- 1Key Laboratory of Geological Hazards on the Three Gorges Reservoir Area, Ministry of Education, China Three Gorges University, Yichang, China
- 2National Field Observation and Landslides Research Station in the Three Gorges Reservoir Area, China Three Gorges University, Yichang, China
- 3China Yangtze Ecology and Environment Co. Ltd., Wuhan, China
In Ganzhou City, China, a complex bedrock lithology and structure, diverse topography, frequent engineering works, and abundant rainfall generate frequent, sudden, small-scale landslides that are difficult to prevent and control. This study integrates evidence data from a field investigation of landslides with geological-engineering analogues to document the distribution and development of these geohazards in Ganzhou City. Based on the distribution of landslides across different types of bedrock and soil, we identify five lithological groups prone to slope failure: granite, metamorphics (slate and phyllite), red sedimentary layers, clastic sedimentary rocks with weak interlayers, and loose Quaternary deposits. Granite and metamorphic bedrock are the two lithologies most prone to landslides. Our analysis of the genesis and mode of slope failure suggests that most landslides in Ganzhou City originated from four modes of slope failure: scouring erosion collapse, steep slope collapse, rock sliding along a rock stratum, and wedge-shaped block sliding and caving. An in-situ model test and numerical simulations were used to explore the evolution of slope deformation and failure on the most landslide-prone lithological groups, and the accumulation of debris post-failure. This work provides a reference for the assessment of the risk from, and the management of, landslide geohazards in Ganzhou City and geologically similar regions.
Introduction
Landslides, the most frequent geohazard in China, have attracted considerable research attention (Wang et al., 2011; Zhou and Zhao, 2013; Deng et al., 2021). Most of this research, however, has focused on large-scale landslides, involving rapid movement and large-scale destruction. In China, such landslides are primarily distributed in the Three Gorges Reservoir region (Gu et al., 2017; Zhang et al., 2018; Huang. et al., 2020a; Wang et al., 2021), southwestern China (Jiang et al., 2014; Gao and Sang 2017), and the northwestern Loess Plateau (Xu et al., 2017; Leng et al., 2018). Small-scale landslides are often overlooked, although they also, cause serious damage. For example, from 1998 to July 2019, there were 22,377 geohazard incidents in China’s Southern Jiangxi Province. In 2015, 2,196 landslide events that caused significant casualties and economic damage were reported (Ke et al., 2017). The southern Jiangxi region has a complex geological structure and bedrock lithology, diverse topography and geomorphology, widespread engineering works, and experiences heavy rainfall. For these reasons, landslides in this region tend to be sudden, frequent, and widespread, but relatively small in scale; therefore, they are extremely difficult to prevent and control (Ke et al., 2017). The steep morphological gradient formed by cutting into slopes to build houses and repair roads is the primary cause of frequent landslides in this region. When disasters occur, houses that are located too close to slopes often result in heavy losses (Figure 1). The mode of slope failure in the Southern Jiangxi Province, and the damage that this failure can incur, were poorly understood by the Ministry of Transport. This has resulted in a failure to provide effective guidelines for house construction and road repair. To facilitate the effective assessment of landslide risk and the management of current and future disasters, the study of slope failure and the dynamics of landslide in the Southern Jiangxi Province is, therefore, necessary (Huang et al., 2016; Huang et al., 2020b).
As mentioned, previous studies of landslides in China have mainly focused on large-scale landslides, such as the Qianjiangping (Du et al., 2013; Yin et al., 2015), Daguangbao (Huang et al., 2012; Cui et al., 2018), and Shaziba (Shen et al., 2021) landslides. The occurrence and slope failure processes of these landslides have been explored through various methods, including high-resolution remote sensing, large-scale physical model tests, and on-site monitoring. In addition, the mode of slope failure and the dynamic run-out processes of these landslides have been investigated in detail (Youssef and Maerz, 2013; Ouyang et al., 2017). However, the methods and results of this research, are not directly applicable to the diverse lithology and small-scale landslides of Ganzhou City, and the mode of slope failure and other characteristics of the latter can be expected to differ significantly from those of large-scale landslides in other areas.
To address this issue, we conducted an extensive geo-engineering field survey of landslides in Ganzhou City. We systematically analysed the distribution, geomorphic and lithological characteristics, the slope failure mode of these geohazards, as well as the factors that influence their development on different lithologies. To further explore the damage caused by various modes of slope failure, we performed in situ model testing on a typical landslide in the city. Additionally, where in-situ experiments were difficult to conduct, we used numerical modelling to simulate the slope failure and accumulation of debris after failure. The insights into the landslide processes gained by these methods can underpin the prevention and control of landslides in Ganzhou City and similar geological settings elsewhere in the world.
Survey of the Study Area and Landslide-influencing Factors
Overview of the Study Area
Ganzhou City is located in a mountain basin in Southern Jiangxi Province, China. The topographical gradient spans from these mountains and their foothills to the centre of the basin (Ganzhou City) and from the south to the north. Undulating mountains and hills account for more than 80% of the total area. The bedrock, with many well-exposed outcrops, comprises Proterozoic to Cainozoic sedimentary and igneous lithologies, such as Sinian gneiss, phyllite, metasandstone, and quartzite; Cambrian feldspar-quartz metasandstone and carbonaceous slate; Ordovician sandstone, siltstone, and shale; Devonian feldspar-quartz sandstone and siltstone glutenite; Carboniferous limestone and dolomite; chert nodules; coal-bearing siliciclastics; Permian siliceous shale and limestone, and Cretaceous sandstone, glutenite siltstone, and strongly weathered multistage granite. The rich mineral resources in the mountains around Ganzhou originated from multistage orogenesis and igneous activity. The region has a subtropical, monsoonal climate, with distinct seasons and abundant rainfall (average annual rainfall: 1856.3 mm).
Factors Influencing Landslides in Ganzhou
From January 1998 to July 2019, a total of 22,377 incidents of slope failure (landslides, slope collapse, debris flows, ground collapse, etc.) occurred in Ganzhou City. Most of these incidents were landslides at a scale generally smaller than 10000 m3. An investigation identified 11,976 hidden geohazards in Ganzhou City (8,159 landslides, 3,592 sites of slope collapse, 181 sites of ground collapse, 43 debris flows, and 1 ground fissure), with landslide geohazard accounting for more than 98%.
In Ganzhou City, landslides occur on a broad range of slopes, which vary between different areas and bedrock. For instance, in the hilly areas, the landslide-prone slopes are mostly between 15° and 25°, and in mid- and low-altitude areas, these slopes are generally between 30° and 45°. Figures 2A,B based on the detailed, 1:50,000 geohazard map of Ganzhou City, show the frequency of landslides in different geomorphic settings: geohazards in Ganzhou City are mainly distributed on slopes with a gentle (15° < α ≤ 35°) and moderate (35° < α ≤ 55°) incline, in mountainous and hilly areas, where steep slopes are relatively small. No landslides occurred on the cliffs.
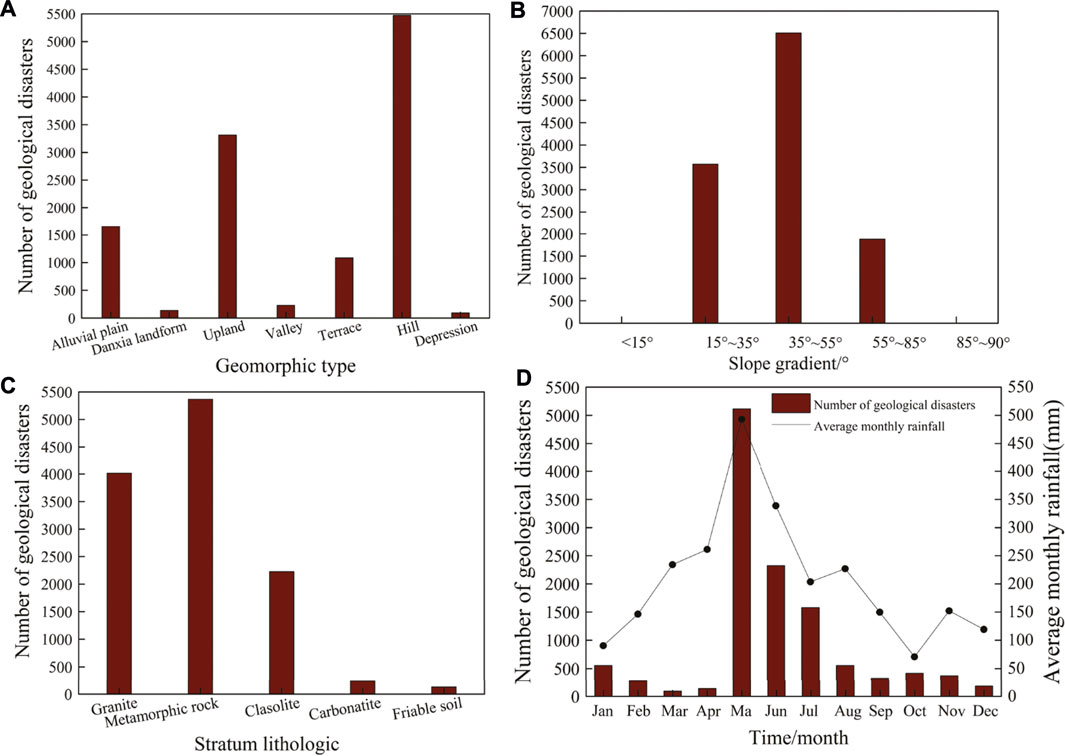
FIGURE 2. Landslides in Ganzhou City from 1998 to 2019 in relation to, (A) topography and geomorphology, (B) slope gradient, (C) lithology, and, (D) average monthly rainfall.
The geological structure of the Ganzhou City area is complex, with two groups of folds, four groups of deep faults, and joints. Structural faults, especially the deep Dayu-Nancheng fault, exercise greater influence on geohazards.
The two main internal factors that influence landslide geohazards in the region are topography and geological structure and composition, with lithology playing a key role. Landslides are frequent on granite and metamorphic bedrock and its soil mantle, and are relatively rare in other bedrock and soils. This is in line with the distribution of multiple geohazards in mountainous and hilly areas where granite and metamorphic bedrock are typically located. Metamorphic and granitic bedrock, the most disaster-prone lithological groups, accounted for 78% of all landslides from 1998 to 2019, followed by red siliciclastic sediments, clastic sediments with weak interlayers, and loose Quaternary deposits and soils (Figure 2C).
The main external factors that influence landslide geohazards in Ganzhou City are rainfall and engineering work. The rainy season in this region is mainly from March to August, with the highest rainfall in May and June (the wet period, accounting for 58.9% of the annual precipitation). The dry season, from November to January, accounts for about 10% of the annual precipitation. During the rest of the year, the precipitation is moderate. Landslides in Ganzhou City mainly occurred from May to June, during the local rainy season. With a maximum frequency in May, landslides lagged behind the maximum rainfall (Figure 2D). Furthermore, Ganzhou City is rich in mineral resources and has a long history of mining. Mining usually involves slope cutting, which makes the slope steeper, leading to frequent landslides under heavy rainfall.
In summary, bedrock lithology, geological structure, topography, and geomorphology are the main internal factors influencing landslide geohazards in Ganzhou, with bedrock lithology being the most important. Rainfall and artificially cut slopes are the main external factors.
Mode of Slope Failure
Based on the distribution of landslides between 1998 and 2019 (*) and the conditions and geomechanical properties of bedrock and soil, five landslide-prone lithological groups were identified in the Ganzhou City area: granite, metamorphics (slate and phyllite), red siliciclastic sediments, clastic sedimentary rocks with weak interlayers, and loose Quaternary deposits. Granite and metamorphic bedrock are more prone to landslides than other lithological groups. Owing to the complexity and diversity of bedrock and soil, different degrees of weathering, and variations in slope form and scale, the same external conditions result in the type and scale of landslides across these lithological groups. on the on-site investigation of slope failure suggested that landslides in Ganzhou City primarily result from the following four genetic mechanisms: a) scouring erosion collapse, b) steep slope collapse, c) rock sliding along a rock stratum, and, d) wedge-shaped block sliding and caving.
Scouring Erosion Collapse
Scouring erosion collapse occurs mainly on Jurassic porphyritic two-cloud potassium feldspar granite with a thick weathering mantle, Baiyun monzonitic granite, Triassic porphyritic monzonitic potassium feldspar granite, and Cretaceous red sandstone–conglomerate. This type of slope collapse occurs under the conditions of a thick weathering mantle and sparse vegetation on the slope surface. The weathered soil has a loose structure, with a coarse particle size and poor sorting, zero to weak viscosity, and high permeability. Weathered soil of this type is easily washed away by water. The erosion collapse proceeds in three stages:
1) Under the action of rainfall, sheet flow on the slope surface, over the fully weathered soil, removes soil particles and forms rills locally (Figure 3A).
2) Erosion progresses from the previous stage: the sheet flow becomes strand flow, and surface erosion gradually evolves into linear, gully erosion through the gradual expansion of rills into gullies. As the gullies deepen and widen, collapse due to gravity becomes increasingly frequent (Figure 3B).
3) As the erosion grooves increase in size, water drops moving along the groove wall further erode the weathered soil at the bottom of the groove. With the gradual intensification of erosion and undermining of the soil by enlarging erosion cavities, the soil in the upper part of the slope collapses due to gravity. As collapse progresses, the mountain slope gradually reshapes, until it acquires a smooth, gentle incline (Figure 3C).
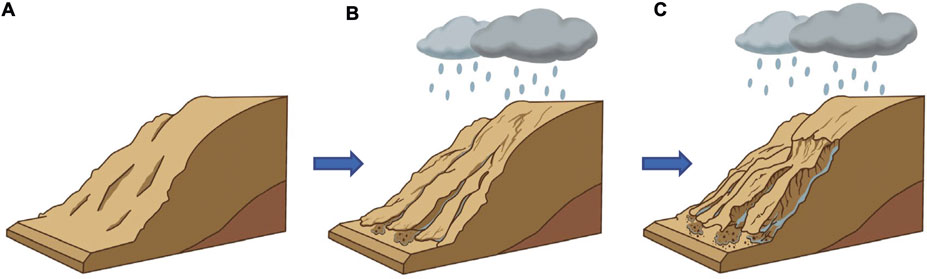
FIGURE 3. Schematic diagram of scouring erosion collapse: (A) First stage; (B) Second stage; (C) Third stage.
A characteristic site of scouring erosion collapse is the resettlement housing area of Chou Village, about 10 miles from Ganzhou City. The bedrock lithology was Triassic porphyritic granite. The entire slope was formed on fully weathered, poorly sorted, loose soil that essentially lacked cohesion. A steep, high landslide, with a height of approximately 10–15 m and a slope of approximately 60–70°, occurred due to the cutting of the slope. A retaining wall of approximately 3–4 m was present at the foot of the slope. The upper part of the retaining wall lacked protection or reinforcement (Figure 4A). Due to continuous, heavy rainfall, grooves and seepage pipes formed both internally and on the slope surface which contained loose, weathered sand (Figure 4A). This loose sand flowed along the slope surface and grooves, forming a sediment flow. Two soil pits (Figures 4C–E) and some fissures (Figure 4B) were present at the top of the landslide. Rainwater gathered in the pits and penetrated the soil, causing fine particles to flow from the large pores. Continuous scouring of the landslide surface by rainwater resulted in the formation of grooves, while unstable soils collapsed locally (Figures 4F–H).
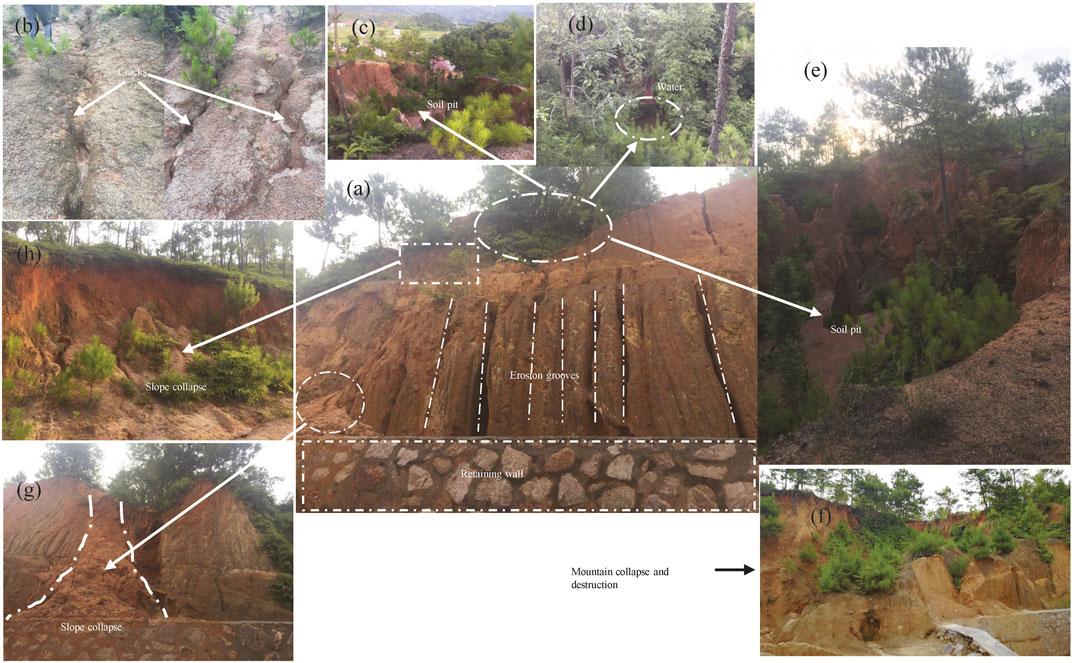
FIGURE 4. Scouring erosion collapse in Zhifu village, Ganzhou City: (A) Erosion grooves on the slope face; (B) Fissures at the top of the slope; (C) Soil pit at the top of the slope; (D) Water in the pit; (E) Another soil pit at the top of the slope; (F) Slope collapse and damage, (G–H) Slope collapse.
Steep Slope Collapse
In the Ganzhou area, steep slope collapse is widespread on high and steep slopes on granite and metamorphic bedrock and their thick, cohesive weathering mantle. Three different modes of slope failure were distinguished on the basis of the thickness of the weathering mantle and the height and gradient of the slope: 1) small-scale collapse landslides, 2) flow-collapse landslides, and, 3) collapse landslides.
(1) Small-scale collapse landslides.
In the study area, small-scale collapse landslides occur primarily on steep slopes with a height of 3–5 m. The upper part of the slope is completely weathered, cohesive soil of medium permeability, while the lower part of the slope is relatively impermeable, or weakly permeable, bedrock. Slope failure occurs in two stages:
1) Owing to artificial slope cutting, the slope surface is unloaded and tension fissures appear (Figure 5A).
2) Under continuous rainfall, rainwater infiltrates the slope, and the weight of the soil increases. The softening of the soil decreases its shear strength, which causes the slope to slip along the underlying bedrock towards the free direction, eventually forming scattered deposits of collapsed soil (Figure 5B).
(2) Flow-collapse landslides.
In the study area, flow-collapse landslides occur mainly on fully weathered granite, slate and phyllite slopes. These slopes are steep, more than 15 m high, and formed on a thick weathering mantle with a loose, cohesive soil of low permeability. The slope failure mechanism is shown in Figure 6.
Under continuous heavy rainfall, the soil reaches a state of supersaturation with water, and its shear strength is greatly reduced. The upper soil collapses and disintegrates, and the loose collapse debris mixes with water to form a debris flow. Soils slide rapidly down the steep slope, forming a flow landslide that travels at a distance of more than 50 m. Such collapse events can cause significant long-range damage.
(3) Collapse landslides.
In the Ganzhou area, collapse landslides mainly occur on steep slopes with a height of more than 5 m and a thick weathering mantle over the jointed bedrock. Landslides of this type involve three stages.
1) Unloading fissures appear on the surface of steeply inclined, natural or artificial slopes due to the decrease in soil weigh (Figure 7A).
2) As slope erosion progresses, unloading fissures link with structural joints (Figure 7B).
3) The fissured soil collapses under heavy rainfall. The collapsed soil impacts loose soil at the lower part of the slope, resulting in the sliding of the loose soil (Figure 7C).
Α small-scale collapse landslide was located behind the Tuniuxia residential house in Qixia Village, Ganzhou City. The bedrock was coarse-grained porphyritic biotite monzonitic granite, covered by an approximately 1–3-m-thick layer of cohesive soil. This soil underwent continuous deformation during the rainy season, and some of it collapsed (Figure 8A).
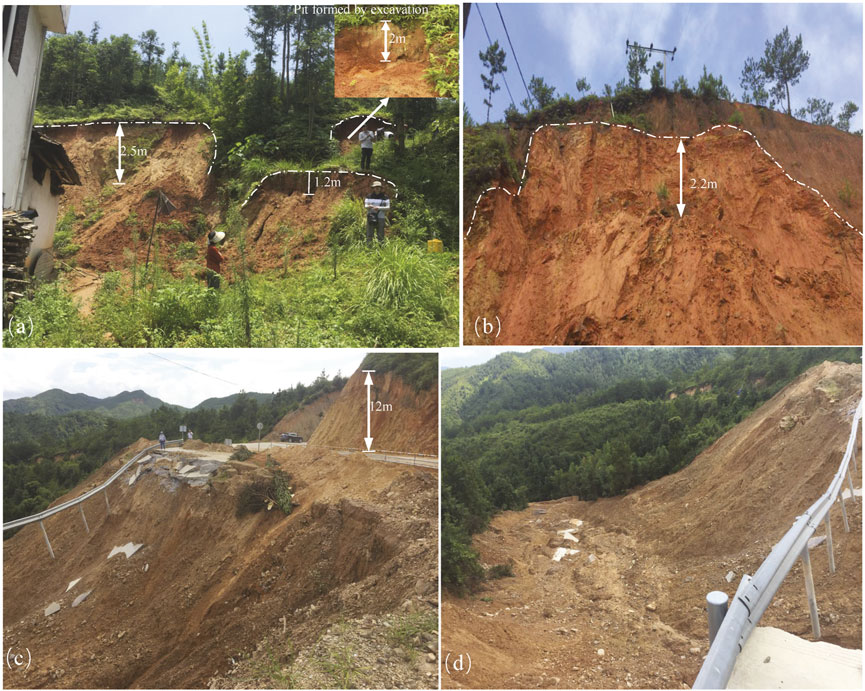
FIGURE 8. (A) Small-scale collapse landslide on a weathered granite slope behind a house in Qixia Village, Ganzhou City. (B) Collapse landslide on a steep slope on weathered granite, behind the Dinglong Village Committee House, Ganzhou City. (C,D) Steep, high slope on metamorphic rock next to the Kuantian County Road (Ganzhou City), which was damaged by a flow-collapse landslide.
A flow-collapse landslide was located near the X433 Kuantian County Road, in Ganzhou City. The bedrock is interbedded slate and phyllite (Z1) with a broken rock mass and thick weathering mantle. The slope is approximately 40–50° and approximately 150 m high. In July 2019, after continuous heavy rainfall, the slope soil slid downward, initiating a mudflow that destroyed the nearby highway. The trailing edge of the landslide was completely emptied, forming an approximately 15-m-high scarp. The mudflow spread over a distance of more than 400 m, and the fan-shaped debris accumulation at its lower part was approximately 40 m. This landslide affected approximately 30 m of the highway (Figures 8C,D).
A collapse landslide was located behind the village committee house in Dinglong Village, Ganzhou City. Here, the lithology is Lower Jurassic coarse-grained porphyritic biotite monzogranite, similar to that at the Qixia village site. The rock mass was jointed and draped with a thick weathering mantle which was also fissured. The slope was covered with yellow-brown, fully weathered soil. When the village committee building was constructed, the slope was cut and its gradient was steepened. On July 10, 2019, during a rainstorm, the slope suddenly failed, and the upper soil collapsed (Figure 8B).
Slope Failure Along a Bedrock Stratum
In the study area, landslides along a bedrock stratum developed on metamorphic bedrock with one or two dominant structural planes of weakness and a thin weathering mantle, and on clastic bedrock with weak interlayers. In both lithological groups, the structural plane of failure (a fracture or bedding plane) tends to dip in the same direction as the slope, at an angle of dip lower than that of the slope. This type of landslide progresses in three stages:
1) One or two groups of dominant structural planes (joints, fissures, bedding planes) were present in the bedrock, inclined in the same direction as the slope, at an inclination lower than that of the slope (Figure 9A).
2) As a result of artificial slope cutting, the structural plane(s) and unloading fissures propagated in the outer part of the slope. The length and width of the fissures expanded, and the fissured bedrock blocks detached from the parent rock body (Figure 9B).
3) The proliferation of fissures leads to an increase in permeability. Rainfall infiltration reduces the shear strength of the structural surface and increases the weight of the rock mass. As a result, the slope failed along the structural surface. The front and back sections of the unstable slope disintegrated, due to the lack of support and traction during sliding. Most of the collapsed debris accumulated at the toe (Figure 9C).
A rockslide along a bedrock stratum was located near the X824 County tunnel in Ganzhou City. Here, the bedrock is Upper Devonian, Zhongpeng Formation conglomerate (at the base) to feldspar quartz sandstone intercalated with shale. The landslide was located near a fault, and the bedrock was cut by dominant structural planes of tectonic origin. At this site, the weathering mantle slipped along such a structural plane after heavy rainfall (Figures 10A–C).
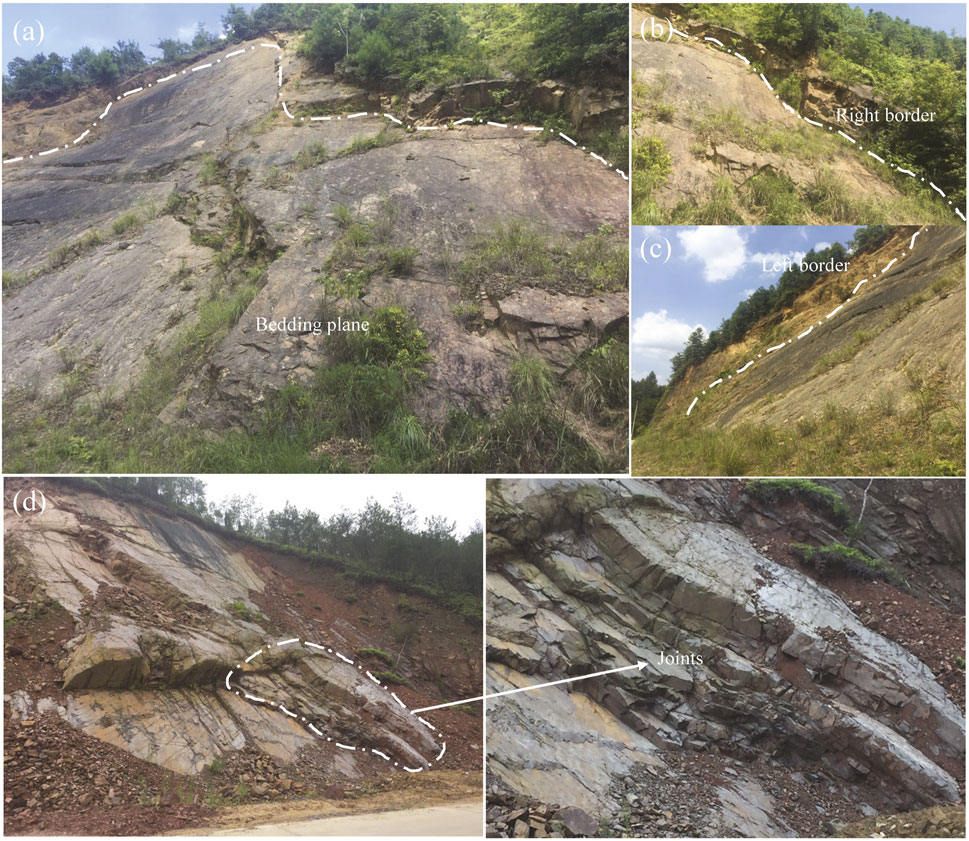
FIGURE 10. Rockslides along a bedrock stratum in Ganzhou City: (A–C) Slope failure along a bedding plane in sandstone, X824 County Road. (D) Metamorphic slope near the Xinwuxiacun Village road.
Another rockslide along a bedrock stratum is located near the Xinwuxia Village road, in Ganzhou City, on the bedrock of limestone and phyllite interbeds of the Upper Sinian Laohutang Formation. The artificial cutting of the slope enlarged the tectonic joints and fissures in the bedrock. The rock blocks also collapsed along the dominant structural plane (Figure 10D).
Wedge Block Sliding and Caving
In Ganzhou, wedge block sliding and caving occurs primarily on steep slopes of jointed and fractured, metamorphic, and clastic bedrock with weak layers and two or more dominant structural planes. Slope failure of this type progresses in three stages.
1) Multiple groups of structural planes in the bedrock intersect to form wedge blocks. These wedge blocks eventually slide in the direction of the slope (Figure 11A).
2) The erosion of steep, high slopes, exposes wedge blocks. Due to the unloading of the bedrock, fissures become wider and longer, rendering the wedge blocks potentially unstable (Figure 11B).
3) Rainfall infiltration weakens the structural plane(s), resulting in sliding or collapse of the unstable blocks (Figure 11C).
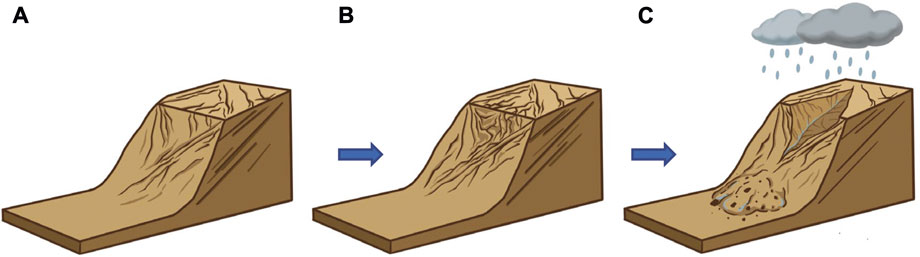
FIGURE 11. Wedge-shaped block sliding and caving: (A) First stage; (B) Second stage; (C) Third stage.
A site of wedge-shaped block sliding and caving is located in Kongmu Village, Ganzhou City. The bedrock is interbedded limestone and phyllite of the Upper Sinian Laohutang Formation, with developed joints and fractures, with there are two or more groups of dominant structural planes in the rock mass, and the rock mass is cut into wedge blocks. There is no protective measure for the slope, and the wedge block collapses under the action of rainfall (Figure 12).
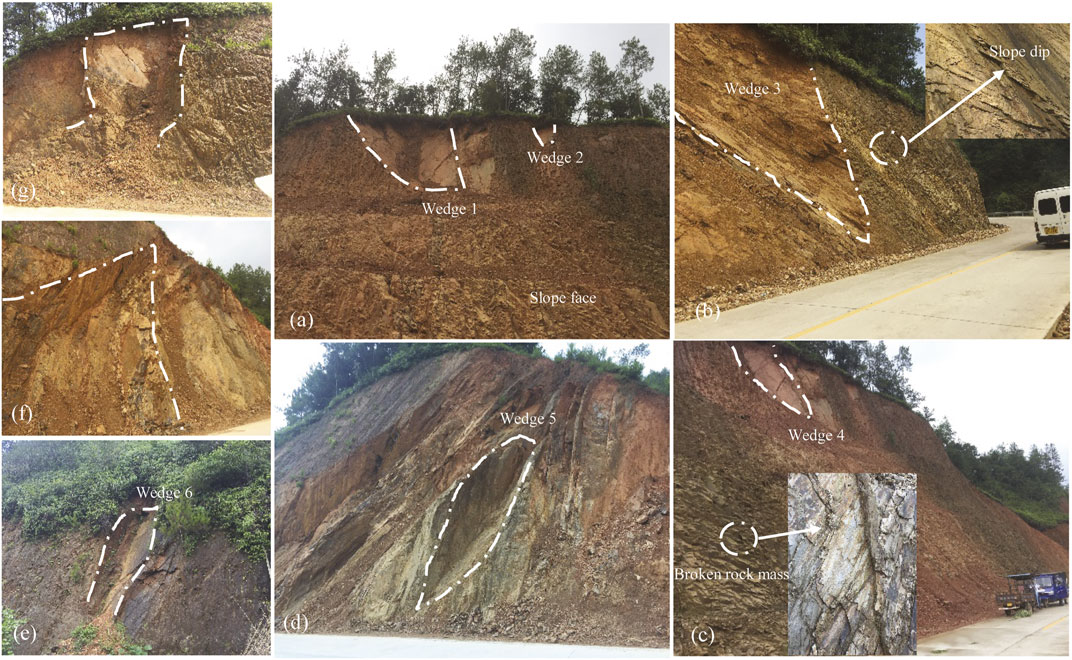
FIGURE 12. Wedge-shaped block sliding on metamorphic rock on the Kongmu Village Highway, Ganzhou City: (A) Slope face; (B) Right side face of slope; (C–E) Left side of the slope; (F,G) Views of the wedge-shaped body.
Evolution of Slope Deformation and Failure Under Rainfall
As mentioned in Factors Influencing Landslides in Ganzhou Section, in the Ganzhou City area, fully weathered granite and metamorphic bedrock were identified as especially prone to landslides from 1998 to 2019: 78% of all landslides occurred on the bedrock of these two lithologies. To further explore the evolution of deformation, failure, and post-failure accumulation on granite and metamorphic bedrock slopes under rainfall, we applied in-situ model testing and numerical simulation on four selected landslide sites with typical deformation characteristics in the Ganzhou City area.
In situ Model Test
Due to funding limitations, in-situ model testing was only conducted at one site: a slope on Tuniuxia Formation granite in Qixia Village, Ganzhou City. The original slope is shown in Figure 13A. The test slope, with a height of approximately 4 m, a gradient of approximately 60°, a length of approximately 5.3 m, and an upper platform width of approximately 2.5 m, was artificially excavated in coarse-grained porphyritic biotite monzogranite (Figure 13B), and physical and mechanical parameters of undisturbed soils were shown in Table 1. The model test, from the manual cutting of the slope (10 days), the installation of the test device (4 days), debugging (15 days), and slope failure (23 days from the onset of rainfall), lasted for approximately 2 months. The monitoring of slope deformation under rainfall showed that runoff occurred on the slope surface during the early stages of rainfall. Runoff was more intense on the left side of the slope (Figure 13C), where the soil was dense, making it difficult for rainwater to infiltrate. Under continuous rainfall, grooves developed and became enlarged on the left side of the slope (Figures 13D–G). However, water penetrated easily into the loose soil on the right side of the slope. The shallow soil in the upper part of the soil profile soon became saturated with water. This softened the soil and increased its weight, causing further expansion of the fissures and the formation of several soil blocks (Figure 13H). We found the upper slope was collapsed on the 22nd day of continuous rainfall (Figure 13I). The entire slope collapsed due to gravity on the 23rd day of continuous rainfall (Figure 13J). The slope failure mode conformed with small-scale landslides on a steep slope, described in Steep Slope Collapse Section.
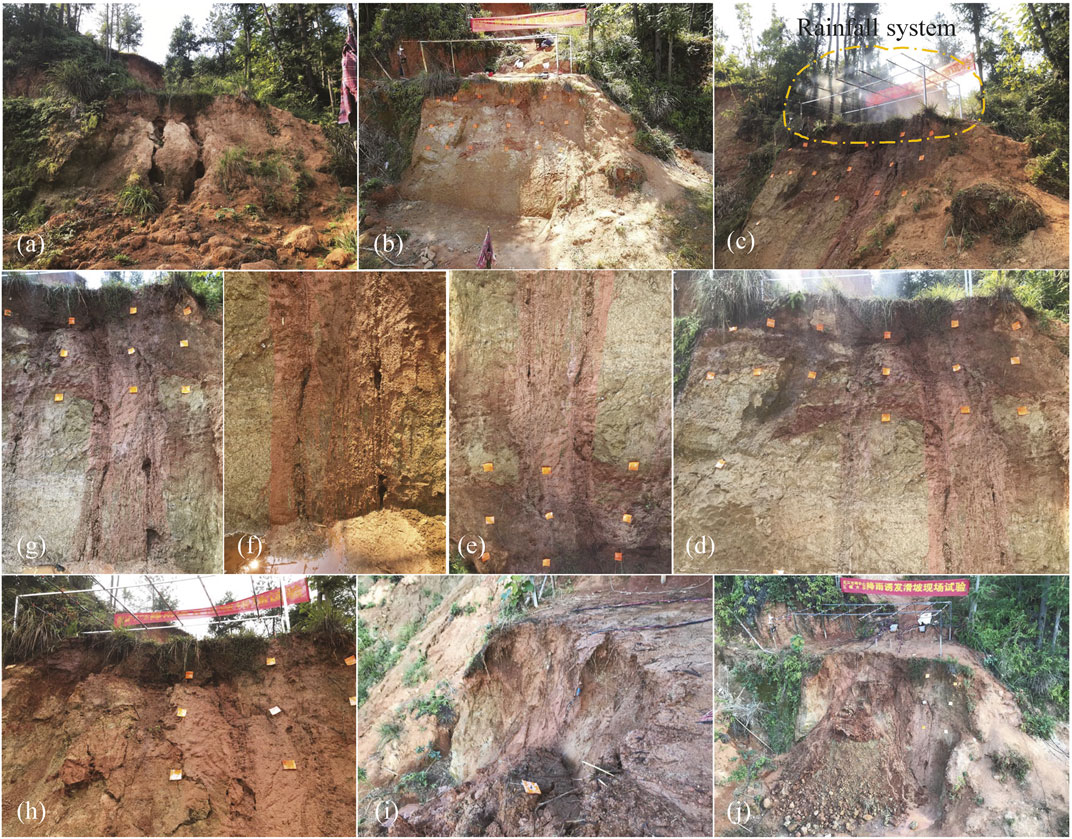
FIGURE 13. Evolution of slope deformation in the in-situ model test in Qixia Village, Ganzhou City: (A) Original slope. (B) Test slope after artificial cutting. (C) Rainfall simulation. (D) Runoff erosion on the slope surface. (E) Grooves on the slope surface, formed on the third day of continuous rainfall. (F) Grooves at the foot of the slope, formed on the third day of continuous rainfall. (G) Slope toe groove, enlarged on the fifth day of continuous rainfall. (H) Abrupt fissuring in the upper part of the soil profile on the 13th day of continuous rainfall. (I) Collapse of the upper slope on the 22nd day of continuous rainfall. (J) Slope collapse on the 23rd day of continuous rainfall.
Numerical Simulation
Based on on-site investigation, slope failure observations, geological engineering analogues, and the systematic analysis of the controls and occurrence of landslides, the following pattern of landslide distribution was identified in the Ganzhou City area: the granite slopes of Shuitou Village were prone to flow-collapse landslides; the metamorphic bedrock slopes adjacent to the highway in Xinwuxia Village were prone to landslides along a rock stratum; and the metamorphic bedrock slopes near the highway in Kongmu Village were prone to wedge-shaped block sliding and caving. The large-scale discrete element software MatDEM was used to simulate these types of slope failures. Software MatDEM is based on the principle of particle flow discrete element method, using the GPU matrix calculation method and three-dimensional contact algorithm, with high performance computing speed, to study the motion and interaction of granular materials. To ensure an accurate and effective simulation of the slope failure process, the parameters inputted in the discrete element model were the mechanical property parameters of strongly weathered soil, broken bedrock mass (slip mass), and bedrock on each actual slope measured through field sampling and laboratory tests (Table 2). The model used in the simulation test was a rock slope with a weak interlayer, a sliding body and bedrock. The sliding body was strongly weathered soil or broken rock. The mechanical parameters of the sliding body are listed in Table 3.
The construction of the model included the creation of a thin shell model 1), assignment of material properties 2), and setting of the soft surface of the sliding zone 3). After the initial model of the slope was established, a gravity load was applied to all elements, and the dynamic evolution of the landslide was simulated by iterative calculation.
As shown in Figure 14, the strongly weathered soil (sliding body) slid in an arc along the weak structural plane. A small number of units jumped and slid further down. Soil sliding generated damping, fracturing, and friction heat. The total heat was higher than that of the rock mass. The sliding time was short; therefore, the sliding distance to the accumulation zone was relatively long (approximately 20 m), and the mass of accumulated debris was large. In addition, there was a clear gradient of increasing soil displacement from the soil’s interior to the slope surface, which mirrored the gradient of soil weathering (increasing towards the surface). The deformation characteristics conformed to those of the flow-collapse landslides.
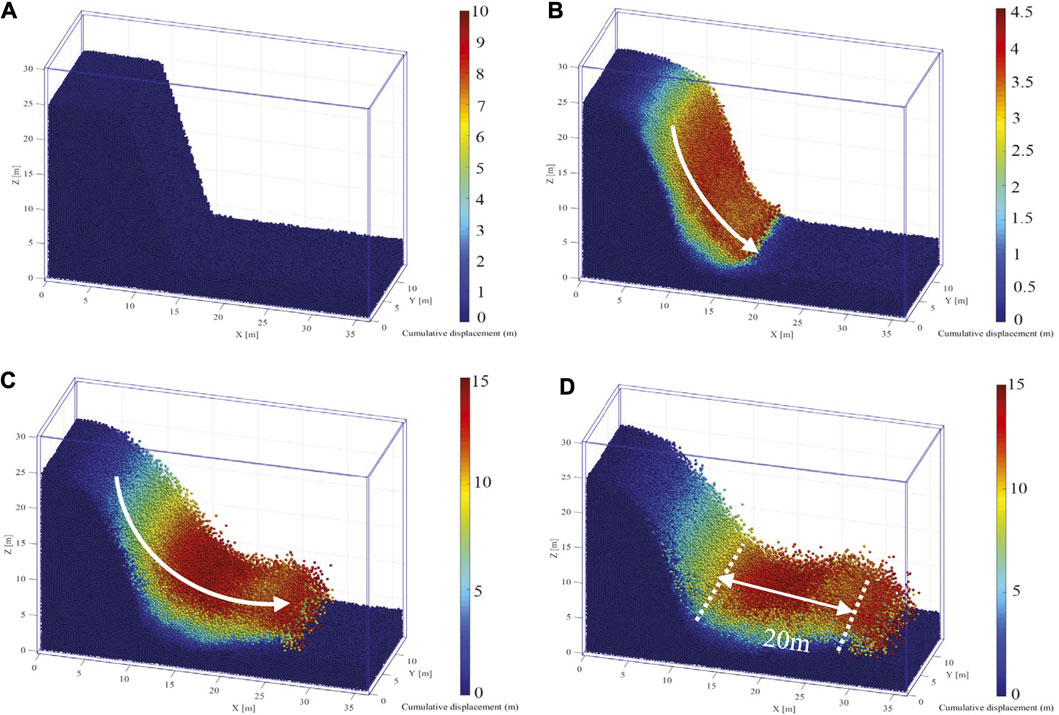
FIGURE 14. Deformation and failure evolution of the granite slope in Shuitou Village, Ganzhou City: (A) First stage; (B) Second stage; (C) Third stage; (D) Fourth stage.
In Figure 15, the fractured bedrock mass (sliding body) slid along a plane of weakness in the bedrock. Owing to the high fracture force and friction resistance in the rock mass unit, most of the energy was consumed during sliding. When the rock mass reached the bottom of the slope, the energy had dissipated, and the rock mass had accumulated approximately 5 m from the foot of the slope. The high speed of the sliding mass, however, resulted in a strong collision on the bottom of the slope, which caused a small number of units in the accumulation area to slide further forward. The deformation and failure characteristics of this simulated landslide conformed to those of rock sliding along a rock stratum.
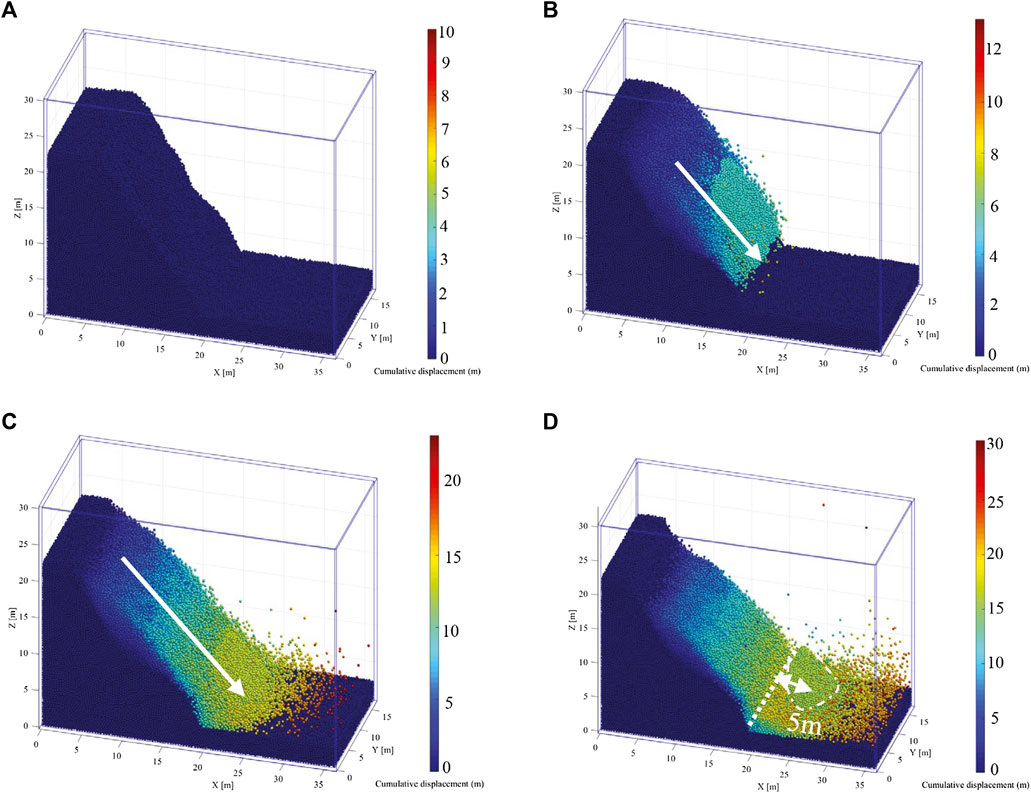
FIGURE 15. Deformation and failure evolution of the metamorphic bedrock slope on the Xinwuxia Village highway, Ganzhou City: (A) First stage; (B) Second stage; (C) Third stage; (D) Fourth stage.
In Figure 16, the wedge-shaped block cut in the slope face collapsed due to gravity along a structural plane of weakness. In the process of downward caving, friction and the degree of fracturing were low, resulting in low energy consumption. Most of the gravitational potential energy was converted into kinetic energy. Therefore, the collision with the rock mass at the bottom of the slope was the largest among the three simulations, causing many units to jump up or slide forward for approximately 15 m from the toe of the slope. The deformation, failure, and post-failure accumulation characteristics of this simulation conformed to those of wedge-shaped block sliding and caving.
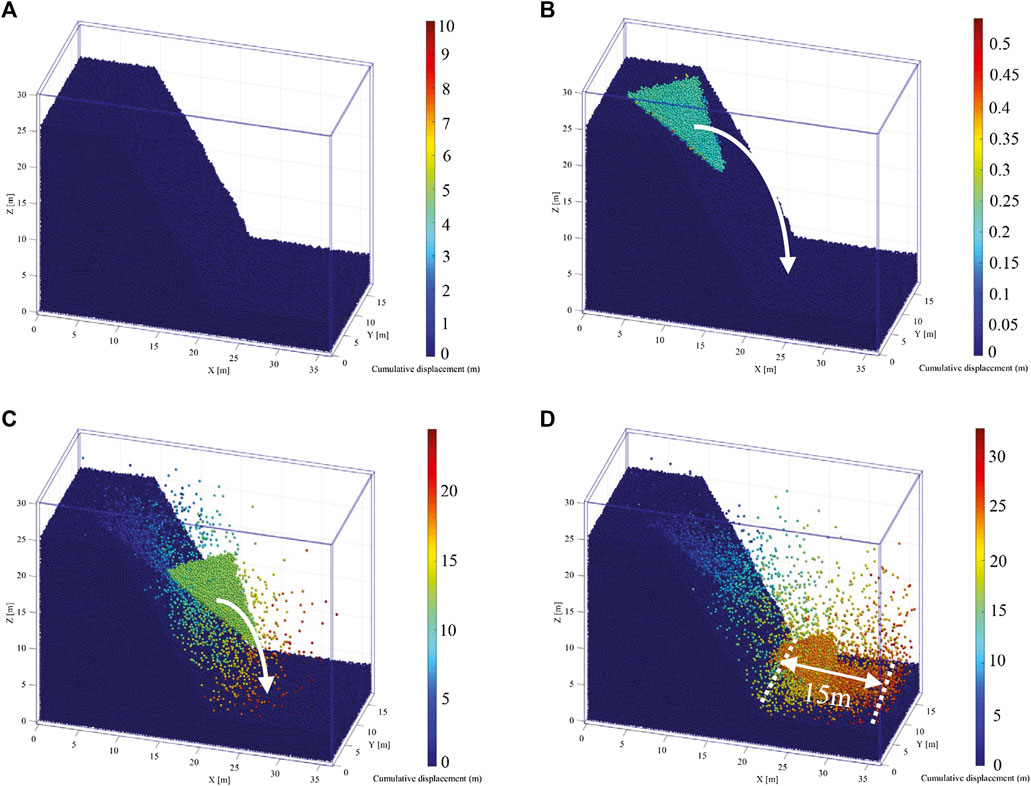
FIGURE 16. Deformation and failure evolution of the metamorphic bedrock slope on the Kongmu Village highway, Ganzhou City: (A) First stage; (B) Second stage; (C) Third stage; (D) Fourth stage.
As the above results show, collapse-flow sliding led to a wide disaster range and a large amount of accumulated soil after failure; sliding along a rock stratum led to a narrow disaster range and a small amount of accumulated soil and rock after failure; finally, wedge-shaped block sliding and caving occurred suddenly and at a high speed, leading to a wide disaster range, but the amount of soil and rock accumulated after failure was small.
Conclusion
Landslide geohazards in Ganzhou City, China, are sudden, widespread, and mainly small-scale. Many landslide sites are concealed by vegetation cover. Landslide geohazards are primarily distributed on the gentle (15° < α ≤ 35°) and moderately steep (35° < α ≤ 55°) slopes of the mountains and hills surrounding the city. More than 78% of all landslides that occurred between 1998 and 2019 were situated on metamorphic and granite bedrock. Structural faulting, especially the Dayu-Nancheng deep fault, exercises an obvious control of landslide geohazards. In addition to internal factors, such as topography, bedrock lithology, and geological structure, landslide geohazards are also influenced by external factors such as rainfall and the frequent cutting of slopes for the construction of houses and roads.
Based on the development, distribution, and influencing factors of landslide geohazards in Ganzhou City, granite, metamorphic (slate and phyllite), and clastic bedrock with weak interlayers were identified as the main bedrock lithologies prone to landslides. A large number of on-site landslide/slope-collapse surveys, the analysis of the mechanism of slope failure suggested that the main types of slope failure in Ganzhou include scouring erosion collapse, steep slope collapse, landslides along a rock stratum, and wedge-shaped block sliding and caving. Among these, scouring erosion slope collapse mainly develops on Jurassic granite with a thick wreathing mantle; steep slope collapse is widespread on steep slopes on granite and metamorphic bedrock, and landslides along a rock stratum and wedge-shaped block sliding and caving mainly occur on metamorphic bedrock with weak structural planes and clastic bedrock with weak interlayers.
In-situ model testing and numerical simulation were deployed to explore the deformation and failure of granite and metamorphic bedrock slopes under rainfall. The field model test reproduced the deformation and failure of small-scale collapse landslides, while the numerical simulation accurately simulated the deformation, failure, and post-failure accumulation of flow-collapse, landslides along a rock stratum, and wedge-shaped block sliding and caving. The results of this study provide a reference for the assessment of the risk from, and the management of, landslide geohazards in the Ganzhou City area and other, similar geological settings.
Data Availability Statement
The original contributions presented in the study are included in the article/Supplementary Material, further inquiries can be directed to the corresponding author.
Author Contributions
QZ is responsible for collating materials and writing the paper; SW provides funding; LW provides opinions on paper writing; FG and DZ participates in the field survey; JY participates in numerical calculations.
Funding
This work was supported by the China Geological Survey, Project DD20190716; 377 0001212020CC60002. QZ also sponsored by Research Fund for Excellent Dissertation of China Three Gorges University (2021BSPY006).
Conflict of Interests
Author DZ was employed by China Yangtze Ecology and Environment Co., Ltd.
The remaining authors declare that the research was conducted in the absence of any commercial or financial relationships that could be construed as a potential conflict of interest.
Publisher’s Note
All claims expressed in this article are solely those of the authors and do not necessarily represent those of their affiliated organizations, or those of the publisher, the editors and the reviewers. Any product that may be evaluated in this article, or claim that may be made by its manufacturer, is not guaranteed or endorsed by the publisher.
References
Cui, S., Pei, X., and Huang, R. (2018). Effects of geological and tectonic characteristics on the earthquake-triggered Daguangbao landslide, China. Landslides 15, 649–667. doi:10.1007/s10346-017-0899-3
Deng, Z., Liu, J., Guo, L., Li, J., Li, J., and Jia, Y. (2021). Pure risk premium rating of debris flows based on a dynamic run-out model: a case study in Anzhou, China. Nat. Hazards 106, 235–253. doi:10.1007/S11069-020-04459-X
Du, J., Yin, K., and Lacasse, S. (2013). Displacement prediction in colluvial landslides, Three Gorges Reservoir, China. Landslides 10, 203–218. doi:10.1007/s10346-012-0326-8
Gao, J., and Sang, Y. (2017). Identification and estimation of landslide-debris flow disaster risk in primary and middle school campuses in a mountainous area of Southwest China. Int. J. Disaster Risk Reduction 25, 60–71. doi:10.1016/j.ijdrr.2017.07.012
Gu, D. M., Huang, D., Yang, W. D., Zhu, J. L., and Fu, G. Y. (2017). Understanding the triggering mechanism and possible kinematic evolution of a reactivated landslide in the Three Gorges Reservoir. Landslides 14, 2073–2087. doi:10.1007/s10346-017-0845-4
Huang, F., Yin, K., He, T., and Zhou, C. (2016). Influencing factor analysis and displacement prediction in reservoir landslides− a case study of Three Gorges Reservoir (China). Tehnički vjesnik 23, 617–626. doi:10.17559/tv-20150314105216
Huang, F., Chen, J., Yao, C., Chang, Z., Jiang, Q., Li, S., et al. (2020b). Susle: A slope and seasonal rainfall-based rusle model for regional quantitative prediction of soil erosion. Bull. Eng. Geol. Environ. 79, 5213–5228. doi:10.1007/s10064-020-01886-9
Huang, R., Pei, X., Fan, X., Zhang, W., Li, S., and Li, B. (2012). The characteristics and failure mechanism of the largest landslide triggered by the Wenchuan earthquake, May 12, 2008, China. Landslides 9, 131–142. doi:10.1007/s10346-011-0276-6
Huang, X., Guo, F., Deng, M., Yi, W., and Huang, H. (2020a). Understanding the deformation mechanism and threshold reservoir level of the floating weight-reducing landslide in the Three Gorges Reservoir Area, China. Landslides 17, 2879–2894. doi:10.1007/s10346-020-01435-1
Jiang, Z., Lian, Y., and Qin, X. (2014). Rocky desertification in Southwest China: impacts, causes, and restoration. Earth-Science Rev. 132, 1–12. doi:10.1016/j.earscirev.2014.01.005
Ke, P., Peng, H. X., and Liang, F., (2017). Distribution characteristics and development environment of geological disasters in Ganzhou City. Saf. Environ. Eng. 24, 33–39. (in Chinese). doi:10.1002/nla.2096
Leng, Y., Peng, J., Wang, Q., Meng, Z., and Huang, W. (2018). A fluidized landslide occurred in the Loess Plateau: a study on loess landslide in South Jingyang tableland. Eng. Geology. 236, 129–136. doi:10.1016/j.enggeo.2017.05.006
Ouyang, C., Zhou, K., Xu, Q., Yin, J., Peng, D., Wang, D., et al. (2017). Dynamic analysis and numerical modeling of the 2015 catastrophic landslide of the construction waste landfill at Guangming, Shenzhen, China. Landslides 14, 705–718. doi:10.1007/s10346-016-0764-9
Shen, D., Shi, Z., Peng, M., Zhang, L., and Zhu, Y. (2021). Preliminary analysis of a rainfall-induced landslide hazard chain in Enshi City, Hubei Province, China in July 2020. Landslides 18, 509–512. doi:10.1007/s10346-020-01553-w
Wang, H. B., Zhou, B., Wu, S. R., Shi, J. S., and Li, B. (2011). Characteristic analysis of large-scale loess landslides: a case study in Baoji City of Loess Plateau of Northwest China. Nat. Hazards Earth Syst. Sci. 11, 1829–1837. doi:10.5194/nhess-11-1829-2011
Wang, S., Pan, Y., Li, W., and Guo, F. (2021). Deformation characteristics, mechanisms, and influencing factors of hydrodynamic pressure landslides in the Three Gorges Reservoir: a case study and model test study. Bull. Eng. Geol. Environ. 80, 3513–3533. doi:10.1007/S10064-021-02120-W
Xu, X.-Z., Guo, W.-Z., Liu, Y.-K., Ma, J.-Z., Wang, W.-L., Zhang, H.-W., et al. (2017). Landslides on the Loess Plateau of China: a latest statistics together with a close look. Nat. Hazards 86, 1393–1403. doi:10.1007/s11069-016-2738-6
Yin, Y.-p., Huang, B., Chen, X., Liu, G., and Wang, S. (2015). Numerical analysis on wave generated by the Qianjiangping landslide in Three Gorges Reservoir, China. Landslides 12, 355–364. doi:10.1007/s10346-015-0564-7
Youssef, A. M., and Maerz, N. H. (2013). Overview of some geological hazards in the Saudi Arabia. Environ. Earth Sci. 70, 3115–3130. doi:10.1007/s12665-013-2373-4
Zhang, Y., Hu, X., Tannant, D. D., Zhang, G., and Tan, F. (2018). Field monitoring and deformation characteristics of a landslide with piles in the Three Gorges Reservoir area. Landslides 15, 581–592. doi:10.1007/s10346-018-0945-9
Keywords: geohazards, landslides, landslide-prone rocks, slope failure mode, in-situ model test, numerical simulation
Citation: Zhan Q, Wang S, Wang L, Guo F, Zhao D and Yan J (2021) Analysis of Failure Models and Deformation Evolution Process of Geological Hazards in Ganzhou City, China. Front. Earth Sci. 9:731447. doi: 10.3389/feart.2021.731447
Received: 27 June 2021; Accepted: 10 August 2021;
Published: 20 August 2021.
Edited by:
Faming Huang, Nanchang University, ChinaReviewed by:
Guang-Liang Feng, Institute of Rock and Soil Mechanics (CAS), ChinaMing Zhang, China University of Geosciences Wuhan, China
Xiulei Li, Chongqing Jiaotong University, China
Copyright © 2021 Zhan, Wang, Wang, Guo, Zhao and Yan. This is an open-access article distributed under the terms of the Creative Commons Attribution License (CC BY). The use, distribution or reproduction in other forums is permitted, provided the original author(s) and the copyright owner(s) are credited and that the original publication in this journal is cited, in accordance with accepted academic practice. No use, distribution or reproduction is permitted which does not comply with these terms.
*Correspondence: Li Wang, d2FuZ2xpX2N0Z3VAMTI2LmNvbQ==