- 1Research Department, Dr. Kurt Wolff GmbH & Co. KG, Bielefeld, Germany
- 2Department of Comprehensive Dentistry, School of Dentistry, University of Texas Health San Antonio, San Antonio, TX, United States
- 3Faculty of Dentistry, University of Toronto, Toronto, ON, Canada
- 4Bionics and Materials Development, Hamm-Lippstadt University of Applied Sciences, Hamm, Germany
- 5Department of Integrated Dentistry, Medical University of Bialystok, Bialystok, Poland
- 6Department of Integrated Dentistry, Poznan University of Medical Sciences, Poznan, Poland
Children are prone to develop dental caries. This is supported by epidemiological data confirming early childhood caries (ECC) as a highly prevalent disease affecting more than every second child worldwide. ECC is known to result from an imbalance between re- and demineralization where demineralization dominates due to frequent acid production by cariogenic bacteria present in oral biofilms. The application of oral care formulations containing remineralizing agents helps to prevent dental caries. As young children are sensitive and usually swallow (intended or unintended) a majority of toothpaste or other oral care products during daily dental care, all ingredients, especially the actives, should be non-toxic. Biomimetic hydroxyapatite [HAP; Ca5(PO4)3(OH)] is known to have favorable remineralizing properties combined with an excellent biocompatibility, i.e., it is safe if accidently swallowed. Several clinical trials as well as in situ and in vitro studies have shown that HAP remineralizes enamel and dentin. Remineralization occurs due to deposition of HAP particles on tooth surfaces forming mineral-mineral bridges with enamel crystals, but also indirectly through calcium and phosphate ions release as well as HAP's buffering properties in acidic environments (i.e., in plaque). HAP induces a homogenous remineralization throughout the subsurface enamel lesions. This review summarizes the current evidence showing HAP as an effective remineralizing agent in oral care products for children. Additional studies showing also further beneficial effects of HAP such as the reduction of biofilm formation and the relief of hypersensitivity in children with molar incisor hypomineralization (MIH). It can be concluded that HAP is an effective and safe remineralizing agent for child dental care.
Introduction
Daily oral hygiene, especially in the morning and evening, and tooth-healthy dietary attitude are the key factors to prevent dental caries (1–3). In addition to the use of a suitable toothbrush, a toothpaste with effective and active anti-caries agents should be used (4–6). For this reason, fluoride-containing toothpaste formulations have been favored for the past years (6). A dose-dependent relationship of fluorides is discussed. One assumes that the addition of several fluoride sources might lead to better results in preventing caries. Hausen et al. (7) have shown that the combination of different fluoride-sources does not lead to a higher caries-preventive effect. Additionally, due to concerns mainly dealing with safety-problems, many parents try to avoid fluoride whenever possible (8). Consequently, effective and safe alternatives to fluorides are needed. Recently published clinical studies have proven the efficacy of microcrystalline hydroxyapatite (HAP) with respect to caries prevention (5, 9–12). HAP is well-known to be biomimetic, or a bionic active ingredient when used in oral care (13, 14). Human enamel consists of approximately 97% HAP (15, 16). By synthesizing this HAP, it can be used as a remineralizing agent in oral care products (16–20).
The introduction of a safe and well accepted active agent for caries prevention can help to reduce caries prevalence in young children which remains high today (1). As fluoride has been shown to be effective in preventing caries, HAP needs to be at least as effective as fluoride. The anti-caries efficacy of HAP has been proven in several randomized clinical trials, while in situ studies have shown its remineralizing effectiveness. This evidence was recently published as a systematic review and meta-analysis that has shown the caries-preventive effect of HAP (5). Remineralization of demineralized parts of the teeth is important for the efficacy of anti-caries agents. While the mode of action of fluoride has been investigated in the past year and was currently revised (21), using HAP is a quite new approach for preventing caries (5, 8). As a well-known calcium-phosphate, it can be considered as safe agent (22).
The aim of this review is to analyze the existing literature regarding HAP as active ingredient in oral care applications and to summarize its caries preventive effects with a special focus on biomimetic remineralization.
Materials and Methods
For this narrative review on the remineralizing mode of action of HAP, literature was obtained from Chen et al. (17), Enax et al. (14), Limeback et al. (5), and O' Hagan-Wong et al. (8). Additionally, same databases and search strategy as presented elsewhere were used to find more recently published papers (5). In short, Ovid Medline (PubMed), EMBASE, Scopus, and Web of Science were chosen as the primary databases. Search terms and strategy were: “hydroxyapatite” AND (“in vitro study” OR “in situ study” OR “in vivo study” OR “remineralization” OR “caries”) AND (“oral care” OR “toothpaste” OR “dentifrice” OR “mouthwash” OR “mouthrinse”). Literature was screened with a special focus on the modes of action with respect to remineralization and caries-prevention of HAP resulting in 29 studies included in this review with respect to (clinical) efficacy.
Hydroxyapatite in Preventive Dental Care for Children
HAP is widely used in oral care products and in dentistry. In the following, the modes of action of HAP, clinical studies, and also biocompatibility with special focus on children are presented.
Modes of Action of Hydroxyapatite
The modes of action of particulate HAP have already been described in detail by Enax et al. (14). An update on the modes of action of particulate hydroxyapatite has recently been published in a systematic review and meta-analysis by Limeback et al. (5). Briefly, with a special focus on remineralization, the modes of action of HAP (Figure 1) is described as follows.
• Formation of a Protective HAP Layer
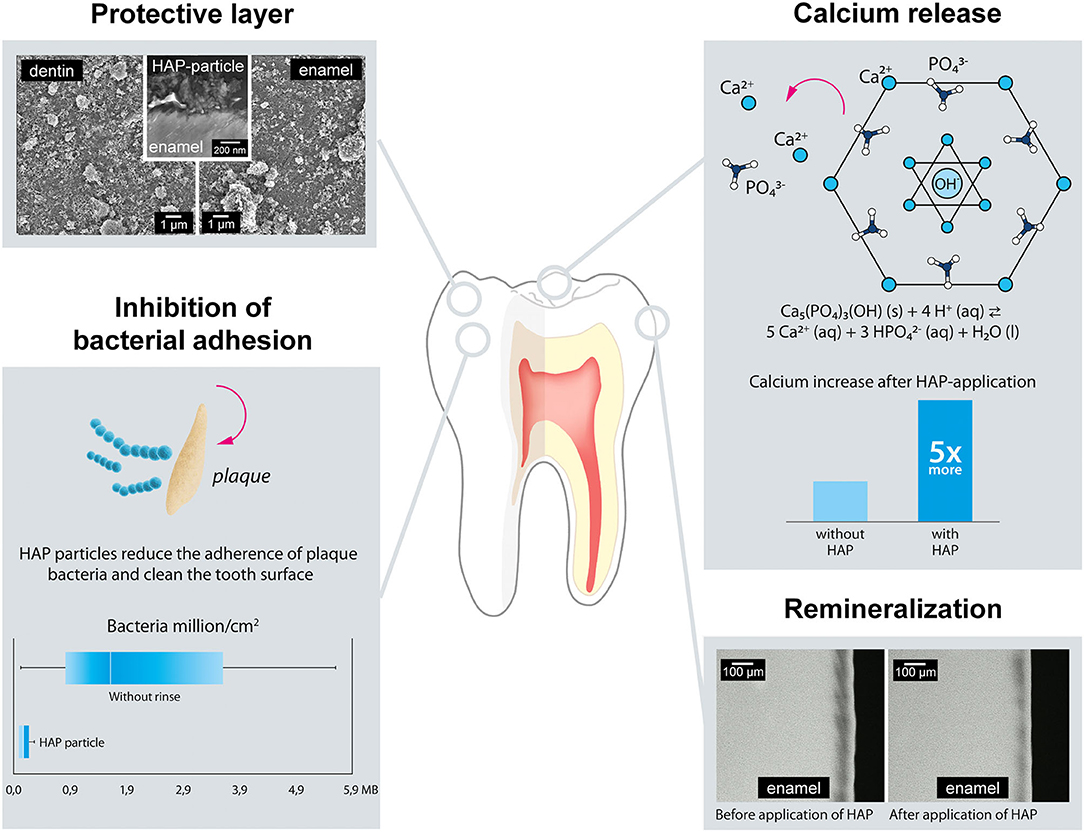
Figure 1. Modes of action of Hydroxyapatite (HAP) with respect to caries prevention and remineralization. HAP forms a protective layer on the tooth-surface. HAP is also known to be a calcium source. Studies have shown that HAP-particles reduce the dental biofilm adhesion. In terms of remineralization, HAP is able to homogenously remineralize caries-lesion.
HAP has a high affinity to human dentin, especially its collagen, but also to enamel (23, 24). HAP microcrystals adhere to the crystallites of human tooth tissue (25–27). Recently published data confirm that HAP particles form mineral-mineral bridges with enamel crystallites (25, 26). This means that HAP from oral care products can adhere to and directly remineralize demineralized tooth tissues at their surface layers (20, 28–30). Adherence to the surface layer can protect the natural tooth from subsequent acid attacks, including acids from food substances (31–33). The HAP layer has also been shown to survive several acid attacks, using a cyclic pH-model (26). Constant application of HAP from oral care products will therefore lead to densification and renewal of the adhering layer, thus enhancing its protective characteristics.
• Calcium Release and pH-Buffering
HAP does not only form a protective layer at the exposed surfaces of teeth, but can also be incorporated into dental biofilm (13, 34–37). Acids derived from either food or bacteria will dissolve the particulate HAP (34, 36), resulting to release of calcium ions and increase the plaque pH (34, 35), with the following benefits.
• The acids attack the protective HAP layer, but not directly the tooth. The tooth will be protected.
• Calcium-release from HAP remineralizes demineralized parts of the enamel also in its subsurface layers. Additionally, increase in calcium concentration shifts the chemical equilibrium of tooth-HAP from dissolution to a more stable state.
• An increase in the pH protects the tooth from demineralization by weakening acids. As the critical pH of enamel is about 5.5, a pH where tooth mineral starts to dissolve more easily, a minor change can help to protect the tooth surface.
• Inhibition of Bacterial Plaque Adhesion
Several in situ studies have investigated the reduction of bacterial adhesion on dental surfaces (enamel, dentin) and the surfaces of other materials exposed to the oral environment, such as dental implants (titan) and dental restorations (polymethyl-methacrylate) (38–42). Most of these studies used chlorhexidine (0.2%, a strong antibacterial/bactericidal agent) as positive control. The reduction in number of bacteria on (tooth-) surfaces after the use of HAP mouthwashes was comparable to that observed with chlorhexidine (38, 39, 43). Interestingly, in contrast to chlorhexidine, the mode of action of HAP is not bactericidal or bacteriostatic (14, 37). It seems that tooth surfaces modified with HAP-particles prevent the initial adherence of bacteria (so called early colonizers) (44) to the teeth. Kensche et al. showed that bacteria adhere to the free HAP particles in saliva instead of adhering to the tooth surface, thus reducing the ability of bacteria to form plaque on the tooth surfaces (39). By adhering to HAP particles, the bacteria form aggregates that are easily cleared away from the oral cavity through saliva swallowing or mouth rinsing together with loose particles (39). As dental biofilm formation is reduced, this also decreases the risk of demineralization of dental surfaces by acids derived from microorganisms (35, 37).
• Deep Remineralization of Caries Lesions
Tooth demineralization occurs all day and is in healthy conditions in an equilibrium with remineralization. Salivary ions, especially calcium and phosphate ions, help to remineralize demineralized surfaces. However, a diet with frequent carbohydrate exposure leads to a net-demineralization of dental surfaces. Consequently, the application of remineralizing agents seems to be important (36). Clinical studies have shown that HAP-toothpaste is effective in preventing dental caries (5, 9–12, 45). In situ studies help to investigate the mechanism of action of HAP in preventing tooth demineralization (20, 29). Irrespective of the type of application, toothpaste, or gel, HAP leads to a more homogenous remineralization compared to fluoride (14). Fluoride remineralization is limited to the surface layer of the lesion (28–30), while HAP remineralization seems to be deeper and homogenous throughout the subsurface layer of the lesion (20, 28–30). The HAP nano- or microparticles penetrate into the micropores in carious tooth tissue, and templates de novo hydroxyapatite crystal formation around each particle, by continuously attracting large amounts of calcium and phosphate ions from the surrounding solution into the tooth tissue, thus promoting crystal integrity and growth (46). This biomimetic mineralization process enables the regeneration of enamel and dentin. Additionally, HAP will remineralize the surface of carious lesions. It can be suggested that HAP particles are a calcium source-as mentioned above. The application of HAP can also be seen as “neo”-mineralization (5, 8).
Anti-Caries and Remineralization Studies on Hydroxyapatite
Several in vitro studies have shown that HAP remineralizes demineralized enamel and dentin, and also protects teeth from demineralization (13, 14). However, for clinical relevance and evidence, high quality randomized clinical trials are needed for each active ingredient used in oral care and every other discipline in medicine. Clinical settings have demonstrated the ability of HAP to protect teeth from dental caries. Five randomized controlled trials (RCTs) presented evidence that HAP has anti-caries properties (5, 8–12, 45). The first being a placebo controlled clinical trial with children in elementary school in Japan, published back in 1989 (45). This long-term 3-year clinical trial investigated caries increment and showed significant reduction in decayed, missing, filled (permanent) teeth (DMFT) with the use of a HAP toothpaste (5% HAP content). Two other single-blind, randomized clinical caries trials that measured incipient and proximal caries concluded significant caries remineralization effects (11, 12). Both clinical trials reported direct measurement of anti-caries effects in young adults and children after exposure to HAP toothpaste with use of ICDAS (the international caries detection and assessment system) (47), DIAGNOdent, photographic pixel changes and interproximal digital radiography. It seems that the use of multiple measurement methods removed the subjectivity in assessment of the HAP-induced remineralization effect, and increased the reliability and sensitivity of the measurement. Interestingly, from a methodological point of view, Badiee et al. (13) included fluoride toothpaste as positive control, and both toothpastes significantly reduced enamel white spot lesions among patients after orthodontic treatment (12). A long-term study by Grocholewicz et al. (11) compared the remineralizing effect of a HAP gel (10% HAP) in combination with ozone gas therapy, with subject inclusion criteria of ICDAS II > code 1 active caries lesions. Subjects were randomly assigned into three groups (group I: HAP only, group II: ozone only, group III: HAP + ozone), and evaluated after 1 and 2 years. The study showed a reduction in early caries lesions after the first year, and a decline in caries was still observed after the second year when using HAP. Bitewing radiographs confirmed enamel surface and subsurface remineralization after use of HAP. The HAP anti-caries effect was suggested to be enhanced by ozone gas application (11).
Two well-designed double-blind RCTs by Schlagenhauf et al. (10) and Paszynska et al. (9), provided further evidence with respect to the efficacy of HAP in terms of caries prevention and remineralization. Both studies used baseline criteria of ICDAS > code 1 active caries lesions in a long-term observation of patients at risk for caries. Schlagenhauf et al. (10) concluded that a toothpaste with HAP (10% HAP) was as effective as a regular fluoride toothpaste (1,400 ppm fluoride formulated as amine fluoride and stannous fluoride) in preventing progression of caries in high caries risk subjects (orthodontic treatment) after 6 months observation (10). A recently published multicenter RCT by Paszynska et al. (9) on HAP toothpaste (10% HAP) showed an improvement with respect to remineralization of caries lesions in primary tooth enamel. The HAP toothpaste was as effective as fluoride toothpastes (500 ppm fluoride formulated as amine fluoride) in young children with primary dentition in double blinded examinations (9). Thus, the HAP toothpaste was found not inferior to the fluoride control toothpaste after 1-year observation. The HAP-group presented a slight reduction with respect to enamel caries (ICDAS II ≥ code 1) per surface by 4.2% compared to the fluoride-group. The exact one-sided upper 95% confidence limit for the difference in proportion of participants with increase in ICDAS II score ≥ 1 was 9.8%, which is below the non-inferiority margin. This demonstrated non-inferiority of the HAP-containing toothpaste compared to the fluoride control toothpaste when used for a long-term period (9).
Based on the above overview, it can be concluded that the use of biomimetic HAP as an active ingredient in oral-care products may be a useful clinical tool in planning oral care among high caries risk children. The studies confirm that patients can use HAP oral care products, and dental professionals can recommend them confidently for clinical applications in preventing dental decay, both in children and adults.
Biocompatibility and Safety of Hydroxyapatite
Biocompatibility is defined as the ability of a biomaterial to perform its desired function with respect to a medical therapy, without eliciting an undesirable local or systemic effects in the recipient or beneficiary of that therapy, but generating the most appropriate beneficial cellular or tissue response in that specific situation, and optimizing the clinically relevant performance of that therapy” (48). As HAP and fluoride are the only active ingredients used in oral care products with a proven anti-caries efficacy (5, 6), both will be discussed in the following paragraphs with respect to their biocompatibility.
Hydroxyapatite
HAP is a calcium phosphate with both a micro- and nano-crystalline morphology. It is the essential mineral component of teeth and bone. It has been widely applied as a biomaterial in medicine and dentistry due to its excellent bioactivity (the potential to induce calcium phosphate deposits) and osteoconductivity (the ability to serve as a scaffold for bone formation) (49, 50). This mineral presents additional favorable features: it is non-toxic, non-immunogenic, and it does not elicit significant inflammatory reactions in cells and tissues, which has been confirmed by numerous in vitro and in vivo studies (50–55). Consequently, HAP meets the basic biocompatibility requirements of the definition cited above (48).
HAP can be synthesized in various crystalline morphologies and particles sizes (from nano- to micrometer size) (25, 41). HAP in nano scale seems to be of particular interest to scientists studying the issue of material biocompatibility (56). Nanoparticles present extraordinary features that can be helpful for development and improvement of applications in many branches, including dentistry and medicine (53). However, especially in dentistry and oral care applications, micro-sized HAP-particles have been shown to be effective in remineralization and other processes (26, 29, 37, 39, 57).
HAP, as biomimetic agent, is not limited with respect to regulatory aspects when used in oral care products, thus different HAP-containing oral care products (i.e., toothpaste, mouth rinse, gel) can be combined and used even several times a day. A review of the literature demonstrates that HAP nanoparticles are non-toxic (22). HAP nanoparticles have been reported to cause a rise in the concentration of calcium ions in the cell cytoplasm. However, in the living organism this excess of calcium ions may be easily eliminated by the calcium pump located in the cell membrane (58). HAP is used, also in nano-forms, as bone-substitute and implant-coating and no adverse effects have been reported since it is biologically resorbed by osteoclasts and macrophages (59). An oral administration of HAP is safe when swallowed as the particles are dissolved in the stomach by the gastric acid resulting in a release of calcium and phosphate ions (34, 60). One may think HAP can be taken up by oral mucosa cells. However, to date and to the best of the authors knowledge, no study reports that HAP has been detected in oral mucosa tissues in vivo. Additionally, morphology and size of the calcium phosphate (nano-) particles seems to have no significant impact on the biological response of human cells (22). In conclusion, the risk related to exposure to calcium phosphate, including hydroxyapatite, in doses that are generally applied in dentistry, health care products, and cosmetics is very low and according to the available data it is not clinically significant (13, 22, 56).
Fluoride
Fluoride is the ionic, negatively charged form of fluorine, that reacts with positive ions such as sodium or calcium to form stable chemical molecules (61). Fluoride's ability to inhibit the progression of dental caries is well documented in the literature, even though only a small proportion (1%) of the clinical trials have been published in the past 10 years (6). Fluoride needs to be applied topically on a regular daily basis to have an anti-caries effect (4). The anti-caries action of fluoride is mainly based on the inhibition of enamel demineralization, and the promotion of enamel remineralization with the help of calcium and phosphate derived from saliva (21, 62). Antibacterial properties are also discussed, because fluoride inhibits specific bacterial enzymes, especially enolase (63, 64). In contrast to this, other studies came to the conclusion that counterions are responsible for the antibacterial effect (65).
Despite the beneficial anti-caries effect of fluoride, the ingestion of fluoride may result in toxic and detrimental effects (66, 67). An adequate intake of fluoride from all sources has been calculated to be 50 μg/kg body weight per day at which the risk of dental fluorosis is set to a minimum (68, 69). Oral hygiene products (toothpastes, mouth-rinses, and gels) can increase total fluoride intake over and above foods and beverages, including water (70). Studies show that parents apply ~50% more of the recommended toothpaste-amount for children on their kids toothbrushes. It has been also shown that children swallow most of their applied toothpaste leading to an increased risk of dental fluorosis, when fluoride-toothpaste is used (67, 71). The average consumption of fluoride due to the toothpaste swallowing while toothbrushing was estimated to be ~1.4 μg/kg body weight per day for adults and 11.5 μg/kg body weight per day for children (72). Approximately 80% of the fluoride toxicity incidents were registered in children younger than the age of 6 (73).
The toxicity of fluoride caused by excessive ingestion is classified into acute and chronic toxicity. The acute fluoride toxicity usually appears due to the accidental consumption of fluoride compounds. The minimum toxic dose of fluoride has been estimated at 5 mg/kg body weight. The lethal dose has been set between 7 and 16 mg/kg body weight (73). Common signs and symptoms of acute fluoride toxicity include gastro-intestinal disturbances (hypersalivation, nausea, vomiting, abdominal pain, diarrhea), hypocalcemia, muscle tetany, drop in the blood pressure, hyperkalemia, cardiac arrhythmia, coma, and failure of the renal and respiratory system terminating in death (74).
With respect to fluoride toxicity, five possible mechanisms are discussed: (73).
• formation of hydrofluoric acid in a humid environment and the burn of tissues as result of low pH;
• impaired nerve function due to hypocalcemia resulting from the reaction of fluoride with calcium ions;
• electrolyte imbalance due to hypocalcemia and hyperkalemia consequently leading to cardiac arrhythmia;
• inhibition of cell enzymes;
• oxidative stress duo to excessive production of free radicals
The effects of chronic toxicity depend mainly on the amount and duration of fluoride exposure. The earliest indicator of chronic fluoride toxicity is dental fluorosis, which appears when the chronic intake of fluoride exceeds 1 mg/l or 0.1 mg/kg daily during the period of tooth development. Excessive fluoride ingestion may also lead to skeletal fluorosis, structural and functional changes in the kidneys, irritation of the gastro-intestinal tract, suppression of the immune system and degenerative changes in the nervous system (74). The US National Toxicology Program recently published a draft report critically evaluating the evidence for human developmental neurotoxicity of fluoride in animal and human (75). However, in a critique of the human evidence some researchers claimed that the literature did not support the presumption that fluoride should be assessed as a human developmental neurotoxicant at the current exposure-levels in Europe. Following this, Guth et al. came to the conclusion that from the current available data, no final conclusion on the safety of fluorides could be made and more research is needed (76). In addition to the documented severe adverse effects of fluoride, unwanted side effects are also reported from dentistry: Fluoride is known to corrode implants and also negatively impact the application of orthodontic treatments (31, 77–79). In contrast to this, HAP has shown excellent biocompatibility in these settings (31, 77).
Hydroxyapatite in Oral Care Products
Oral care products containing HAP as the active ingredient are marketed as toothpastes, mouthwashes, dental lotions, polishing pastes, whitening liquids, and oral care gels. The first clinical trial on caries prevention with a HAP toothpaste was performed in Japan with school children. This placebo-controlled trial has shown the high potential of HAP to prevent dental caries (45). These results were corroborated in further studies, including randomized clinical trials (9–12, 80, 81).
Most toothpastes available over the counter contain mainly fluoride as caries-preventive agent. Fluorides have been shown to be effective in preventing dental caries (6). However, most of the studies (~ 80%) supporting this fact were published 30+ years ago (81). Additionally, many discussed modes of action of fluoride (e.g., increase in acid-solubility) are questionable (21). It is also known that fluorides need calcium and phosphate ions from saliva to be effective (62). A randomized clinical trial (three arms) with a 3-year follow-up period published by Hausen et al. in demonstrated that the addition of several fluoride compounds does not prevent dental caries more efficiently than brushing with toothpaste containing a single fluoride compound (7). It should be noted that the daily amount of fluoride intake is restricted because of its known toxicity (82, 83). Recent studies showed also the potential of fluorides to corrode dental braces and implants (31, 78, 79, 84). Besides, young children do swallow most of their toothpaste (67). Caution is needed when fluoride is ingested regularly. Studies in areas where fluoride from water was ingested frequently showed cognitive impacts (for example reduction in IQ) when babies were exposed prenatally (85) and postnatally (86) to higher fluoride concentrations. Further studies have been published showing negative impact of fluoride on general health (82, 87–89). These research data led to an increased concern about cumulative fluoride exposure from all sources. Consequently, safe and effective alternatives to fluorides are needed (5, 8). This alternative may be calcium-based active agents (1, 14).
Toothpastes contain several different components to improve their efficacy as oral care products an HAP is becoming a preferred multifunctional agent (14, 61, 90, 91). From the view of a developer of cosmetic and medical products, toothpastes are one of the most challenging products. Most of the components are provided as powders. Consequently, a high proportion of powders needs to be formulated in water, which is in most cases the basic constituent of most toothpastes that are available. One important characteristic of toothpastes and mouthwashes with respect to oral health is the pH value (92). In healthy condition, the pH of the oral cavity is around 7.2. Acidic conditions, especially with a pH < 6, will eventually lead to enamel dissolution. Consequently, the pH of oral care-products should be higher or ideally not lower than approximately pH = 7.2. While this is true for HAP-containing oral care products, most fluoride products for children are acidic (see Table 1). However, it should be taken into account that not only toothpaste-pH is important for de-and remineralization of enamel and dentin, but also the characteristics of the dental biofilm (i.e., bacterial composition, metabolites, acidic or acidogenic biofilm), salivary composition (i.e., calcium, phosphate, carbonate), salivary flow and the interaction of them all.
The importance of the use of HAP in toothpastes has been shown in several different clinical settings as well as different well-published in vitro studies (5). HAP is not only effective in remineralizing enamel of permanent teeth, but also enamel of the primary dentition (20, 29, 30). Additionally, research has been performed on dentin, and root caries remineralization (24, 28, 93). The efficacy of HAP has been proven in different settings, including remineralizing settings, cyclic demineralization-remineralization settings, and most important in net-demineralization settings (5). The results from either toothpaste or mouthwash studies can be transferred to either of those product categories as it has been shown that HAP adheres to and remineralizes tooth surfaces with both types of application (toothpaste and mouthwash).
Other Application Fields of Hydroxyapatite
Besides reversing the formation of initial caries lesions (through remineralization) and preventing new caries development, HAP has other effects that are utilized for applications in dentistry. When added to oral care products it helps to whiten teeth, relief sensitivity, rebuild and protect dental hard tissues against erosion. Recently published studies report the whitening effect of HAP-containing oral care products (94–97). HAP adheres to the teeth and, as white particulate active, it leads to a changed light-scattering (94). Following this, teeth appear whiter. HAP has also been shown to relief dentin hypersensitivity. The first clinical trial on HAP toothpastes against tooth sensitivity was published in 1987 by Huettemann and Doenges from the university hospital in Giessen, Germany (57). Their main finding was that HAP toothpaste relieved dentin hypersensitivity, even better than the local anesthetic benzocain used in other toothpastes. In a network systematic review, Hu et al. found that nano-HAP toothpastes may be the best desensitizing toothpastes for treatment of dentin hypersensitivity, followed by arginine toothpaste (81). When added to high concentration professional bleaching gels, HAP can reduce the dentin hypersensitivity often associated with teeth whitening (98). HAP has also been used to enhance the success of HAP-coated dental implants (99), improving bone repair during oral surgery or periodontal surgery (100), and improving dental restorative materials (101). HAP has anti-gingivitis properties by reducing plaque levels (102, 103). Further in situ studies demonstrated that HAP in mouthwashes was as effective as 0.2% chlorhexidine in reducing dental biofilm adhesion (38, 39). Clinical studies also showed this anti-gingivitis effectiveness with HAP in toothpaste (103, 104). As HAP is a white particulate crystal, teeth will appear whiter after application (94). A recently published RCT also shows the benefit of HAP in reducing pain sensitivity in children suffering from MIH (molar incisor hypomineralization) (105).
Conclusions
Oral care products containing HAP have been proven to be safe and effective in terms of preventing dental caries. The modes of action of HAP pave the way for the caries preventive effect of this biomimetic agent. Remineralization of dental caries is one of the well-known mechanisms. HAP has been shown to remineralize both dentin and enamel. Other benefits of HAP have been published, one of which is the reduction of sensitivity also associated with hypomineralized teeth (81, 105). As calcium phosphate, HAP is safe when swallowed and no unwanted effects are documented (22).
Author Contributions
All authors were involved in writing and editing the manuscript.
Conflict of Interest
FM and JE are employees of Dr. Kurt Wolff GmbH & Co. KG.
The remaining authors declare that the research was conducted in the absence of any commercial or financial relationships that could be construed as a potential conflict of interest.
Publisher's Note
All claims expressed in this article are solely those of the authors and do not necessarily represent those of their affiliated organizations, or those of the publisher, the editors and the reviewers. Any product that may be evaluated in this article, or claim that may be made by its manufacturer, is not guaranteed or endorsed by the publisher.
Acknowledgments
The authors thank Markus Morick (employee at Dr. Wolff Group) for the preparation of Figure 1.
References
1. Meyer F, Enax J. Early childhood caries: epidemiology, aetiology, and prevention. Int J Dent. (2018) 2018:7. doi: 10.1155/2018/1415873
2. van Loveren C. Sugar restriction for caries prevention: amount and frequency. which is more important? Caries Res. (2019) 53:168-75. doi: 10.1159/000489571
3. Sheiham A, James WP. Diet and dental caries: the pivotal role of free sugars reemphasized. J Dent Res. (2015) 94:1341-7. doi: 10.1177/0022034515590377
5. Limeback H, Enax J, Meyer F. Biomimetic hydroxyapatite and caries prevention: a systematic review and meta-analysis. Can J Dent Hyg. (2021) 55:148-59.
6. Walsh T, Worthington HV, Glenny AM, Marinho VCC, Jeroncic A. Fluoride toothpastes of different concentrations for preventing dental caries. Cochrane Database Syst Rev. (2019) 3:CD007868. doi: 10.1002/14651858.CD007868.pub3
7. Hausen H, Kärkkäinen S, Seppä L. Application of the high-risk strategy to control dental caries. Community Dent Oral Epidemiol. (2000) 28:26–34. doi: 10.1034/j.1600-0528.2000.280104.x
8. O'Hagan-Wong K, Enax J, Meyer F, Ganss B. The use of hydroxyapatite toothpaste to prevent dental caries. Odontology. (2021) 110:223-30. doi: 10.1007/s10266-021-00675-4
9. Paszynska E, Pawinska M, Gawriolek M, Kaminska I, Otulakowska-Skrzynska J, Marczuk-Kolada G, et al. Impact of a toothpaste with microcrystalline hydroxyapatite on the occurrence of early childhood caries: a 1-year randomized clinical trial. Sci Rep. (2021) 11:2650. doi: 10.1038/s41598-021-81112-y
10. Schlagenhauf U, Kunzelmann K-H, Hannig C, May TW, Hösl H, Gratza M, et al. Impact of a non-fluoridated microcrystalline hydroxyapatite dentifrice on enamel caries progression in highly caries-susceptible orthodontic patients: a randomized, controlled 6-month trial. J Invest Clin Dent. (2019) 10:e12399. doi: 10.1111/jicd.12399
11. Grocholewicz K, Matkowska-Cichocka G, Makowiecki P, Drozdzik A, Ey-Chmielewska H, Dziewulska A, et al. Effect of nano-hydroxyapatite and ozone on approximal initial caries: a randomized clinical trial. Sci Rep. (2020) 10:11192. doi: 10.1038/s41598-020-67885-8
12. Badiee M, Jafari N, Fatemi S, Ameli N, Kasraei S, Ebadifar A. Comparison of the effects of toothpastes containing nanohydroxyapatite and fluoride on white spot lesions in orthodontic patients: a randomized clinical trial. Dent Res J. (2020) 17:354-9. doi: 10.4103/1735-3327.294328
13. Meyer F, Amaechi BT, Fabritius HO, Enax J. Overview of calcium phosphates used in biomimetic oral care. Open Dent J. (2018) 12:406-23. doi: 10.2174/1874210601812010406
14. Enax J, Fabritius HO, Fabritius-Vilpoux K, Amaechi BT, Meyer F. Modes of action and clinical efficacy of particulate hydroxyapatite in preventive oral health care – state of the art. Open Dent J. (2019) 13:274-87. doi: 10.2174/1874210601913010274
15. Fabritius H-O, Enax J, Meyer F. Eine Reise ins Innere unserer Zähne/A Journey into our Teeth. Wiesbaden: Titus Verlag (2021).
16. Dorozhkin SV, Epple M. Biological and medical significance of calcium phosphates. Angew Chem Int Ed. (2002) 41:3130-46. doi: 10.1002/1521-3773(20020902)41:17<3130::AID-ANIE3130>3.0.CO;2-1
17. Chen L, Al-Bayatee S, Sultan Z, Shavandi A, Brunton P, Ratnayake J. Hydroxyapatite in oral care products—a review. Materials. (2021) 14:4865. doi: 10.3390/ma14174865
18. Grewal N, Sharma N, Kaur N. Surface remineralization potential of nano-hydroxyapatite, sodium monofluorophosphate, and amine fluoride containing dentifrices on primary and permanent enamel surfaces: an in vitro study. J Indian Soc Pedodont Prev Dentistry. (2018) 36:158-66. doi: 10.4103/JISPPD.JISPPD_142_17
19. Juntavee A, Juntavee N, Sinagpulo AN. Nano-hydroxyapatite gel and its effects on remineralization of artificial carious lesions. Int J Dentistry. (2021) 2021:7256056. doi: 10.1155/2021/7256056
20. Amaechi BT, Alshareif DO, Azees PAA, Shehata MA, Lima PP, Abdollahi A, et al. Anti-caries evaluation of a nano-hydroxyapatite dental lotion for use after toothbrushing: an in situ study. J Dent. (2021) 115:103863. doi: 10.1016/j.jdent.2021.103863
21. Epple M, Enax J, Meyer F. Prevention of caries and dental erosion by fluorides-a critical discussion based on physico-chemical data and principles. Dent J. (2022) 10:6. doi: 10.3390/dj10010006
22. Epple M. Review of potential health risks associated with nanoscopic calcium phosphate. Acta Biomater. (2018) 77:1-14. doi: 10.1016/j.actbio.2018.07.036
24. Tschoppe P, Zandim DL, Martus P, Kielbassa AM. Enamel and dentine remineralization by nano-hydroxyapatite toothpastes. J Dent. (2011) 39:430-7. doi: 10.1016/j.jdent.2011.03.008
25. Fabritius-Vilpoux K, Enax J, Herbig M, Raabe D, Fabritius H-O. Quantitative affinity parameters of synthetic hydroxyapatite and enamel surfaces in vitro. Bioinspir Biomim Nan. (2019) 8:141-53. doi: 10.1680/jbibn.18.00035
26. Fabritius-Vilpoux K, Enax J, Mayweg D, Meyer F, Herbig M, Raabe D, et al. Ultrastructural changes of bovine enamel and dentin surfaces under chemical erosion in presence of biomimetic hydroxyapatite crystallites. Bioinspir Biomim Nan. (2021) 10:132-45. doi: 10.1680/jbibn.21.00017
27. Fabritius-Vilpoux K, Enax J, Meyer F, Fabritius H-O. In vitro Microstructural Analysis of Erosion Protection by a Hydroxyapatite-Gel. Washington, DC: IADR/AADR/CADR General Session (2020).
28. Amaechi BT, Phillips TS, Evans V, Ugwokaegbe CP, Luong MN, Okoye LO, et al. The potential of hydroxyapatite toothpaste to prevent root caries: a pH-cycling study. Clin Cosmet Investig Dent. (2021) 13:315-24. doi: 10.2147/CCIDE.S319631
29. Amaechi BT, AbdulAzees PA, Alshareif DO, Shehata MA, Lima PPdCS, Abdollahi A, et al. Comparative efficacy of a hydroxyapatite and a fluoride toothpaste for prevention and remineralization of dental caries in children. BDJ Open. (2019) 5:18. doi: 10.1038/s41405-019-0026-8
30. Amaechi BT, AbdulAzees PA, Okoye LO, Meyer F, Enax J. Comparison of hydroxyapatite and fluoride oral care gels for remineralization of initial caries: a pH-cycling study. BDJ Open. (2020) 6:9. doi: 10.1038/s41405-020-0037-5
31. Lelli M, Marchisio O, Foltran I, Genovesi A, Montebugnoli G, Marcaccio M, et al. Different corrosive effects on hydroxyapatite nanocrystals and amine fluoride-based mouthwashes on dental titanium brackets: a comparative in vitro study. Int J Nanomedicine. (2013) 8:307-14. doi: 10.2147/IJN.S35245
32. Colombo M, Beltrami R, Rattalino D, Mirando M, Chiesa M, Poggio C. Protective effects of a zinc-hydroxyapatite toothpaste on enamel erosion: SEM study. Ann Stomatol. (2016) 7:38-45. doi: 10.11138/ads/2016.7.3.038
33. Bossù M, Saccucci M, Salucci A, Di Giorgio G, Bruni E, Uccelletti D, et al. Enamel remineralization and repair results of Biomimetic Hydroxyapatite toothpaste on deciduous teeth: an effective option to fluoride toothpaste. J Nanobiotechnology. (2019) 17:17. doi: 10.1186/s12951-019-0454-6
34. Cieplik F, Rupp CM, Hirsch S, Muehler D, Enax J, Meyer F, et al. Ca2+ release and buffering effects of synthetic hydroxyapatite following bacterial acid challenge. BMC Oral Health. (2020) 20:85. doi: 10.1186/s12903-020-01080-z
35. Sudradjat H, Meyer F, Loza K, Epple M, Enax J. In vivo effects of a hydroxyapatite-based oral care gel on the calcium and phosphorus levels of dental plaque. Eur J Dent. (2020) 14:206-11. doi: 10.1055/s-0040-1708456
36. Meyer F, Enax J, Epple M, Amaechi BT, Simader B. Cariogenic biofilms: development, properties, and biomimetic preventive agents. Dent J. (2021) 9:88. doi: 10.3390/dj9080088
37. Meyer F, Enax J. Hydroxyapatite in oral biofilm management. Eur J Dent. (2019) 13:287-90. doi: 10.1055/s-0039-1695657
38. Hannig C, Basche S, Burghardt T, Al-Ahmad A, Hannig M. Influence of a mouthwash containing hydroxyapatite microclusters on bacterial adherence in situ. Clin Oral Investig. (2013) 17:805-14. doi: 10.1007/s00784-012-0781-6
39. Kensche A, Holder C, Basche S, Tahan N, Hannig C, Hannig M. Efficacy of a mouthrinse based on hydroxyapatite to reduce initial bacterial colonisation in situ. Arch Oral Biol. (2017) 80:18-26. doi: 10.1016/j.archoralbio.2017.03.013
40. Nobre CMG, König B, Pütz N, Hannig M. Hydroxyapatite-based solution as adjunct treatment for biofilm management: an in situ study. Nanomat. (2021) 11:2452. doi: 10.3390/nano11092452
41. Nobre CMG, Pütz N, Hannig M. Adhesion of hydroxyapatite nanoparticles to dental materials under oral conditions. Scanning. (2020) 2020:6065739. doi: 10.1155/2020/6065739
42. Nobre CMG, Pütz N, König B, Rupf S, Hannig M. Modification of in situ biofilm formation on titanium by a hydroxyapatite nanoparticle-based solution. Front Bioing Biotech. (2020) 8:311. doi: 10.3389/fbioe.2020.598311
43. Jones CG. Chlorhexidine: is it still the gold standard? Periodontol. (1997) 15:55-62. doi: 10.1111/j.1600-0757.1997.tb00105.x
44. Mira A, Simon-Soro A, Curtis MA. Role of microbial communities in the pathogenesis of periodontal diseases and caries. J Clin Periodontol. (2017) 44:S23-38. doi: 10.1111/jcpe.12671
45. Kani K, Kani M, Isozaki A, Shintani H, Ohashi T, Tokumoto T. Effect of apatite-containing dentifrices on dental caries in school children. J Dent Health. (1989) 19:104-9. doi: 10.5834/jdh.39.104
46. Amaechi BT, Loveren Cv. Fluorides and non-fluoride remineralization systems. Monogr Oral Sci. (2013) 23:15-26. doi: 10.1159/000350458
47. Ismail AI, Sohn W, Tellez M, Amaya A, Sen A, Hasson H, et al. The International Caries Detection and Assessment System (ICDAS): an integrated system for measuring dental caries. Community Dent Oral Epidemiol. (2007) 35:170-8. doi: 10.1111/j.1600-0528.2007.00347.x
48. Williams DF. On the mechanisms of biocompatibility. Biomat. (2008) 29:2941-53. doi: 10.1016/j.biomaterials.2008.04.023
49. Calasans-Maia MD, Melo BRd, Alves ATNN, Resende RFdB, Louro RS, Sartoretto SC, et al. Cytocompatibility and biocompatibility of nanostructured carbonated hydroxyapatite spheres for bone repair. J Appl Oral Sci. (2015) 23:599-608. doi: 10.1590/1678-775720150122
50. Gutiérrez-Prieto SJ, Fonseca LF, Sequeda-Castañeda LG, Díaz KJ, Castañeda LY, Leyva-Rojas JA, et al. Elaboration and biocompatibility of an eggshell-derived hydroxyapatite material modified with Si/PLGA for bone regeneration in dentistry. Int J Dent. (2019) 2019:5949232. doi: 10.1155/2019/5949232
51. Kamadjaja MJK, Abraham JF, Laksono H. Biocompatibility of portunus pelagicus hydroxyapatite graft on human gingival fibroblast cell culture. Med Arch. (2019) 73:303-6. doi: 10.5455/medarh.2019.73.378-381
52. Chadda H, Naveen SV, Mohan S, Satapathy BK, Ray AR, Kamarul T. Cytotoxic evaluation of hydroxyapatite-filled and silica/hydroxyapatite-filled acrylate-based restorative composite resins: an in vitro study. J Prosthet Dent. (2016) 116:129-35. doi: 10.1016/j.prosdent.2015.12.013
53. Remya NS, Syama S, Sabareeswaran A, Mohanan PV. Investigation of chronic toxicity of hydroxyapatite nanoparticles administered orally for one year in wistar rats. Mater Sci Eng C Mater Biol Appl. (2017) 76:518-27. doi: 10.1016/j.msec.2017.03.076
54. Sadowska JM, Wei F, Guo J, Guillem-Marti J, Ginebra MP, Xiao Y. Effect of nano-structural properties of biomimetic hydroxyapatite on osteoimmunomodulation. Biomat. (2018) 181:318-32. doi: 10.1016/j.biomaterials.2018.07.058
55. Motskin M, Wright DM, Muller K, Kyle N, Gard TG, Porter AE, et al. Hydroxyapatite nano and microparticles: correlation of particle properties with cytotoxicity and biostability. Biomat. (2009) 30:3307-17. doi: 10.1016/j.biomaterials.2009.02.044
56. Coelho CC, Grenho L, Gomes PS, Quadros PA, Fernandes MH. Nano-hydroxyapatite in oral care cosmetics: characterization and cytotoxicity assessment. Sci Rep. (2019) 9:11050. doi: 10.1038/s41598-019-47491-z
57. Huettemann RW, Doenges H. Untersuchungen zur Therapie überempfindlicher Zahnhälse mit Hydroxylapatit. Dtsch Zahnärztl Z. (1987) 42:486-8
58. Lopreiato R, Giacomello M, Carafoli E. The plasma membrane calcium pump: new ways to look at an old enzyme. J Biol Chem. (2014) 289:10261-8. doi: 10.1074/jbc.O114.555565
59. Tonino AJ, van der Wal BCH, Heyligers IC, Grimm B. Bone remodeling and hydroxyapatite resorption in coated primary hip prostheses. Clin Orthop Relat Res. (2009) 467:478-84. doi: 10.1007/s11999-008-0559-y
60. Margolis HC, Moreno EC. Kinetics of hydroxyapatite dissolution in acetic, lactic, and phosphoric acid solutions. Calcif Tissue Int. (1992) 50:137-43. doi: 10.1007/BF00298791
61. Epple M, Enax J. Moderne Zahnpflege aus chemischer Sicht. ChiuZ. (2018) 4:218–28. doi: 10.1002/ciuz.201800796
62. Cate JMt. Review on fluoride, with special emphasis on calcium fluoride mechanisms in caries prevention. Eur J Oral Sci. (1997) 105:461-5. doi: 10.1111/j.1600-0722.1997.tb00231.x
63. Marquis RE. Antimicrobial actions of fluoride for oral bacteria. Can J Microbiol. (1995) 41:955-64. doi: 10.1139/m95-133
64. Randall JP, Seow WK, Walsh LJ. Antibacterial activity of fluoride compounds and herbal toothpastes on Streptococcus mutans: an in vitro study. Aust Dent J. (2015) 60:368-74. doi: 10.1111/adj.12247
65. Shani S, Friedman M, Steinberg D. Relation between surface activity and antibacterial activity of amine-fluorides. Int J Pharm. (1996) 131:33-9. doi: 10.1016/0378-5173(95)04299-7
66. Patil MM, Lakhkar BB, Patil SS. Curse of fluorosis. Indian J Pediatr. (2018) 85:375-83. doi: 10.1007/s12098-017-2574-z
67. Ekambaram M, Itthagarun A, King NM. Ingestion of fluoride from dentifrices by young children and fluorosis of the teeth–a literature review. J Clin Pediatr Dent. (2011) 36:111-21. doi: 10.17796/jcpd.36.2.3106602470287130
68. Dong H, Yang X, Zhang S, Wang X, Guo C, Zhang X, et al. Associations of low level of fluoride exposure with dental fluorosis among U.S. children and adolescents, NHANES 2015-2016. Ecotoxicol Environ Saf. (2021) 221:112439. doi: 10.1016/j.ecoenv.2021.112439
69. Limeback H, Robinson C. Fluoride therapy. Comp Prev Dent. (2012) 2012:251-82. doi: 10.1002/9781118703762.ch16
70. Meyer-Lueckel H, Grundmann E, Stang A. Effects of fluoride tablets on caries and fluorosis occurrence among 6- to 9-year olds using fluoridated salt. Comm Dent Oral Epidemiol. (2010) 38:315-23. doi: 10.1111/j.1600-0528.2010.00539.x
71. Creeth J, Bosma ML, Govier K. How much is a ‘pea-sized amount'? A study of dentifrice dosing by parents in three countries. Int Dent J. (2013) 63:25-30. doi: 10.1111/idj.12076
72. EFSA. Scientific opinion on dietary reference values for fluoride. EFSA J. (2013) 11:419. doi: 10.2903/j.efsa.2013.3419
73. Martínez-Mier EA. Fluoride: its metabolism, toxicity, and role in dental health. J Evid-Based Compl Alt Med. (2012) 17:28-32. doi: 10.1177/2156587211428076
74. Ullah R, Zafar MS, Shahani N. Potential fluoride toxicity from oral medicaments: a review. Iranian J Basic Med Sci. (2017) 20:841-8. doi: 10.22038/IJBMS.2017.9104
75. NTP. Systematic Review of Fluoride Exposure and Neurodevelopmental and Cognitive Health Effects. Durham, NC: Draft NTP monograph/US Department of Health and Human Services (2019).
76. Guth S, Hüser S, Roth A, Degen G, Diel P, Edlund K, et al. Toxicity of fluoride: critical evaluation of evidence for human developmental neurotoxicity in epidemiological studies, animal experiments and in vitro analyses. Arch Toxicol. (2020) 94:1375-415. doi: 10.1007/s00204-020-02725-2
77. Verma P, Muthuswamy Pandian S. Bionic effects of nano hydroxyapatite dentifrice on demineralised surface of enamel post orthodontic debonding: in-vivo split mouth study. Prog Orthod. (2021) 22:39. doi: 10.1186/s40510-021-00381-5
78. Noguti J, de Oliveira F, Peres RC, Renno AC, Ribeiro DA. The role of fluoride on the process of titanium corrosion in oral cavity. Biometals. (2012) 25:859-62. doi: 10.1007/s10534-012-9570-6
79. Penarrieta-Juanito G, Sordi MB, Henriques B, Dotto MER, Teughels W, Silva FS, et al. Surface damage of dental implant systems and ions release after exposure to fluoride and hydrogen peroxide. J Periodontal Res. (2019) 54:46-52. doi: 10.1111/jre.12603
80. Hu M-L, Zheng G, Zhang Y-D, Yan X, Li X-C, Lin H. Effect of desensitizing toothpastes on dentine hypersensitivity: a systematic review and meta-analysis. J Dent. (2018) 75:12-21. doi: 10.1016/j.jdent.2018.05.012
81. Hu ML, Zheng G, Lin H, Yang M, Zhang YD, Han JM. Network meta-analysis on the effect of desensitizing toothpastes on dentine hypersensitivity. J Dent. (2019) 88:103170. doi: 10.1016/j.jdent.2019.07.008
83. Regulation (EC) No 1223/2009 of the European Parliament and of the Council of 30 November 2009 on cosmetic products 2009. Available online at: https://eur-lex.europa.eu/legal-content/EN/ALL/?uri=celex%3A32009R1223
84. Pritam A, Priyadarshini A, Hussain K, Kumar A, Kumar N, Malakar A. Assessment of nickel and chromium level in gingival crevicular fluid in patients undergoing orthodontic treatment with or without fluoridated tooth paste. J Pharm Bioallied Sci. (2021) 13:1588-90. doi: 10.4103/jpbs.jpbs_302_21
85. Green R, Lanphear B, Hornung R, Flora D, Martinez-Mier EA, Neufeld R, et al. Association between maternal fluoride exposure during pregnancy and IQ scores in offspring in Canada. JAMA Pediatr. (2019) 2019:e191729. doi: 10.1001/jamapediatrics.2019.1729
86. Till C, Green R, Flora D, Hornung R, Martinez-Mier EA, Blazer M, et al. Fluoride exposure from infant formula and child IQ in a Canadian birth cohort. Environ Epidemiology. (2019) 3:395-6. doi: 10.1097/01.EE9.0000610416.37531.62
87. Malin AJ, Lesseur C, Busgang SA, Curtin P, Wright RO, Sanders AP. Fluoride exposure and kidney and liver function among adolescents in the United States: NHANES, 2013–2016. Environ Int. (2019) 2019:105012. doi: 10.1016/j.envint.2019.105012
88. Choi AL, Sun G, Zhang Y, Grandjean P. Developmental fluoride neurotoxicity: a systematic review and meta-analysis. Environ Health Perspect. (2012) 120:1362-8. doi: 10.1289/ehp.1104912
89. Bashash M, Thomas D, Hu H, Angeles Martinez-Mier E, Sanchez BN, Basu N, et al. Prenatal fluoride exposure and cognitive outcomes in children at 4 and 6-12 years of age in Mexico. Environ Health Perspect. (2017) 125:097017. doi: 10.1289/EHP655
90. Enax J, Fabritius H-O, Amaechi BT, Meyer F. Hydroxylapatit als biomimetischer Wirkstoff für die Remineralisation von Zahnschmelz und Dentin. ZWR - Das Deutsche Zahnärzteblatt. (2020) 129:277-83. doi: 10.1055/a-1167-4888
91. Enax J, Epple M. Synthetic hydroxyapatite as a biomimetic oral care agent. Oral Health Prev Dent. (2018) 1:7-19. doi: 10.3290/j.ohpd.a39690
93. Amaechi BT, Phillips TS, Evans V, Enax J, Meyer F. Hydroxyapatite toothpaste for root caries prevention: an pH-cycling study. In: 68th ORCA Congress Zagreb (2021).
94. Sarembe S, Enax J, Morawietz M, Kiesow A, Meyer F. n vitro whitening effect of a hydroxyapatite-based oral care gel. Eur J Dent. 2020 14:335-41. doi: 10.1055/s-0040-1714759
95. Dabanoglu A, Wood C, Garcia-Godoy F, Kunzelmann KH. Whitening effect and morphological evaluation of hydroxyapatite materials. Am J Dent. (2009) 22:23-9.
96. Hojabri N, Kaisarly D, Kunzelmann K-H. Adhesion and whitening effects of P11-4 self-assembling peptide and HAP suspension on bovine enamel. Clin Oral Investig. (2020) 25:3237-47. doi: 10.1007/s00784-020-03654-1
97. Shang R, Kunzelmann KH. Biomimetic tooth-whitening effect of hydroxyapatite-containing mouthrinses after long-term simulated oral rinsing. Am J Dent. (2021) 34:307-12.
98. Gümüştaş B, Dikmen B. Effectiveness of remineralization agents on the prevention of dental bleaching induced sensitivity: a randomized clinical trial. Int J Dent Hyg. (2021). doi: 10.1111/idh.12524
99. Yazdani J, Ahmadian E, Sharifi S, Shahi S, Maleki Dizaj S. A short view on nanohydroxyapatite as coating of dental implants. Biomed Pharmacother. (2018) 105:553-7. doi: 10.1016/j.biopha.2018.06.013
100. Oliveira HL, Da Rosa WLO, Cuevas-Suárez CE, Carreño NLV, da Silva AF, Guim TN, et al. Histological evaluation of bone repair with hydroxyapatite: a systematic review. Calcif Tissue Int. (2017) 101:341-54. doi: 10.1007/s00223-017-0294-z
101. Jardim RN, Rocha AA, Rossi AM, de Almeida Neves A, Portela MB, Lopes RT, et al. Fabrication and characterization of remineralizing dental composites containing hydroxyapatite nanoparticles. J Mech Behav Biomed Mater. (2020) 109:103817. doi: 10.1016/j.jmbbm.2020.103817
102. Hegazy SA, Salama IR. Antiplaque and remineralizing effects of Biorepair mouthwash: a comparative clinical trial. Pediatr Dent J. (2016) 26:89-94. doi: 10.1016/j.pdj.2016.05.002
103. Harks I, Jockel-Schneider Y, Schlagenhauf U, May TW, Gravemeier M, Prior K, et al. Impact of the daily use of a microcrystal hydroxyapatite dentifrice on de novo plaque formation and clinical/microbiological parameters of periodontal health. A randomized trial. PLoS ONE. (2016) 11:e0160142. doi: 10.1371/journal.pone.0160142
104. Hagenfeld D, Prior K, Harks I, Jockel-Schneider Y, May TW, Harmsen D, et al. No differences in microbiome changes between anti-adhesive and antibacterial ingredients in toothpastes during periodontal therapy. J Periodontal Res. (2019) 54:645. doi: 10.1111/jre.12645
Keywords: hydroxyapatite, caries, children, remineralization, teeth, toothpaste, oral care
Citation: Meyer F, Enax J, Amaechi BT, Limeback H, Fabritius H-O, Ganss B, Pawinska M and Paszynska E (2022) Hydroxyapatite as Remineralization Agent for Children's Dental Care. Front. Dent. Med. 3:859560. doi: 10.3389/fdmed.2022.859560
Received: 21 January 2022; Accepted: 23 March 2022;
Published: 25 April 2022.
Edited by:
Ali Mentes, Marmara University, TurkeyReviewed by:
Jithendra Tharanga Ratnayake, University of Otago, New ZealandSivakumar Nuvvula, Narayana Dental College and Hospital, India
Copyright © 2022 Meyer, Enax, Amaechi, Limeback, Fabritius, Ganss, Pawinska and Paszynska. This is an open-access article distributed under the terms of the Creative Commons Attribution License (CC BY). The use, distribution or reproduction in other forums is permitted, provided the original author(s) and the copyright owner(s) are credited and that the original publication in this journal is cited, in accordance with accepted academic practice. No use, distribution or reproduction is permitted which does not comply with these terms.
*Correspondence: Frederic Meyer, ZnJlZGVyaWMubWV5ZXImI3gwMDA0MDtkcndvbGZmZ3JvdXAuY29t
†These authors have contributed equally to this work