- 1State Key Laboratory of Hydraulics and Mountain River Engineering, Sichuan University, Chengdu, China
- 2College of Water Resource and Hydropower, Sichuan University, Chengdu, China
Characterized by large scale, high frequency, and strong destructiveness, debris flow has become the most noticeable geohazards throughout the world, especially in the mountainous areas of southwestern China. On August 20, 2019, large-scale heavy rainfall pummeled Wenchuan County, Sichuan Province, Southwestern China, which resulted in a cluster of debris flows (the “8·20” clustered debris flows event), and caused considerable economic losses (approximately 3.4 billion RMB were lost) and heavy casualties (48,862 people were displaced, 16 people died and 22 people went missing). Based on field investigation, image data interpretation, mechanism analysis, and other methods, this study reveals the formation mechanism, dynamic evolutionary process, and impacts of human activities on the “8·20” clustered debris flows event. Results from a comprehensive analysis indicate that the occurrence of short-term, high-intensity rainfall and the excessive supply of solid material were the main factors that triggered this catastrophic event. With the debris flow flowing into the main river, this event presented an extremely apparent disaster chain effect. It is also found that improper site selection and inadequate design of human activities played a crucial role in the movement process of the debris flow that directly aggravated the losses. Finally, to improve debris flow prediction and prevention, some early warning and mitigation measures are discussed.
Introduction
Debris flow occurs when masses of poorly sorted sediment, agitated and saturated with water, surge down slopes in response to gravitational attraction (Iverson, 1997). In recent years, heavy economic losses and casualties, together with serious damage to the environment caused by debris flow, have become a social issue of great concern around the world. This is especially the case for the mountainous areas of southwestern China, where approximately 15,797 debris-flow ravines have been identified (Cui et al., 2003). On May 12, 2008, a devastating earthquake (Ms 8.0) occurred in Wenchuan County, Sichuan Province, Southwest China (Huang and Li, 2009). More than 56,000 landslides with a total area of 811 km2 were triggered by the earthquake (Dai et al., 2011), leaving a vast amount of loose material on steep hill slopes or in channels (Koi et al., 2008; Dong et al., 2009; Parker et al., 2011), as shown in Figure 1. It is confirmed that the frequency and magnitude of local debris flow increased significantly after the 2008 Wenchuan earthquake due to the availability of loose solid material (Tang et al., 2009; Cui et al., 2011; Chen et al., 2013). For instance, immediately following the 2008 Wenchuan earthquake, 837 debris flows occurred in the 42 earthquake-hit counties of Sichuan Province (Huang and Li, 2009).
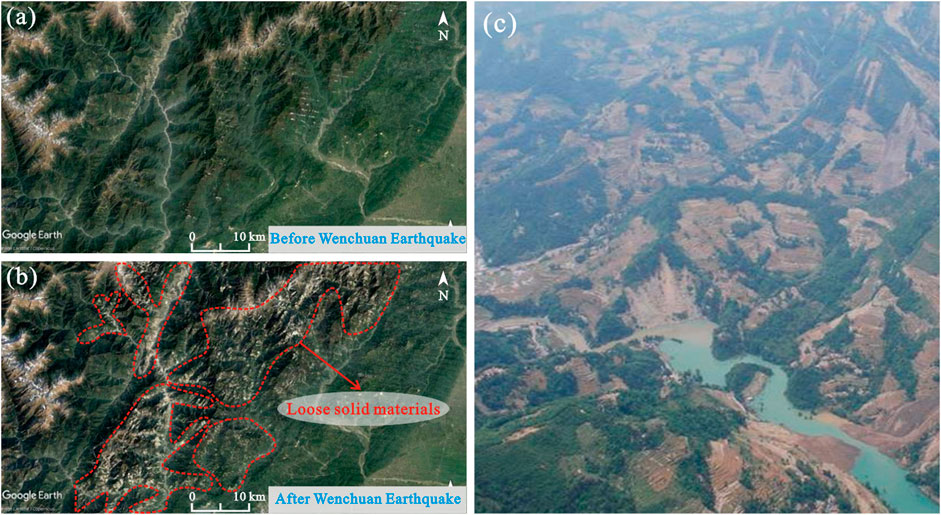
FIGURE 1. Source conditions of loose solid materials in mountainous areas of southwestern China: (A) satellite image of the southwestern mountainous area before the Wenchuan earthquake; (B) satellite image of the southwestern mountainous area after the Wenchuan earthquake; and (C) typical topographic and geomorphologic conditions of the southwestern mountainous area.
In general, the triggering of debris flow is inseparable from three essential factors: sufficient available loose material, generation of an ample amount of surface run-off, and steepness of the drainage channels on the slopes (Takahashi, 1981). This finding hints at the important relationship between rainfall threshold and sediment supply conditions in the formation of debris flow. Based on field investigation and statistical analyses, it was found that triggering rainfall thresholds, such as cumulative antecedent rainfall and 1-h rainfall intensity, for debris flow decreased significantly after strong earthquake events (Chen et al., 2009; Shieh et al., 2009; Tang et al., 2009; Yu et al., 2014), which indicates that debris flow more easily formed during heavy rainstorm events. Some studies have emphasized that the conditions for debris flow directly caused by considerable loose material, which was produced in the 2008 Wenchuan earthquake, will remain active for 20–30 years (Cui et al., 2008; Cui et al., 2009; Xie et al., 2009; Huang and Fan, 2013).
Threats of debris flow disasters compel people to undertake engineering measures to control their occurrence and to rein in their formation, circulation, and accumulation areas. Even though a large amount of money and time has been invested, the occurrence of catastrophic events has proven that some previous preventative engineering measures constructed in debris flow gullies have not fulfilled their expected role of control, but instead have magnified the scale of the disaster (Wang et al., 2012; Wang, 2013; Chen et al., 2015). One reason for this is that an excess supply of loose solid material was not sufficiently considered (Chen et al., 2019), causing the projects to be unable to prevent and control the extraordinary scale of the debris flow in the earthquake area.
In this paper, the “8·20” clustered debris flows event that occurred in Wenchuan County is selected as a case study to understand the formation mechanism, dynamic evolutionary process, and impacts from human activities. First, the geomorphologic and geological, hydrological, and climatic conditions that are prone to debris flow in Wenchuan County are presented. Second, the triggering mechanism from high-intensity rainfall and the dynamic evolution of debris flow under an excessive supply of solid material are analyzed. Third, the effects of human engineering activities and the disaster-causing characteristics of the “8·20” clustered debris flows event are summarized. Finally, aiming at improving debris flow prevention, we discuss some early warning and mitigation measures.
Study Area
Wenchuan County is located in the middle of Sichuan Province, southeast of the Tibetan Qiang Autonomous Prefecture of Ngawa, and on the northwestern edge of the Sichuan Basin (Figure 2A). The county is bounded by 30°45′ and 31°43′ north latitude and 102°51′ and 103°44′ east longitude. The total area of Wenchuan County is 4,084 km2 and its permanent population was 102,500 at the end of 2018.
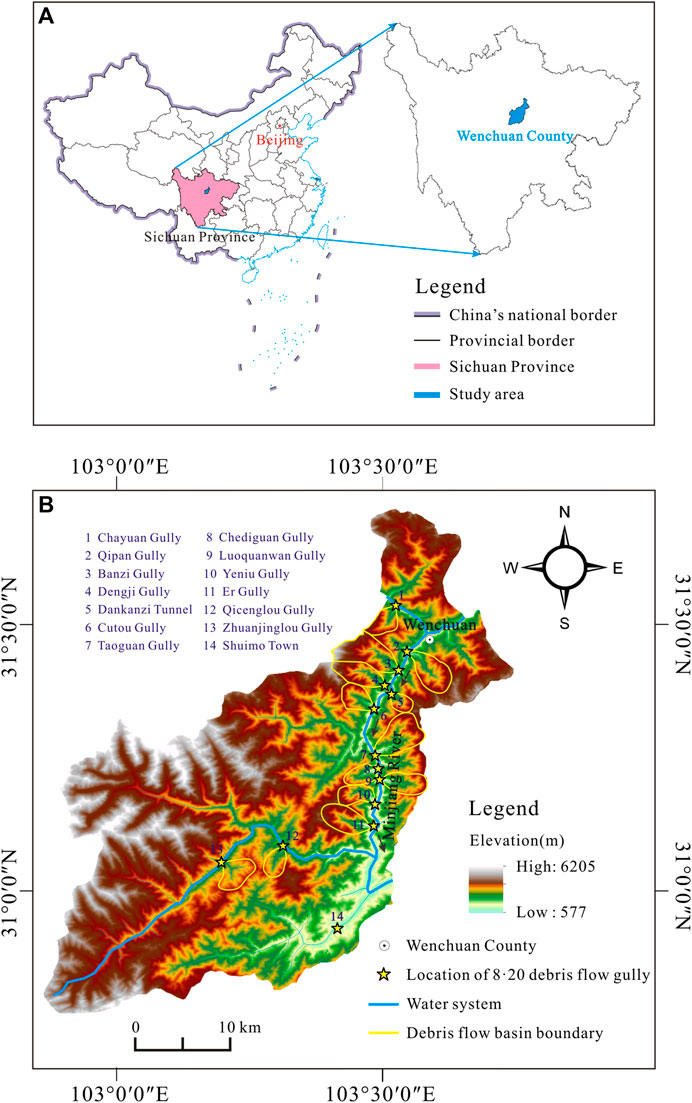
FIGURE 2. Site location of the study area: (A) the location of the study area in China; and (B) the geomorphic map of the study area and the distribution of debris flows in the “8·20” event.
The “8·20” Clustered Debris Flows Event
On August 20, 2019, Wenchuan County suffered large-scale heavy rainfall that caused a cluster of debris flow disasters (Figure 2B). All villages and towns in the territory were affected to varying degrees. A large number of houses were destroyed in the area through which the debris flow moved and some sections of multiple important roads (such as Duwen Expressway and National Highways G213, 317, and 350) were blocked or interrupted. Worse still, several hydropower stations were seriously damaged (Figure 3). Furthermore, some debris flows transported large amounts of solid material into the Minjiang River thereby causing a partial blockage or forming a debris dam, resulting in a series of secondary disasters (Figure 3B). This event caused substantial economic losses (approximately 3.4 billion RMB) and heavy casualties (48,862 people were displaced, 16 people died, and 22 people went missing).
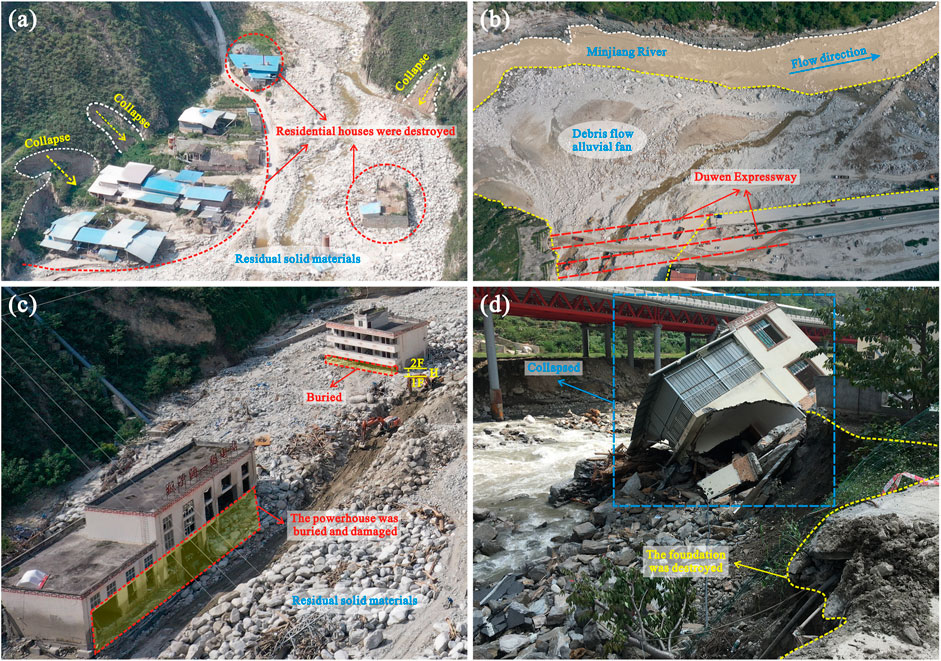
FIGURE 3. Damage caused by debris flows in the “8·20” event: (A) buried houses in Banzi Gully; (B) the blocked Minjiang River and destroyed traffic facilities at the mouth of the Dengji Gully; (C) damage to the Banzi Gully Grade I Hydropower Station; and (D) destroyed buildings along the Minjiang River.
Geomorphologic and Geological Conditions
Wenchuan County is located in the transitional mountainous belt between the Qinghai–Tibetan Plateau and West Sichuan Plain, which is dominated by high and middle mountains; the northeast contains the Longmen Mountains, while the southwest contains the Qionglai Mountains. Elevation gradually increases from the southeast to the northwest, as shown in Figure 2, and the elevation of the mountains is approximately 4,000 m. The terrain of Wenchuan County is complex with high mountains, steep slopes, and deep valleys. This topography is extremely conducive to the accumulation of loose solid material and the collection of surface runoff; moreover, it provides favorable topographic conditions for the rapid formation of debris flow.
The geological conditions of Wenchuan County are complicated. As a result of the extrusion of the Qinghai-Tibet Plateau and the Sichuan Basin, the Longmenshan fault region has always been active, resulting in a high frequency of strong earthquakes. The Yingxiu–Beichuan fault zone, belonging to the main central fault zone of Longmenshan, runs through the southeastern part of the study area and is inferred to be the origin of the Wenchuan earthquake that occurred on May 12, 2008 (Li et al., 2008; Xie et al., 2009). A high frequency of earthquakes significantly worsened the geological conditions of Wenchuan County, causing extensive collapses and landslides. Many geologic units are present in Wenchuan County, with outcrops that are Ediacaran to Quaternary in age. Surficial rocks are highly weathered and commonly contain fissures of different sizes. In addition, loose unconsolidated Quaternary deposits and strongly weathered magmatic rocks are widely distributed in the form of terraces and alluvial fans. Consequently, a tremendous amount of loose solid material has accumulated on the hill slopes and in the gullies, providing an abundant material supply for the formation of debris flow.
Hydrological and Climatic Conditions
The primary river system in Wenchuan County is the Minjiang River, an important tributary of the upper reaches of the Yangtze River. The Minjiang River enters from northern Wenchuan County and runs through the county to the east. Wenchuan County has a temperate monsoon climate. Due to the disparity in topography, the climate changes from the southeast to the northwest, showing a relatively complete vertical climatic zonation. The average annual rainfall in Wenchuan County is 1,190.9 mm and the precipitation is unevenly distributed from month to month. As shown in Figure 4, the rainfall varies considerably during the monsoon and dry seasons. Moreover, the annual precipitation is mainly concentrated in the period from May to September.
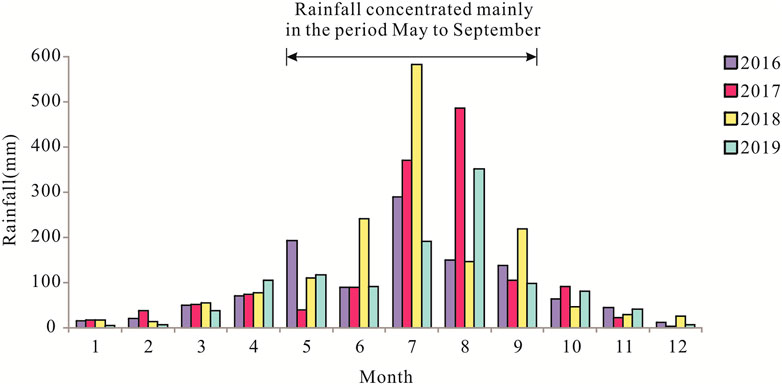
FIGURE 4. The distribution of rainfall in Wenchuan County from 2016 to 2019 (measured at the Dujiangyan Station).
Formation and Evolutionary Mechanisms
Triggering Rainfall
Figure 5A illustrates the daily rainfall history in the study area in August 2019. The monthly total rainfall in August was 350.5 mm. Of this amount, the rainfall on August 20 was the largest (124.4 mm), reaching the rainstorm level. From 02:00 on August 19 to 14:00 on August 22, a heavy storm occurred in Wenchuan County, where there were 12 stations with accumulated rainfall over 100 mm and five stations with rainfall over 200 mm, and the maximum accumulated rainfall was 332.6 mm at the Shouxi Sanjiang station. According to the rainfall data from the Dujiangyan surface meteorological station presented in Figure 5B, the rainstorm that triggered the “8.20” clustered debris flows event started at approximately 20:00 on August 19 and ended at approximately 09:00 on August 20, and the accumulated rainfall amount was 117.6 mm. The maximum hourly rainfall intensity was 28.5 mm, which occurred at 21:00 on August 19. The accumulated precipitation and rainfall intensity that triggered the debris flow were 87.5 and 24.2 mm/h, respectively, which were recorded at 03:00 a.m. on August 20, 2019.
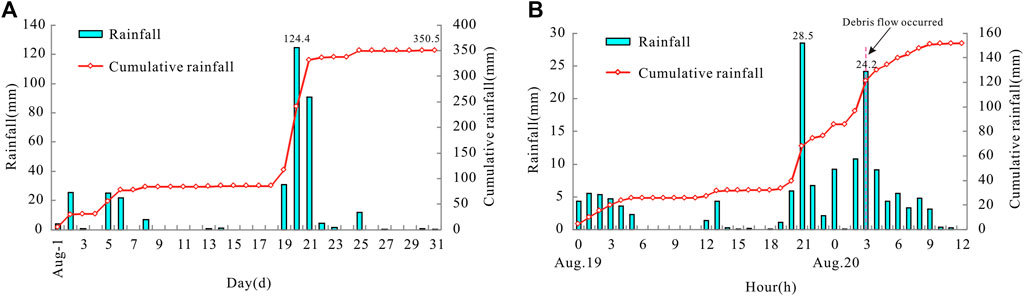
FIGURE 5. Rainfall conditions in Wenchuan County: (A) rainfall conditions in Wenchuan County in August 2019; and (B) distribution of hourly and cumulative rainfall in Wenchuan County on August 19–20, 2019 (measured at the Dujiangyan Station).
Formation Mechanism Analysis
As shown in Figure 6, the formation of a debris flow is very complicated and mainly includes basin hydrological processes, rainfall infiltration processes, soil instability and failure processes, slope and gully erosion processes, solid material supply processes, and soil-water coupling processes. The rainwater falling into the basin produces surface run-off and underground run-off after a series of processes, such as evaporation, plant interception, depression filling, and infiltration. However, there are mostly small watersheds with steep terrain in the study area, and thus, the effect of the filling process is not significant. Furthermore, due to the geohazards induced by earthquakes, the vegetation was severely damaged (Yang et al., 2012; Gan et al., 2019; Shen et al., 2020), causing the interception by plants to have little effect on the run-off during a rainstorm. Therefore, run-off occurs much more easily and quickly after high-intensity rainfall in the study area.
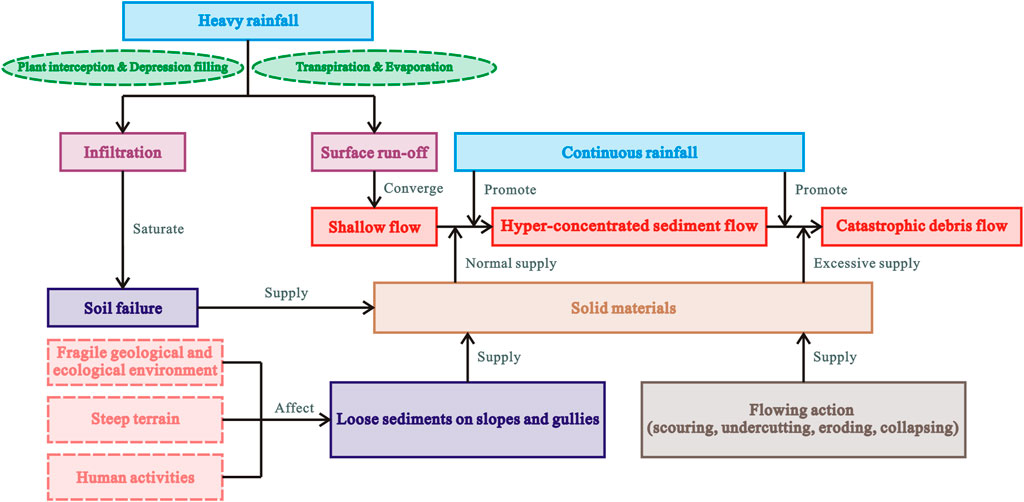
FIGURE 6. Analysis of the formation and dynamic evolutionary mechanism of the debris flow disaster in southwestern China.
At the beginning of rainfall, rainwater was mainly infiltrated. As the rainfall continued, the water content and pore water pressure of loose soil on the slope surface increased (Zhou et al., 2020), and the soil gradually became saturated, resulting in soil instability and failure. Subsequently, the soil gradually fluidized in the process of sliding, contributing to the source of solid material required for the formation of debris flow. The steep terrain providing sufficient energy conditions also contributed to run-off formation and rapid collection. As previously discussed, there is much loose solid material on the slope surface and in the gullies in the study area (Figure 7A). Under the effects of rainfall and run-off, the solid material accumulated on the slope surface, converged from a height to the gullies, and moved together with the gully deposits. Large potential energy was transformed into high kinetic energy, leading the channel deposits to scour and migrate downstream continuously, turning into a debris flow.
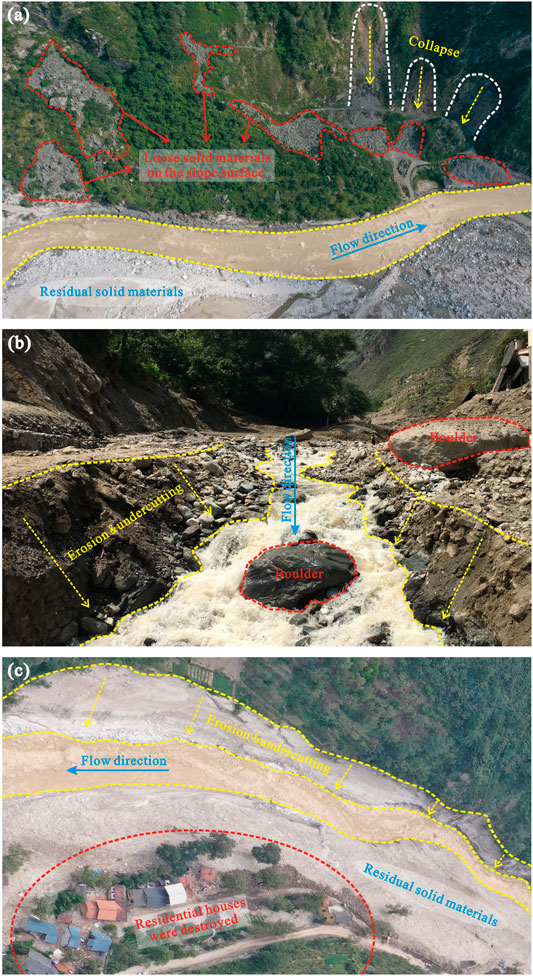
FIGURE 7. Typical traces left behind by the “8·20” clustered debris flows event: (A) loose solid materials on the slope surface and in the gully; (B) the deposits and terraces were strongly undercut and laterally eroded; and (C) buried residential houses along the bank of Cutou Gully.
Dynamic Evolutionary Characteristics
The excessive supply of solid material along the way was a critical reason for the amplification and scale of the debris flow (Bovis and Jakob, 1999; Jakob et al., 2005; McGuire et al., 2017). Based on the field investigation, the characteristics of the debris flow paths showed that high-speed fluid had great erosive energy, strongly undercutting and laterally eroding the deposits and terraces on both sides of the channel, as shown in Figure 7B. Otherwise, as the debris flow moved downstream, parts of the toes of the slopes were scraped and eroded away, resulting in shallow landslides on the slopes and collapse as the slopes lost support. The above processes produced abundant solid material in the formation and transition areas as a supplement to the debris flow, having a crucial influence on its dynamic evolution. As a result of the further enlargement of the scale of the debris flow, the houses and engineering facilities on both sides of the gully were seriously threatened and damaged, as shown in Figure 7C.
The field investigation has shown that the severe flood disaster caused by the debris flow, which poured into the Minjiang River and blocked the river partially or completely, is another factor in the disastrous outcome. Affected by heavy rainfall, many rivers in Wenchuan County suffered major floods or even extraordinary floods. According to the statistical results in recent years, the two main tributaries of the Minjiang River, the Caopo River Basin and Shouxi River Basin, reached a peak discharge of 573 m3/s with a return period of more than 100 years and 1,860 m3/s with a 50-years return period, respectively. The debris flow transported a large mass of solid material into the Minjiang River, forming debris-flow alluvial fans, thereby blocking the river. Therefore, the flow area of the river decreased rapidly, causing the water level in the upper reaches to rise sharply and the velocity and erosion potential of the flood to increase (Liang et al., 2001; Yanites et al., 2006; Liu et al., 2016). As shown in Figure 8, farmland, houses, transportation routes, hydropower stations, and other important infrastructure in the upper reaches were completely submerged by flooding caused by the debris-flow alluvial fan blocking the Minjiang River. Moreover, since the debris-flow alluvial fan forced flooding to divert to the opposite bank, which developed long-distance abnormal scouring downstream, the foundations of the houses near the riverbank were damaged, resulting in the collapse of several houses, as shown in Figure 8B.
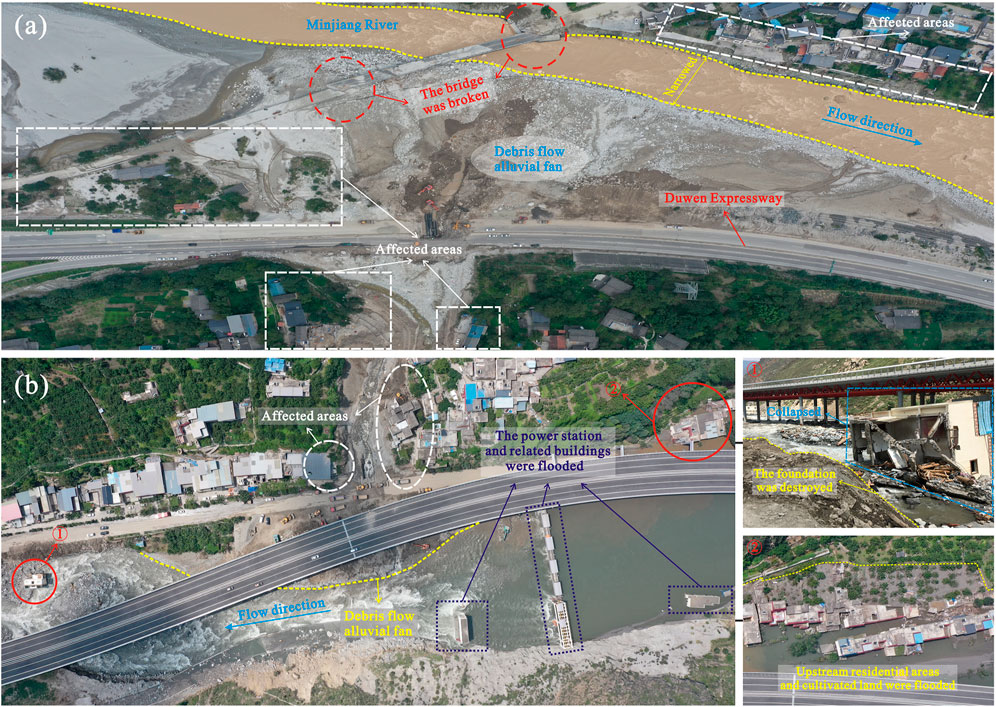
FIGURE 8. Extrusion of the channel caused by the alluvial fan from the debris flow and the typical disaster characteristics: (A) blockage of the Minjiang River and long-distance abnormal scouring to the opposite bank caused by the alluvial fan at the mouth of the Cutou Gully; and (B) severe flood disaster caused by the alluvial fan at the mouth of the Chayuan Gully.
Impacts of Human Activities
Amplification of the Destruction by the Bursting of Check Dams
Debris flow causes heavy damage and human casualties, which are also closely related to human engineering activities in addition to factors such as heavy rainfall and excessive solid material supply in gullies. Field reconnaissance indicated that the bursting of check dams was a significant reason for the amplification of the scale of the disaster and the enhancement of the destructive power at the mouth of the gullies. Building check dams in debris-flow gullies is a common engineering measure to control hazards from debris flow (You, 2001; Shrestha et al., 2008; Yuan et al., 2018). If the check dam can withstand the impact and erosion from the debris flow, there is no doubt that building a check dam is useful in regulating the peak discharge, intercepting large boulders and falling rocks, and greatly reducing the damage from the debris flow downstream. Conversely, if the structure of the check dam is destroyed by a large debris flow, the check dam will have an effect similar to block and burst, which inevitably leads to a considerable increase in the peak flow discharge (Chen et al., 2015). As shown in Figures 9A–C, these check dams proved to be useless during the “8·20” clustered debris flows event. When the debris flow moved to the check dam, the water-solid material mixture was deposited behind the check dam, and the energy also accumulated continuously, resulting in high pressure on the check dam. In the “8·20” clustered debris flows event, these failed check dams demonstrated insufficient design storage capacity, which caused the check dams to be quickly filled with solid material from the debris flow. Consequently, the check dam was buried, resulting in the risk of check dam failure significantly increased (Wang et al., 2021). In addition, the poor quality and performance of the check dam made it difficult to resist the scouring, erosion, and impact of the debris flow, so that the dam body was fragmented and damaged, gradually losing its effectiveness. Ultimately, the hyperconcentrated debris flow, which was deposited behind the check dam, ran across the dam crest by overflow. The failure of the check dam caused a significant increase in debris flow volume and devastating capacity, resulting in downstream houses and infrastructure such as roads and bridges being destroyed instantaneously.
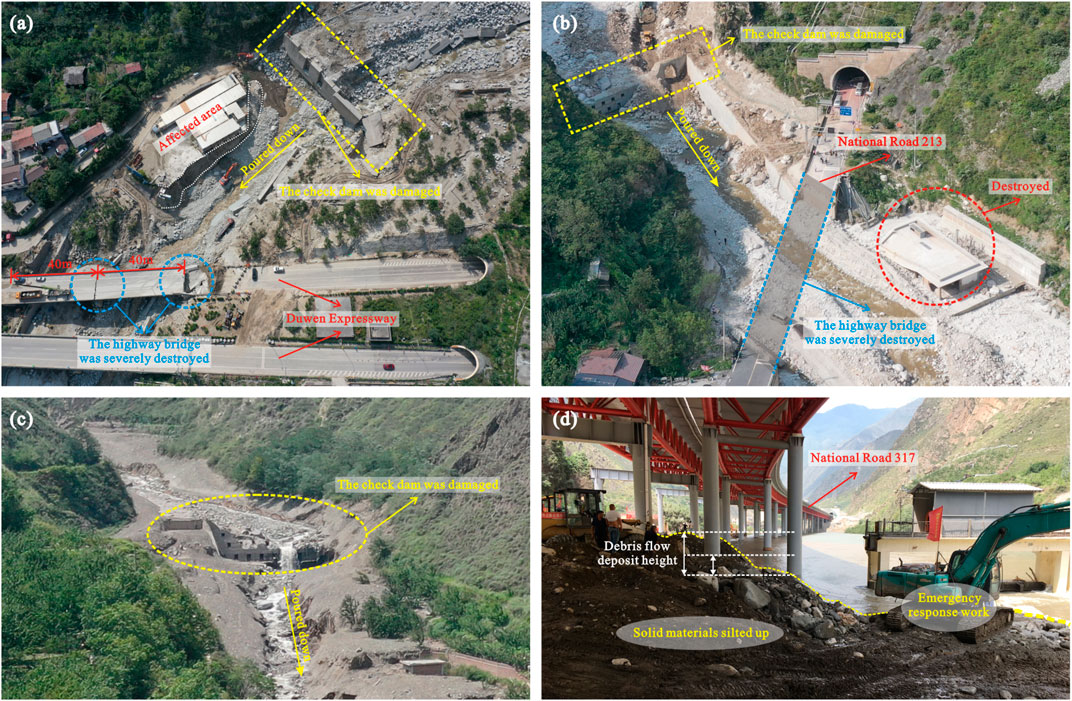
FIGURE 9. Typical traces of engineering facility damage caused by the “8·20” clustered debris flows event: (A) damage to the check dam and bridge pier columns in Banzi Gully; (B) damage to the check dam and highway bridge in Dengji Gully; (C) a destroyed check dam in Chayuan Gully; and (D) abundant solid materials deposited at the highway bridges and culverts.
Aggravating the Disaster by Damage to Transportation Facilities
In mountainous regions, highway bridges and culverts, pavement, and corresponding protective structures, which cross debris-flow gullies, are very vulnerable to being impacted and destroyed by debris flow (Zhong and Chen, 2010; Yang et al., 2016). According to incomplete statistics, approximately 45 roads and 26 bridges in Wenchuan County were damaged and interrupted as a result of the “8·20” clustered debris flows event. For instance, the left bridge of Banzi Gully, which has a total length of 90.5 m and consists of two 40-m T-shaped beams, was severely destroyed (Figure 9A). Similarly, the deck of the Dengjigou Bridge, approximately 50 m in length, completely collapsed (Figure 9B). In the early morning of August 20, the high-speed debris flow carrying large amounts of solid material flowed down from regions of high topography downstream through the gullies. However, the existence of highway bridges and culverts crossing the downstream area of the gullies hindered the movement of the debris flow, during which time disaster occurred.
The crucial problem that caused these serious damages is that the design of these bridges and culverts did not fully consider the impact, erosion, and flow requirements of such a large-scale debris flow. Impacts, scouring, burying, siltation, and abrasion are the types of damage to the highway bridges and culverts that were typically affected by the debris flow. When the debris flow crossed, the strong scouring and undercutting effect of debris flow continuously eroded the lower soil of the bridge foundation and abraded the pier columns, resulting in a decrease in the bearing capacity of the bridge. Finally, under the combined action of gravity and debris flow impact, the bridge foundation became unstable and the bridge deck collapsed (Figure 9A). Besides, the overall impact force of debris flow carrying huge boulders would push the foundations and pier columns of the bridge, and even directly destroy the bridge, as shown in Figure 9B. Figure 9D shows the solid material from the debris flow deposited at the highway bridges and culverts at the mouth of the gully. Another noteworthy problem is that the long-term accumulation of loose solid material under the bridge has not been cleaned up in time or effectively, which is also one of the reasons for the lack of cross-section when the debris flow event occurred. Furthermore, severe damage to transportation facilities is much more likely to delay emergency rescue work, such as searching and rescuing missing people and transferring disaster victims.
Discussion
There is no doubt that rainfall is the predominant factor leading to these disasters. The rainfall threshold is defined as the minimum level required to trigger a debris flow event. At present, the widely used method to determine the rainfall thresholds triggering debris flow is to conduct statistical analysis on precipitation data that induced the debris flow events in the past, extract relevant rainfall parameters or establish empirical relationships (Caine, 1980; Guzzetti et al., 2007; Zhou et al., 2014). Cui et al. (2010) concluded that the critical accumulated precipitation and rainfall intensity for triggering debris flow in the study area before the Wenchuan earthquake ranged between 320 and 350 mm and between 55 and 60 mm/h, respectively. Previous studies have shown that the rainfall thresholds for the subsequent generation of debris flow within the affected area were remarkably lower after the Wenchuan earthquake. For example, the critical accumulated precipitation and rainfall intensity that triggered the giant debris flow in Yingxiu Town on August 14, 2010 were 162.1 and 16.4 mm/h, respectively (Tang et al., 2011a), and correspondingly, the values of the catastrophic debris flow in Qipan Gully on July 11, 2013 were 88.0 and 21.6 mm/h, respectively (Hu and Huang, 2017). This variability in the rainfall thresholds is directly related to the abundant loose solid material in the formation area, which increased rapidly due to earthquake disturbance. Compared with the corresponding values of 87.5 and 24.2 mm/h in the “8·20” event discussed above, the rainfall thresholds for debris flow initiation in the Wenchuan area has not yet recovered to the pre-earthquake level, even more than ten years after the Wenchuan earthquake. However, with the reduction of effective material sources that can be transformed into debris flow, the rainfall thresholds will gradually recover over time, but how long it takes to achieve recovery requires further in-depth study. For this reason, meteorological monitoring for disaster warnings should be timely and accurate. However, a practical problem worthy of attention is that the rainfall stations in the study area are mostly distributed in the urban area, while they are less distributed in the disaster-prone mountainous areas; thus, it is difficult to truly reflect the rainfall situation in the mountainous areas and to guide people to take protective action in advance. This problem provides a warning to people that it is of great importance to establish an accurate, complete and quick-response rainfall early-warning system in the mountainous areas of Southwest China.
The hazard pattern changes with the dynamic evolution of disasters. As shown in Figure 6, the formation and development of a catastrophic debris flow event is a result of the sufficient and continuous interaction of water and sediment. Indisputably, abundant antecedent rainfall provides hydrodynamic conditions for the formation of debris flow, triggering the movement of loose solid material (Tang et al., 2011b; Guo et al., 2016; Hu et al., 2016). Furthermore, the rapid flow ceaselessly incorporates a supply of new solid material through new landslides and collapses, slope-rill erosion, and channel-bed erosion. Consequently, the ratio of sediment to water is constantly increasing, and finally, when the flow density is larger than 1.3 g/cm3 (Institute Of Mountain Hazards And Environment, 2000), it turns into a debris flow. As a result of the oversupply of loose material, the scale, velocity, and transportation capacity of the debris flow continue to increase, consequently producing tremendous destructive power. In addition to the characteristics of a simultaneous occurrence, rapid movement, and strong destructive impact, the field investigation also found that the “8·20” clustered debris flows event presented an extremely apparent chain of disaster effect. This indicates that debris flow not only causes damage but can also become the stimuli for other disasters and eventually cause more serious losses. The chain of disaster during the “8·20” event can be divided into two forms according to the different degrees of debris flow blocking the main river channel (Figure 10). One is that the debris flow moved into the main river channel, causing a partial blockage, squeezing the original river channel, uplifting the riverbed, and forcing the flood to divert, resulting in erosion of the opposite bank. The other is the movement of debris flow into the main river channel, building a temporary debris-flow dam that led to the formation of a barrier lake in the upstream area, resulting in an inundation disaster. The subsequent failure of the debris-flow dam caused a massive flood outburst to scour downstream areas.

FIGURE 10. Schematic diagram of the disaster chain effect of the “8·20” clustered debris flows event.
In addition to natural processes, human activities also play a crucial role in the formation and movement processes of debris flow. Affected by topography, mountain residents usually live at the mouth of gullies or on both sides of rivers. The dense distribution of buildings and infrastructure results in a high concentration of population in the area, which increases the potential for catastrophic damage from water-induced hazards. The impact of human activities on debris flow disasters includes two main aspects: indirect effects and direct effects (Gan et al., 2018). The former mainly emphasizes damage to the natural environment, such as the unreasonable exploitation and utilization of vegetation, soil, and water resources. The latter is mainly manifested in the amplification effect of human large-scale engineering activities on debris flow disasters, including improper human dwelling site selection, inadequate design of engineering works, poor-quality workmanship, and other aspects. In the “8·20” clustered debris flows event, it was found that the inadequate design of some engineering mitigation measures and traffic facilities aggravated the disaster losses, mainly due to insufficient consideration of the excessive supply of solid materials in the earthquake-active mountainous areas. This painful reality reminds us once again that the scale and destructive power of debris flow in earthquake-active mountainous areas are significantly different from those in nonseismic areas due to the availability of excessive loose solid materials. It has been proven that in the study area, debris flow disasters prevention and control projects constructed according to traditional engineering methods and design standards are no longer suitable. Therefore, how to make proper engineering decisions to prevent and control the hazards from debris flow has become a question that requires thoughtful consideration. Fortunately, compared with natural processes, which are complicated and difficult to change, the additional hazards caused by these human factors can be ameliorated once the seriousness of the problem is recognized.
In recent years, in view of the substantial loss of life and property caused by these disasters, people have adopted a variety of prevention and control measures against debris flow disasters in the mountainous areas of Southwest China and they have achieved noticeable results. For example, in the lower reaches of Yanmen Gully in Wenchuan County, a drainage channel with a width of 24 m was built, and the intersection angle between the Yanmen Gully and Minjiang River was changed to avoid the disaster caused by sediment deposition (Figure 11A). It is worth mentioning that this measure successfully withstood the test of the “8.20” event. Figure 11B shows the cascade sediment retaining project built in the debris flow gully in Qingping Township, Mianzhu City, Sichuan Province. Nevertheless, the occurrence of catastrophic events has proven that these efforts are far from sufficient.
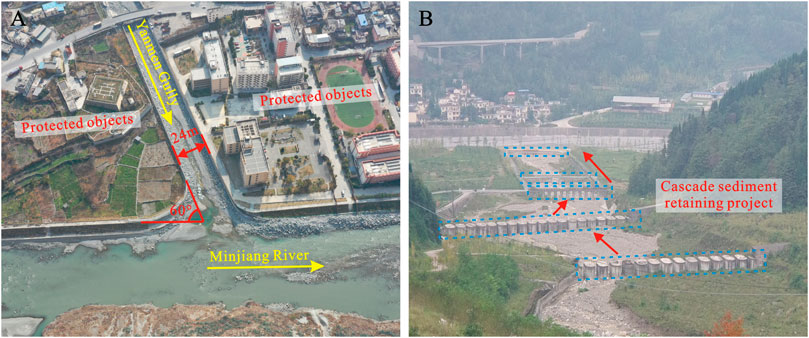
FIGURE 11. Typical cases of disaster prevention and control measures and achievements in recent years: (A) a drainage channel built in Yanmen Gully, Wenchuan County, Sichuan Province; and (B) the cascade sediment retaining project built in Qingping Township, Mianzhu City, Sichuan Province.
In general, collective relocation away from hazardous areas that are susceptible to debris flow is the best method to prevent disasters. However, whether from the perspective of economic feasibility or realistic possibility, collective relocation is difficult to fully realize. Therefore, seeking a more extensive and comprehensive system of integrated prevention and control is urgently needed. First, strengthening ecological restoration in disaster-stricken areas is indispensable. For example, the effectiveness of soil and water conservation efforts can be improved through forestry, agricultural, or planting measures in response to local conditions, thereby controlling solid material sources to a certain extent. Meanwhile, by drawing upon the experience from previous successful projects, engineering prevention and control measures should be rebuilt and perfected based on sufficient consideration of the solid material supply in each debris flow gully. In addition, judging from the characteristics of the “8·20” event, the Water Conservancy Department and relevant government departments should strengthen the monitoring and dredging work of the main river channels and gully beds to mitigate the impact from similar disaster chain effects in the future. Moreover, a perfect monitoring system is the basis for effective early warning. Thus, it is also crucial to establish a full-time remote intelligent wireless monitoring and early warning system, which can realize the collection, transmission, and processing of information such as landslide deformation, rainfall, soil saturation, mud level, water level, and flow velocity in the study area. Once a monitoring value exceeds the warning value set in this system, the warning information will be released in real-time. Finally, a series of nonengineering measures should also be adopted to warn people to take protective actions in advance and to reduce losses as much as possible when the event happens again. These nonengineering measures include but are not limited to adopting simple and reliable alarm signals, planning escape routes, formulating temporary relocation schemes, and elaborating emergency rescue work deployment.
Conclusion
In this paper, the “8·20” clustered debris flows event in Wenchuan County is used as a case study to better understand its formation mechanism, dynamic evolutionary process, and the impacts on human activities. Based on field investigation, image data interpretation, mechanism analysis, and some other methods, the main conclusions can be drawn as follows:
(1) The “8·20” event had the remarkable characteristics of simultaneous occurrence, rapid movement, and strong destructiveness. Field investigations indicate that the disaster points were mainly distributed along the Minjiang River, where human engineering activities are highly concentrated. Furthermore, this event presented an extremely apparent disaster chain effect, leading to the expansion of disaster losses.
(2) Regional short-term, high-intensity rainfall is considered the direct trigger of the “8·20” event. According to the rainfall data from the Dujiangyan surface meteorological station, the critical accumulated precipitation and rainfall intensity for triggering this event were 87.5 and 24.2 mm/h, respectively, which were still far from the pre-earthquake level.
(3) The excessive supply of solid material is a key factor that cannot be ignored in the prediction and prevention of debris flow disasters in the study area. The occurrence of the devastating “8·20” event in Wenchuan County demonstrates that the abundant supply of solid material can greatly change fluid characteristics, making the disaster uncontrollable.
(4) In addition to natural factors, human activities also play a crucial role in the formation and movement processes of debris flow. On the one hand, inappropriate human activities damage the ecological environment to a certain extent and indirectly influence the occurrence and development of debris flow. On the other hand, the transformation of natural topography by large-scale human engineering activities directly affects the evolutionary process of debris flow. Once these projects are improperly located or inadequately designed, the risk of geological hazards is likely to increase.
(5) When collective relocation is difficult to achieve, it is particularly important to utilize the necessary ecological measures to control material sources and to effectively improve the performance of the engineering measures to achieve water and sediment separation. Furthermore, the extensive establishment of a full-time remote intelligent wireless monitoring and early warning system is also urgently needed. Finally, a series of nonengineering measures are also worthy of additional attention.
Data Availability Statement
The original contributions presented in the study are included in the article/Supplementary Material, further inquiries can be directed to the corresponding author.
Author Contributions
YL: formal analysis (lead), methodology, and writing-original draft preparation (lead); X-nL: investigation (lead), writing-review and editing; B-rG: validation, visualization; X-kW: funding acquisition, resources; X-gY: conceptualization, investigation; H-bL: formal analysis, visualization, writing-original draft; X-yL: visualization; J-wZ: conceptualization (lead), funding acquisition, investigation, writing-review and editing (lead).
Funding
This work was funded by the National Natural Science Foundation of China (51639007) and the Sichuan Youth Science and Technology Innovation Research Team Project (2020JDTD0006).
Conflict of Interest
The authors declare that the research was conducted in the absence of any commercial or financial relationships that could be construed as a potential conflict of interest.
Acknowledgments
Kindly suggestions provided by the Field Chief Editor Prof. Acocella and Specialty Chief Editor Prof. Woo are greatly acknowledged. Critical comments from the reviewers greatly improved the initial manuscript.
References
Bovis, M. J., and Jakob, M. (1999). The Role of Debris Supply Conditions in Predicting Debris Flow Activity. Earth Surf. Process. Landforms, 24, 1039–1054. 10.1002/(sici)1096-9837(199910)24:11<1039::aid-esp29>3.0.co;2-u
Caine, N. (1980). The Rainfall Intensity: Duration Control of Shallow Landslides and Debris Flows. Geografiska Annaler. Ser. A, Phys. Geogr. 62 (1/2), 121. doi:10.2307/520449
Chen, H.-X., Zhang, L.-M., Zhang, S., Xiang, B., and Wang, X.-F. (2013). Hybrid Simulation of the Initiation and Runout Characteristics of a Catastrophic Debris Flow. J. Mt. Sci. 10 (2), 219–232. doi:10.1007/s11629-013-2505-z
Chen, M.-l., Liu, X.-n., Wang, X.-k., Zhao, T., and Zhou, J.-w. (2019). Contribution of Excessive Supply of Solid Material to a Runoff-Generated Debris Flow during its Routing along a Gully and its Impact on the Downstream Village with Blockage Effects. Water 11 (1), 169. doi:10.3390/w11010169
Chen, N., Yang, C., Zhou, W., Hu, G., Li, H., and Hand, D. (2009). The Critical Rainfall Characteristics for Torrents and Debris Flows in the Wenchuan Earthquake Stricken Area. J. Mt. Sci. 6 (4), 362–372. doi:10.1007/s11629-009-1064-9
Chen, X., Cui, P., You, Y., Chen, J., and Li, D. (2015). Engineering Measures for Debris Flow hazard Mitigation in the Wenchuan Earthquake Area. Eng. Geology. 194, 73–85. doi:10.1016/j.enggeo.2014.10.002
Cui, P., Chen, X.-Q., Zhu, Y.-Y., Su, F.-H., Wei, F.-Q., Han, Y.-S., et al. (2009). The Wenchuan Earthquake (May 12, 2008), Sichuan Province, China, and Resulting Geohazards. Nat. Hazards 56 (1), 19–36. doi:10.1007/s11069-009-9392-1
Cui, P., Hu, K., Zhuang, J., Yang, Y., and Zhang, J. (2011). Prediction of Debris-Flow Danger Area by Combining Hydrological and Inundation Simulation Methods. J. Mt. Sci. 8 (1), 1–9. doi:10.1007/s11629-011-2040-8
Cui, P., Wei, F. Q., He, S. M., You, Y., Chen, X. Q., Li, Z. L., et al. (2008). Mountain Disasters Induced by the Earthquake of May 12 in Wenchuan and the Disasters Mitigation. J. Mountain Sci. 26 (3), 280–282. doi:10.35123/geo-expo_2017_4
Cui, P., Wei, F. Q., Xie, H., Yang, K., He, Y. P., and Ma, D. T. (2003). Debris Flow and Disaster Reduction Strategies in Western China. Quat. Sci. 12, (02), 142–151. doi:10.1016/j.ijdrr.2019.101405
Cui, P., Zhuang, J., Chen, X., Zhang, J., and Zhou, X. (2010). Characteristics and Countermeasures of Debris Flow in Wenchuan Area after the Earthquake. J. Sichuan Univ. (Engineering Sci. Edition) 42 (05), 10–19. doi:10.1007/s11629-016-3965-8
Dai, F. C., Xu, C., Yao, X., Xu, L., Tu, X. B., and Gong, Q. M. (2011). Spatial Distribution of Landslides Triggered by the 2008 Ms 8.0 Wenchuan Earthquake, China. J. Asian Earth Sci. 40 (4SI), 883–895. doi:10.1016/j.jseaes.2010.04.010
Dong, J.-J., Lee, C.-T., Tung, Y.-H., Liu, C.-N., Lin, K.-P., and Lee, J.-F. (2009). The Role of the Sediment Budget in Understanding Debris Flow Susceptibility. Earth Surf. Process. Landforms 34 (12), 1612–1624. doi:10.1002/esp.1850
Gan, B.-r., Liu, X.-n., Yang, X.-g., Wang, X.-k., and Zhou, J.-w. (2018). The Impact of Human Activities on the Occurrence of Mountain Flood Hazards: Lessons from the 17 August 2015 Flash Flood/debris Flow Event in Xuyong County, South-Western China. Geomatics, Nat. Hazards Risk 9 (1), 816–840. doi:10.1080/19475705.2018.1480539
Gan, B.-r., Yang, X.-g., Zhang, W., and Zhou, J.-w. (2019). Temporal and Spatial Evolution of Vegetation Coverage in the Mianyuan River basin Influenced by strong Earthquake Disturbance. Sci. Rep. 9 (1), 16762. doi:10.1038/s41598-019-53264-5
Guo, X., Cui, P., Li, Y., Zhang, J., Ma, L., Mahoney, W. B., et al. (2016). Spatial Features of Debris Flows and Their Rainfall Thresholds in the Wenchuan Earthquake-Affected Area. Landslides 13 (5), 1215–1229. doi:10.1007/s10346-015-0608-z
Guzzetti, F., Peruccacci, S., Rossi, M., and Stark, C. P. (2007). Rainfall Thresholds for the Initiation of Landslides in central and Southern Europe. Meteorol. Atmos. Phys. 98 (3), 239–267. doi:10.1007/s00703-007-0262-7
Hu, T., and Huang, R.-q. (2017). A Catastrophic Debris Flow in the Wenchuan Earthquake Area, July 2013: Characteristics, Formation, and Risk Reduction. J. Mt. Sci. 14 (1), 15–30. doi:10.1007/s11629-016-3965-8
Hu, W., Dong, X. J., Xu, Q., Wang, G. H., van Asch, T. W. J., and Hicher, P. Y. (2016). Initiation Processes for Run-Off Generated Debris Flows in the Wenchuan Earthquake Area of China. Geomorphology 253, 468–477. doi:10.1016/j.geomorph.2015.10.024
Huang, R., and Fan, X. (2013). The Landslide story. Nat. Geosci 6 (5), 325–326. doi:10.1038/ngeo1806
Huang, R. Q., and Li, W. L. (2009). Analysis of the Geo-Hazards Triggered by the 12 May 2008 Wenchuan Earthquake, China. Bull. Eng. Geol. Environ. 68 (3), 363–371. doi:10.1007/s10064-009-0207-0
Institute Of Mountain Hazards And Environment (2000). Debris Flows in China. Beijing: Commercial Press.
Iverson, R. M. (1997). The Physics of Debris Flows. Rev. Geophys. 35 (3), 245–296. doi:10.1029/97rg00426
Jakob, M., Bovis, M., and Oden, M. (2005). The Significance of Channel Recharge Rates for Estimating Debris-Flow Magnitude and Frequency. Earth Surf. Process. Landforms 30 (6), 755–766. doi:10.1002/esp.1188
Koi, T., Hotta, N., Ishigaki, I., Matuzaki, N., Uchiyama, Y., and Suzuki, M. (2008). Prolonged Impact of Earthquake-Induced Landslides on Sediment Yield in a Mountain Watershed: The Tanzawa Region, Japan. Geomorphology 101 (4), 692–702. doi:10.1016/j.geomorph.2008.03.007
Li, H. B., Fu, X. F., Jér O Me, V. D. W., Si, J. L., Wang, Z. X., Hou, L. W., et al. (2008). Co-seisimic Surface Rupture and Dextral-Slip Oblique Thrusting of the MS 8.0 Wenchuan Earthquake. Acta Geologica Sinica 82 (12), 1623–1643. doi:10.1007/978-3-642-03759-7_3
Liang, Z. Y., Li, M., Kuang, S. F., and Xu, Y. N. (2001). River Channel Changes Due to Converge of Debris Flow. J. Soil Water Conservation 7, (S1), 112–115. doi:10.1201/9781315644479-364
Liu, J., You, Y., Chen, X., Chen, X., Chen, X., Chen, X., et al. (2016). Mitigation Planning Based on the Prediction of River Blocking by a Typical Large-Scale Debris Flow in the Wenchuan Earthquake Area. Landslides 13 (5), 1231–1242. doi:10.1007/s10346-015-0615-0
McGuire, L. A., Rengers, F. K., Kean, J. W., and Staley, D. M. (2017). Debris flow initiation by runoff in a recently burned basin: Is grain-by-grain sediment bulking or en masse failure to blame? Geophys. Res. Lett. 44 (14), 7310–7319. doi:10.1002/2017GL074243
Parker, R. N., Densmore, A. L., Rosser, N. J., de Michele, M., Li, Y., Huang, R., et al. (2011). Mass Wasting Triggered by the 2008 Wenchuan Earthquake Is Greater Than Orogenic Growth. Nat. Geosci 4 (7), 449–452. doi:10.1038/ngeo1154
Shen, P., Zhang, L. M., Fan, R. L., Zhu, H., and Zhang, S. (2020). Declining Geohazard Activity with Vegetation Recovery during First Ten Years after the 2008 Wenchuan Earthquake. Geomorphology 352, 106989. doi:10.1016/j.geomorph.2019.106989
Shieh, C. L., Chen, Y. S., Tsai, Y. J., and Wu, J. H. (2009). Variability in Rainfall Threshold for Debris Flow after the Chi-Chi Earthquake in central Taiwan, China. Int. J. Sediment Res. 24 (2), 177–188. doi:10.1016/s1001-6279(09)60025-1
Shrestha, B. B., Nakagawa, H., Kawaike, K., and Baba, Y. (2008). Numerical and Experimental Study on Debris-Flow Deposition and Erosion Upstream of a Check Dam. Proc. Hydraulic Eng. 52, 139–144. doi:10.2208/prohe.52.139
Takahashi, T. (1981). Debris Flow. Annu. Rev. Fluid Mech. 13 (1), 57–77. doi:10.1146/annurev.fl.13.010181.000421
Tang, C., Li, W., Ding, J., and Huang, X. (2011a). Field Investigation and Research on Giant Debris Flow on August 14,2010 in Yingxiu Town,epicenter of Wenchuan Earthquake. Earth Sci. 36 (01), 172–180. doi:10.4028/www.scientific.net/amr.610-613.771
Tang, C., Zhu, J., Ding, J., Cui, X. F., Chen, L., and Zhang, J. S. (2011b). Catastrophic Debris Flows Triggered by a 14 August 2010 Rainfall at the Epicenter of the Wenchuan Earthquake. Landslides 8 (4), 485–497. doi:10.1007/s10346-011-0269-5
Tang, C., Zhu, J., Li, W. L., and Liang, J. T. (2009). Rainfall-triggered Debris Flows Following the Wenchuan Earthquake. Bull. Eng. Geol. Environ. 68 (2), 187–194. doi:10.1007/s10064-009-0201-6
Wang, D., Qu, H., Shen, W., and He, S. (2021). Dynamic Response Analysis for Debris Flow Dam with Sediments. Adv. Eng. Sci. 53 (02), 1–9.
Wang, G. L. (2013). Lessons Learned from Protective Measures Associated with the 2010 Zhouqu Debris Flow Disaster in China. Nat. Hazards 69 (3), 1835–1847. doi:10.1007/s11069-013-0772-1
Wang, Z. Y., Qi, L., Wang, X., Qi, L., Wang, X., and Wang, X. (2012). A Prototype experiment of Debris Flow Control with Energy Dissipation Structures. Nat. Hazards 60 (3), 971–989. doi:10.1007/s11069-011-9878-5
Xie, H., Zhong, D. L., Jiao, Z., and Zhang, J. S. (2009). Debris Flow in Wenchuan Quake-Hit Area in 2008. J. Mountain Sci. 27 (4), 501–509. doi:10.21660/2015.15.4381
Yang, C., Ren, X., Huang, H., Ren, X., Huang, H., and Huang, H. (2012). The Vegetation Damage Assessment of the Wenchuan Earthquake of May 2008 Using Remote Sensing and GIS. Nat. Hazards 62 (1), 45–55. doi:10.1007/s11069-011-0036-x
Yang, M., Chen, H., and Chen, S. (2016). “Study on the Failure Mechanism of Bridge Pier in the Active Debris Flow Regions,” in Proceedings of 2016 International Conference on Power, Energy Engineering and Management (PEEM 2016), Bangkok, Thailand, January 2016, 246–250.
Yanites, B. J., Webb, R. H., Griffiths, P. G., and Magirl, C. S. (2006). Debris Flow Deposition and Reworking by the Colorado River in Grand Canyon, Arizona. Water Resour. Res. 42 (11), W11411. doi:10.1029/2005WR004847
You, Y. (2001). Characteristics of Intercepting Sediment of Debris Flow Check Dam. J. Soil Water Conservation 11, (01), 113–115. doi:10.5194/nhessd-3-5777-2015
Yu, B., Wu, Y., and Chu, S. (2014). Preliminary Study of the Effect of Earthquakes on the Rainfall Threshold of Debris Flows. Eng. Geology. 182, 130–135. doi:10.1016/j.enggeo.2014.04.007
Yuan, D., Liu, J.-f., You, Y., Liu, D.-c., Sun, H., Zhang, L., et al. (2018). The Siltation of Debris Flow behind Check Dam in the Midstream of Bailong River. J. Mt. Sci. 15 (1), 100–113. doi:10.1007/s11629-017-4484-y
Zhong, Y., and Chen, H. (2010). Destroyed Mechanism Analysis of Bridge Buried by Debris Flow. J. Chongqing Jiaotong University(Natural Sciences) 29 (5), 667–669. doi:10.1111/j.1755-6724.1992.mp5002001.x
Zhou, J.-w., Li, H.-b., Lu, G.-d., Zhou, Y., Zhang, J.-y., and Fan, G. (2020). Initiation Mechanism and Quantitative Mass Movement Analysis of the 2019 Shuicheng Catastrophic Landslide. Q. J. Eng. Geology. Hydrogeology 54, 121–152. doi:10.1144/qjegh2020-052
Keywords: debris flow, formation mechanism, supply condition, dynamic evolutionary process, human activities
Citation: Li Y, Liu X-n, Gan B-r, Wang X-k, Yang X-g, Li H-b, Long X-y and Zhou J-w (2021) Formation-Evolutionary Mechanism Analysis and Impacts of Human Activities on the 20 August 2019 Clustered Debris Flows Event in Wenchuan County, Southwestern China. Front. Earth Sci. 9:616113. doi: 10.3389/feart.2021.616113
Received: 11 October 2020; Accepted: 14 May 2021;
Published: 26 May 2021.
Edited by:
Chong Xu, Ministry of Emergency Management, ChinaReviewed by:
Ming Zhang, China University of Geosciences Wuhan, ChinaYulong Cui, Anhui University of Science and Technology, China
Copyright © 2021 Li, Liu, Gan, Wang, Yang, Li, Long and Zhou. This is an open-access article distributed under the terms of the Creative Commons Attribution License (CC BY). The use, distribution or reproduction in other forums is permitted, provided the original author(s) and the copyright owner(s) are credited and that the original publication in this journal is cited, in accordance with accepted academic practice. No use, distribution or reproduction is permitted which does not comply with these terms.
*Correspondence: Jia-Wen Zhou, jwzhou@scu.edu.cn