- Petrochina Northwest Research Institute of Petroleum Exploration and Development, Lanzhou, China
The branched alkanes with quaternary carbon atoms (BAQCs) and monomethylakanes (MMAs), cycloalkanes, monoethylalkanes (MEAs) have been detected by GC-MS in the saturated hydrocarbons fractions of Carboniferous black shale from the Wuwei Basin in northwest China. Nine series of BAQCs (series A-I) are present in Carboniferous black shale, which are concomitant with two series of long-chain cycloalkanes, two series of MMAs and one series of MEAs. All of these series are expressed as exclusively odd or exclusively even carbon numbers with consistent changes of abundance in the samples, meanwhile, based on the analysis of basic geochemical characteristics and other biomarkers of the samples, we presume that it possessed a similar parent materials or evolutionary process and weak oxidation-reduction sedimentation environments. In addition, the results show that the abundance of the long-chain branched alkanes is highly correlated with the odd-even predominances (OEP (15–19)) of the short-chain n-alkane. The lower the OEP (15–19) values of the short-chain n-alkane are (more significant the even-carbon dominance), the higher the abundance of the long-chain branched alkanes. The significant even-carbon dominance of the short-chain n-alkanes indicates the strong input of archaea or algal organic matter. The relatively wide occurrence and high abundance of these alkanes suggests a relationship with specific paleoenvironment and paleoclimate conditions.
Introduction
Since their recognition, long-chain branched alkanes with quaternary carbons (BAQCs) have been considered a new and independent biomarker (Kenig et al., 2003; Bai et al., 2006). The detection of BAQCs has been reported in sediments from the Paleoproterozoic Era (2.2Ga) to the present (Dill et al., 1988; Shiea et al., 1990; Flaviano et al., 1994; Kenig et al., 1995; Derenne et al., 1996; Mycke et al., 1998; Aroui et al., 2000; Logan et al., 2001; Kenig et al., 2002; Kenig et al., 2003; Bai et al., 2006; Zhang et al., 2007; Fang et al., 2010; Zhang et al., 2014). BAQCs are compounds with many pseudohomologous series. In addition to the most commonly reported 5, 5-diethylalkane (Series A), the BAQCs mainly include 6,6-diethylalkane (Series B), 5-butyl-5-ethylalkane (Series C), 6-butyl-6-ethylalkane (Series D), etc. Kenig et al. (2005) detected nine pseudohomologous series of BAQCs in the Mesozoic black shale of Canada, which showed exclusively odd-carbon or exclusively even-carbon distribution patterns, and the author concluded that they had distinct and special biogenic sources.
Kenig et al. (2003) proposed that BAQCs only existed in special environments and that their appearance indicates a weak oxidizing sedimentary environment that can be used to reconstruct the transitional paleoenvironment (Menor-Salván et al., 2010). Other studies have shown that the location of the peak carbon of Series A reflects regular spatial changes, which may indicate different climates. In hot and humid climates, the main peak of Series A is dominated by C21 or C23, whereas it is dominated by C27 in cold and arid climates (Bai et al., 2006; Zhang et al., 2007). However, this application still requires numerous studies on the relationship between the characteristics of BAQCs and the sedimentary environment in different areas, particularly quantitative studies. Unfortunately, due to the low content of BAQCs, there is still a lack of sufficient supporting data.
Nine series of branched alkanes with quaternary carbons are present in Carboniferous black shale samples from the Wuwei Basin of China, which are concomitant with two series of long-chain cycloalkanes and other long-chain branched alkanes. We quantitatively analyzed the characteristics of the structure, abundance and distribution of each series of compounds, determined the distribution of the long-chain branched alkanes and further discuss their sources and environmental significance to provide a reference for future applications of BAQCs.
Study Site
The Wuwei Basin is located in western China (Figure 1A). Because it is located far from the Qilian orogenic belt, it was weakly affected by the Xishan tectonic movement. Thus, this basin is one of the areas in the Qilian-Alashan region with well-developed and preserved Carboniferous strata (Li et al., 2010). Complete sequences of the Carboniferous strata are widely exposed on the periphery of the basin. Emergence stratums are of the Qianheishan formation, Chouniugou formation, Jingyuan formation, Yanghugou formation and Taiyuan formation (Figure 1B). The sedimentary strata in this area mainly consist of continental strata and marine strata. During the late Carboniferous period, the climate of the basin was warm and humid with biological prosperity and with a sedimentary environment of desalting lagoon, littoral marsh, delta, littoral and neritic deposit. The dark mudstone and shale of the Yanghugou Group that were deposited during this period are the main source rocks of the basin (Yan and Yuan 2011; Zhao et al., 2021).
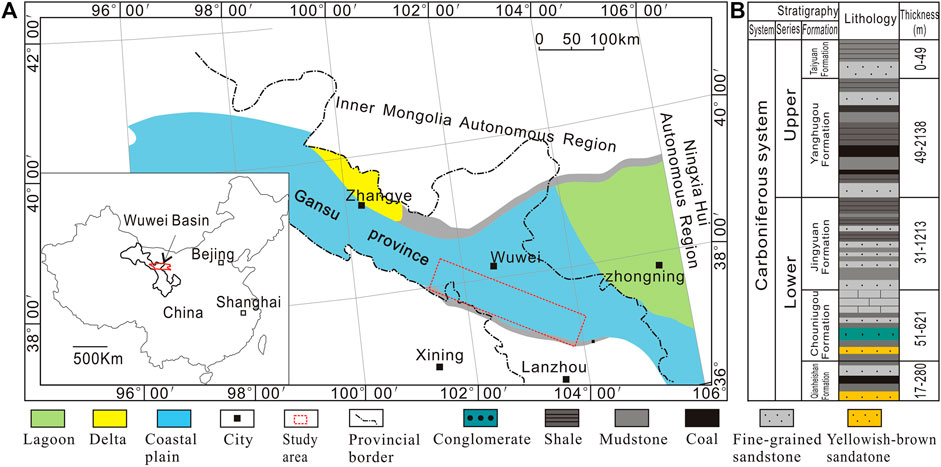
FIGURE 1. (A) Geological sketch map of the Wuwei Basin and the location of studied section; (B) Stratigraphy of the studied area.
Materials and Method
The 40 black shale samples in this study were all collected from 12 freshly exposed profiles of the Carboniferous Yanghugou formation in the Wuwei Basin (Table 1). An ELTRA CSI analyser and a Rock-Eval 6 pyrolysis analyser were employed to measure the TOC, S1, S2, and Tmax values. The total organic carbon (TOC) of the samples is between 1.9 and 42.8% with an average of 9.1%. The vitrinite reflectance (Ro) measured by microscope is between 1.5 and 2.6% with an average of 1.93%, which indicates a high abundance of organic matter and a high maturity. The δ13C (VPDB) of kerogen measured by stable isotope ratio mass spectrometer (MAT 253, Thermo Fisher Scientific) equiped with element analyzer is between -25.1‰ and -21.0‰ with an average of -23.5‰, which reflects the samples with humic type organic matter.
Experiment and Analysis
The black shale samples were rinsed with distilled water, dried naturally and ground to 100-mesh. Then the ground samples were extracted by Soxhlet extraction for 72 h with chloroform solvents. The extracts were filtered, evaporated to dryness and were subjected to silica gel column chromatography eluted with n-hexane, dichloromethane and methanol to yield saturated hydrocarbons, aromatic hydrocarbons and non-hydrocarbons, respectively. The saturated hydrocarbons were directly analyzed by gas chromatography-mass spectrometry (GC-MS) to obtain their detailed composition using 5973N mass spectrometer was equipped with 6890N gas chromatography produced by Agilent Technologies of the United States. Its chromatographic column was a HP-5 fused quartz capillary column (30 m × 0.25 mm × 0.25 μm), and the carrier gas was helium. Its starting temperature was 80°C, and the heating rate was 4 C/min. After being heated to 300°C, the temperature was held constant for 30 min. The temperature of the ion source was 250°C, and the ionization voltage was 70 eV.
The trace elements of samples were measured by a Nu Attom Laser Ablation Inductively Coupled Plasma Mass Spectrometer (LA-ICP-MS) produced by the Nu Instruments Company of the United Kingdom. The samples were pretreated using the acid-soluble method. The samples were first ground to 200 mesh and dried at 105°C for approximately 3 h to remove the water. Then, 50 g of the rock samples was placed into a polytetrafluoroethylene-loaded liner and dissolved by dropping HNO3, HF and HClO4. Finally, the mixture was added to an Rh internal standard solution and diluted with deionized water until the Rh concentration was 10 ng/ml before testing.
Results and Discussion
Structure Identification of the Long-Chain Branched/Cyclic Alkanes
Based on the comparison of the GC-MS results of long-chain branched/cyclic alkanes reported by previous studies (Kenig et al., 2003; Greenwood et al., 2004; Zhang et al., 2014), we initially identified nine series of BAQCs that are present in the Carboniferous black shale samples from the Wuwei Basin of China, which are concomitant with two series of long-chain cycloalkanes, two series of monomethylalkanes and one series of monoethylalkanes (Figure 2). The BAQCs are mainly Series A, Series B, Series C, Series D, 3,3-diethylalkane, 5-ethyl-5-methylalkane, 3-ethyl-3-methylalkane, 7,7-diethylalkane and 2,2-dimethylalkane. The cyclic branched alkanes are mainly long-chain cyclohexane and cyclopentane as well as long-chain 3-methylalkane, 7-methylalkane and 5-ethylalkane. These series of BAQCs in this study are not completely consistent with Kenig et al. (2005), who detected nine series of BAQCs in the Mesozoic black shale of Canada, which indicates the series diversity of BAQCs.
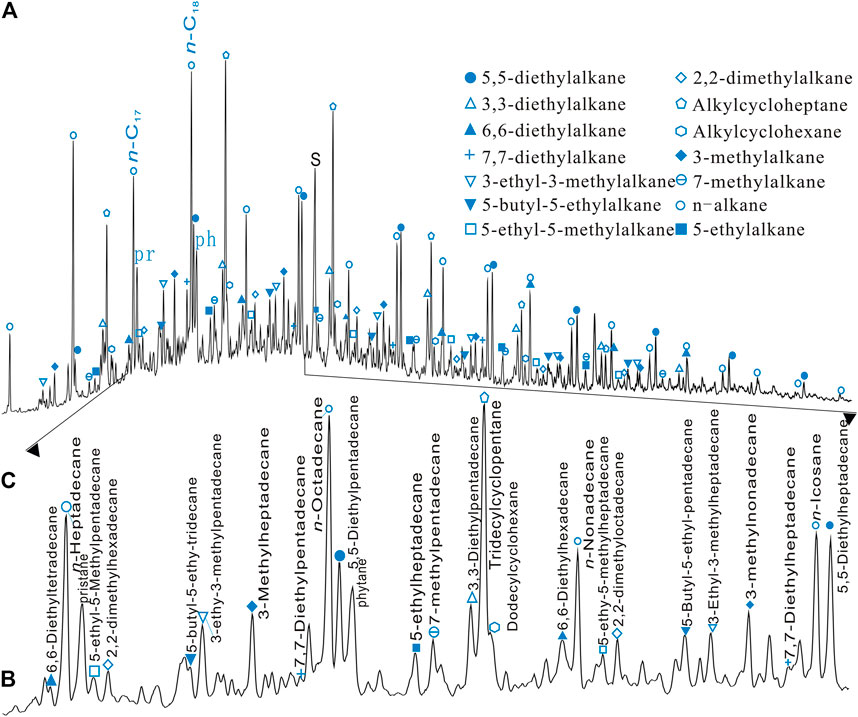
FIGURE 2. (A) Total ion curret (TIC) of the long-chain branched and cyclic hydrocarbon fraction of P18-4 sample; (B) Detail of A; (C) Detail of B with full name identification.
BAQCs are prone to break at the quaternary carbon atom to form a characteristic ion peak in the mass spectrum. In addition, the substituents contained in the quaternary carbon atoms break easily. The lost fragments of molecular ions can be used to determine the existing substituents. The characteristic ions and ion series generated after the breakage can be used to determine the compound types. For example, C21-5, 5-diethylalkane has a molecular weight of 296, and it can form a characteristic peak with an m/z 127 after losing the maximum 5,5-diethyl quaternary carbon substituent. In addition, due to the rearrangement of H atom in the cationic fragment with a quaternary carbon, it can form an ion peak with an m/z value of 126 after losing 1H. The relatively strong [M-29]+ 267) and weak [M-57]+ 239) ion fragments are equivalent to the breakage of two ethyls and one butyl. Similarly, the characteristic peaks of 6,6-diethylalkane, 5-butyl-5-ethylalkane, 6-butyl-6-ethylalkane, 3,3-diethylalkane, 5-ethyl-5-methylalkane, 3-ethyl-3-methylalkane, 7,7-diethylalkane and 2,2-dimethylalkane are m/z141, m/z155, m/z169, m/z (99, 98), m/z113, m/z85, m/z155, and m/z57, respectively, whereas the base peaks of the long-chain cyclohexane and cyclopentane are m/z83 and m/z69, respectively.
Previous studies have used the relative response factor (RRF) to quantify the difference between branched alkanes and n-alkane with the same carbon numbers after chromatographic flushing (Kissin et al., 1986). The RRF is an effective indicator for compound identification, especially for pseudohomologous families. The RRF values of the long-chain branched/cyclic alkanes calculated in this study are given in Table 2 and Figure 3. The RRF values of Series A-D calculated by Greenwood et al. (2004) are consistent with the results of this study, which further demonstrates the reliability of the compound identification in this study.
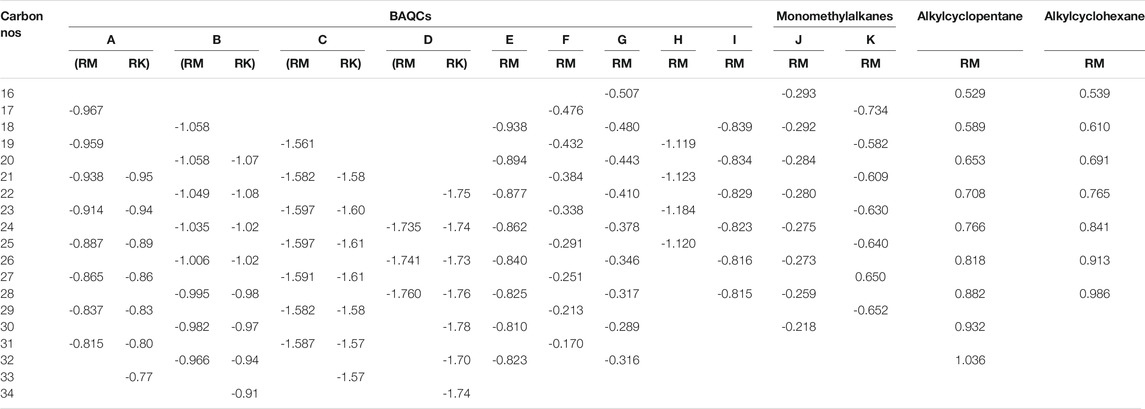
TABLE 2. Relative retention factors (RRF) for long-chain branched/cyclic alkanes measured with a HP-5MS Capillary column.
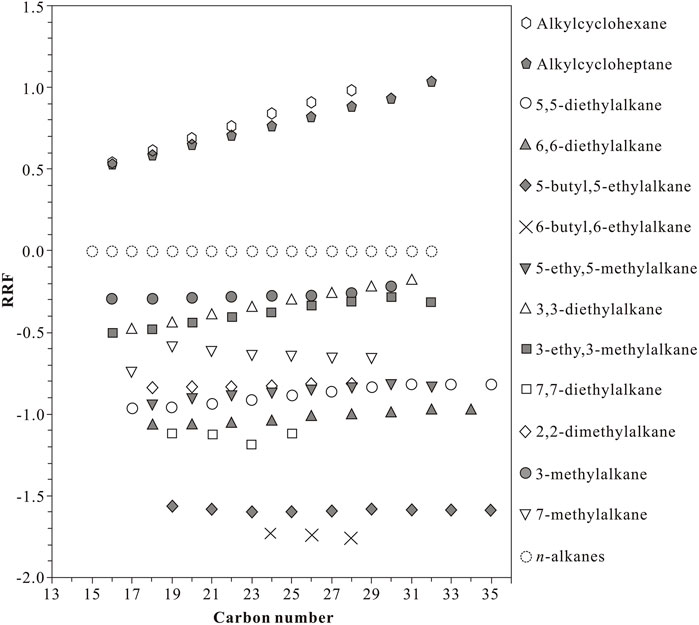
FIGURE 3. The correlations between Relative retention factors (RRF) of long-chain branched/cyclic alkanes and number of carbon.
The RRF is calculated as follows:
where n represent the carbon number; RTXn represent the response time of the branched alkane with n carbons; RTCn express the response time of the n-alkane with n carbons; and RTCn-1 express the response time of the n-alkane with n-1 carbons.
The results of this study show that the RRF values of the long-chain branched alkanes are all negative with values between -1.76 and -0.17, whereas the RRF values of the long-chain cycloalkanes are between 0.54 and 1.04. These results indicate that the peaks of the long-chain branched alkanes occur earlier than those of the n-alkanes with the same carbon number, whereas the peaks of the long-chain cycloalkanes occur later. Further analysis shows that the RRF values of BAQCs with different structures have the following characteristics: ①In branched alkanes of the same series, the RRF increases with the carbon number of the main chain. For example, the RRF of C31-5,5-diethylalkane is -0.82, whereas the RRF of C17-5,5-diethylalkane is -0.97. ②In BAQCs with the same branch, the RRF is more negative with a higher location of the branched substituents. For example, the RRF values of 7,7-diethylalkane, 6,6-diethylalkane, 5,5-diethylalkane and 3,3-diethylalkane are -1.38 to -1.12, -1.06 to -0.97, -0.97 to -0.82 and -0.48 to -0.17, respectively. ③When the locations of the substituents are the same, the RRF is more negative with longer branches. For example, the RRF of 6-butyl-6-ethylalkanes is -1.76 to -1.74, whereas the RRF of 6,6-diethylalkane is -1.06 to -0.97. In summary, the RRF is more negative in branched alkanes with lower carbon numbers, longer branches and higher locations of the substituent. This may be because these compounds have a smaller structural volume, so the response time in the chromatographic column is reduced, and they can be eluted earlier. The determination of the distribution of the RRF values of this series of compounds will provide a useful scientific basis for the identification of these compounds in the future.
Distribution Characteristics of Long-Chain Branched/Cyclic Alkanes
Characteristics of the Carbon Numbers
The carbon number of the main peak and the length of the carbon chain are widely recognized as effective indicators of organic matter’s origin, evolution and paleoenvironment. However, whether this method can be used to study branched alkanes still requires investigation. Each series of long-chain alkane compounds is expressed as an exclusively odd-carbon or even-carbon distribution pattern (Table 3) with a unimodal peak. However, the distribution of the carbon numbers of each series of compounds is different. For example, the carbon number distribution of Series A is the widest with a range of C17-C35 and some samples range from C17 to C37 compounds, whereas that of long-chain cyclohexane is the narrowest with a range of C16-C28. Different distributions of carbon numbers may indicate that the formation pathways of different series of compoundsare different.
The main peak carbon numbers of each series of long-chain branched/cyclic alkanes are mainly distributed between C18 and C20 (Table 3), and they are concentrated. The main peaks of the even-carbon compounds are mainly C18, whereas those of the odd-carbon compounds are mainly C21. Both are dominated by medium carbon numbers. Bai et al. (2006) investigated modern soils and found that the main peak carbon number distribution of Series A may sensitively indicate changes in the soil ecology and climate. In hot and humid climates, the main peaks of Series A are generally dominated by C21 or C23, whereas they are dominated by the high-carbon-number C27 in cold and arid climates. The main peaks of Series A in the study area are C21, which indicates a hot and humid paleoclimatic condition during sample deposition (Kenig et al., 2002; Kenig et al., 2003; Kenig et al., 2005; Zhang et al., 2007). This is consistent with the warm climate during the Carboniferous deposition (Yan and Yuan 2011), which further confirms that this indicator can be used to infer the climate during the depositional period.
Characteristics of the Relative Abundance
In the case of absolute quantification without the use of internal standard before extraction, relative abundance is often used to reflect the content differences between different compounds. According to the relative abundance calculation method proposed by Zhang et al. (2007), the author estimated the relative abundance by the ratios of n-alkanes. In all the samples analyzed in this study, series A is of the highest abundance and the position of the peak is located just behind the even n-alkanes, where one carbon atom is missing. Meanwhile, the main peak carbon numbers of each series of long-chain branched/cyclic alkanes are mainly nC18. Based on above assumption, we use the ratio between the main peak height of each series and n-octodecane to discuss their relative abundance. The results show that the contents of different series of compounds in the same sample vary significantly. For example, in sample P18-4 (Table 3), the relative abundance of Series A is 0.57, followed by 3,3-diethylalkane with a relative abundance of 0.37. The relative abundance is lowest for Series D (0.04). In addition, the other concomitant long-chain alkanes have high relative abundances, which are up to 1.08 for long-chain cyclopentane and much higher than that of the BAQCs. The relative abundance of long-chain 3-methylalkane is also high (0.57), which is comparable with that of the BAQCs.
The relative abundances of the long-chain branched alkanes in the 40 samples vary significantly. For example, the relative abundances of Series A in the samples are between 0.06 and 0.73, whereas the further evidence shows that the changes in the abundance of all series of compounds are consistent. As shown in Figure 4, the relative abundances of 3,3-diethylalkane, 3-ethyl-3-methylalkane and 3-methylalkaneall increase with an increase in Series A, which indicates simultaneous changes. Zhang et al. (2014) detected eight series of BAQCs, long-chain cyclopentane and cyclohexane in modern lake sediment profiles and found that the changes in the abundance of all series of compounds in the sedimentary profiles were consistent. Our results are consistent with those of Zhang et al. (2014). These simultaneous changes indicate that the long-chain branched/cyclic alkanes of all of the series may have originated from the same matter or depict homologous characteristics, which suggests that BAQCs may not exist independently and should be considered as a whole with long-chain cycloalkanes and other long-chain branched alkanes in future research.
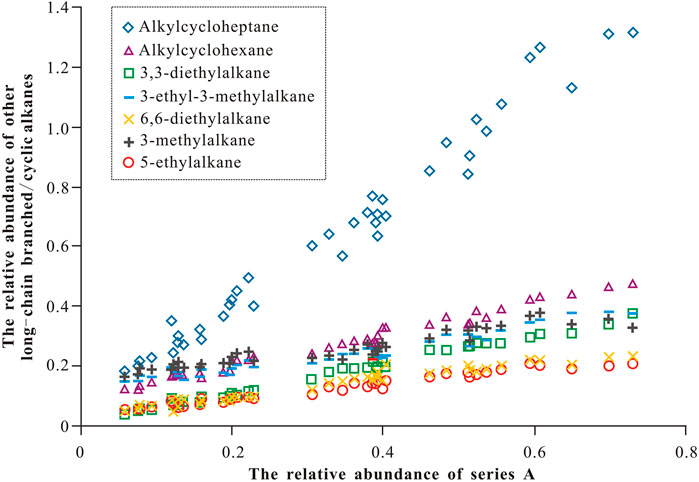
FIGURE 4. Relative abundances variation correlations between series A and other long-chain branched/cyclic alkanes (with 10 samples).
Correlation Between Long-Chain Branched Alkanes and n-alkanes
Short-chain n-alkanes (∑C21-) are mainly from bacteria and algae, whereas long-chain n-alkanes (∑C22+) are mainly from higher plants (Meyers and Ishiwatari 1993). Scholars commonly use ∑C21-/∑C22+ to identify the sources of organic matter. The ∑C21-/∑C22 + values of the samples in this study are between 0.66 and 5.06 with an average of 1.57, which indicates that the organic matter in the samples is mainly bacteria and algae. Zhang et al. (2014) found that the most common Series A of long-chain branched alkanes and short-chain odd-carbon n-alkanes in organic matter in modern lake sediment are highly correlated and suggested that long-chain branched alkanes were mainly from aquatic heterotrophic bacteria. However, the results of this study show a poor correlation between Series A and C21-/∑C22+ for forty samples (Figure 5), which indicates that the parental sources of the long-chain branched alkanes and n-alkanes are different. In addition, the δ13C values of Series A measured by Greenwood et al. (2004) are 5–8‰ lighter than those of the n-alkanes with the corresponding carbon number, which further demonstrates that the long-chain branched alkanes are from independent sources.
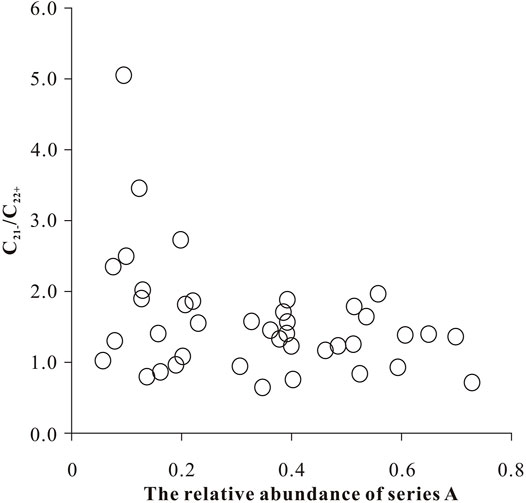
FIGURE 5. The correlations between the relative abundance of Series A and C21-/C22+ of n-alkanes (with 40 samples).
The short-chain n-alkanes in the samples from the study area show significant even-carbon dominance (low OEP15-19 values with a range of 0.47–1.16, and the OEP values of 75% of the samples are less than 1), which indicates the strong transformation of bacteria to organic matter (Elias et al., 1997; Zhang et al., 2014). The relative abundance of Series A in the samples and the OEP values show a significant negative correlation (Figure 6). The more significant the even-carbon dominance (the smaller the OEP value) is, the higher the abundance of Series A. Based on these results, we suggest that the sources of the long-chain branched alkanes may be related to bacteria that can transform algae organisms.
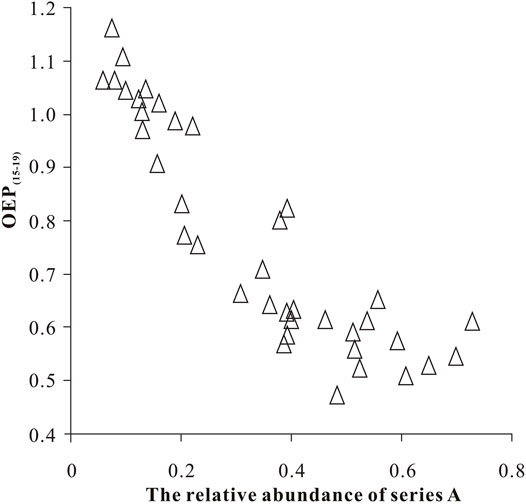
FIGURE 6. The correlations between the relative abundance of Series A and OEP of short-chain n-alkanes (with 40 samples).
The sources of long-chain branched/cyclic alkanes are still uncertain at present. Since it is difficult to detect the C2n branched/cyclic alkanes in organisms, some scholars suggest that such compounds are mainly from plastic contamination (Grosjean and Logan 2007; Brocks et al., 2008). Regarding the BAQCs detected in this study, we believe that the possibility of subsequent contamination can be ruled out. First, contamination during sample storage and experiments can be ruled out because they are detected in neither the contrast sample nor laboratory blank sample. Furthermore, the fact that such compounds is not from laboratory contamination has been confirmed by many laboratories. Second, samples are collected from the rural desert area without human domestic waste. Thus, these samples are unlikely to suffer from plastic contamination. Finally, land surface soil contamination can be ruled out. Though Bai et al. (2006) found BAQCs during soil analysis, the main peaks of the A series in the soil around the study area (arid-cold climate in western China) are all high carbon number C29, which are significantly different from the A series with main peaks of medium carbon number C21. In addition, samples are rinsed multiple times before testing. Thus, the probability of soil contamination is very small. On the other hand, long-chain branched/cyclic alkanes are also discovered in deep-sea hydrothermal reservoirs, further proving that it cannot be plastic contamination (Kenig et al., 2003).
Scholars generally believe that the likelihood of contamination is small, and they are prone to accept biogenic origin (Kenig et al., 2005). Based on the environment detected according to the branched/cyclic alkanes, they likely originate from some bacteria or algae. Every series of long-chain branched alkanes detected in this study is expressed only as an odd-carbon or even-carbon distribution pattern. In addition, the changes in abundance of several series occur simultaneously and regularly. This evidence indicates that they may have come from specific (or unified) biological sources. However, the specific parental material source of the long-chain branched alkanes is uncertain. Based on the detected environment, scholars have speculated that the long-chain branched alkanes may be from bacteria or algae, such as existing prokaryotes (Kenig et al., 2002), nonphotosynthetic sulfide-oxidizing bacteria (Kenig et al., 2003), thermophilic bacteria and thiophilic bacteria in a closed environment (Greenwood et al., 2004), heterotrophic bacteria (Flaviano et al., 1994; Zhang et al., 2014), cyanobacteria (Shiea et al., 1990; Aroui et al., 2000) and green algae (Derenne et al., 1996).
Additional research shows that the living environments of these archaeas and bacteria are similar to the detected environment of the long-chain branched alkanes. For example, they mostly belong to existing prokaryotes, which are consistent with the detection of long-chain branched alkanes in the sediments from the Paleoproterozoic Era to the present (Kenig et al., 2003). Moreover, they commonly appear in areas with rapid decomposition of organic matter, such as swamps, wetland soil, and aquatic sediments, which is consistent with the fact that long-chain branched alkanes are mostly detected in black shales (Kenig et al., 2003). These prokaryotes are also present in deep-sea thermal water vents, andlong-chain branched alkanes have also been detected in deep-sea thermal fluids (Kenig et al., 2003). In addition, such microbes mostly occur to environments with still water and suitable temperatures (20°C-40°C), and they coexist with sulfide-oxidizing bacteria, which is consistent with the previous assertion that the long-chain branched alkanes may come from closed environments with thermophilic bacteria and thiophilic bacteria characteristics (Kenig et al., 2003).
However, most of these prokaryotes can only survive in hypoxic environments in which organic matter is rapidly decomposed, which is different from the previous assertion that long-chain branched alkanes indicate a weak oxidizing environment (Kenig et al., 2003; Bai et al., 2006; Zhang et al., 2007). The content of the redox-sensitive trace elements including U, Mo, V and Ni, are enriched in reducing environments (Francois 1988; Russell and Morford 2001; Schroder and Grotzinger 2007) in the sedimentary rocks can be used to reconstruct the redox status of the sedimentary environment in the study area. The U and Mo contents in the study area are universally higher than those of standard rocks (PAAS), which have standard values of two for Mo and 3.1 for U. The “enrichment factor” (EF) is an effective indicator for comparing the respective enrichments of Mo and U (Tribovillard et al., 2006; Algeo and Tribovillard 2009; Tribovillard et al., 2012).
Where
X and Y stand for the weight concentrations of elements X and Y, respectively. Y can be Th or Al; in this study, we used Th. If XEF is greater than 1, this indicates that the element has undergone enrichment.
In the study area, the UEF values were between 0.9 and 9.2, the MoEF values were in the range of 0.1–207, and most samples reflected enrichment characteristics (Table 4), which suggests a strong reducing environment during deposition. In addition, several studies have suggested that Ni/Co. > 4 (Yarincik et al., 2000) and &U > 1 (Wignall and Twitchett 1996; Kimura and Watanabe 2001) indicate that the sedimentary rocks formed in a hypoxic environment. In our samples, the Ni/Co. value is between 4.10 and 42.36, and &U is between 0.72 and 1.67 (Table 4), which generally indicates a strong reducing environment. At the same time, long-chain branched/cyclic alkanes have been detected in deep-sea thermal fluids and at lake bottoms (Kenig et al., 2003; Zhang, et al., 2014), which further to demonstrate that long-chain branched/cyclic alkanes exist on hypoxic environments. In summary, this study suggests that long-chain branched/cyclic alkanes may come from prokaryotic archaea or bacteria and that the appearance of long-chain branched/cyclic alkanes may indicate a hypoxic environment.
Conclusion
This study is the first to detect multiple series of BAQCs (series A-I), cycloalkanes, MMAs, MEAs in the Carboniferous shales of the Wuwei Basin, China. Each series is expressed as an exclusively odd-carbon or even-carbon distribution pattern, and multiple types of the same series can appear concurrently with regular changes in abundance. All of these series are expressed as exclusively odd or exclusively even carbon numbers with consistent changes of abundance in the samples. Meanwhile, based on the analysis of basic geochemical characteristics and other biomarkers of the samples, we presume that it possessed a similar parent materials or evolutionary process and weak oxidation-reduction sedimentation environments. In addition, the results show that the abundance of the long-chain branched alkanes is highly correlated with the odd-even predominances (OEP (15–19)) of the short-chain n-alkane. The lower the OEP (15–19) values of the short-chain n-alkane are, the higher the abundance of the long-chain branched alkanes. The significant even-carbon dominance of the short-chain n-alkanes indicates the strong input of archaea or algal organic matter. These archaeas or algal organic matter are able to survive in specific paleoenvironments with the rapid decomposition of organic matter. The relatively wide occurrence and high abundance of these alkanes suggests a relationship with specific paleoenvironment and paleoclimate conditions.
Data Availability Statement
The original contributions presented in the study are included in the article/supplementary material, further inquiries can be directed to the corresponding author.
Author Contributions
LX: Conceptualization, Writing-original draft, Writing-review and editing. Responsible for sample measuring, Validation. JW: Funding acquisition, Project administration, Participate in Writing-review or editing.
Funding
This study was funded by a Major Scientific project of PetroChina Company Limited (Grant number 2016ZX05003-006X) and the National Natural Science Foundation of China (No.42172169).
Conflict of Interest
Authors LX and JW were employed by company Petrochina Northwest Research Institute of Petroleum Exploration and Development.
Publisher’s Note
All claims expressed in this article are solely those of the authors and do not necessarily represent those of their affiliated organizations, or those of the publisher, the editors and the reviewers. Any product that may be evaluated in this article, or claim that may be made by its manufacturer, is not guaranteed or endorsed by the publisher.
References
Algeo, T. J., and Tribovillard, N. (2009). Environmental Analysis of Paleoceanographic Systems Based on Molybdenum-Uranium Covariation. Chem. Geology. 268 (3), 211–225. doi:10.1016/j.chemgeo.2009.09.001
Aroui, K., Conaghan, P. J., Walter, M. R., Bischoff, G. C. O., and Grey, K. (2000). Reconnaissance Sedimentology and Hydrocarbon Biomarkers of Ediacarian Microbial Mats and Acritarchs, Lower Ungoolya Group, Officer Basin. Precambrian Res. 100, 235–280. doi:10.1016/S0301-9268(99)00076-5
Bai, Y., Fang, X., Wang, Y., Kenig, F., Chen, X., and Wang, Y. (2006). Branched Alkanes with Quaternary Carbon Atoms in Chinese Soils: Potential Environmental Implications. Chin. Sci. Bull. 51 (9), 1115–1122. doi:10.1007/s11434-006-1115-3
Brocks, J. J., Grosjean, E., and Logan, G. A. (2008). Assessing Biomarker Syngeneity Using Branched Alkanes with Quaternary Carbon (BAQCs) and Other Plastic Contaminants. Geochimica et Cosmochimica Acta 72 (3), 871–888. doi:10.1016/j.gca.2007.11.028
Derenne, S., Largeau, C., and Berkaloff, C. (1996). First Example of an Algaenan Yielding an Aromatic-Rich Pyrolysate, Possible Geochemical Implications on marine Kerogen Formation. Org. Geochem., 24 (6-7), 617–627. doi:10.1016/0146-6380(96)00053-8
Dill, H., Teschner, M., and Wehner, H. (1988). Petrography, Inorganic and Organic Geochemistry of Lower Permian Carbonaceous Fan Sequences (Brandschiefer Series)-Federal Republic of Germany: Constraints to Their Paleogeography and Assessment of Their Source Rock Potential. Chem. Geol., 67 (3-4), 307–325. doi:10.1016/0009-2541(88)90136-2
Elias, V. O., Simoneit, B. R. T., and Cardoso, J. N. (1997). Even N-Alkane Predominances on the Amazon Shelf and a Northeast Pacific Hydrothermal System. Naturwissenschaften 84, 415–420. doi:10.1007/s001140050421
Feng, X., Simpson, A. J., Gregorich, E. G., Elberling, B., Hopkins, D. W., Sparrow, A. D., et al. (2010). Chemical Characterization of Microbial-Dominated Soil Organic Matter in the Garwood Valley, Antarctica. Geochimica et Cosmochimica Acta 74 (22), 6485–6498. doi:10.1016/j.gca.2010.08.019
Flaviano, C., Berre, F. L., Derenne, S., Largeau, C., and Connan, J. (1994). First Indications of the Formation of Kerogen Amorphous Fractions by Selective Preservation. Role of Non-hydrolysable Macromolecular Constituents of Eubacterial Cell walls. Org. Geochem. 22 (3-5), 759–771. doi:10.1016/0146-6380(94)90137-6
Francois, R. (1988). A Study on the Regulation of the Concentrations of Some Trace Metals (Rb, Sr, Zn, Pb, Cu, V, Cr, Ni, Mn and Mo) in Saanich Inlet Sediments, British Columbia, Canada. Mar. Geol. 83 (1), 285–308. doi:10.1016/0025-3227(88)90063-1
Greenwood, P. F., Arouri, K. R., Logan, G. A., and Summons, R. E. (2004). Abundance and Geochemical Significance of C2n Dialkylalkanes and Highly Branched C3n Alkanes in Diverse Meso- and Neoproterozoic Sediments. Org. Geochem. 35 (3), 331–346. doi:10.1016/j.orggeochem.2003.10.013
Grosjean, E., and Logan, G. A. (2007). Incorporation of Organic Contaminants into Geochemical Samples and an Assessment of Potential Sources: Examples from Geoscience Australia marine Survey S282. Org. Geochem. 38, 853–869. doi:10.1016/j.orggeochem.2006.12.013
Kenig, F., Simons, D.-J. H., Crich, D., Cowen, J. P., Ventura, G. T., Rehbein-khalily, T., et al. (2003). Branched Aliphatic Alkanes with Quaternary Substituted Carbon Atoms in Modern and Ancient Geologic Samples. Proc. Natl. Acad. Sci. 100 (22), 12554–12558. doi:10.1073/pnas.1735581100
Kenig, F., Simons, D.-J. H., Crich, D., Cowen, J. P., Ventura, G. T., Rehbein-Khalily, T., et al. (2005). Structure and Distribution of Branched Aliphatic Alkanes with Quaternary Carbon Atoms in Cenomanian and Turonian Black Shales of Pasquia Hills (Saskatchewan, Canada). Org. Geochem. 36 (1), 117–138. doi:10.1016/j.orggeochem.2004.06.014
Kenig, F., Simons, D. H., and Crich, D. (2002). Alkanes with a Quaternary Carbon centre: a 2200 Myr Record of Sulfide Oxidizing Bacteria. Geochim. Cosmochim. Ac. 66 (15A), A393.
Kenig, F., Sinninghe Damsté, J. S., Kock-van Dalen, A. C., Rijpstra, W. I. C., Huc, A. Y., and de Leeuw, J. W. (1995). Occurrence and Origin of Mono-, Di-, and Trimethylalkanes in Modern and Holocene Cyanobacterial Mats from Abu Dhabi, United Arab Emirates. Geochimica et Cosmochimica Acta 59 (14), 2999–3015. doi:10.1016/0016-7037(95)00190-5
Kimura, H., and Watanabe, Y. (2001). Oceanic Anoxia at the Precambrian-Cambrian Boundary. Geology 29 (11), 995–998. doi:10.1130/0091-7613(2001)029<0995:oaatpc>2.0.co;2
Kissin, Y. V., Feulmer, G. P., and Payne, W. B. (1986). Gas Chromatographic Analysis of Polymethyl-Substituted Alkanes. J. Chromatogr. Sci. 24 (4), 164–169. doi:10.1093/chromsci/24.4.164
Li, R., Liu, G., Ma, G., Gao, G., Fang, J., and Liu, Y. (2010). Sequence Stratigraphic Analysis of Carboniferous in Wuwei Basin. Geoscience 24 (6), 1048–1056. [In Chinese with English abstract].
Logan, G. A., Hinman, M. C., Walter, M. R., and Summons, R. E. (2001). Biogeochemistry of the 1640 Ma McArthur River (HYC) lead-zinc Ore and Host Sediments, Northern Territory, Australia. Geochimica et Cosmochimica Acta 65 (14), 2317–2336. doi:10.1016/S0016-7037(01)00599-3
Menor-Salván, C., Tornos, F., Fernández-Remolar, D., and Amils, R. (2010). Association between Catastrophic Paleovegetation Changes during Devonian-Carboniferous Boundary and the Formation of Giant Massive Sulfide Deposits. Earth Planet. Sci. Lett. 299 (3-4), 398–408. doi:10.1016/j.epsl.2010.09.020
Meyers, P. A., and Ishiwatari, R. (1993). Lacustrine Organic Geochemistry-An Overview of Indicators of Organic Matter Sources and Diagenesis in lake Sediments. Org. Geochem. 20 (7), 867–900. doi:10.1016/0146-6380(93)90100-P
Mycke, B., Michaelis, W., and Degens, E. T. (1988). Biomarkers in Sedimentary Sulfides of Precambrian Age. Org. Geochem. 13 (4-6), 619–625. doi:10.1016/0146-6380(88)90081-2
Russell, A. D., and Morford, J. L. (2001). The Behavior of Redox-Sensitive Metals across a Laminated-Massive-Laminated Transition in Saanich Inlet, British Columbia. Mar. Geol. 174 (1-4), 341–354. doi:10.1016/S0025-3227(00)00159-6
Schröder, S., and Grotzinger, J. P. (2007). Evidence for Anoxia at the Ediacaran-Cambrian Boundary: the Record of Redox-Sensitive Trace Elements and Rare Earth Elements in Oman. J. Geol. Soc. 164, 175–187. doi:10.1144/0016-76492005-022
Shiea, J., Brassell, S. C., and Ward, D. M. (1990). Mid-chain Branched Mono- and Dimethyl Alkanes in Hot spring Cyanobacterial Mats: A Direct Biogenic Source for Branched Alkanes in Ancient Sediments? Org. Geochem. 15 (3), 223–231. doi:10.1016/0146-6380(90)90001-G
Tribovillard, N., Algeo, T. J., Baudin, F., and Riboulleau, A. (2012). Analysis of marine Environmental Conditions Based Onmolybdenum-Uranium Covariation-Applications to Mesozoic Paleoceanography. Chem. Geology. 324-325 (24), 46–58. doi:10.1016/j.chemgeo.2011.09.009
Tribovillard, N., Algeo, T. J., Lyons, T., and Riboulleau, A. (2006). Trace Metals as Paleoredox and Paleoproductivity Proxies: An Update. Chem. Geology. 232, 12–32. doi:10.1016/j.chemgeo.2006.02.012
Wignall, P. B., and Twitchett, R. J. (1996). Oceanic Anoxia and the End Permian Mass Extinction. Science 272 (5265), 1155–1158. doi:10.1126/science.272.5265.1155
Yan, C., and Yuan, J. (2011). Sedimentary Environment and Hydrocarbon Potential of Carboniferous in Wuwei Basin. Nat. Gas Geosci. 22, 267–274. [In Chinese]. doi:10.11764/j.issn.1672-1926.2011.02.267
Yarincik, K. M., Murray, R. W., Lyons, T. W., Peterson, L. C., and Haug, G. H. (2000). Oxygenation Hhistory of Bbottom Wwaters in the Cariaco Basin, Venezuela, over the Ppast 578,000 Yyears: Results from Rredox-Ssensitive Mmetals (Mo, V, Mn, and Fe). Paleoceanography 15, 593–604. doi:10.1029/1999PA000401
Zhang, H., Chang, F., Li, B., Lei, G., Chen, Y., Zhang, W., et al. (2007). Branched Aliphatic Alkanes of Shell Bar Section in Qarhan Lake, Qaidam Basin and Their Paleoclimate Significance. Chin. Sci. Bull. 52, 1248–1256. doi:10.1007/s11434-007-0167-3
Zhang, Y., Su, Y., Liu, Z., Chen, X., Yu, J., Di, X., et al. (2014). Long-chain Branched/cyclic Alkanes in Recent Sediment of Lake Fuxian and Their Environmental Implications. Chin. Sci. Bull. 59, 1139–1150. doi:10.1007/s11434-014-0159-z
Keywords: BAQCs. C2n compounds, long-chain cyclicalkane, biomarker, characteristics and origin, carboniferous shales
Citation: Xu L and Wang J (2022) Distribution Characteristics of Long-Chain Branched Alkanes With Quaternary Carbon Atoms in the Carboniferous Shales of the Wuwei Basin, China. Front. Earth Sci. 9:819732. doi: 10.3389/feart.2021.819732
Received: 22 November 2021; Accepted: 30 December 2021;
Published: 20 January 2022.
Edited by:
Yongli Wang, Institute of Geology and Geophysics (CAS), ChinaReviewed by:
Zhifu Wei, Institute of Geology and Geophysics (CAS), ChinaGen Wang, Northwest Institute of Eco-Environment and Resources (CAS), China
Copyright © 2022 Xu and Wang. This is an open-access article distributed under the terms of the Creative Commons Attribution License (CC BY). The use, distribution or reproduction in other forums is permitted, provided the original author(s) and the copyright owner(s) are credited and that the original publication in this journal is cited, in accordance with accepted academic practice. No use, distribution or reproduction is permitted which does not comply with these terms.
*Correspondence: Li Xu, xuli0055@126.com