- 1Key Laboratory of Poyang Lake Wetland and Watershed Research Ministry of Education, School of Geography and Environment, Jiangxi Normal University, Nanchang, China
- 2State Key Laboratory of Lake Science and Environment, Nanjing Institute of Geography and Limnology, Chinese Academy of Sciences, Nanjing, China
- 3Department of Geosciences, Faculty of Land Resource Engineering, Kunming Univerity of Science and Technology, Kunming, China
- 4Geological Exploration Technology Institute of Jiangsu Province, Nanjing, China
Lake carbon burial is of vital significance in global carbon cycle and carbon budget, particularly in the large deepwater lakes. However, carbon burial in large deepwater lakes is hard to estimate due to the difficulty in obtaining high spatial-resolution samples. In this study, we investigated distributions of total organic carbon (TOC) and inorganic carbon (TIC), two main carbon components in lake sediments, based on dozens of surficial sedimentary samples (n = 26) covering whole Lake Qinghai, the largest saline lake in China. The results showed that the TOC content, with a range of 1.4–4.8%, was significantly higher in the lake area near the northern lakeshore where human activities are concentrated and lower in the lake areas near the Buha River mouth and the eastern lake area. In contrast, the TIC content, ranging from 1.5 to 3.8%, increased from the northwestern and southeastern lake areas toward the lake center, and mainly depended on hydro-chemical and hydraulic characteristics. The inorganic carbon burial (47.77 ± 19.73 Gg C yr−1) was approximately equal to organic carbon burial (47.50 ± 22.68 Gg C yr−1) and accounted for about 50% of the total carbon burial (95.27 ± 37.74 Gg C yr−1), suggesting that saline lakes constitute a large inorganic carbon pool in addition to an organic carbon pool. Because of saline water body type in arid and semiarid regions and alpine Qinghai–Tibet Plateau, lakes in these regions have huge inorganic carbon burial potential and important contributions to the global carbon budget.
Introduction
Because of the rich organism community and/or lots of carbonate precipitation as well as the ideal burial environment, lake sediments have a great burial capacity of atmospheric carbon (Einsele et al., 2001). Organic carbon (OC) burial was estimated to be 1.25 Mt yr−1 in European lakes (Kastowski et al., 2011) and ∼12.6 Tg C yr−1 in lakes/ponds of the conterminous United States (Clow et al., 2015). It was concluded that organic carbon accumulation in global lakes displayed a rate (42 Tg yr−1) that approached half of that in the ocean (100 Tg yr−1) (Dean and Gorham, 1998). Therefore, lakes play an important role in the carbon cycle and budget and are given considerable official and scientific concerns.
Carbon in lake sediments mainly comprises OC and inorganic carbon (IC). The former stems from terrestrial organism residues derived from watershed and aquatic organism residues derived from lake (Meyers and Ishiwatari, 1993), while the latter consists of lithogenic carbonate inputted by runoff, wind, and endogenic carbonate (including biogenic carbonate) (Anas et al., 2015). Little attention, however, was paid to IC burial in lake sediments. In fact, the inorganic carbon content in sediments of many hydrologically closed temperate lakes was comparable to or even higher than that of organic carbon (Einsele et al., 2001; Gyawali et al., 2019; Chen et al., 2021). Particularly, inorganic carbon could be an essential component in carbon burial in those hydrologically closed lakes.
Previous studies on carbon burial in large lakes mainly focused on several specific sites and lack high-resolution systematic investigation. However, spatial patterns of organic and inorganic carbon in sediments of large deepwater lake and related controlling factors remain complicated and rarely studied on account of the higher cost of high spatial-resolution sampling. Since sedimentary organic and inorganic carbon tend to be susceptible to plenty of drivers, such as carbon sources, climate, sedimentary environments, and human activities (i.e. land use and nutrient supply) (Tenzer et al., 1997; Rumolo et al., 2011; Yu et al., 2015; He et al., 2021), estimation of carbon burial in a large lake based merely on several sites is questionable. High-resolution sampling in a large lake is advantageous to eliminate the inaccuracy of several sites and identify the impacts of human activities and natural conditions on carbon as an implication for environmental restoration and construction surrounding the lake.
Lake Qinghai is located in the northeastern Tibetan Plateau and is the largest saline lake in China. It has been endowed with an enormous scientific and social value for its unique location and high climatic sensitivity (Qin and Huang, 1998; Ji et al., 2005; Henderson et al., 2007; An et al., 2012). Both organic and inorganic carbon contents in sediments were considered to be indicators of environmental and climatic changes, and thus were used for paleoclimatic reconstruction on different timescales (Yu and Kelts, 2002; An et al., 2012; Liu et al., 2014b). In the context of global warming and intensified human activity, as a geographical and paleoclimatic archive at the third pole, the carbon sequestration capacity of Lake Qinghai also needs to be evaluated based on a systematic investigation of the carbon content. For Lake Qinghai, Einsele et al. (2001) and Xu et al. (2013) evaluated carbon burial of its Holocene sediments and modern sediments, respectively. However, the distribution of the sedimentation rate (Xu et al., 2010), eutrophic elements (Chen et al., 2012), and the modern sedimentation process (Shang et al., 2009) in Lake Qinghai surficial sediments showed significant spatial variability. Similarly, to a certain degree, as human-induced changes occur manifestly around and in the lake (Zhang et al., 2013; Luo et al., 2017; Lian et al., 2019), carbon in the sediments of Lake Qinghai could also exhibit spatial heterogeneity due to non-anthropogenic and anthropogenic factors, which is currently unknown.
Here, high-resolution surficial sediments (n = 26) uniformly covering the whole Lake Qinghai were collected. Based on total organic carbon (TOC), total inorganic carbon (TIC), total nitrogen content (TN), organic matter C/N atomic ratio, mineralogical methods, and regional socioeconomic statistics, this study aims to 1) explore the source of OC and IC in Lake Qinghai sediments, 2) investigate the contributions of natural and anthropogenic factors to the spatial variation of TOC and TIC across the lake basin, and 3) further estimate the carbon burial in Lake Qinghai.
Materials and Methods
Lake Qinghai (99˚36´-100˚47´E, 36˚32´-37˚15´N, ∼3,193.8 m a s l.) (Figure 1), located in the temperate continental climate and arid/semiarid region in the northeastern Tibetan Plateau, is the largest saline lake in China (lake size: ∼4,400 km2, average water depth: ∼21 m, maximum depth of 31.4 m) (Liu et al., 2003; Dong et al., 2018). The mean annual air temperature and precipitation are 1.2 °C and 336.6 mm, respectively (Wang et al., 1998). Strong westerlies and/or northwesterly winds prevail over Lake Qinghai during all seasons, except summer (Dong et al., 2018). The Buha River, Shaliu River, Haergai River, Quanji River, and Heima River are the main tributaries of the lake; of which, the Buha River on the west is the largest with a runoff contribution of ∼46.9% (Chang et al., 2017). Catchment zonal soil mainly consists of chestnut soil; the bedrock of the lake’s surrounding is dominated by metamorphic rocks and acidic magmatic rocks, and there is limited limestone in the west (Xu et al., 2013). Main vegetation comprises temperate steppe, shrub, alpine grassland, and meadow (Shang et al., 2009).
As an important tourist attraction and one of the national nature reserves, Lake Qinghai is significantly affected by humans with environmental damage or/and ecological construction in recent years. There are four counties in the Lake Qinghai Basin: Tianjun County where the Buha River flows through, Gonghe County in the area south of Lake Qinghai, Gangcha County near to the Shaliu River, and Haiyan County in the area north of the lake where industrial and agricultural activities are concentrated. The Lake Qinghai Basin is an important livestock base, within which there are five large state-owned farms and ranches. Tourism around the lake is under rapid development. Besides, various ecosystem protection and construction projects covering the Lake Qinghai Basin are being implemented, such as degraded grassland management projects, desertification land control projects, and wetland protection projects.
Twenty-six surficial sediment samples (top of 1 cm) covering the whole lake were altogether collected from Lake Qinghai with Kajak gravity corer in September 2017, and 3 surficial soil and 6 fluvial sediment samples from the drainage area were sampled in September 2019 (Figure 1 and Table 1). We measured the volume of each sample before lyophilization and weighed each dry sample after lyophilization for 26 lake sediments.
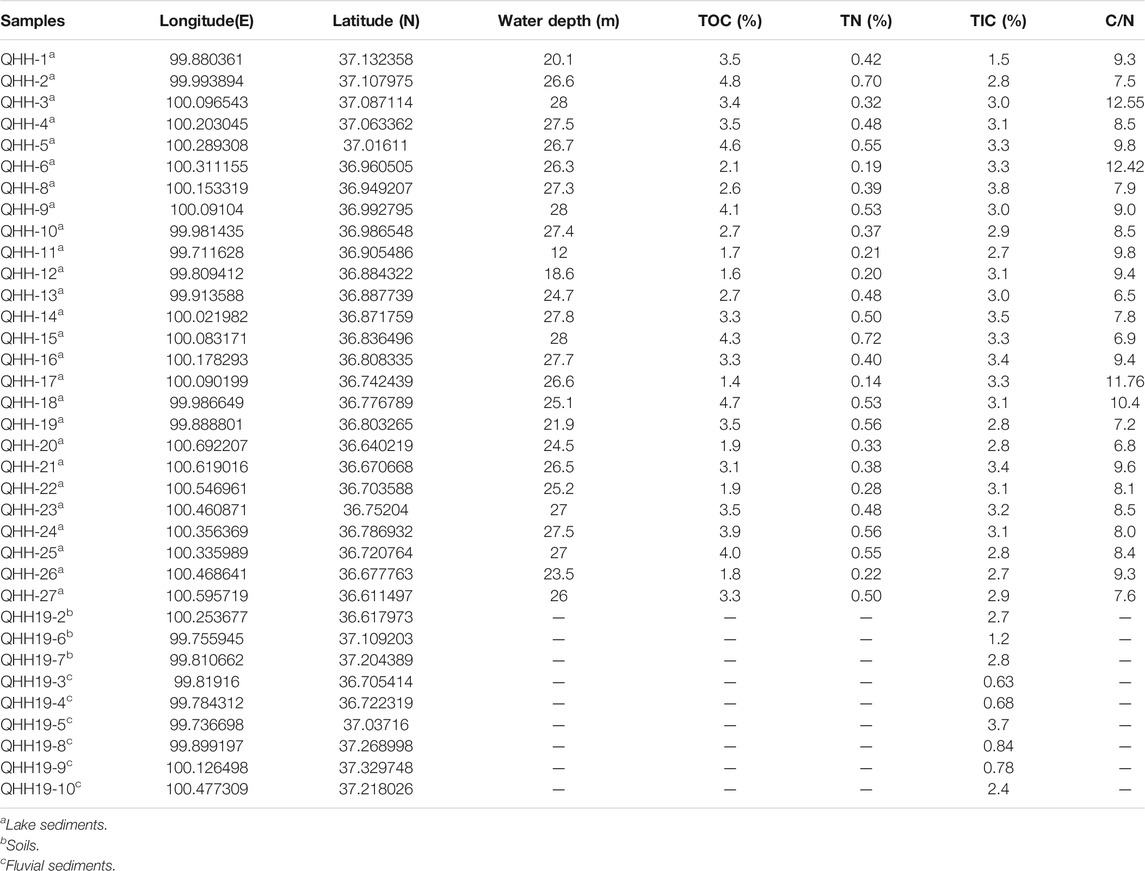
TABLE 1. Location, water depth, total organic carbon content (TOC), total nitrogen content (TN), total inorganic carbon content (TIC), and atomic C/N ratio of surficial samples.
Total organic carbon, total nitrogen content, total inorganic carbon, and mineral compositions in collected samples were measured. For TOC and TN measurements, 0.5 g of each sample was weighed into a 50 ml centrifuge tube, pretreated with HCl (10%) and heated in a water bath (60°C) for 3 h, rested for 12 h to remove the carbonates, and then rinsed with distilled water repeatedly after 3–4 times centrifugation (5,000 r/min). Finally, samples were freeze-dried and ground, and measured on the EA 3000 elemental analysis instrument at the State Key Laboratory of Lakes and Environment, Nanjing Institute of Geography and Limnology, Chinese Academy of Sciences. Measured TOC was the organic carbon content of the carbonate-free sample. Thus, the TOC content in the bulk sample should be calibrated as TOCmeasured × (1- carbonate content).
For TIC measurement, Fourier transform infrared spectrometry (FTIR) was used to quantify the carbonate content of each sample following the specific steps of Ji et al. (2009) and Meng et al. (2015) because this technique has high precision, small measurement error, simply pretreatment, and high measurement efficiency (Ji et al., 2009). Samples were ground and dried at 70 °C and placed in a sample cup for analyzing in a Thermo Nicolet 6700 FTIR with a diffuse reflectance attachment from wavenumbers 4000–400 cm−1 at Nanjing University. The absorption peaks at 2,513–2,522 cm−1 representing total carbonate was measured for its reflectance band area (Figure 2A). The quantitative equation of the total carbonate content, namely, y = 0.000181x+0.0237 (y: total carbonate content, x: band area at 2,513 cm−1; R2 = 0.988, RMSE = 0.0197), was constructed by adding a certain amount of pure carbonate into the carbonate-free matrix that was made by thoroughly removing the carbonate of Lake Qinghai sediments using HCl. The detection limit of carbonate in sediments was 0.1%, and the measuring error of this method was lower than 2% (Meng et al. (2015). Concerning the content of authigenic carbonate relative to the total carbonate content (TCC), we decided to use the calculation results of Xu et al. (2010) that authigenic carbonates accounted for approximately 95% of the total carbonates in Lake Qinghai sediments. Therefore, the TIC content of Lake Qinghai sediments could be estimated with TCC×95% × 12/100. To further determine mineralogical types of carbonates in Lake Qinghai sediments, surrounding soils, and fluvial sediments, the X-ray diffraction analysis (XRD) was also performed on selected representative samples with the Panalytical multifunctional X-ray powder diffractometer at the State Key Laboratory of Lakes and Environment, Nanjing Institute of Geography and Limnology, Chinese Academy of Sciences.
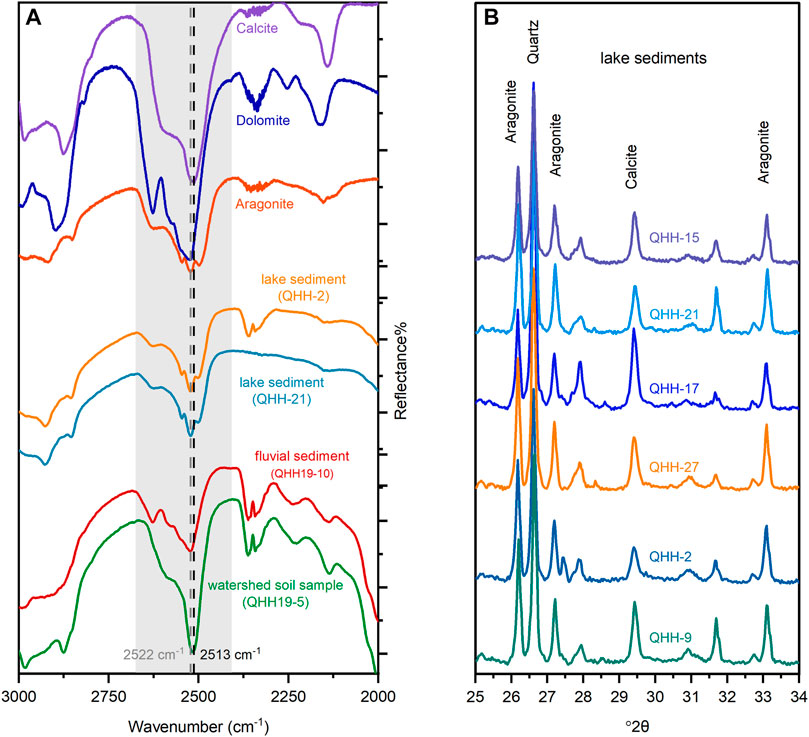
FIGURE 2. FTIR and XRD analyses on Lake Qinghai sediments and watershed fluvial sediments. (A) FTIR spectrum of pure standard calcite, dolomite, aragonite mineral, of samples in the lake sediments (QHH-2 and QHH-21), and of samples in the watershed fluvial sediments (QHH19-10 and QHH19-5). Lake sediments have similar FTIR spectrum features with pure aragonite rather than calcite and dolomite, while watershed samples show a FTIR spectrum feature close to calcite or dolomite rather than aragonite. (B) XRD results of lake sediments (QHH-2, QHH-9, QHH-15, QHH-17, QHH-21, and QHH-27) also indicate that aragonite is the main component of carbonate mineral and followed by calcite.
The organic carbon burial rate (OCBR) and the inorganic carbon burial rate (ICBR) were calculated based on TOC, TIC, sedimentation rate (SR), and dry bulk density (DBD), according to Dean and Gorham. (1998):
where Md = weight of dry sample and V = volume of wet bulk sample (measured before lyophilization). Sedimentation rates of 26 sites were roughly evaluated by Kriging interpolation with the sedimentation rates reported in the study by Xu et al. (2010). As an alpine deepwater lake, previous studies found that Lake Qinghai has stable sedimentary environment in each site, showing a relatively low and stable modern deposition rate in recent decades (Zhang et al., 2009; Fu et al., 2015). Modern SR data of Lake Qinghai reported by Xu et al. (2010) were derived from 11 cores covering the whole Lake Qinghai. Therefore, we believe the SR data of Xu et al. (2010) were relatively reliable.
Results
The distribution of TOC showed great spatial difference in Lake Qinghai surficial sediments ranging from 1.4 to 4.8% (with average of 3.1%, CV = 0.32) (Figure 3A and Table 1). The western and eastern lake areas had a low TOC value (mean value of 2.4%), while a high content (mean value of 3.7%) occurred in the northern and central areas. (Figure 3A). Specifically, there were eight prominent low-content sites (i.e., QHH-11 (1.7%) and QHH-12 (1.6%) near the Buha river mouth, QHH-17 (1.4%) in the southern lake area, and QHH-20 (1.9%), QHH-22 (1.9%), and QHH-26 (1.8%) in the eastern lake area) and seven relative higher content sites (i.e., QHH-2 (4.8%), QHH-5 (4.6%), QHH-9 (4.1%), QHH-15 (4.3%), QHH-18 (4.7%), QHH-24 (3.9%), and QHH-25 (4.0%)) (Table 1). TN ranged in concentration from 0.14 to 0.72% (Table 1). Atomic C/N ratios varied but were all between 6.5 and 12.5 (mean 8.9) (Table 1; Figure 4A).
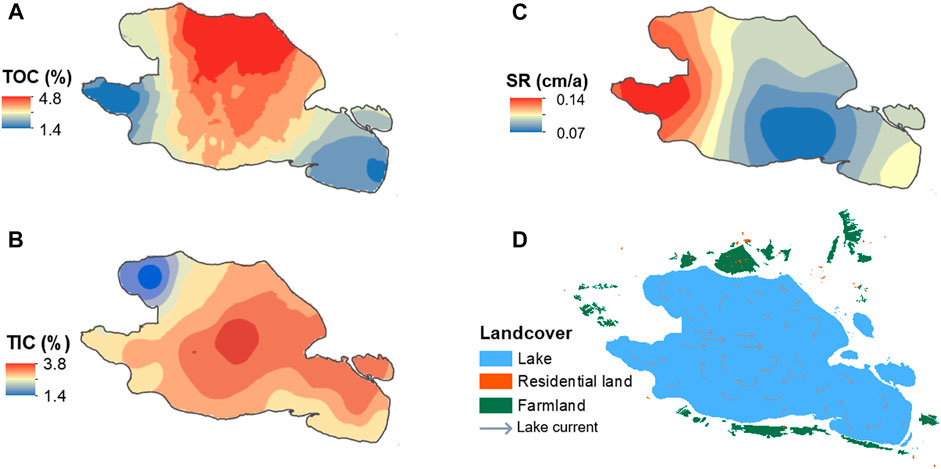
FIGURE 3. Spatial distribution of (A) TOC, (B) TIC, (C) sediment accumulation rate (Xu et al., 2010), and (D) land use around Lake Qinghai in 2000 modified from Liu et al. (2014a) and lake current characteristics modified from Han et al. (2016).
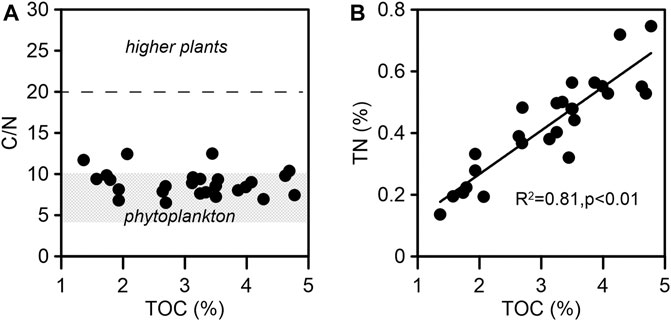
FIGURE 4. (A) Plot between C/N and TOC. Typical C/N ratio ranges for higher plants and phytoplankton referred to the study by Hedges and Oades. (1997) and Talbot and Lærdal. (2000) were labeled. (B) Relationship between total nitrogen (TN) and TOC.
The range of TIC content was 1.5–3.8% in Lake Qinghai sediments (3.0% on average, CV = 0.14) (Table 1). The spatial distribution of TIC content is shown in Figure 3B. There was a general increasing trend of TIC content from the northwestern and southeastern lake areas to the central lake area, with the lowest value (1.5%) near the northwestern shore of the lake (QHH-1) and the highest in the central QHH-8 (3.8%) (Figure 3B and Table 1).
Discussion
Source of Organic and Inorganic Carbon
The carbon source in lake sediments is related to the budget of carbon burial. There was a strong linear relationship between TOC and TN (R2 = 0.81, p < 0.01, Figure 4B), demonstrating that organic nitrogen was the dominant form of total nitrogen (Schubert and Calvert, 2001; Liu et al., 2010). The organic matter C/N atomic ratio is commonly used to qualitatively distinguish the contribution of terrestrial vegetation and aquatic organisms to organic carbon in lake sediments. Terrestrial vascular plants are poor in nitrogen, so the C/N ratio is high, while aquatic plants are nitrogen rich with a low C/N ratio (Meyers and Lallier-vergés, 1999; Müller and Mathesius, 1999). It was suggested that the C/N ratio of phytoplankton is less than 10 (Hedges and Oades, 1997). C/N ratios greater than 20 were believed to denote terrestrial sources of organic matter, while values in 10–20 were considered to be signals of a mixture of aquatic plants and higher plants (Talbot and Johannessen, 1992; Tyson, 1995; Talbot and Lærdal, 2000). The C/N atomic ratios of Lake Qinghai surficial sediments ranged from 6.5 to 12.5, with values of 22 samples below 10 (Table1; Figure 4A). Therefore, organic matter in Lake Qinghai sediments may be mainly of endogenous origin. The investigation of organic carbon isotopic compositions of long-chain n-alkanes of modern sediments and aquatic plants also revealed that the organic matter in Lake Qinghai sediments was mainly derived from the endogenous aquatic organisms (Liu et al., 2015). In addition, the preservation of organic carbon in sediments is an important aspect of OC burial. A recent study found that the contribution of TOC degradation to dissolved inorganic carbon was less than 10% in Lake Qinghai (Sun et al., 2019). This may result from the stratification of lake water and the permanently anoxic bottom environment (Sobek et al., 2009). Therefore, TOC in Lake Qinghai was mainly derived from aquatic organisms and well preserved in sediments.
Mineralogical and geochemical methods were used to determine the source and composition of TIC (carbonate) in Lake Qinghai sediments. Among the carbonate mineral types, aragonite is unstable so it hardly exists in ancient bedrock (Kunzler and Goodell, 1970; Martín-García et al., 2019), which is consistent with investigation of modern sediments around the Lake Qinghai Basin (Meng et al., 2019). Thus, aragonite in lake sediments should be autogenetic that precipitated from lake water or/and derived from biogenic origin; this was also supported by the result that no aragonite was found in the basin soil and fluvial sediments (Figure 2). The mineralogical measurement using both FTIR and XRD found that aragonite dominated the carbonate mineral in Lake Qinghai sediments. Within FTIR spectra, the absorption peak shapes of fluvial sediments and topsoil samples are similar to those of pure calcite, and the characteristic peak position is at ca. 2,513 cm−1 (Figure 2A), whereas the shapes of lacustrine sediments are similar to those of pure aragonite, and the characteristic peak position is at ca. 2,522 cm−1 (Figure 2A). The XRD results (Figure 2B) further supported the judgment that aragonite (d104 = 3.39) was the dominant component of carbonate in Lake Qinghai surficial sediments, which was consistent with the results from the study by Yu and Kelts. (2002). In addition, the δ18O values of carbonate in Lake Qinghai sediments were more positive than those in the surrounding topsoil and were close to equilibrium δ18O values of lake water (Liu et al., 2018), which also supported the mainly authigenic origin of carbonate in the lake. The evidence demonstrated the rationalization of the assumption selected in this study that authigenic carbonates accounted for approximately 95% of the total carbonates in Lake Qinghai surface sediments based on estimation of Xu et al. (2010).
Spatial Variation of Organic and Inorganic Carbon
TOC and TIC contents in Lake Qinghai had different spatial patterns. TOC content was the highest in the northern lake area and declined outward, with a prominent low value in the shallow part facing the Buha River mouth and the eastern lake area (Figure 3A). The spatial variation of TOC was associated with different driving forces. High TOC content in the northern lake area was corresponding to a relatively lower sedimentation rate and intense human activities in the north bank, along which farmland and residential land concentrates (Figures 3C,D). The sedimentation rate in the northern lake area was relatively lower despite the three rivers’ inflow (Figure 1, Figure 3C). The limited dilution effect of terrestrial detrital materials was conducive to the accumulation of organic carbon. In addition, anthropogenic nutrient loading by fertilizer use and domestic wastewater increased the trophic level of the northern lake area, generating such high level of TOC concentration in this part.
The low TOC content at the shallow part near the Buha River mouth (water depth: 10–18 m, Figure 1 and Table 1) was the result of the high sedimentation rate and low fine-grain proportion. The high sedimentation rate in the Buha River mouth (Figure 3C) reflected a wealth of terrestrial debris input diluting sedimentary organic carbon concentration (Xu et al., 2010). Besides, a good correlation was found between organic matter concentration and the surface area of grains in sediment (Mayer, 1994; Hedges and Keil, 1995) because fine-grained components are effective to organic carbon preservation and are easily resuspended and migrated away from shallow water to the deep water zone (Thompson and Eglinton, 1978; Tenzer et al., 1997). The proportion of coarse particles in the littoral zone and estuary areas is greater than that of coarse particles in the deep lake zone due to continually discharged terrestrial clastic minerals (Håkanson and Jansson, 2002). Therefore, there was quite a low TOC content in the area near the Buha River mouth. For the eastern lake area with a low TOC content, there was a considerable area of sand and bare land in the east bank with no river flowing in (Figure 1). Rare human settlements appeared there, resulting in no point source pollution. These possibly resulted jointly in a lower TOC content. In summary, the distributions of TOC in Lake Qinghai were mainly related to sedimentation rates, human activities, and the grain size.
In contrast to TOC, the TIC content displayed a pattern that approximated concentric circles rising from the lakeshore to the center (Figure 3B). Supersaturation of carbonate in lake water determined TIC deposition in Lake Qinghai (Liu et al., 2003). However, inflow rivers are unevenly distributed (Figure 1). The lake area accepting riverine fresh water may experience salinity reduction and a decrease in carbonate saturation, hence a diminishment of carbonate deposition and TIC content in the sediment. Quite the opposite, in the central part of the lake where there is shortage of freshwater replenishment, evaporation has more intensive influence on the lake water, which may cause relatively more carbonate precipitation (Shen et al., 2001).
On the other hand, the prevailing northwest wind-induced overall eastward and clockwise water currents (Figure 3D) (Han et al., 2016). Sediment focusing related to waves and currents may redistribute carbonate minerals to the central and deep basins (Håkanson and Jansson, 2002; Terasmaa and Punning, 2006). This can be reflected by the significantly low TIC content in the upwind northwestern lake area (Figure 3B). Therefore, TIC distribution in Lake Qinghai can be mainly attributed to the hydro-chemical characteristics of its lake water and hydraulic conditions controlled by prevailing northwest wind.
Total C Burial Estimation in Lake Qinghai Surficial Sediments
In order to investigate how much carbon was sequestered in Lake Qinghai in recent years, total organic and inorganic carbon burial were estimated by multiplying carbon burial rates with the lake area. Consequently, OCBRs ranged from 4.54 to 30.50 (average value of 10.80) g C m−2 yr−1, while ICBRs were between 3.32–24.46 (mean value of 10.86) g C m−2 yr−1. Correspondingly, total OC burial was 47.50 ± 22.68 Gg C yr−1 and IC burial was 47.77 ± 19.73 Gg C yr−1. Thus, total annual carbon burial in Lake Qinghai was concluded to be 95.27 ± 37.74 Gg C yr−1, which is slightly higher than 76 Gg C yr−1 as reported in the study by Xu et al. (2013), which is estimated based on samples mainly derived from central and eastern Lake Qinghai. On the one hand, at least for the last 100–200 years, contemporary lake C burial was likely to be higher than that of the historical value (Dietz et al., 2015; Heathcote et al., 2015; Zhang et al., 2019). On the other hand, the difference in carbon burial estimation for this large lake might be made possible by sampling at different sites. In this study, estimated from OCBRs and ICBRs of each sedimentary sample at 26 sites, total OC and IC burial were vastly different with coefficients of variation of 0.48 and 0.41, respectively. To prevent over/underestimation, it is necessary to consider the spatial heterogeneity while assessing the total C burial of a whole lake based merely on a single sample or sedimentary core, even if sampling in deepwater areas with a stable sedimentary environment of a lake.
The OCBR of Lake Qinghai (14.7 g C m−2 yr−1) was in line with the global mean value of 10–15 g m−2 yr−1 (Tranvik et al., 2009) and close to the average level in the Tibet Plateau Lake Region (14.3 g m−2 yr−1) (Zhang et al., 2017), but it was lower than that of other lake regions in China (Zhang et al., 2017). To be specific, for the last 150 years, the mean OCBR of lakes was 25.4 g m−2 yr−1 in the Northeast Mountain and Plain Lake Region, 30.6 g m−2 yr−1 in the Eastern Plain Lake Region, 30.4 g m−2 yr−1 in the Inner Mongolian-Xinjiang Lake Region, and 24.3 g m−2 yr−1 in the Yunnan-Guizhou Plateau Lake Region (Zhang et al., 2017). A possible reason of lower OCBRs in Lake Qinghai may be the lower trophic level and primary productivity, owing to a lower temperature and less anthropogenic pollution (Wang et al., 1998; Bi et al., 2018). The oligotrophic Lake Alchichica, Mexico, with similar hypolimnetic anoxia conducive to organic carbon preservation, had OCBRs (14.9 g m−2 yr−1) (Alcocer et al., 201 4) comparable to those of Lake Qinghai. Taken equally, lakes of the high northern latitudes had low OCBRs due to frigid climatic patterns and scarce anthropogenic impacts. For example, OCBRs ranged 1–10 g m−2 yr−1 for SW Greenland lakes (Anderson et al., 2019) and from 5.3 to 24.6 g m−2 yr−1 for Arctic Sweden (Lundin et al., 2015).
Lake Qinghai had obviously lower OCBRs than many eutrophic lakes, such as Lake Greifen in Switzerland (50–60 g C m−2 yr−1) (Hollander et al., 1992), Rostherne Mere (96.10 g C m−2 yr−1), and Tatton Mere (62.51 g C m−2 yr−1) in the United Kingdom (Scott, 2014). In Minnesota, the United States, OCBRs in ∼89% of agriculturally affected lakes were above 50 g C m−2 yr−1 (Anderson et al., 2013). Notably, the eutrophic Lake Chaohu that has undergone heavy pollution had a lower OCBR of 10.01 g m−2 yr−1 than Lake Qinghai (Wu et al., 2016) (Figure 5). The exorbitant nutrient levels even led to a decreased carbon sequestration capacity of Lake Chaohu. It was deduced that this owed much to the degradation and decomposition of macrophytes when the lake switched to be cyanobacteria-dominated (Wu et al., 2016).
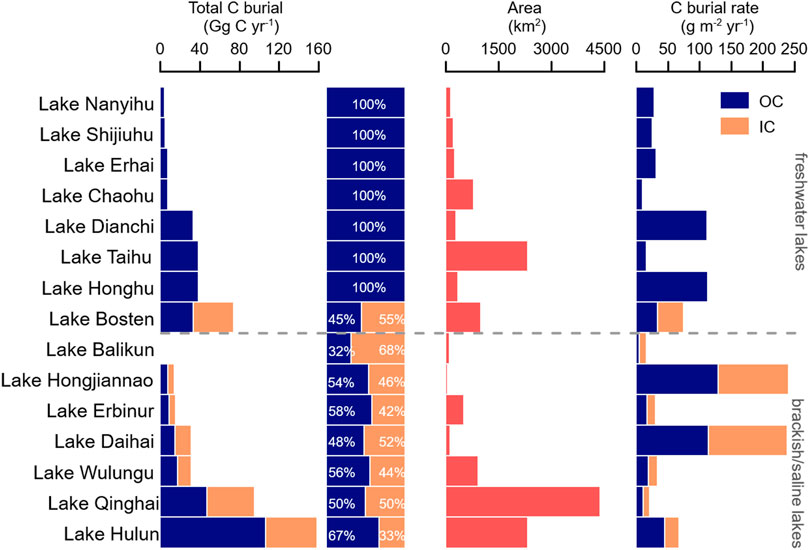
FIGURE 5. Area, organic, and inorganic carbon burial rate of Lake Qinghai, Lake Balikun, Lake Hongjiannao, Lake Hulun, Lake Erbinur, Lake Daihai, Lake Wulungu, Lake Bosten (Lan et al., 2015), Lake Erhai (Liu et al., 2019), Lake Dianchi (Huang et al., 2018), Lake Shijiuhu, Lake Taihu, Lake Honghu, Lake Nanyihu (Gui et al., 2012), and Lake Chaohu (Dong et al., 2012). Total C burial was calculated by multiplying carbon burial rates with lake area.
Large lakes generally have slow C sedimentation rates (Algesten et al., 2004), however, the large Lake Hulun (2,330 km2) showed a relatively higher OCBR (45.5 g m−2 yr−1) (Lan et al., 2015) (Figure 5). Lake Hulun has long suffered natural eutrophication resulting from the combined influences of climate warming and drying, water volume reduction, and increased evaporation (Chuai, 2011). In brief, increased primary productivity of a lake, whether it is the consequence of growing temperature or anthropogenic nutrient loading, can thereby promote organic carbon accumulation, resulting in high OCBRs in lake sediments.
Different from open freshwater lakes that mainly deposit organic carbon, inorganic carbon burial contributed approximately 50% to the total carbon burial in Lake Qinghai (Figure 5). Because of the supersaturation of carbonate minerals in water bodies, inorganically precipitated carbonate generally exists in brackish and saline lakes (Einsele et al., 2001; Lammers and Depaolo, 2010; Balci et al., 2018). The semi-enclosed status and high salinity condition in Lake Qinghai lead to a mass of carbonate precipitation and sequestration, resulting in a large IC burial proportion. High IC burial proportion was also found in multiple lakes that have similar hydro-chemical characteristics to Qinghai-Tibet alpine and arid/semiarid regions (Figure 5). For example, IC burial accounted for 68, 46, 42, 52, 44, and 33% of the total C burial in Lake Balikun, Lake Hongjiannao, Lake Erbinur, Lake Daihai, Lake Wulungu, and Lake Hulun, respectively (Lan et al., 2015) (Figure 5). It should be noted that a tiny minority of freshwater lakes also have abundant carbonate minerals. For example, IC burial in freshwater Lake Bosten was even higher than OC burial (Lan et al., 2015), possibly due to the formation of biogenic carbonate or seasonal changes in water chemistry (Kelts and Hsü, 1978; Loeppert and Suarez, 1996). Anyway, saline lakes compose a crucial inorganic carbon pool in addition to an organic carbon pool.
Owing to a large area and high IC burial, total annual C burial of Lake Qinghai surficial sediments (95.27 ± 37.74 Gg C yr−1) was higher than that in many other lakes. Among the 15 lakes shown in Figure 5, Lake Qinghai ranked first in the area and second in total annual C burial despite the relatively lower carbon burial rates. Most lakes (10 of 15) with a smaller surface area showed a lower total C burial even with a much higher organic and/or inorganic carbon burial rate (Figure 5). Based on a total lake area of 3,185.06 km2, only 11.5 Gg C yr−1 of organic carbon was estimated to be sequestrated annually in sediments of lakes in SW Greenland (Anderson et al., 2019). The organic form of carbon exists in all lakes, but some specific lakes have additional authigenic IC precipitation (Cole et al., 2007), which increases the total carbon burial (Figure 5). Therefore, the lake area and water body types are main factors that strongly affect the carbon burial in different lakes, which tallies with the conclusion of Clow et al. (2015).
A large number of lakes (most are closed or semi-closed saltwater type) are spread over the Qinghai–Tibet Plateau, with an area more than 50% of the total area of lakes in China (Wan et al., 2016). Carbon burial in Tibet Plateau lakes is therefore undoubtedly an important component of regional and even global carbon cycle which remain unstudied. More attention and exploration are required for comprehensive understanding of organic and inorganic carbon burial in lake sediments at the Third Pole.
Conclusion
Organic carbon of Lake Qinghai was mainly derived from aquatic organisms and well preserved in lake sediments. The TOC content ranged from 1.4 to 4.8% (mean 3.1%) with significant spatial divergence. It was higher in the northern lake area adjacent to the bank with strong human activities and lower in the Buha River mouth and the eastern lake area. The inorganic carbon was dominated by authigenic aragonite with content ranging 1.5–3.8% (mean 3.0%), showing a high value in the lake central area and a significantly low level in the northwestern lake area. This spatial pattern of TIC can be mainly attributed to hydro-chemical characteristics of its lake water and hydraulic conditions controlled by prevailing northwest wind. The OCBR of Lake Qinghai surficial sediments ranged from 4.54 to 30.50 g C m−2 yr−1, while ICBRs varied from 3.32 to 24.46 g C m−2 yr−1. Correspondingly, total annual organic carbon burial (47.50 ± 22.68 Gg C yr−1) was approximately equal to inorganic carbon burial (47.77 ± 19.73 Gg C yr−1). Both OC burial and IC burial contributed about 50%, suggesting that saline lakes are not only a crucial organic carbon pool but also an important inorganic carbon pool. Lake Qinghai sequestered 95.27 ± 37.74 Gg C yr−1, demonstrating a huge carbon sequestration potential due to its large area. Our study provides scientific and technological support for understanding, under the influence of human activities and hydraulic condition, the spatial variation characteristics of carbon in large and deepwater lakes with conditions analogous with Lake Qinghai. This is also an archive for further research on carbon burial in plateau lakes on different timescales.
Data Availability Statement
The original contributions presented in the study are included in the article/Supplementary Material; further inquiries can be directed to the corresponding authors.
Author Contributions
CX performed the test, data processing, analysis and wrote the manuscript. MX conceptualized and designed the research, and reviewed the manuscript. SY reviewed and edited the manuscript. WZ processed the data and plotted. ZB processed the data and plotted. ZB analyzed the data and plotted. ZE funded the research.
Funding
This work was supported by the National Science Foundation of China (Nos. 42025707 and 41790423) and the Second Tibetan Plateau Scientific Expedition and Research Program (STEP) (Grant No. 2019QZKK0202) and Jiangxi Normal University's Domestic and Overseas Study Program for Postgraduates.
Conflict of Interest
The authors declare that the research was conducted in the absence of any commercial or financial relationships that could be construed as a potential conflict of interest.
Acknowledgments
The data set is provided by National Tibetan Plateau Data Center (http://data.tpdc.ac.cn/). We thank Zhenyu Ni and Rong Chen from the State Key Laboratory of Lake Science and Environment, Nanjing Institute of Geography and Limnology, and Dongliang Ning from the School of Geography Sciences, Nantong University, for field sampling and their constructive suggestions. We also thank Jianru Cheng from the School of Earth Sciences and Engineering, Nanjing University for assistance in carbonate testing.
References
Alcocer, J., Ruiz-Fernández, A. C., Escobar, E., Pérez-Bernal, L. H., Oseguera, L. A., and Ardiles-Gloria, V. (2014). Deposition, Burial and Sequestration of Carbon in an Oligotrophic, Tropical lake. J. Limnol 73 (2), 223–235. doi:10.4081/jlimnol.2014.783
Algesten, G., Sobek, S., Bergström, A.-K., Ågren, A., Tranvik, L. J., and Jansson, M. (2004). Role of Lakes for Organic Carbon Cycling in the Boreal Zone. Glob. Change Biol. 10, 141–147. doi:10.1111/j.1365-2486.2003.00721.x
An, Z., Colman, S. M., Zhou, W., Li, X., Brown, E. T., Jull, A. J. T., et al. (2012). Interplay between the Westerlies and Asian Monsoon Recorded in Lake Qinghai Sediments since 32 Ka. Sci. Rep. 2, 619. doi:10.1038/srep00619
Anas, M. U. M., Scott, K. A., and Wissel, B. (2015). Carbon Budgets of Boreal Lakes: State of Knowledge, Challenges, and Implications. Environ. Rev. 23 (3), 275–287. doi:10.1139/er-2014-0074
Anderson, N. J., Appleby, P. G., Bindler, R., Renberg, I., Conley, D. J., Fritz, S. C., et al. (2019). Landscape-Scale Variability of Organic Carbon Burial by SW Greenland Lakes. Ecosystems 22 (8), 1706–1720. doi:10.1007/s10021-019-00368-8
Anderson, N. J., Dietz, R. D., and Engstrom, D. R. (2013). Land-use Change, Not Climate, Controls Organic Carbon Burial in Lakes. Proc. R. Soc. B. 280 (1769), 20131278. doi:10.1098/rspb.2013.1278
Balci, N., Demirel, C., Akcer Ön, S., Gültekin, A. H., and Kurt, M. A. (2018). Evaluating Abiotic and Microbial Factors on Carbonate Precipitation in Lake Acigöl, a Hypersaline lake in Southwestern Turkey. Quat. Int. 486, 116–128. doi:10.1016/j.quaint.2017.12.046
Bi, r., Zhang, h., Li, h., Chang, f., Duan, l., He, y., et al. (2018). Characteristics and Changes of Water Quality Parameters of Qinghai Lake in 2015. J. Water Resour. Res. 7 (1), 74. doi:10.12677/JWRR.2018.71009
Chang, B., He, K.-N., Li, R.-J., Sheng, Z.-P., and Wang, H. (2017). Linkage of Climatic Factors and Human Activities with Water Level Fluctuations in Qinghai Lake in the Northeastern Tibetan Plateau, China. Water 9 (7), 552. doi:10.3390/w9070552
Chen, H., Zhu, L., Wang, J., Ju, J., Ma, Q., and Xu, T. (2021). Paleoclimate Changes over the Past 13,000 Years Recorded by Chibuzhang Co Sediments in the Source Region of the Yangtze River, China. Palaeogeogr. Palaeoclimatol. Palaeoecol. 573, 110433. doi:10.1016/j.palaeo.2021.110433
Chen, x., Zhu, y., Fu, x., and Luo, y. (2012). Investigation of Eutrophic Elements Distribution and Their Correlation in Qinhai Lake Surface Sediments. J. Agro-Environment Sci. 31 (02), 181–187.
Chuai, X. (2011). Study on Lake Eutrophication as Well as the Criteria and Control Standard for Phosphorus in China. Nanjing: Nanjing University.
Clow, D. W., Stackpoole, S. M., Verdin, K. L., Butman, D. E., Zhu, Z., Krabbenhoft, D. P., et al. (2015). Correction to Organic Carbon Burial in Lakes and Reservoirs of the Conterminous United States. Environ. Sci. Technol. 49 (24), 14741. doi:10.1021/acs.est.5b00373
Cole, J. J., Prairie, Y. T., Caraco, N. F., McDowell, W. H., Tranvik, L. J., Striegl, R. G., et al. (2007). Plumbing the Global Carbon Cycle: Integrating Inland Waters into the Terrestrial Carbon Budget. Ecosystems 10 (1), 172–185. doi:10.1007/s10021-006-9013-8
Dean, W. E., and Gorham, E. (1998). Magnitude and Significance of Carbon Burial in Lakes, Reservoirs, and Peatlands. Geology 26 (6), 535. doi:10.1130/0091-7613(1998)026<0535:masocb>2.3.co;2
Dietz, R. D., Engstrom, D. R., and Anderson, N. J. (2015). Patterns and Drivers of Change in Organic Carbon Burial across a Diverse Landscape: Insights from 116 minnesota Lakes. Glob. Biogeochem. Cycles 29 (5), 708–727. doi:10.1002/2014gb004952
Dong, H., Song, Y., and Zhang, M. (2018). Hydrological Trend of Qinghai Lake over the Last 60 years: Driven by Climate Variations or Human Activities? J. Water Clim. Change 10 (3), 524–534. doi:10.2166/wcc.2018.033
Dong, X., Anderson, N. J., Yang, X., chen, X., and Shen, J. (2012). Carbon Burial by Shallow Lakes on the Yangtze Floodplain and its Relevance to Regional Carbon Sequestration. Glob. Change Biol. 18 (7), 2205–2217. doi:10.1111/j.1365-2486.2012.02697.x
Einsele, G., Yan, J., and Hinderer, M. (2001). Atmospheric Carbon Burial in Modern lake Basins and its Significance for the Global Carbon Budget. Glob. Planet. Change 30, 167–195. doi:10.1016/S0921-8181(01)00105-9
Fu, g.-y., Sha, z.-j., Zhang, k., Zhao, s., and Guo, l. (2015). Research of Deposition Rate in Northwestern Qinghai Lake Based on the137Cs and 210Pb Dating. J. Salt Lake Res. 23 (2), 7–14.
Gui, Z.-f., Xue, B., Yao, S.-c., Wei, W.-j., and Yi, S. (2012). Organic Carbon Burial in lake Sediments in the Middle and Lower Reaches of the Yangtze River Basin, China. Hydrobiologia 710 (1), 143–156. doi:10.1007/s10750-012-1365-9
Gyawali, A. R., Wang, J., Ma, Q., Wang, Y., Xu, T., Guo, Y., et al. (2019). Paleo-environmental Change since the Late Glacial Inferred from Lacustrine Sediment in Selin Co, central Tibet. Palaeogeogr. Palaeoclimatol. Palaeoecol. 516, 101–112. doi:10.1016/j.palaeo.2018.11.033
Han, y., Hao, l., Wang, q., Ma, d., and Ji, h. (2016). Prevailing Winds Controlled Hydrodynamic Characteristics of Qinghai Lake. Yellow River 38 (3), 51–55. doi:10.3969/j.issn.1000-1379.2016.03.015
He, H., Liu, G., Zou, Y., Li, X., Ji, M., and Li, D. (2021). Coupled Action of Rock Weathering and Aquatic Photosynthesis: Influence of the Biological Carbon Pump Effect on the Sources and Deposition of Organic Matter in Ngoring Lake, Qinghai-Tibet Plateau, China. Catena 203, 105370. doi:10.1016/j.catena.2021.105370
Heathcote, A. J., Anderson, N. J., Prairie, Y. T., Engstrom, D. R., and del Giorgio, P. A. (2015). Large Increases in Carbon Burial in Northern Lakes during the Anthropocene. Nat. Commun. 6 (1), 10016. doi:10.1038/ncomms10016
Hedges, J. I., and Keil, R. G. (1995). Sedimentary Organic Matter Preservation: an Assessment and Speculative Synthesis. Mar. Chem. 49 (2), 81–115. doi:10.1016/0304-4203(95)00008-F
Hedges, J. I., and Oades, J. M. (1997). Comparative Organic Geochemistries of Soils and marine Sediments. Org. Geochem. 27 (7), 319–361. doi:10.1016/S0146-6380(97)00056-9
Henderson, A., Holmes, J., and Leng, M. (2007). Atmospheric Circulation Changes during the Little Ice Age in NW China: A Late Holocene Oxygen Isotope Record from Lake Qinghai, NE Tibetan Plateau. San Francisco, CA: AGU Fall Meeting Abstracts.
Hollander, D. J., McKenzie, J. A., and Haven, H. L. t. (1992). A 200 Year Sedimentary Record of Progressive Eutrophication in Lake Greifen (Switzerland): Implications for the Origin of Organic-Carbon-Rich Sediments. Geol 20, 825–828. doi:10.1130/0091-7613(1992)020<0825:aysrop>2.3.co;2
Huang, C., Zhang, L., Li, Y., Lin, C., Huang, T., Zhang, M., et al. (2018). Carbon and Nitrogen Burial in a Plateau lake during Eutrophication and Phytoplankton Blooms. Sci. Total Environ. 616-617, 296–304. doi:10.1016/j.scitotenv.2017.10.320
Ji, J., Ge, Y., Balsam, W., Damuth, J. E., and Chen, J. (2009). Rapid Identification of Dolomite Using a Fourier Transform Infrared Spectrophotometer (FTIR): A Fast Method for Identifying Heinrich Events in IODP Site U1308. Mar. Geology. 258 (1), 60–68. doi:10.1016/j.margeo.2008.11.007
Ji, J., Shen, J., Balsam, W., Chen, J., Liu, L., and Liu, X. (2005). Asian Monsoon Oscillations in the Northeastern Qinghai-Tibet Plateau since the Late Glacial as Interpreted from Visible Reflectance of Qinghai Lake Sediments. Earth Planet. Sci. Lett. 233, 61–70. doi:10.1016/j.epsl.2005.02.025
Kastowski, M., Hinderer, M., and Vecsei, A. (2011). Long-term Carbon Burial in European Lakes: Analysis and Estimate. Glob. Biogeochem. Cycles 25 (3), a–n. doi:10.1029/2010GB003874
Kelts, K., and Hsü, K. J. (1978). “Freshwater Carbonate Sedimentation,” in Lakes: Chemistry, Geology, Physics. Editor A. Lerman (New York, NY: Springer New York), 295–323. doi:10.1007/978-1-4757-1152-3_9
Kunzler, R. H., and Goodell, H. G. (1970). The Aragonite-Calcite Transformation; a Problem in the Kinetics of a Solid-Solid Reaction. Am. J. Sci. 269, 360–391. doi:10.2475/ajs.269.4.360
Lammers, L., and Depaolo, D. (2010). The Influence of Solution Stoichiometry on Surface-Controlled Ca Isotope Fractionation during Ca Carbonate Precipitation from Mono Lake, California. San Francisco, CA: AGU Fall Meeting Abstracts.
Lan, J., Xu, H., Liu, B., Sheng, E., Zhao, J., and Yu, K. (2015). A Large Carbon Pool in lake Sediments over the Arid/semiarid Region, NW China. Chin. J. Geochem. 34 (3), 289–298. doi:10.1007/s11631-015-0047-5
Lian, X., Qi, y., Wang, h., Zhang, j., and Yang, r. (2019). Spatial Pattern of Ecosystem Services under the Influence of Human Activities in Qinghai Lake Watershed. J. Glaciology Geocryology 41 (5), 1254–1263. doi:10.7522/j.issn.1000-0240.2019.0531
Liu, E., Shen, J., Zhang, E., Wu, Y., and Yang, L. (2010). A Geochemical Record of Recent Anthropogenic Nutrient Loading and Enhanced Productivity in Lake Nansihu, China. J. Paleolimnol 44 (1), 15–24. doi:10.1007/s10933-009-9382-z
Liu, h., Liu, e., Yu, z., Zhang, e., Lin, q., Wang, r., et al. (2019). Spatio-temporal Patterns of Organic Carbon Burial in the Sediment of Lake Erhai in China during the Past 100 Years. J. Lake Sci. 31 (1), 282–292. doi:10.18307/2019.0126
Liu, j., Zhuang, d., Wang, j., Zhou, w., and Wu, s. (2014). Landuse/Landcover Data of the QinghaiLake River Basin (2000). Beijing, China: National Tibetan Plateau Data Center.
Liu, W., Yang, H., Wang, H., An, Z., Wang, Z., and Leng, Q. (2015). Carbon Isotope Composition of Long Chain Leaf Wax N -alkanes in lake Sediments: A Dual Indicator of Paleoenvironment in the Qinghai-Tibet Plateau. Org. Geochem. 83-84, 190–201. doi:10.1016/j.orggeochem.2015.03.017
Liu, W., Zhang, P., Zhao, C., Wang, H., An, Z., and Liu, H. (2018). Reevaluation of Carbonate Concentration and Oxygen Isotope Records from Lake Qinghai, the Northeastern Tibetan Plateau. Quat. Int. 482, 122–130. doi:10.1016/j.quaint.2018.03.038
Liu, X., Colman, S. M., Brown, E. T., An, Z., Zhou, W., Jull, A. J. T., et al. (2014b). A Climate Threshold at the Eastern Edge of the Tibetan Plateau. Geophys. Res. Lett. 41 (15), 5598–5604. doi:10.1002/2014gl060833
Liu, X. Q., Shen, J., Wang, S. M., Zhang, E., and Cai, Y. F. (2003). A 16000-year Paleoclimatic Record Derived from Authigenetic Carbonate of Lacustrine Sediment in Qinghai Lake. Geol. J. China Universities 9, 38–46. doi:10.3969/j.issn.1006-7493.2003.01.005
Loeppert, R. H., and Suarez, D. (1996). Carbonate and gypsum, Methods of Soil Analysis. United States: Soil Science Society of America and American Society of Agronomy, 437–474.
Lundin, E. J., Klaminder, J., Bastviken, D., Olid, C., Hansson, S. V., and Karlsson, J. (2015). Large Difference in Carbon Emission - Burial Balances between Boreal and Arctic Lakes. Sci. Rep. 5, 14248. doi:10.1038/srep14248
Luo, c., Xu, c., Cao, y., and Tong, l. (2017). Monitoring of Water Surface Area in Lake Qinghai from 1974 to 2016. J. Lake Sci. 29 (5), 1245–1253. doi:10.18307/2017.0523
Martín-García, R., Alonso-Zarza, A. M., Frisia, S., Rodríguez-Berriguete, Á., Drysdale, R., and Hellstrom, J. (2019). Effect of Aragonite to Calcite Transformation on the Geochemistry and Dating Accuracy of Speleothems. An Example from Castañar Cave, Spain. Sediment. Geology. 383, 41–54. doi:10.1016/j.sedgeo.2019.01.014
Mayer, L. M. (1994). Relationships between mineral Surfaces and Organic Carbon Concentrations in Soils and Sediments. Chem. Geology. 114 (3), 347–363. doi:10.1016/0009-2541(94)90063-9
Meng, X., Liu, L., Balsam, W., li, S., He, T., Chen, J., et al. (2015). Dolomite Abundance in Chinese Loess Deposits: A New Proxy of Monsoon Precipitation Intensity. Geophys. Res. Lett. 42, 10391–10398. doi:10.1002/2015GL066681
Meng, X., Liu, L., Zhao, W., He, T., Chen, J., and Ji, J. (2019). Distant Taklimakan Desert as an Important Source of Aeolian Deposits on the Chinese Loess Plateau as Evidenced by Carbonate Minerals. Geophys. Res. Lett. 46 (9), 4854–4862. doi:10.1029/2018GL081551
Meyers, P. A., and Ishiwatari, R. (1993). Lacustrine Organic Geochemistry—An Overview of Indicators of Organic Matter Sources and Diagenesis in lake Sediments. Org. Geochem. 20 (7), 867–900. doi:10.1016/0146-6380(93)90100-P
Meyers, P. A., and Lallier-vergés, E. (1999). Lacustrine Sedimentary Organic Matter Records of Late Quaternary Paleoclimates. J. Paleolimnology 21 (3), 345–372. doi:10.1023/A:1008073732192
Müller, A., and Mathesius, U. (1999). The Palaeoenvironments of Coastal Lagoons in the Southern Baltic Sea, I. The Application of Sedimentary Corg/N Ratios as Source Indicators of Organic Matter. Palaeogeogr. Palaeoclimatol. Palaeoecol. 145 (1), 1–16. doi:10.1016/S0031-0182(98)00094-7
Qin, B., and Huang, Q. (1998). Evaluation of the Climatic Change Impacts on the Inland Lake – A Case Study of Lake Qinghai, China. Climatic Change 39 (4), 695–714. doi:10.1023/A:1005319616456
Rumolo, P., Barra, M., Gherardi, S., Marsella, E., and Sprovieri, M. (2011). Stable Isotopes and C/N Ratios in marine Sediments as a Tool for Discriminating Anthropogenic Impact. J. Environ. Monit. 13 (12), 3399–3408. doi:10.1039/c1em10568j
Schubert, C. J., and Calvert, S. E. (2001). Nitrogen and Carbon Isotopic Composition of marine and Terrestrial Organic Matter in Arctic Ocean Sediments:. Deep Sea Res. Oceanographic Res. Pap. 48 (3), 789–810. doi:10.1016/S0967-0637(00)00069-8
Scott, D. R. (2014). Carbon Fixation, Flux and Burial Efficiency in Two Contrasting Eutrophic Lakes in the UK (Rostherne Mere & Tatton Mere). Loughborough: Loughborough University.
Shang, X., Li, X., An, Z., Ji, M., and Zhang, H. (2009). Modern Pollen Rain in the Lake Qinghai basin, China. Sci. China Ser. D-earth Sci. 52 (10), 1510–1519. doi:10.1007/s11430-009-0150-8
Shen, J., Zhang, E., and Xia, W. (2001). Records from lake Sediments of the Qinghai Lake to Minor Climatic and Environmental Changes of the Past about 1000 Years. Quat. Sci. Rev. 21 (6), 508–513.
Sobek, S., Durisch-Kaiser, E., Zurbrügg, R., Wongfun, N., Wessels, M., Pasche, N., et al. (2009). Organic Carbon Burial Efficiency in lake Sediments Controlled by Oxygen Exposure Time and Sediment Source. Limnol. Oceanogr. 54, 2243–2254. doi:10.4319/lo.2009.54.6.2243
Sun, D., He, Y., Wu, J., Liu, W., and Sun, Y. (2019). Hydrological and Ecological Controls on Autochthonous Carbonate Deposition in Lake Systems: A Case Study from Lake Wuliangsu and the Global Perspective. Geophys. Res. Lett. 46 (12), 6583–6593. doi:10.1029/2019gl082224
Talbot, M. R., and Johannessen, T. (1992). A High Resolution Palaeoclimatic Record for the Last 27,500 Years in Tropical West Africa from the Carbon and Nitrogen Isotopic Composition of Lacustrine Organic Matter. Earth Planet. Sci. Lett. 110 (1-4), 23–37. doi:10.1016/0012-821X(92)90036-U
Talbot, M. R., and Lærdal, T. (2000). The Late Pleistocene - Holocene Palaeolimnology of Lake Victoria, East Africa, Based upon Elemental and Isotopic Analyses of Sedimentary Organic Matter. J. Paleolimnology 23 (2), 141–164. doi:10.1023/A:1008029400463
Tenzer, G., Meyers, P., and Knoop, P. (1997). Sources and Distribution of Organic and Carbonate Carbon in Surface Sediments of Pyramid Lake, Nevada. J. Sediment. Res. 67 (5), 884–890. doi:10.1306/D4268667-2B26-11D7-8648000102C1865D
Terasmaa, J., and Punning, J.-M. (2006). Sedimentation Dynamics in a Small Dimictic lake in Northern Estonia. Proc. Estonian Acad. Sci. Biol. Ecol. 55, 228–242.
Thompson, S., and Eglinton, G. (1978). The Fractionation of a Recent Sediment for Organic Geochemical Analysis. Geochimica et Cosmochimica Acta 42, 199–207. doi:10.1016/0016-7037(78)90132-1
Tranvik, L. J., Downing, J. A., Cotner, J. B., Loiselle, S. A., Striegl, R. G., Ballatore, T. J., et al. (2009). Lakes and Reservoirs as Regulators of Carbon Cycling and Climate. Limnol. Oceanogr. 54 (6), 2298–2314. doi:10.4319/lo.2009.54.6_part_2.2298
Tyson, R. V. (1995). Sedimentary Organic Matter. Heidelberg: Springer Netherlands. doi:10.1007/978-94-011-0739-6
Wan, W., Long, D., Hong, Y., Ma, Y., Yuan, Y., Xiao, P., et al. (2016). A lake Data Set for the Tibetan Plateau from the 1960s, 2005, and 2014. Sci. Data 3 (1), 160039. doi:10.1038/sdata.2016.39
Wang, s., Dou, h., Chen, k., Wang, x., and Jiang, j. (1998). China Lakes Record. Beijing: Science Press Ltd.
Wu, P., Gao, C., Chen, F., and Yu, S. (2016). Response of Organic Carbon Burial to Trophic Level Changes in a Shallow Eutrophic lake in SE China. J. Environ. Sci. 46, 220–228. doi:10.1016/j.jes.2016.05.003
Xu, H., Lan, J., Liu, B., Sheng, E., and Yeager, K. M. (2013). Modern Carbon Burial in Lake Qinghai, China. Appl. Geochem. 39, 150–155. doi:10.1016/j.apgeochem.2013.04.004
Xu, H., Liu, X., An, Z., Hou, Z., Dong, J., and Liu, B. (2010). Spatial Pattern of Modern Sedimentation Rate of Qinghai lake and a Preliminary Estimate of the Sediment Flux. Chin. Sci. Bull. 55 (4-5), 621–627. doi:10.1007/s11434-009-0580-x
Yu, J. Q., and Kelts, K. R. (2002). Abrupt Changes in Climatic Conditions across the Late-glacial/Holocene Transition on the N. E. Tibet-Qinghai Plateau: Evidence from Lake Qinghai, China. J. Paleolimnology 28 (2), 195–206. doi:10.1023/A:1021635715857
Yu, Z. T., Wang, X. J., Zhang, E. L., Zhao, C. Y., and Liu, X. Q. (2015). Spatial Distribution and Sources of Organic Carbon in the Surface Sediment of Bosten Lake, China. Biogeosciences 12 (22), 6605–6615. doi:10.5194/bg-12-6605-2015
Zhang, F., Bin, X. U. E., and Shuchun, Y. A. O. (2019). Spatiotemporal Pattern of Inorganic Carbon Sequestration in Lake Hulun since 1850. J. Lake Sci. 31, 1770–1782. doi:10.18307/2019.0617
Zhang, F., Yao, S., Xue, B., Lu, X., and Gui, Z. (2017). Organic Carbon Burial in Chinese Lakes over the Past 150 Years. Quat. Int. 438, 94–103. doi:10.1016/j.quaint.2017.03.047
Zhang, j., Chen, y., Ge, j., and Nie, x. (2013). Land Use/cover Change and Land Sources Management in the Area Around the Qinghai Lake of China in 1977-2010. J. Desert Reaseach 33 (4), 1256–1266. doi:10.7522/j.issn.1000-694X.2013.00177
Keywords: Lake Qinghai, organic carbon, inorganic carbon, carbon cycle, carbon burial
Citation: Chen X, Meng X, Song Y, Zhang B, Wan Z, Zhou B and Zhang E (2021) Spatial Patterns of Organic and Inorganic Carbon in Lake Qinghai Surficial Sediments and Carbon Burial Estimation. Front. Earth Sci. 9:714936. doi: 10.3389/feart.2021.714936
Received: 26 May 2021; Accepted: 21 June 2021;
Published: 22 July 2021.
Edited by:
Zhuolun Li, Lanzhou University, ChinaCopyright © 2021 Chen, Meng, Song, Zhang, Wan, Zhou and Zhang. This is an open-access article distributed under the terms of the Creative Commons Attribution License (CC BY). The use, distribution or reproduction in other forums is permitted, provided the original author(s) and the copyright owner(s) are credited and that the original publication in this journal is cited, in accordance with accepted academic practice. No use, distribution or reproduction is permitted which does not comply with these terms.
*Correspondence: Xianqiang Meng, xqmeng@niglas.ac.cn; Yinxian Song, songyinxian@gmail.com