoic Foreland Basin and Mountain System in Central and Western China. Earth Sci. Front. 7 (3), 55–72. (In Chinese).
Liu, S. F., Ke, A. R., Wu, L. Y., and Huang, S. J. (1997). Sediment Provenance Analysis and its Tectonic Significance in the Foreland Basin of the Ordos Southwestern Margin. Acta Sedimentologica Sinica 15 (1), 156–160. (in Chinese).
Liu, S. F. (1994). Mesozoic Tectonic Stratigraphic Analysis and Dynamic Mechanism of the Western Margin of the Ordos Basin. Wuhan: China University of Geosciences. (in Chinese).
Liu, S. F., and Yang, S. G. (1996). Lateral Extrusion Tectonics with Restricted Boundaries on the Western Margin of the Ordos Basin. Reg. Geology. China, 353–360. (in Chinese).
Liu, S. F., and Yang, S. G. (1997). The Differences between the Southwestern and the Northwestern Ordos Basin and Their Forming Mechanism. Chin. J. Geology. 32 (3), 397–408. (in Chinese).
Liu, S., Lin, C., Liu, X., and Zhuang, Q. (2018). Syn-tectonic Sedimentation and its Linkage to Fold-Thrusting in the Region of Zhangjiakou, North Hebei, China. Sci. China Earth Sci. 61 (6), 681–710. doi:10.1007/s11430-017-9175-3
Liu, S., Su, S., and Zhang, G. (2013). Early Mesozoic Basin Development in North China: Indications of Cratonic Deformation. J. Asian Earth Sci. 62, 221–236. doi:10.1016/j.jseaes.2012.09.011
Liu, X., Shi, W., Hu, J., Fu, J., Yan, J., and Sun, L. (2019). Magnetostratigraphy and Tectonic Implications of Paleogene-Neogene Sediments in the Yinchuan Basin, Western North China Craton. J. Asian Earth Sci. 173 (APR.15), 61–69. doi:10.1016/j.jseaes.2019.01.016
Ma, X. Y., You, Z. D., Tan, Y. J., Yang, W. R., Li, D. X., and Wu, Z. W. (1961). Several Basic Problems on the Tectonics of China. Acta Geologica Sinica 41 (1), 30–44. (in Chinese).
Mercier, J. L., Carey, E., Sebrier, M., and Stein, S. (1991). Palaeostress Determinations from Fault Kinematics: Application to the Neotectonics of the Himalayas–Tibet and the Central Andes. Philos. Trans. R. Soc. Lond. 337, 41–52.
Ningxia Hui Autonomous Region Geological Survey Institute (2017). Regional Geology of China: Ningxia[M]. Beijing: Geological Publishing House. (in Chinese).
Ramsay, J. G. (1987). The Techniques of Modern Structural Geology: Ⅱ. Folds and Fractures. London: Academic Press.
Shi, W., Chen, H., Li, Z. H., and Qiu, S. D. (2016). Regional Geologic Investigation Methods in Neotectonic and Active Tectonic Area. J. Geomechanics 22 (4), 856–867. (in Chinese).
Shi, W., Dong, S.-W., Ratschbacher, L., Tian, M., Li, J.-H., and Wu, G.-L. (2013a). Meso-Cenozoic Tectonic Evolution of the Dangyang Basin, North-central Yangtze Craton, Central China. Int. Geology. Rev. 55 (3), 382–396. doi:10.1080/00206814.2012.715732
Shi, W., Dong, S., Liu, Y., Hu, J., Chen, X., and Chen, P. (2015a). Cenozoic Tectonic Evolution of the South Ningxia Region, Northeastern Tibetan Plateau Inferred from New Structural Investigations and Fault Kinematic Analyses. Tectonophysics 649, 139–164. doi:10.1016/j.tecto.2015.02.024
Shi, W., Dong, S. W., Hu, J. M., Zhang, Z. Y., and Liu, G. (2007). An Analysis of Superposed Deformation and Tectonic Stress Fields of the Western Segment of Daba Mountains Foreland. Acta Geologica Sinica 81 (10), 1314–1327. (in Chinese).
Shi, W., Dong, S. W., Huang, X. F., Chen, H., and Cui, J. J. (2017). Jurassic Deformation at the Western Margin of the East Asia Continent: A Case Study of Ductile Deformation in the Central Segment of the Bangong Lake-Nujiang Belt. J. Geomechanics 23 (4), 515–525. (in Chinese).
Shi, W., Dong, S. W., Li, J. H., Tian, M., and Wu, G. L. (2013c). Formation of the Moping Dome in the Xuefengshan Orocline, Central China and its Tectonic Significance. Acta Geologica Sinica(English Edition) 87 (3), 720–729.
Shi, W., Dong, S., Zhang, Y., and Huang, S. (2015b). The Typical Large-Scale Superposed Folds in the Central South China: Implications for Mesozoic Intracontinental Deformation of the South China Block. Tectonophysics 664, 50–66. doi:10.1016/j.tecto.2015.08.039
Shi, W., Liu, Y., Liu, Y., Chen, P., Chen, L., Cen, M., et al. (2013b). Cenozoic Evolution of the Haiyuan Fault Zone in the Northeast Margin of the Tibetan Plateau. Earth Sci. Front. 20 (4), 1–17. (in Chinese).
Shi, W., Zhang, Y., Dong, S., Hu, J., Wiesinger, M., Ratschbacher, L., et al. (2012). Intra-continental Dabashan Orocline, Southwestern Qinling, Central China. J. Asian Earth Sci. 46, 20–38. doi:10.1016/j.jseaes.2011.10.005
Shi, W., Zhang, Y. Q., and Ma, Y. S. (2006). Elevation Distribution Pattern of Red Clay Beds in the Regions East and West of Liupanshan and Neotectonic Significance. Mar. Geology. Quat. Geo-logy 26 (5), 123–130. (in Chinese).
Song, H. L., Zhang, C. H., and Wang, G. H. (2013). Structural Geology. Beijing: Geological Publishing House. (in Chinese).
Sperner, B., and Zweigel, P. (20102010). A Plea for More Caution in Fault-Slip Analysis. Tectonophysics 482, 29–41. doi:10.1016/j.tecto.2009.07.019
Tang, X. Y., and Wang, D. Yi. (1988). The Characteristics and Evolution of the Thrust Nappe Tectonic Belt and its Petroleum Potential in the West Ordos Basin. Oil Gas Geology. 9 (1), 1–10. (in Chinese).
Wang, F., Liu, C. Y., Zhao, H. G., Yang, X. K., and Su, C. Q. (2006). Relationship between Helanshan Basin and Ordos Basin. Acta Petrolei Sinica 27 (4), 15–17. (in Chinese).
Wang, W. T., Zhang, P. Z., Kirby, E., Wang, L. H., Zhang, G. L., Zheng, D. W., et al. (2011). A Revised Chronology for Tertiary Sedimentation in the Sikouzi Basin: Implications for the Tectonic Evolution of the Northeastern Corner of the Tibetan Plateau. Tectonophysics 505 (1/2/3/4), 100–114. doi:10.1016/j.tecto.2011.04.006
Wang, Y. C., Dong, S. W., Chen, X. H., and Shi, W. (2017a). New Time Constraint on the Initiation of Yanshan Movement from Jurassic Growth Strata in the Daqingshan Area, Inner Mongolia. Chin. Sci. Bull. 62 (12), 1274–1277. (in Chinese).
Wang, Y. C., Dong, S. W., Shi, W., Chen, X. H., and Jia, L. M. (2017b). The Jurassic Structural Evolution of the Western Daqingshan Area, Eastern Yinshan Belt, North China. Int. Geology. Rev. 59 (15), 1–23. doi:10.1080/00206814.2017.1300784
Yang, Z. Y., Yuan, W., Tong, Y. B., Liu, J., and Wang, Y. (2014). Tectonic Affinity Reconnaissance of the Alxa Block in the Pre-mesozoic. Acta Geoscientica Sinica 35 (6), 673–681. (in Chinese).
Yuan, W., and Yang, Z. (2015). The Alashan Terrane Was Not Part of North China by the Late Devonian: Evidence from Detrital Zircon U-Pb Geochronology and Hf Isotopes. Gondwana Res. 27 (3), 1270–1282. doi:10.1016/j.gr.2013.12.009
Zhang, J., Ma, Z. J., and Ren, W. J. (2005). The Sedimentary Characteristics of Cenozoic Strata in Central and Southern Ningxia and Their Relationships with the Development of the Qinghai-Tibetan Plateau. Acta Geologica Sinica 79 (6), 757–773. (in Chinese).
Zhang, J. S., He, Z. X., Fei, A. Q., Li, T. B., and Huang, X. N. (2008). Epicontinental Mega Thrust and Nappe System at North Segment of the Western Rim of the Ordos Block. Chin. J. Geology. 43 (2), 251–281. (in Chinese).
Zhang, K. (1983). “Discussion on Helan-Graben∥Inner Mongolia Petroleum Society,” in Proceedings of Petroleum Geology in the Western Margin of the Ordos Basin (Hohhot: Inner Mongolia People’s Publishing House), 29–40. (in Chinese).
Zhang, K. (1989). Ordos Fault Block Structure and Resources. Xi’an: Shaanxi Science and Technology Press. (in Chinese).
Zhang, P. Z., Zheng, D. W., Yin, G. M., Yuan, D. Y., Zhang, G. L., Li, C. Y., et al. (2006). Discussion on Late Cenozoic Growth and Rise of Northeastern Margin of the Tibetan Plateau. Quat. Sci. 26 (1), 5–13. (in Chinese).
Zhang, Y., Liao, C., Shi, W., Zhang, T., and Guo, F. (2007). Jurassic Deformation in and Around the Ordos Basin, North China. Earth Sci. Front. 14 (2), 182–196. (in Chinese). doi:10.1016/s1872-5791(07)60016-5
Zhang, Y. Q., Dong, S. W., and Shi, W. (2003a). Cretaceous Deformation History of the Tan-Lu Fault Zone in Shandong Province, Eastern China. J. Geodynamics 363 (3), 243–258. doi:10.1016/s0040-1951(03)00039-8
Zhang, Y. Q., Dong, S. W., Zhao, Y., and Zhang, T. (2007). Jurassic Tectonics of North China: A Synthetic View. Acta Geologica Sinica 81 (11), 1462–1480. (in Chinese).
Zhang, Y. Q., Ma, Y. S., Yang, N., Shi, W., and Dong, S. W. (2003b). Cenozoic Extensional Stress Evolution in North China. J. Geodynamics 36 (5), 591–613.
Zhang, Y. Q., Mercier, J. L., and Vergély, P. (1998). Extension in the Rift Systems Around the Ordos (China), and its Contribution to the Extrusion Tectonics of South China with Respect to Gobi-Mongolia. Tectonophysics 285 (1), 41–75. doi:10.1016/s0040-1951(97)00170-4
Zhang, Y. Q., Shi, W., Liao, C. Z., and Hu, B. (2006). Fault Kinematic Analysis and Change in Late Mesozoic Tectonic Stress Regimes in the Peripheral Zones of the Ordos Basin, North China. Acta Geologica Sinica 80 (5), 639–647. (in Chinese).
Zhao, H. G. (2003). Structural Characteristics and the Evolution in Western Ordos Basin. Xi’an: Northwest University. (in Chinese).
Keywords: ordos block, kexueshan basin, superimposed deformation, fault kinematics, tectonic stress field
Citation: Cheng Y, Gao R, Lu Z, Li W, Su H, Han R and Chen H (2022) Meso-Cenozoic Tectonic Evolution of the Kexueshan Basin, Northwestern Ordos, China: Evidence from Palaeo-Tectonic Stress Fields Analyses. Front. Earth Sci. 10:845475. doi: 10.3389/feart.2022.845475
Received: 29 December 2021; Accepted: 31 January 2022;
Published: 07 March 2022.
Edited by:
Hanlin Chen, Zhejiang University, ChinaCopyright © 2022 Cheng, Gao, Lu, Li, Su, Han and Chen. This is an open-access article distributed under the terms of the Creative Commons Attribution License (CC BY). The use, distribution or reproduction in other forums is permitted, provided the original author(s) and the copyright owner(s) are credited and that the original publication in this journal is cited, in accordance with accepted academic practice. No use, distribution or reproduction is permitted which does not comply with these terms.
*Correspondence: Rui Gao, gaorui66@mail.sysu.edu.cn