- 1Department of Plant Science, Peatland Ecology Research Group and Centre for Northern Studies, Université Laval, Quebec, QC, Canada
- 2Direction Environnement, Hydro-Québec, Montreal, QC, Canada
Mineral roads in peatlands change the nature of the substrate, influence the water table level of the peatland on either side of the road and the physicochemical characteristics of the water and peat. These changes can in turn affect plant community composition. The efficiency of an innovative and affordable method for the restoration of peatlands impacted by roads was evaluated: the Burial Under Peat Technique. To be considered effective from an ecological point of view, the technique should meet restoration goals by 1) confining the chemical elements and compounds potentially leaching from the mineral material; 2) creating and maintaining a restored surface elevation similar to the adjacent peatland for optimal rewetting; and 3) re-establishing typical peatland vegetation communities. Three years post-restoration, water sampled at various depths and distances to the buried road presented chemical elements and compounds concentrations similar to the means measured in the pristine surrounding peatland for most of the ions analyzed. The different steps of the technique ensured the reestablishment of an elevation similar to the surrounding peatland. The return of peatland plant communities was slow, mainly due to local factors (e.g., presence of drainage ditches). Furthermore, the Burial Under Peat Technique fulfilled the restoration objectives in re-establishing an acid organic soil. Finally, it is a cost-effective method in comparison to completely removing the mineral material and transporting new material to fill the depression left by the excavation of the road.
Introduction
Peatlands cover roughly 4 million km2 of the Northern Hemisphere (Yu et al., 2010), which represents 25–30% of the circumboreal forest biome (Wieder et al., 2006). It is inevitable that access roads built for industrial activities such as resource extraction, forestry, the deployment or maintenance of electricity transmission networks or the petrol industry cross paths with peatlands in the boreal zone (Pasher et al., 2013). Roads impact various components of peatland ecosystems. In addition to locally stopping the peatland carbon accumulation function, the introduction of the mineral material may restrict the hydrological connectivity across the road and, thus, influence the water table level (Bocking et al., 2017), the physicochemical properties (Plach et al., 2017; Saraswati et al., 2020), the tree and shrub productivity (Saraswati et al., 2020) and the net CO2 sequestration of the surrounding peatland (Plach et al., 2017). While road impacts on peatlands have been the subject of several studies in the past decade, there is a need for research regarding restoration techniques (Williams-Mounsay et al., 2021).
Water flows slowly through peat, but linear structures such as roads can create barriers and cause upstream flooding and downstream desiccation problems (Umeda et al., 1985; Miller, 2011; Bocking et al., 2017) which may enhance the overall peatland CH4 emissions (Saraswati and Strack, 2019). Furthermore, the construction of a road within a peatland also introduces minerals and other nutrients into an oligotrophic acid peaty substrate which have the potential to diffuse laterally. However, the estimation of the distance to which chemical elements and compounds may leach into the adjacent ecosystem is complex, mainly due to the anisotropic characteristic of Sphagnum peatlands (i.e., peat hydraulic conductivity is greater horizontally than vertically; Beckwith et al., 2003). Besides, the presence of a gentle slope within a peatland can cause superficial flow, influencing chemical elements and compounds leaching distances.
The modifications in the concentration, ionic form and ratio of the chemical elements within the substrate can modify vegetation composition and diversity, considering that the composition of vegetation communities is closely tied to the hydrological and physicochemical characteristics of the plant species ecological niches (Gignac et al., 1991, 2004). Therefore, the ecosystem can shift from species adapted to poor acidic environments to species preferring richer environments (Kooijman and Bakker, 1995; de Mars et al., 1996; Laiho et al., 2003; Müllerová et al., 2011). When a formerly limiting nutrient is added to a Sphagnum peatland, characterized by oligotrophic conditions, graminoid species tend to proliferate whereas bryophytes and other slow-growing species decline (Hogg et al., 1995; Limpens et al., 2011). Consequently, the introduction of a mineral access road in a Sphagnum peatland could favor the spread of unwanted or invasive species to the detriment of bog species.
Road mitigation techniques, such as scarification, topsoiling, planting, removal of stream crossing structures and partial or complete decommissioning, have been developed for forest biomes (Cots et al., 1991; Luce, 1997; Bagley, 1998; Switalski et al., 2004; Sosa-Pérez and MacDonald, 2017). Most of these techniques are not adapted to peatlands because the mineral material remains on site, acting as a barrier for hydrological connectivity and a potential source of nutrient enrichment within the peatland. Road decommissioning in peatlands involves removing the mineral material and other materials used (e.g., geotextile, logs) and backfilling with peat, sawdust or woodchips. Backfilling is required to restore an elevation similar to the undisturbed surrounding peatland. In the absence of backfilling, open water bodies may be formed and be colonized by monospecific invasive plant communities (e.g., cattail, common reed, etc.), greatly hindering the establishment of natural pool edges plant communities (Bourgeois et al., 2012) and causing greenhouse gas emissions (Hamilton et al., 1994; Strack and Zuback, 2013).
Prior to this research project, only a few attempts to restore peatlands disturbed by mineral infrastructures have been conducted, particularly in oil sands regions of Alberta (Canada), indicating a study gap between studies on the effects of roads on peatlands and research on the mitigation of these effects. Decommissioning in the known projects involved the removal of the mineral material, either partially (Osko et al., 2014) or completely (Pilon, 2015), and the removal of a well-pad by the burial under a peat layer (Sobze et al., 2012). In Pilon (2015) a slow decompression of the peat was observed following the complete removal of the clay covering it. This meant that the water flow through the disturbance was no longer being obstructed (Pilon, 2015). In Osko (2015), the partial removal of clay did not sufficiently improve water flow. By lowering the surface of the mineral material, depressions were created, where water accumulated instead of flowing toward the adjacent peatland. Osko (2015) recommended filling the excavation with stockpiled peat to restore the hydrological connectivity. Finally, the burial of the well-pad clay, more specifically the vertical inversion of the clay layer and the underlying peat, was an effective technique to reach an elevation similar to what is observed in the surrounding peatland (Sobze et al., 2012). These studies underline the technical knowledge gaps regarding restoration methods adapted to peatlands impacted by roads.
This project aims at developing a restoration technique for peatlands disturbed by linear mineral disturbances based on the burying of the material used for their construction. To favor the hydrological flow through an artificial acrotelm (surface layer of peat where the water table fluctuates), the mineral material must be confined in the catotelm (bottom layer of peat, constantly under anaerobic conditions). The long term lateral diffusion of chemical elements and compounds from the buried mineral should be considered negligible as a result of several unique properties of peat. Firstly, the peat in the catotelm, where the mineral material will be confined, is characterized by a low hydraulic conductivity (Letts et al., 2000). Secondly, peat has a high-buffering capacity that is linked to its high cation exchange capacity (CEC) which increases with peat decomposition (Puustjärvi 1956). Furthermore, the growth of roots and the assimilation of plant nutritive chemical elements are processes that require oxygen (Bates, 2009). Thus, only the species located within or close to the restored strip and that possess specialized structure for oxygen transportation (wetland species; Justin and Armstrong, 1987; Colmer, 2003) could benefit from the mineral material that is buried in the anaerobic zone (catotelm).
The objectives of this study were to (1) confine the chemical elements and compounds potentially released by the mineral material, (2) restore, on the former road, a layer of organic soil of similar elevation to the surrounding peatland, and (3) initiate the revegetation of plant communities characteristic of peatlands and limit the spread of undesired plants. We hypothesized that burying the mineral material should confine the chemical elements and compounds and that the peat substrate on the former road with an elevation similar to the surrounding peatland (water-saturated but not flooded) should favor the establishment of Sphagnum peat mosses and other typical peatland species and be an indicator of the restoration of the hydrological connectivity across the former road. The undisturbed right-of-way and the surrounding pristine peatland were used as reference ecosystems (local models or targets derived from multiple sources of information to characterize the state of the ecosystem as it would be if it had not been degraded; McDonald et al., 2016).
Material and Methods
Study Site
In 2012, a temporary access road was built in a 9,000-hectare peatland complex at Sainte-Eulalie (Québec, Canada; 46.098°N, −72.288°W) for the purpose of reconstructing a 60 year-old powerline (Supplementary Figure S1). The mean annual temperature and total precipitations are respectively 4.9°C and 968 mm (of which 84% falls as rain), based on 30-years monthly mean values (1981–2010; Government of Canada, 2018). The access road was built in a power-line right-of-way that bisects a peatland complex. Agricultural type ditches are present on both sides of the 30 m right-of-way to maintain relatively dry access to the transmission towers. The effect of the ditches can be seen in the graph of the water table fluctuation (Figure 1). Indeed, we can observe that the water level decreases rapidly after rain events, unlike undrained peatlands where water level decreasing is gradual. Nevertheless, since there is no maintenance on the ditches, their effectiveness is limited considering that the lowest level reached by the water table in 2015 is −33 cm. The access road was 3 km long with an average width of 4.5 m. It was composed of basalt gravel placed on a geotextile membrane. The road was reinforced with logs underneath the geotextile in the wetter areas, to prevent machinery from sinking into the peat. Typical open raised bog plant communities as found in the province of Québec (e.g., Sphagnum mosses, ericaceous shrubs; Couillard and Grondin, 1986) compose the right-of-way and the surrounding natural peatland vegetation.
Restoration Techniques
The Burial Under Peat Technique (BUPT) was applied on the 3-km road in the fall of 2012, a few months after the construction of the road. Half of the access road is in a treed peatland and the other half is in a Sphagnum-peatland, only the latter section is discussed in this paper (Supplementary Figure S2). The restoration work was performed with an excavator that operated exclusively over the surface of the access road to avoid additional disturbances and machinery sinking in the adjacent peatland. The following steps (Figure 2) were repeated in short segments equivalent to the reach of the excavator’s arm (approximately 5 m long in our case): 1) the mineral material, as well as the geotextile membrane and logs when present, were removed from the disturbed area and stockpiled on the road, behind the excavator (after visual evaluation of thickness of the road, in certain areas excessive mineral material has been removed off-site), 2) the underlying peat was excavated and placed beside the road on plywood boards or other types of easily movable platforms, thus creating a pit, 3) the road material stacked behind the excavator was transferred back into the pit, 4) the road material was covered with the excavated peat and the surface peat was flattened to the mean elevation of the surrounding peatland, 5) the peat surface was revegetated with diaspores harvested with the excavator’s bucket from the adjacent peatland, shredded with the bucket’s teeth to ensure it was spread uniformly, and finally, light pressure was applied to the reintroduced vegetal material with the bucket to favor better contact with the substrate. Once all the steps were completed, the excavator backed up and restarted the process on the next segment of the road.
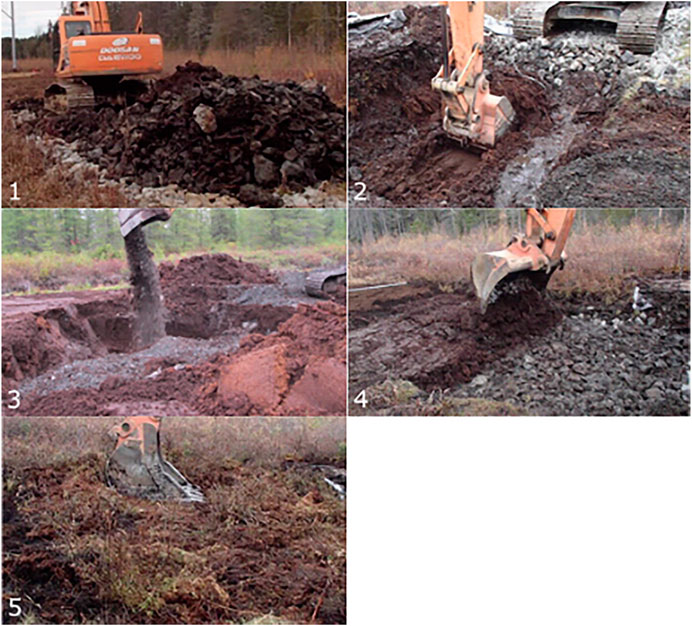
FIGURE 2. Steps of the Burial Under Peat Technique: 1) excavation and stockpiling of the mineral material, 2) excavation of the underlying peat, 3) filling the pit with the mineral material, 4) covering the mineral material with excavated peat and leveling the soil surface with the adjacent peatland, and 5) revegetating of the peat surface with diaspores from adjacent peatland.
During the restoration work, we aimed to establish a peat layer of at least 40 cm thick over the mineral material to ensure the construction material was buried in the catotelm under permanent anoxic conditions. The thickness of the acrotelm of the surrounding peatland should be assessed prior to restoration work so the threshold can be adjusted to site conditions. At this experimental site, a mean peat thickness of 52 cm was estimated to recover the foreign material. Furthermore, during restoration, the revegetation step, which is inspired by the Moss Layer Transfer Technique (MLTT; Graf and Rochefort 2016) was done at a ratio varying from 1:5 to 1:10, depending on the diaspores quality and availability in the adjacent peatland. The ratio refers to the area of the collection site to the area of the restoration site. For example, a ratio of 1:10 means that the collection site is ten times smaller than the restoration site. Drainage ditches were not blocked in the study site as they must be maintained for powerline maintenance and vegetation management.
Reference Ecosystems
Since the peatland of the right-of-way has been disturbed by the presence of drainage ditches and vegetation maintenance (tree cutting), it is necessary to consider the right-of-way undisturbed by the road as a target ecosystem, and not the surrounding pristine peatland. Thus, the reference ecosystem for the vegetation and elevation data is the part of the right-of-way undisturbed by the access road and pylons (Supplementary Figure S1). However, since we do not know the lateral diffusion distance of the chemical elements from the buried road and that this distance is potentially greater than the width of the right-of-way, it was essential to obtain chemistry data from an ecosystem uninfluenced by the road. For this reason, we consider the natural peatland surrounding the right-of-way as a reference ecosystem for the chemistry data (Supplementary Figure S2).
Water Chemistry
Peat pore water was sampled to measure physicochemical conditions within and around the restored strip. Two sampling campaigns took place 3 years post-restoration, in June and September 2015. Water was sampled across four transects, in piezometers collecting surface pore water in the acrotelm (depth of 20 cm) and catotelm pore water (depth of 50 cm) in the middle of the restored strip (0 m) and at different distances from the former edge of the road (0.5, 1, 2, and 10 m; Supplementary Figure S2). Water samples were also collected in piezometers in the surrounding pristine peatland outside of the right-of-way, which serves as a reference ecosystem dataset for the physicochemistry.
Water pH and electrical conductivity were measured directly in the field with a handheld device (Hanna instruments 98,129, Woonsocket, RI, United States). Electrical conductivity values were corrected according to pH and water temperature (Sjör, 1950). The concentration of inorganic N, P and S forms (N/NH4+, N/NO3−, total P, P/PO43− and S/SO42−), some major metallic cations (K, Mg, Ca, Na, and Al), two trace metals (Fe and Mn) and a major anion (Cl−) were analyzed in the laboratory. Thereupon, in this paper, we will refer to them using the generic term: chemical elements and components.
Fe, Ca, K, S/SO42−, Na, Mg, Mn, Al, and P/PO43− were extracted according to the method of Amacher et al. (1990). Total phosphorus was mineralized according to Parkinson and Allen (1975). The extracts were assayed by Inductively Coupled Plasma with optical emission spectrometry (ICP-OES, model 5,110 from Agilent Technologies, Santa Clara, CA, United States). The ammoniacal nitrogen and nitrate nitrogen were extracted according to the method used in Keeney and Nelson (1982) then dosed by continuous flow injection (FIA Quickchem 8500 Serie two from Lachat, Loveland, CO, United States) according to the standard method “Ammonia in surface water, wastewater” (Quikchem method 10-107-06-2-B) for N/NH4+ and according to the standard method ”Nitrate in 2M KCl soil extracts” (Quikchem method 12-107-04-1-F) for N/NO3−. Finally, the chlorides were extracted according to the method of Buykx et al. (2004) then dosed by continuous flow injection (FIA Quickchem 8500 Serie 2 from Lachat, Loveland, CO, United States) according to the method “Determination of chloride by flow injection analysis colorimetry” (Quikchem method 10-117-07-1-C). Certified reference materials (CRMs) were used to validate analytical measurement methods.
Surface Elevation
To verify if there was compression or decompression of the peat profile following the BUPT, the relative elevation of the restored surface was surveyed at two different times: after 1 year (in September) and 3 years (in August) post-restoration. Elevation was measured on three transects perpendicular to the former road (formerly four but one transect had to be eliminated since the reference stick was lost between the surveys), each covering the restored strip and the adjacent organic soil of the undisturbed right-of-way on both sides (Supplementary Figure S2), the latter being the reference ecosystem for elevation. Elevation was measured at 13 locations in the restored strip (every 20 cm) and 22 locations in the right-of-way (every 50 cm). The same sampling points were measured during each survey campaign, allowing data pairing. Relative elevation was measured with a leveling laser (LP410, Sokkia, Mississauga, ON, Canada). The concrete bases of nearby transmission towers were used as benchmarks.
Vegetation
To assess vegetation establishment following the restoration, the cover of each plant species was estimated 3 years post-restoration from their vertical projection on the ground, in 1 m × 1 m quadrats for vascular plants and in 25 cm × 25 cm quadrats for bryophyte species. Quadrats were randomly disposed in the area showed in Supplementary Figure S3. Eight quadrats of each type were surveyed over the restored road, as well as four quadrats of each type in the reference ecosystem (the adjacent undisturbed right-of-way).
Analyses
In Canada, from the month of July to September, the variations in concentration of most peatland chemical elements and compounds studied by Vitt et al. (1995) were little and stable, particularly for Sphagnum dominated peatlands (bog and poor fen). Consequently, data from water sampled in June, out of the snowmelt period, and in September were combined as one dataset. Descriptive analyses were used to evaluate the efficiency of the BUPT to confine chemical elements and compounds that could potentially be released by the mineral material, and to compare chemical conditions in and around the restored area with the natural reference ecosystem.
Paired t-tests were performed to evaluate if the elevations measured at the same location one winter and three years post-restoration were different. The elevation differences were then compared between the restored strip and the reference ecosystem with Welch’s t-test (independent means with unequal variances). Descriptive analyses were used to assess vegetation recovery on the restored strip in comparison to the reference ecosystem.
Results
Chemical Elements and Compounds
Figure 3 regroup the chemical elements and compounds not by ecological significance but by patterns of response.
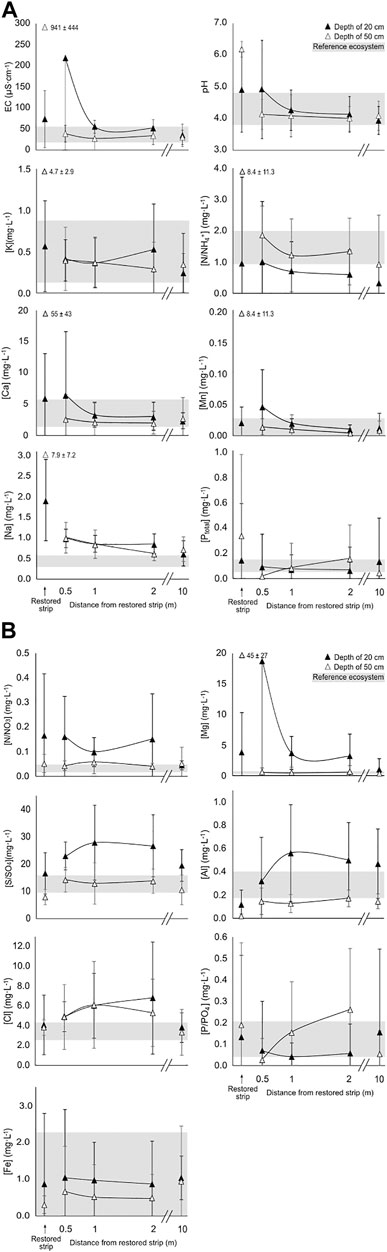
FIGURE 3. Mean pore water (±CI95%) of corrected electrical conductivity (EC), pH and concentration of K, N/NH4+, Ca, Mn, Na, Ptotal, N/NO3, Mg, S/SO4, Al, Cl, P/PO4 and Fe (n = 4, data combined from two sampling campaigns in June and September 2015) sampled at two depths (20 and 50 cm) in the restored strip and at 0.5, 1, 2, and 10 m from the restored strip. The gray area represents the mean of the reference ecosystem (nearby pristine peatland) values (mean ±CI95%, n = 4).
EC/pH Pattern of Response
The electrical conductivity (EC), proven to be a practical indicator for the other chemical elemental responses, summarizes the main findings regarding containment by the Burial Under Peat Technique for several elements [see Figure 3; the grey band represents the means values (mean ± CI95%) found in the reference ecosystem of the study]. Particularly high values of electrical conductivity (EC), compared to what is observed in the reference ecosystem, were found exclusively in the pore water sampled in the restored strip at a depth of 50 cm. For water pH, the mineral material buried in the peatland had only an alkalizing influence in the restored strip (20 and 50 cm deep) and at the close edge (half a meter) of the buried road for the surface water (20 cm deep). As suggested by the EC/pH patterns of response, K, N/NH4+, Ca, Mn, Na and Ptotal (Figure 3) showed particularly high concentrations exclusively in the pore water sampled at a depth of 50 cm in the restored strip; although slightly higher Na concentration were found up to 2 m at both depths.
Nitrate Pattern of Response
The NO3 pattern of response (N/NO3, Mg, S/SO4, Al and Cl; Figure 3) is characterized by catotelm samples (50 cm depth) with concentrations similar to that of the reference ecosystem over the entire length of the sampled transect, except for the water sampled at a depth of 50 cm in the restored strip which had particularly a high Mg concentration. The chloride (Cl) concentration of the water sampled in the catotelm (50 cm) was rather the same as for the surface samples (20 cm). The NO3 pattern of response also presented higher concentrations than in the reference ecosystem in surface water sampled up to a distance of 2 m, but back to the mean levels of the reference ecosystem at 10 m. However, the concentration of S/SO4, Al and Cl in the restored strip were similar to that observed in the reference ecosystem (the natural peatland surrounding the right-of-way).
Phosphate Pattern of Response
P/PO4 and Fe in the pore water sampled in the restored strip and at up to 10 m away from it (for both depth of 20 and 50 cm) had elemental concentration values similar to the means found in the reference ecosystem. Al and Cl, which were presented in the precedent pattern, could also figure in this pattern since the confidence interval (CI95%) at all distances and both depths are overlapping with the means of the reference ecosystem, suggesting there is no significant differences.
Elevation
Paired t-tests revealed that the elevation dataset 3-years post-restoration was significantly different than the dataset 1-yr post-restoration both in the restored strip and the reference ecosystem (Table 1). However, the differences in elevation (Δ elevation) between one winter following the restoration and 3-years post-restoration were similar between the restored strip and the reference ecosystem (Figure 4). However, the Δ elevation on T3 was statistically different (Welch t-test, p-value = 0.0049) between the restored strip (CI95% = −4.2 to −1.2 cm) and the adjacent reference ecosystem (CI95% = −6.5 to −4.5 cm) but not impactful considering the typical microtopography of hummocks and hollows in pristine peatlands (Nungesser, 2003).

TABLE 1. p value (95%) from paired t-test with datasets from 1-year and 3-years post-restoration [subsampling per transect: restored strip, n = 13; reference ecosystem (undisturbed peatland contiguous to the restored strip), n = 22].
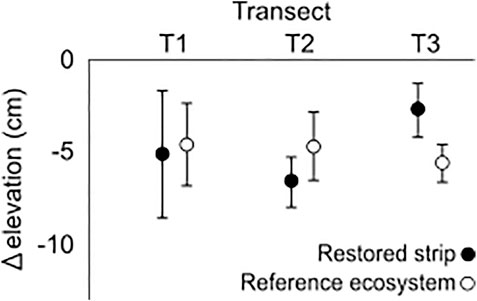
FIGURE 4. Mean elevation differences (±CI95%) 1 and 3 years post-restoration on the three transects [subsampling per transect: restored strip, n = 13; reference ecosystem (undisturbed peatland contiguous to the restored strip), n = 22].
Vegetation
Three years post-restoration, the bryophyte cover was low onto the restored strip (CI95% = 2–17%) in comparison to the reference ecosystem (CI95% = 94–100%). Nevertheless, the bryophyte cover in the restored strip was dominated by Sphagnum (S. rubellum, S. medium, S. angustifolium) species with the presence of three pioneer mosses often found in degraded peatland (Polytrichum strictum, Dicranella cerviculata and Pohlia nutans; Poulin et al., 1999; Poulin et al., 2005; Tuittila et al., 2000). Vegetation cover of the main plant strata found in the peatland differed between the restored strip and the reference ecosystem, except for trees and shrubs other than ericaceous species (Figure 5). However, species of this stratum were different between the restored strip (mostly Rubus hispidus) and the reference ecosystem (mostly Larix laricina, Betula populifolia and Aronia melanocarpa). The cover of ericaceous shrubs was high in the reference ecosystem (CI95% = 49–81%) but null in the restored strip. The herbaceous cover was high in the restored strip (CI95% = 29–53%) in comparison to the reference ecosystem (CI95% = 3–7%). Indeed, Scirpus cyperinus widely colonized entire sections of the restored strip. From the 22 vascular species surveyed in the restored strip, 20 are species found in wetlands [ten are Obligate Wetland (two of them mostly occur in peatland), six are Facultative Wetland, three are Facultative and one is Facultative Upland;.
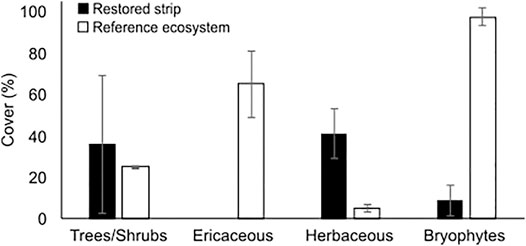
FIGURE 5. Mean total cover of the main vegetation strata (±CI95%) three years post-restoration in the restored strip (n = 8) and the reference ecosystem (undisturbed peatland contiguous to the restored strip, n = 4).
Discussion
The Burial Under Peat Technique (containment under at least 40 cm of peat) proved to limit the enrichment of the usual chemical signature of peatland from elements that could have diffused from the foreign mineral material brought onto the site for the temporary road construction, which in turn could have caused changes in the vegetation composition. Furthermore, the elevation of the surface of the restored strip was stable over the course of this study and supported the establishment of typical peatland vegetation.
Chemical Elements and Compounds Containment by the BUPT
The Burial Under Peat Technique is an effective method to limit the contamination of the peatland by the chemical elements and compounds released by the mineral material. The concentration of most of the chemical elements and compounds measured in the surface water (20 cm deep) of the restored strip was within or near the reference ecosystem means. This indicates that three years post-restoration, there was no vertical migration of the buried chemical elements and compounds toward the surface with the water table variations. Knowing that the lowest water table depth measured was approximately 30 cm (Figure 1), the mineral material buried at 50 cm deep was thus completely comprised in the catotelm, i.e., the bottom layer of peatlands that is permanently below the water table. Therefore, lateral diffusion of chemical elements and compounds from the buried mineral should be negligible in the long term due to the particular properties of the peat (anisotropy, hydraulic conductivity linked to the degree of peat decomposition and the high-buffering capacity due to the cation exchange capacity).
The surface water (depth of 20 cm) had high concentrations of magnesium, nitrates, and sulfates in the restored strip and within at least the first 2 m from it. However, this could also be a residual effect of the presence of the road before the restoration work since the concentrations of chemical elements and compounds within the surface water at a distance of 10 m from the road were the same as in the reference ecosystem. High nitrate and sulfate concentrations may be explained as a side effect of the peat inversion during the BUPT, accentuated by a low water table. Indeed, during the restoration, the peat that was historically in anaerobic conditions, from the long-term peatland development, was suddenly exposed to oxygen. It was then possible for erobic bacteria to oxidize ammoniacal nitrogen into nitrite and then nitrate through the nitrification process (Regina et al., 1996; Limpens et al., 2006). The reoxidation of elemental sulfur and sulfides, that occurs when the water table is low, generates sulfate ions that are dissolved when the water table level rises again (Bayley et al., 1986; Eimers et al., 2003), which might explain the higher concentration of sulphates found. Overall, we consider the physicochemistry of the restored strip and its margins adequate to support and maintain typical bog vegetation. In the longer term, it would be of interest to monitor the efficiency of the BUPT to confine nutrients. However, considering peatland ecology and its resilience, physicochemical conditions are expected to be stable through time.
Substrate Elevation After the BUPT
There was no compression of the peat in the restored strip following the restoration with the BUPT over the course of this study. The elevation differences between year 1 and 3 after the restoration work were similar to that in the restored strip and the reference ecosystem. During the restoration, the leveling of the peat to a similar mean elevation as the reference ecosystem with the excavator’s bucket was sufficient to avoid drastic topographic changes in the restored strip. Rigorous daily surveillance of the work may have played a role in achieving satisfying surface elevation. The general lowering of 5 cm between survey on both the restored strip and the reference ecosystem may be an effect of bog surface oscillation (Howie and Hebda, 2018). The goal to restore and maintain a substrate elevation similar to the surrounding peatland was thus achieved.
Revegetation failures in road impacted peatland restoration projects are frequently caused either by extended flooding created by a low surface elevation (Osko, 2015) or by dry substrates caused by high elevations and excessive drainage (Johansen et al., 2017). Since the restored site has already gone through three winters, allowing the substrate to settle under the effect of precipitations and the weight of the snow, no significant changes in the elevation of the restored strip are expected in the future. Although previous studies might have suggested creating a dome-shaped surface over the restored area to compensate for possible substrate compaction, our study shows that there is no need to do so. Doing so could create drier conditions and compromise the survival of the reintroduced vegetation during the critical period of the first growing seasons (Chirino et al., 2006).
Revegetation
The revegetation method used during BUPT successfully led to the reintroduction of plant species typical of wetlands, as only a few species with low cover were non-wetland species. However, 3 years post-restoration the plant communities of the restored strip did not evolve toward the reference ecosystem. The low establishment of peatland species in the restored strip may be explained by the presence of active drainage ditches maintaining a low water table within the right-of-way and inadequate donor material. A straw application was shown to be a critical step during peatland restoration with the MLTT (Rochefort et al., 2003) to prevent diaspores desiccation and to create favorable microclimatic conditions for plant establishment during the first growing seasons (Price et al., 1998). However, straw application was set aside during the BUPT to minimize costs and circulation over the restored area.
In contrast, results for the revegetation of a peatland impacted by a mineral road in Chénéville, Québec (Canada) where drainage ditches were absent, showed an extremely fast establishment of peatland plant communities only one year following the restoration (Sphagnum cover CI95% = 14–52%; Pouliot, 2018) even without a straw mulch application. The authors believe that the combination of an adequate substrate elevation, a high water table due to the absence of drainage, good diaspores quality and constant work supervision led to this success in this other study (Pouliot, 2018).
Resources
The BUPT is cost-effective compared to the complete removal of the road mineral material because it requires fewer resources as well as fewer workers and heavy machinery, no peat input, and minimal mineral material transport out of the site. The most important expense linked to the complete removal technique is the transportation of the mineral out of the site (the further away the dumping site, the more expensive it is) and to the importation of peat onto the site. Furthermore, the purchase of the peat entails significant costs. The BUPT has minimal needs in machinery, such as a backhoe, and the absence of peat purchase and transportation makes it a technique of choice for the restoration of peatlands impacted by long linear mineral roads, especially in hard-to-access or remote areas. Besides, the time taken to complete both techniques on a 60 m road was estimated to be similar (∼3 linear meters per hour) in another project described in Pouliot (2018). At Sainte-Eulalie, the BUPT had a pace of about 4.5 linear meters per hour.
However, the use of the BUPT is limited by the depth of the underlying peat. The minimum peat thickness required to apply the BUPT is approximately 1 m. In peatlands where the peat depth is insufficient, complete or partial removal of the road is recommended, depending on the thickness of the mineral material.
Conclusion
The restoration of peatlands impacted by mineral linear disturbances is at an early stage of development, but this study presents a new effective, innovative and affordable technique. Indeed, 3-years post-restoration results suggest that the Burial Under Peat Technique limits the peatland contamination by confining the chemical elements and compounds of the mineral material, and by establishing and maintaining an organic surface of similar elevation than the surrounding peatland without subsequent compaction or decompaction. Applying the principles of the long-proven Moss Layer Transfer Technique, but without the straw mulch application and the fertilization steps, is an indispensable step of the BUPT. The low moss cover observed in our study site three years following the restoration reminds us of the importance to re-establish a high water table and to transfer adequate plant material (Quinty and Rochefort, 2003). Finally, because the BUPT application requires a certain level of meticulousness that makes a difference in the achievement of restoration goals, we stress the capital importance of constant site supervision to adjust the working method and apply corrective measures when needed.
Data Availability Statement
The raw data supporting the conclusions of this article will be made available by the authors, without undue reservation.
Author Contributions
LR conceived the experiments. ML, KP, and AB were involved in planning and supervised the work. KP performed the measurements. KP processed the experimental data, performed the analysis, drafted the manuscript and designed the figures. MN aided with the statistics and worked on the manuscript. All authors discussed the results and commented on the manuscript.
Funding
Financial support for this study was provided by Hydro-Québec. Financial support to write this paper was provided by the Natural Sciences and Engineering Research Council of Canada (CRDPJ 517951-17) and the Canadian Sphagnum Peat Moss Association. Funds for open access publication fees were provided by Hydro-Québec.
Conflict of Interest
The authors declare that the research was conducted in the absence of any commercial or financial relationships that could be construed as a potential conflict of interest.
Acknowledgments
Financial support for this study was provided by Hydro-Québec. We thank Geneviève Corfa, Ghislain St-Laurent, Marie-Ève Pelletier-Marion and Caroline Dubé from Hydro-Québec who made this project possible and the members of the Golder Associates team for onsite supervision. Financial support to write this paper was provided by the Natural Sciences and Engineering Research Council of Canada and the Canadian Sphagnum Peat Moss Association. Special thanks to Sandrine Hogue-Hugron and Claire Boismenu from the Peatland Ecology Research Group for their help and support.
Supplementary Material
The Supplementary Material for this article can be found online at: https://www.frontiersin.org/articles/10.3389/feart.2021.658470/full#supplementary-material
References
Amacher, M. C., Henderson, R. E., Breithaupt, M. P., Seale, C. L., and La Bauve, J. M. (1990). Unbuffered and Buffered Salt Methods for Exchangeable Cations and Effective Cation-Exchange Capacity. Soil Sci. Soc. Am. J. 54 (8), 1036–1042. doi:10.2136/sssaj1990.03615995005400040018x
Bagley, S. (1998). The Road-Ripper’s Guide to Wildland Road Removal. Missoula, MT: Wildlands Center for Preventing Roads.
Bates, J. W. (2009). “Mineral Nutrition and Substratum Ecology,” in Bryophyte Biology. Editors B. Goffinet, and A.J. Shaw 2nd edition (Cambridge, United Kingdom: Cambridge University Press), 248–311.
Bayley, S. E., Behr, R. S., and Kelly, C. A. (1986). Retention and Release of S from A Freshwater Wetland. Water Air Soil Pollut. 31, 101–114. doi:10.1007/bf00630824
Beckwith, C. W., Baird, A. J., and Heathwaite, A. L. (2003). Anisotropy and Depth-Related Heterogeneity of Hydraulic Conductivity in a Bog Peat. I: Laboratory Measurements. Hydrol. Process. 17, 89–101. doi:10.1002/hyp.1116
Bocking, E., Cooper, D. J., and Price, J. (2017). Using Tree Ring Analysis to Determine Impacts of a Road on a Boreal Peatland. For. Ecol. Manag. 404, 24–30. doi:10.1016/j.foreco.2017.08.007
Bourgeois, B., Hugron, S., and Poulin, M. (2012). Establishing a moss Cover Inhibits the Germination of Typha Latifolia, an Invasive Species, in Restored Peatlands. Aquat. Bot. 100, 76–79. doi:10.1016/j.aquabot.2012.03.010
Buykx, S. E. J., van den Hoop, M. A. G. T., and de Joode, P. (2004). Simultaneous Extraction of Bromide, Chloride, Fluoride and Sulfate from Soils, Waste- and Building Materials. J. Environ. Monitor. 6, 552–558. doi:10.1039/b400378k
Chirino, C., Campeau, S., and Rochefort, L. (2006). Sphagnum Establishment on Bare Peat: The Importance of Climatic Variability and Sphagnum Species Richness. Appl. Veg Sci. 9, 285–294. doi:10.1658/1402-2001(2006)9[285:seobpt]2.0.co;2
Colmer, T. D. (2003). Long-distance Transport of Gases in Plants: A Perspective on Internal Aeration and Radial Oxygen Loss from Roots. Plant Cel Environ 26, 17–36. doi:10.1046/j.1365-3040.2003.00846.x
Couillard, L., and Grondin, P. (1986). La végétation des milieux humides du Québec. Montreal: Les Publications du Québec, 400.
de Mars, H., Wassen, M. J., and Peeters, W. H. M. (1996). The Effect of Drainage and Management on Peat Chemistry and Nutrient Deficiency in the Former Jegrznia-Floodplain (NE-Poland). Vegetatio 126, 59–72.
Eimers, C., Dillon, P. J., Schiff, S. L., and Jeffries, D. S. (2003). The Effects of Drying and Re-wetting and Increased Temperature on Sulphate Release from upland and Wetland Material. Soil Biol. Biochem. 13 (12), 1663–1673. doi:10.1016/j.soilbio.2003.08.013
Gignac, L. D., Vitt, D. H., and Bayley, S. E. (1991). Bryophyte Response Surfaces along Ecological and Climatic Gradients. Vegetatio 93, 29–45.
Gignac, L. D., Gauthier, R., Rochefort, L., and Bubier, J. (2004). Distribution and Habitat Niches of 37 Peatland Cyperaceae Species across a Broad Geographic Range in Canada. Can. J. Bot. 82, 1292–1313. doi:10.1139/b04-081
Government of Canada (2018). Canadian Climate Normals 1981–2010 Station Data. Temperature and Precipitation Graph for 1981 to 2010 Canadian Climate Normals of ST WENCESLAS and Cheneville. Available at: http://climat.meteo.gc.ca/climate_normals/results_1981_2010_f.html?stnID=5586&autofwd=1http://climat.meteo.gc.ca/climate_normals/results_1981_2010_e.html?stnID=5522&autofwd=1 (Accessed April 16, 2017).
Graf, M. D., and Rochefort, L. (2016). “A Conceptual Framework for Ecosystem Restoration Applied to Industrial Peatlands,” in Peatland Restoration and Ecosystem Services: Science, Policy and Practice. Editors A. Bonn, T. Allott, M. Evans, H. Joosten, and R. Stoneman (Cambridge: Ecological Reviews of Cambridge University Press), 192–212.
Hamilton, J. D., Kelly, C. A., Rudd, J. W. M., Hesslein, R. H., and Roulet, N. T. (1994). Flux to the Atmosphere of CH4and CO2from Wetland Ponds on the Hudson Bay Lowlands (HBLs). J. Geophys. Res. 99, 1495–1510. doi:10.1029/93jd03020
Hogg, P., Squires, P., and Fitter, A. H. (1995). Acidification, Nitrogen Deposition and Rapid Vegetational Change in a Small valley Mire in Yorkshire. Biol. Conservation 71 (2), 143–153. doi:10.1016/0006-3207(94)00040-w
Howie, S. A., and Hebda, R. J. (2018). Bog Surface Oscillation (Mire Breathing): A Useful Measure in Raised Bog Restoration. Hydrological Process. 32 (11), 1518–1530. doi:10.1002/hyp.11622
Johansen, M. D., Aker, P., Klanderud, K., Olsen, S. L., and Skrindo, A. B.(2017). Restoration of Peatland by Spontaneous Revegetation after Road Construction. Appl. Veg. Sci. 20 (4), 631–640.
Justin, S. H. F. W., and Armstrong, W. (1987). The Anatomical Characteristics of Roots and Plant Response to Soil Flooding. New Phytol. 106, 465–495. doi:10.1111/j.1469-8137.1987.tb00153.x
Keeney, D. R., and Nelson, D. W. (1982). “Nitrogen-inorganic Forms,” in Methods of Soil Analysis, Part 2. Editors A.L. Page, R.H Miller, and D.R. Keeney. 2nd Ed. (Madison, Wisconsin: American Society of Agronomy, Inc., and Soil Science Society of America, Inc.), 643–698.
Kooijman, A. M., and Bakker, C. (1995). Species Replacement in the Bryophyte Layer in Mires: The Role of Water Type, Nutrient Supply and Interspecific Interactions. J. Ecol. 83 (1), 1–8. doi:10.2307/2261145
Laiho, R., Vasander, H., Penttilä, T., and Laine, J. (2003). Dynamics of Plant-Mediated Organic Matter and Nutrient Cycling Following Water-Level Drawdown in Boreal Peatlands. Glob. Biogeochem. Cycles 17 (2), 111–119. doi:10.1029/2002GB002015
Letts, M. G., Roulet, N. T., Comer, N. T., Skarupa, M. R., and Verseghy, D. L. (2000). Parametrization of Peatland Hydraulic Properties for the Canadian Land Surface Scheme. Atmosphere-Ocean 38, 141–160. doi:10.1080/07055900.2000.9649643
Limpens, J., Granath, G., Gunnarsson, U., Aerts, R., Bayley, S., Bragazza, L., et al. (2011). Climatic Modifiers of the Response to Nitrogen Deposition in Peat‐forming Sphagnum Mosses: a Meta‐analysis. New Phytol. 191 (2), 496–507. doi:10.1111/j.1469-8137.2011.03680.x
Limpens, J., Heijmans, M. P. D., and Berendse, F. (2006). “The Nitrogen Cycle in Boreal Peatlands,” in Boreal Peatlands Ecosystems. Ecological Studies, Vol. 188. Editors K. R Wieder, and D.H. Vitt (Berlin, Germany: Springer-Verlag), 195–230.
Luce, C. H. (1997). Effectiveness of Road Ripping in Restoring Infiltration Capacity of forest Roads. Restor Ecol. 5 (3), 265–270. doi:10.1046/j.1526-100x.1997.09731.x
McDonald, T., Gann, G. D., Jonson, J., and Dixon, K. W. (2016). International Standards for the Practice of Ecological Restoration – Including Principles and Key Concepts. Washington, D.C: Society for Ecological Restoration.
Miller, C. A. (2011). The Effect of Long-Term Drainage on Plant Community Composition, Biomass, and Productivity in Boreal continental Peatlands. Guelph, Ontario: MSc thesis, University of Guelph.
Müllerová, J., Vítková, M., and Vítek, O. (2011). The Impacts of Road and Walking Trails upon Adjacent Vegetation: Effects of Road Building Materials on Species Composition in a Nutrient Poor Environment. Sci. Total Environ. 409 (19), 3839–3849. doi:10.1016/j.scitotenv.2011.06.056
Nungesser, M. K. (2003). Modelling Microtopography in Boreal Peatlands: Hummocks and Hollows. Ecol. Model. 165 (2–3), 175–207. doi:10.1016/s0304-3800(03)00067-x
Osko, T. (2015). Peatland Road Reclamation: Issues and Considerations Associated with Deep Fill. Calgary, Alberta: Durvile Publications Ltd.
Osko, T., Vogel, C., Pilon, J., Petrone, R., La Farge, C., Williams, K., et al. (2014). Natural Restoration of Peat and Re-usability of Fill Material after Road Reclamation on a Fen. Peace River, Alberta: Durvile Publications Ltd.
Parkinson, J. A., and Allen, S. E. (1975). A Wet Oxidation Procedure Suitable for the Determination of Nitrogen and mineral Nutrients in Biological Material. Commun. Soil Sci. Plant Anal. 6 (1), 1–11. doi:10.1080/00103627509366539
Pasher, J., Seed, E., and Duffe, J. (2013). Development of Boreal Ecosystem Anthropogenic Disturbance Layers for Canada Based on 2008 to 2010 Landsat Imagery. Can. J. Remote Sensing 39 (1), 42–58. doi:10.5589/m13‐007
Pilon, J. (2015). Characterization of the Physical and Hydraulic Properties of Peat Impacted by a Temporary Access Road. Ontario: MSc thesis, University of Waterloo.
Plach, J. M., Wood, M. E., Macrae, M. L., Osko, T. J., and Petrone, R. M. (2017). Effect of a Semi‐permanent Road on N, P, and CO2 Dynamics in a Poor Fen on the Western Boreal Plain, Canada. Ecohydrol 10 (7), e1874. doi:10.1002/eco.1874
Poulin, M., Rochefort, L., and Desrochers, A. (1999). Conservation of Bog Plant Species Assemblages: Assessing the Role of Natural Remnants in Mined Sites. Appl. Veg. Sci. 2, 169–180. doi:10.2307/1478980
Poulin, M., Rochefort, L., Quinty, F., and Lavoie, C. (2005). Spontaneous Revegetation of Mined Peatlands in Eastern Canada. Can. J. Bot. 83, 539–557. doi:10.1139/b05-025
Pouliot, K. (2018). Les routes minérales en tourbières à sphaignes : restauration par enfouissement. Québec: Mémoire M.Sc., Université Laval, 68.
Price, J., Rochefort, L., and Quinty, F. (1998). Energy and Moisture Considerations on Cutover Peatlands: Surface Microtopography, Mulch Cover and Sphagnum Regeneration. Ecol. Eng. 10, 293–312. doi:10.1016/s0925-8574(98)00046-9
Puustjärvi, V. (1956). On the Cation Exchange Capacity of Peats and on the Factors of Influence upon its Formation. Acta Agriculturae Scand. 6 (4), 410–449. doi:10.1080/00015125609438024
Quinty, F., and Rochefort, L. (2003). Peatland Restoration Guide. Second Edition. Québec, Canada: Canadian Sphagnum Peat Moss Association and New Brunswick Department of Natural Resources and Energy.
Regina, K., Nykänen, H., Silvola, J., and Martikainen, P. J. (1996). Fluxes of Nitrous Oxide from Boreal Peatlands as Affected by Peatland Type, Water Table Level and Nitrification Capacity. Biogeochemistry 35, 401–418. doi:10.1007/bf02183033
Rochefort, L., Quinty, F., Campeau, S., Johnson, K., and Malterer, T. (2003). North American Approach to the Restoration of Sphagnum Dominated Peatlands. Wetl. Ecol. Manag. 11 (1–2), 3–20. doi:10.1023/a:1022011027946
Saraswati, S., Bhusal, Y., Trant, A. J., and Strack, M. (2020). Roads Impact Tree and Shrub Productivity in Adjacent Boreal Peatlands. Forests 11 (5), 594. doi:10.3390/f11050594
Saraswati, S., and Strack, M. (2019). Road Crossings Increase Methane Emissions from Adjacent Peatland. J. Geophys. Res. Biogeosci. 124 (11), 3588–3599. doi:10.1029/2019jg005246
Sjörs, H., and Sjors, H. (1950). On the Relation between Vegetation and Electrolytes in north Swedish Mire Waters. Oikos 2, 241–257. doi:10.2307/3564795
Sobze, J.-M., Rochefort, L., and Schoonmaker, A. (2012). Wellsite clay Pad Removal and Inversion Peatland Restoration. Can. Reclamation 12 (1), 10–13. doi:10.3368/er.34.3.225
Sosa-Pérez, G., and MacDonald, L. H. (2017). Reductions in Road Sediment Production and Road-Stream Connectivity from Two Decommissioning Treatments. For. Ecol. Manag. 398 (8), 116–129. doi:10.1016/J.FORECO.2017.04.031
Strack, M., and Zuback, Y. C. A. (2013). Annual Carbon Balance of a Peatland 10 Yr Following Restoration. Biogeosciences 10, 2885–2896. doi:10.5194/bg-10-2885-2013
Switalski, T., Bissonette, J., DeLuca, T., Luce, C., and Madej, M. (2004). Benefits and Impacts of Road Removal. Front. Ecol. Environ. 2 (1), 21–28. doi:10.1890/1540-9295(2004)002[0021:baiorr]2.0.co;2
Tuittila, E.-S., Rita, H., Vasander, H., and Laine, J. (2000). Vegetation Patterns Around Eriophorum Vaginatum L. Tussocks in a Cut-Away Peatland in Southern Finland. Can. J. Bot. 78 (1), 47–58. doi:10.1139/b99-159
Umeda, Y., Tsujii, T., and Inoue, T. (1985). Influence of Banking on Groundwater Hydrology in Peatland. J. Fac. Agric. Hokkaido Univ. 62 (3), 222–235.
Vitt, D. H., Bayley, S. E., and Jin, T.-L. (1995). Seasonal Variation in Water Chemistry over a Bog-Rich Fen Gradient in continental Western Canada. Can. J. Fish. Aquat. Sci. 52 (3), 587–606. doi:10.1139/f95-059
Wieder, R. K., Vitt, D. H., and Benscoter, B. W. (2006). “Peatlands and the Boreal forest,” in Boreal Peatland Ecosystems (Berlin, Heidelberg: Springer), 1–8.
Williams-Mounsey, J., Grayson, R., Crowle, A., and Holden, J. (2021). A Review of the Effects of Vehicular Access Roads on Peatland Ecohydrological Processes. Earth-Science Rev. 214, 103528. doi:10.1016/j.earscirev.2021.103528
Keywords: peatland restoration, decommissioning, bog, road, reclamation
Citation: Pouliot K, Rochefort L, LeBlanc M-C, Guêné-Nanchen M and Beauchemin A (2021) The Burial Under Peat Technique: An Innovative Method to Restore Sphagnum Peatlands Impacted by Mineral Linear Disturbances. Front. Earth Sci. 9:658470. doi: 10.3389/feart.2021.658470
Received: 25 January 2021; Accepted: 31 May 2021;
Published: 11 June 2021.
Edited by:
Samuel Abiven, University of Zurich, SwitzerlandReviewed by:
Rod Chimner, Michigan Technological University, United StatesSteve Pratte, Zhejiang University, China
Copyright © 2021 Pouliot, Rochefort, LeBlanc, Guêné-Nanchen and Beauchemin. This is an open-access article distributed under the terms of the Creative Commons Attribution License (CC BY). The use, distribution or reproduction in other forums is permitted, provided the original author(s) and the copyright owner(s) are credited and that the original publication in this journal is cited, in accordance with accepted academic practice. No use, distribution or reproduction is permitted which does not comply with these terms.
*Correspondence: Line Rochefort, TGluZS5Sb2NoZWZvcnRAZnNhYS51bGF2YWwuY2E=