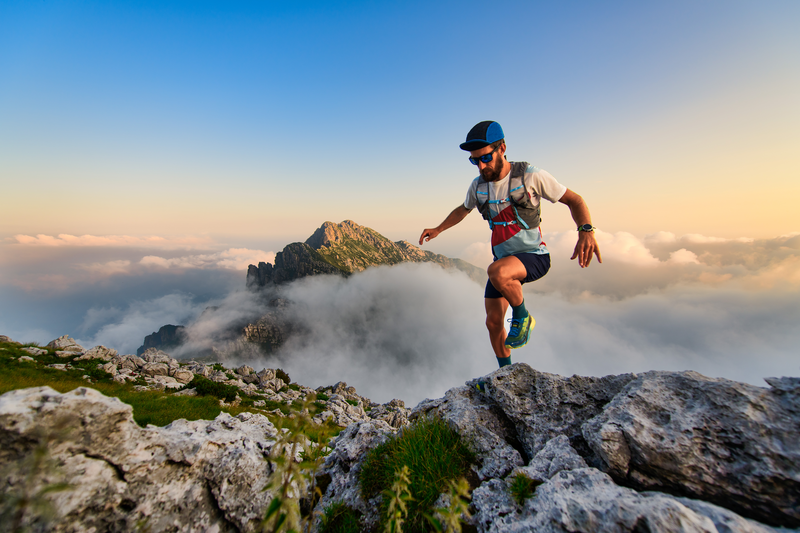
94% of researchers rate our articles as excellent or good
Learn more about the work of our research integrity team to safeguard the quality of each article we publish.
Find out more
REVIEW article
Front. Dent. Med , 29 November 2021
Sec. Regenerative Dentistry
Volume 2 - 2021 | https://doi.org/10.3389/fdmed.2021.780973
This article is part of the Research Topic Epigenetic Regulation of Stem Cells Derived from Craniofacial Tissues View all 8 articles
Maxillofacial bone defects are common medical problems caused by congenital defects, necrosis, trauma, tumor, inflammation, and fractures non-union. Maxillofacial bone defects often need bone graft, which has many difficulties, such as limited autogenous bone supply and donor site morbidity. Bone tissue engineering is a promising strategy to overcome the above-mentioned problems. Osteoimmunology is the inter-discipline that focuses on the relationship between the skeletal and immune systems. The immune microenvironment plays a crucial role in bone healing, tissue repair and regeneration in maxillofacial region. Recent studies have revealed the vital role of immune microenvironment and bone homeostasis. In this study, we analyzed the complex interaction between immune microenvironment and bone regeneration process in oral and maxillofacial region, which will be important to improve the clinical outcome of the bone injury treatment.
Bone resorption and bone formation are largely regulated by a variety of immune responses under normal and pathogenic conditions. Full understanding of the principles in bone homeostasis is vital to treat patients with traumatic injuries, osteonecrosis, arthritis, bone infection, osteoporosis, metabolic bone disease, tumors (1). Maxillofacial bone is a dynamic tissue and maintains a constant balance between bone loss and subsequent repair with participation of the immune system to a large extent (2). Over the years, maxillofacial bone defects are difficult and challenging problems for maxillofacial surgeons resulting from tumor, trauma, congenital defects, reconstructive surgery, non-union of fractures, infection, or periodontal disease. Repair, reconstruction together with regeneration in bone defects remain a challenge in the oral and maxillofacial region (3). Osteo-immunology has opened the field that explored the complex cellular and molecular networks involved in oral maxillofacial osteolytic diseases, explored the interaction between metabolism of bone and immune response and provided background for the study of chronic inflammatory disease associated with bone loss (4, 5). Periodontal health depends on the local balance among immune cells, cytokines and mediators (6). Simultaneously, bone homeostasis is closely related to immune cells and immune derived cytokines. The challenge is to require this homeostatic equilibrium of the oral microbiome, the moderate inflammation and the adaptive alveolar bone remodeling. The main purpose of this review is to explain the effect of osteo-immunology on maxillofacial bone.
Bone homeostasis is a highly coordinated process responsible for bone formation, bone repair and bone remodeling. In addition to the traditional bone cells, immune cells containing neutrophils, B cells, macrophages and T cells, were also implicated in remodeling of bone (7). In osteolytic inflammatory diseases, such as osteoarthritis of the temporomandibular joint, periodontal disease, apical periodontitis and maxillofacial bone sarcomas, inflammation leads to tissue destruction, especially bone loss by the continuous release of osteoclastogenic mediators. Mandible and maxilla are the only bones direct exposure to a microbial contaminated environment, such as periodontal disease or during and after surgical procedures, containing tooth extraction, some resection of jawbone tumors and so on (7). The acute innate immune response is crucial in the early stage of bone healing after injury, which depends on immune cells and cytokines (8, 9). Immune cells are essential in bone repair by sensing the extracellular signals, eventually drive bone remodeling by regulating osteogenesis or osteoclastogenesis (10). Therefore, interaction between local stem cells and immune cells in the oral microenvironment may modify the regenerative process (11) (see Figure 1).
Figure 1. Cytokines and immune cells are important factors in regulating bone resorption in bone homeostasis. Osteoclasts and osteoblasts differentiation and activation are driven by immune cells, including B cells, T cells, dendritic cells, macrophages and neutrophils, and cytokines, containing TNF-a, IL-1, IL-6, IL-17 and IFN-γ.
Neutrophils, which are a type of polymorphonuclear leukocytes, are the most abundant immune cells in human peripheral blood (12). Neutrophils are universally acknowledged as one of the major participants in acute inflammation (13, 14). Neutrophils often act as the front line of defense to be recruited to an inflammatory site to fight off pathogens by eliminating pathogens, cell debris as well as microorganisms (15, 16). Neutrophils play a critical role in the acute inflammatory response and wound healing, especially at the beginning of hemostasis and inflammation (12). Neutrophils are also all importantly influential in chronic inflammatory or aging-related diseases, including periodontitis, diabetes and rheumatoid arthritis (17). Furthermore, periodontitis is related to increased risk of certain systemic diseases, such as atherosclerosis and rheumatoid arthritis (18). Neutrophils are involved in periodontal inflammatory responses. Neutrophils are accumulated in periodontal tissues and also enriched in the fluid of gingival crevice (19, 20). Neutrophils are increased in the process of inflammation and are vital for periodontal tissue homeostasis (21, 22). Neutrophils can secrete cytokines that enhance the survival rate of B cells and plasma cells, which is associated with periodontitis and has a causal relationship with periodontal bone loss in mice (23). Neutrophils show heterotypic adhesion to osteoblasts and regulate the function of osteoblasts in osteoimmunological regulation of periodontal diseases (24, 25). Neutrophils play an important part in the pathogenesis of bisphosphonate-associated osteonecrosis of the jaws and impaired normal wound healing (26).
Macrophages are cells of innate immunity that are present in nearly all tissues, where they make a substantial contribution to development, homeostasis and regeneration (27, 28). In fracture healing, macrophages migrated into the fracture area and had an impact on the long term outcome of bone repair (29). Macrophages can not only remove the temporary fibrin matrix, necrotic cells and damaged tissues via phagocytosis at the fracture site but also recruit vascular progenitor cells and mesenchymal stem cells (MSCs) from the bone marrow, periosteum and circulation (30). Therefore, bone repair requires a long-time regenerative response to achieve anatomical and functional recovery of bone. Macrophages are essential for the initiation of bone repair and also participate in the regulation of bone regeneration during normal bone homeostasis (31). Bone macrophages in vivo are close to osteoblasts, can regulate bone formation, support fracture healing and play a variety of roles in the potential role of osteocyte proliferation in bone biology and the regulation of bone metastasis (27). Macrophages are responsible for the homeostasis and functions of the alveolar bone (32). Macrophages are only a few in periodontal tissues, but they participate in the pathogenesis of periodontitis by initiating or eliminating inflammation, mediating alveolar bone resorption and localization (33–35). Therefore, the monocyte/macrophage population in alveolar bone participates in the regulation of MSCs and bone homeostasis, which promotes osteogenic differentiation and inhibits adipogenic differentiation of MSCs (36). Together, these results suggest that macrophages function in regulating physiological bone formation and homeostasis.
Dendritic cells are a special type of antigen-presenting cells that can capture, process and present antigens to lymphocytes, so as to initiate and regulate the adaptive immune response (37, 38). Dendritic cells activate a protective antibody response, thereby reduce bone loss (39). Immature dendritic cells may directly participate in the regulation of osteoclasts and lead to bone decline in histiocytosis, rheumatoid arthritis or periodontitis (40, 41). Chemokines secreted by dendritic cells can attract monocytes and neutrophils to sites of inflammation, so as to enhance inflammation and stimulate the expression of osteoclastogenetic factors (42). Dendritic cells can transdifferentiate into osteoclasts and furthermore cause bone resorption in patients with periodontal inflammation (43, 44). Dendritic cells can enhance periodontal bone loss by upregulating Th17 or Th1 response (45). Results in dendritic cells-deficient mice confirmed that dendritic cells deficiency could contribute to bone necrosis after tooth extraction (46). Dendritic cells are essential in initiation and regulation of immune responses in the clinical case of oral cavity (46, 47). Previous studies reported insufficient innate immune response and colonization of oral bacterial communities in bisphosphonate-related osteonecrosis of the jaw (48). Dendritic cells also play a major role in the oral mucosal barrier immunity of the oral cavity and the gut mucosa (49).
T lymphocytes participate in host defense and control the development of immune-mediated inflammatory disease (50). According to the original concept of bone immunology, T cells are critically involved in the mediation of inflammatory repose guided bone loss. Activated T cells indirectly or directly regulate bone health and bone remodeling by secreting various cytokines and factors (50, 51). For example, activated T cells are primary sources of receptor activator of TNF-α and nuclear factor-κB ligand (RANKL) responsible for bone destruction observed under pathological and inflammatory conditions (52). Activated CD8+ and CD4+T cells secrete interleukin-1 (IL-1), tumor necrosis factor (TNF-α), receptor activator of nuclear factor-κ B ligand (RANKL), IL-6, and IL-17 to promote the formation of osteoclast (53). FoxP3+ CD8+ T-lymphocytes produce CTLA-4 and interferon-γ (IFN-γ) to suppress osteoclastogenesis while produce RANKL to enhance osteoclastogenesis (54). However, CD8+ T cells can produce Wnt10b to mediates activation of Wnt signal while γδ T cells secrete IL-17A to accelerate bone regeneration (55). Wnt signaling plays crucial roles in postnatal bone formation. Wnt signaling can promote the commitment of mesenchymal stem cells (MSCs) to osteoblastic lineage and promotes differentiation and bone formation at the critical steps of osteoblast differentiation (56). T lymphocytes and B lymphocytes can result in the production of RANKL, which causes osteoclasts to induce obvious alveolar bone resorption, even tooth loss (57).
B cells are an important branch of the adaptive immune system and can also present antigens and secrete antibodies and cytokines (58). Recent studies have disclosed a regulatory effect of B cells, indicating that B cells affect osteoclasts by producing cytokines (59, 60). It had been reported that B cells can produce TGF-β, IL-6, OPG to inhibit osteoclast formation (61). It is reported that several B cells in healthy gingiva may have an important role to play in preventing bone loss caused by inflammation of periodontium (62). However, B cells can express TNF-α, IL-6, and RANKL to promote osteoclast formation and osteoclastogenesis (60). B cells have been closely related to periodontal homeostasis and disease (57). B cells express RANKL for alveolar bone homeostasis in homogeneous gingival tissue during periodontal disease (63, 64). B cells also play a vital role in attachment loss and alveolar bone resorption in periodontitis in mice, which may be due to activation of B cells and the expression of RANKL in the gingiva (65). Furthermore, B cells deficiency reduces alveolar bone resorption during periodontitis (66). Furthermore, memory B lymphocytes can lead to bone damage in rheumatoid arthritis (67). And B lymphocytes in periodontitis may result in chronic systemic inflammation (68).
Cytokines are intercellular regulators at systemic and local level. Cytokines are derived from immunocompetent cells such as monocytes and T lymphocytes. Chemokines manage the location and migration of immune cells and are essential in the function of the innate immune system. Chemokines can also affect bone marrow to release innate immune cells throughout development, homeostasis, and inflammation (69). Periodontal tissue such as epithelial and endothelial cells as well as fibroblasts are also involved in cytokine formation during inflammatory responses (70, 71). Besides, various cytokines are directly related to the occurrence of osteoporosis in animal models and patients (72). Several chemokines produced by osteophytic tumor cells can promote osteoclasts-mediated bone resorption and promote the osteoclast precursors recruitment and osteoclast precursors differentiation (73).
TNF-α mainly secreted by monocytes, can stimulate the activity of mature osteoclasts, and attract other monocytes (74). Furthermore, TNF-α frequently appeared in the tumor microenvironment and is mainly derived from tumor cells and tumor-associated macrophages (75). Once cancer cells are detained in bone, the bone stores diverse growth factors and cytokines and thus provides an extremely fertile environment for cell growth (76). Invasive tumor cells secrete TNF-α, interleukins and chemokines and change the bone microenvironment, which directly induce osteoclasts and/or promote RANKL expression in osteoblasts and stromal stem cells (77). It was firstly described that TNF-α inhibited bone formation in neonatal rat calvarial organ cultures in 1987 (78). Recently, TNF-α plays a critical role in the pathogenesis of inflammatory bone loss by stimulating osteoclasts bone resorption and inhibiting osteoblast bone formation (79, 80). Further studies showed the inhibitory effects of TNF-α in recruitment of osteoblast progenitors, genes expression produced by mature osteoblasts, and the active influence in osteoblast apoptosis via nuclear factor kB (NF-κB) pathway (81). In many chronic and inflammatory disease, TNF-α plays a negative role in regulating bone homeostasis (79, 82, 83). TNF-α was obviously upregulated in the process of periodontitis (84), which is actively involved in the destruction of periodontal tissues by regulating the activities of leukocytes, osteoclasts (85). Furthermore, the expression levels of TNF-α was closely associated with the severity of periodontitis (86) (see Figure 2).
Figure 2. Cytokines regulation of bone resorption in periodontitis. The expression of cytokines in periodontitis increase bone resorption by osteoclasts, such as TNF-a, IL-1β, IL-6, IL-17 and IFN-γ.
IL-1 plays vital role in immune and inflammatory responses (87). The systemic IL-1 has important roles in regulation of basic metabolic rate, iron metabolism and bone remodeling (88). IL-1 stimulates bone resorption by promoting osteoclast activation (89, 90) and mediates osteoclatogenic effects of TNF-α by up-regulating the expression of RANKL (91). IL-1 also has a prominent role in the pathogenesis of periodontitis (85). The IL-1 family contains two main members, IL-1α and IL-1β. IL-1α promoted osteoclast differentiation by stimulating prostaglandin E2 (92). IL-1α can also exert its biological effects on the alveolar bone modeling process of tooth eruption by enhancing RANKL and TNF-α expressions (93). Previous studies showed that IL-1α is an inducer, which could up-regulate the expression of matrix metalloproteinases (MMPs), such as MMP 13, 9, 7, 1, and 3 in the process of infection or the formation of endodontic and periodontal osteolytic lesions (94–96). IL-1β was detected to inhibit osteogenetic and adipogenetic differentiation of MSCs (97). As one of the important pro-inflammatory mediators, IL-1β is increased in the early stage of fracture healing (98). IL-1β affects alveolar bone resorption in ligature-induced chronic periodontitis by enhancing osteoclastic differentiation (99) (see Figure 2).
IL-6 has been reported to influence osteoclastic differentiation and bone resorption (100, 101). IL-6 exerts a significant impact on immune responses and certain oncological conditions (102). There are studies suggesting that IL-6 is a potential biomarker for oral squamous cell carcinoma in oral cavity and oropharynx (103). In bone, IL-6 is derived from osteoblasts and acts as an important regulator of osteoclastic development (104) and physiologically regulates bone metabolism (105). A recent study indicates that IL-6 induced osteogenesis of stem cells via signal transducer and activator of transcription factor (106). Mounting evidences have demonstrated that IL-6 directly promotes the formation of osteoclast through a RANKL-independent mechanism (101). It is recorded that IL-6 levels in periimplantitis was significantly higher than that in healthy subjects (107). Mice with IL-6 deficiency were also resistant to periodontal bone damage (108). Oral squamous cell carcinoma can not only produce IL-6 but also induce stromal cells to produce IL-6, and provide a suitable microenvironment for osteoclastogenesis (109). IL-6 is produced by oral cancer cells as a precursor protein that induced osteoclastic bone resorption and deficient bone formation through RANKL expression in stromal cells (110). It has been found that IL-6 appears to be a regulator of bone invasion and a direct critical driver of tumor growth and metastasis by oral cancer (111).
IL-17 participates in both acute and chronic inflammatory responses, elicits similar host defense against extracellular bacterial infections and is crucial in inflammatory conditions including autoimmune diseases, cancer and metabolic disorders (112). IL-17 can stimulate osteoclastic bone resorption, suppress bone formation, and result in bone loss in osteoporosis (113, 114). IL-17 accelerates bone loss by promoting pro-osteoclastogenic cytokines accumulation containing TNF-α and RANKL produced by osteoblastic cells (115). Low expression of IL-17 inhibited the ability of bacteria in diabetic animals to induce inflammation and promote alveolar bone absorption in normal germ-free recipients (116). Interestingly, IL-17 expression is correlated with dendritic cells and increased in patients with chronic periodontitis (45). IL-17 can stimulate the synthesis of pro-inflammatory mediators including IL-6 and RANKL or indirectly promote periodontal bone loss (117). Rheumatoid arthritis is a systemic autoimmune disease which regulates inflammatory cytokines expression in the periodontal tissue, such as IL-1, TNF-α, IL-6, and IL-17 (118–121).
IFN-γ is the essential pro-inflammatory cytokines which is involved in the innate and adaptive immune responses (122). Data identify IFN-γ as the major effector cytokine driving pathogenesis in patients with immune-mediated bone diseases, such as postmenopausal osteoporosis and rheumatoid arthritis (53). IFN-γ plays dual effect in osteoclastogenesis. Substantial evidence demonstrated that IFN-γ enhanced bone resorption and led to bone loss under the pathological conditions (123, 124). In contrast, IFN-γ was a key negative regulator of osteoclastogenesis, and mediated the inhibition by IL-2 (BIL-2) in vitro (61, 125). Additional studies revealed that IFN-γ decreased serum calcium concentration and osteoclastic resorption in nude mice (126). It is reported that IFN-γ is effective in treating osteopetrosis by directly suppressing osteoclast differentiation but indirectly promoting bone resorption (127). Therefore, IFN-γ indirectly enhances osteoclastic factors via stimulating immune responses, otherwise, the lack of IFN-γ decreases alveolar bone loss in mice (128).
Destruction of bone homeostasis caused by immune dysfunction provides a clue to seek the therapeutic targets through osteoimmunology. Over the past decade, osteoimmunology plays a vital role in maintaining an adequate pool of cytokine and circulating immune cells to protect bone homeostasis. It provides a new inter-discipline to understand the relationship between the immune and the skeletal systems. Thus, a better understanding of the nexus between the immune and the skeletal systems should be at heart of future research in the area. Research in the field of osteoimmunology would pave path for novel therapeutics for treating bone losses resulted from different inflammation.
LN and JHY wrote the manuscript and conceived and designed insights and discussion. ZHL, LF, YK, YQW, and JTW provided helpful comments on a previous draft. All authors contributed to the article and approved the submitted version.
This work was funded by the National Natural Science Foundation of China [Grant Numbers: 81900962 (YQW) and 82170940 (JHY)].
The authors declare that the research was conducted in the absence of any commercial or financial relationships that could be construed as a potential conflict of interest.
All claims expressed in this article are solely those of the authors and do not necessarily represent those of their affiliated organizations, or those of the publisher, the editors and the reviewers. Any product that may be evaluated in this article, or claim that may be made by its manufacturer, is not guaranteed or endorsed by the publisher.
1. Loi F, Jukka P, Lin TH, Yao ZY, Goodman SB. Inflammation, fracture and bone repair. Bone. (2016) 86:119–30. doi: 10.1016/j.bone.2016.02.020
2. Alvarez C, Cavalla F, Luis AC, Marcela H, Dominique H, Gustavo PG, et al. Osteoimmunology of oral and maxillofacial diseases: translational applications based on biological mechanisms. Front Immunol. (2019) 10:1664. doi: 10.3389/fimmu.2019.01664
3. Fliefel R, Ehrenfeld M, Otto S. Gene therapy for bone defects in oral and maxillofacial surgery: a systematic review and meta-analysis of animal studies. Stem Cells Dev. (2017) 26:215–30. doi: 10.1089/scd.2016.0172
5. Schett G. Bone erosion in rheumatoid arthritis: mechanisms, diagnosis and treatment. Nat Rev Rheumatol. (2012) 8:656–64. doi: 10.1038/nrrheum.2012.153
6. Candel-Martí ME, Alegre-Domingo T, Ata-Ali J, Peñarrocha-Diago M. Interleukins IL-6, IL-8, IL-10, IL-12 and periimplant disease. An update. Medicina Oral Patologia Oral y Cirugia Bucal. (2011) 16:e518–21. doi: 10.4317/medoral.16.e518
7. Einhorn T. Fracture healing: mechanisms and interventions. Nat Rev Rheumatol. (2015) 11:45–54. doi: 10.1038/nrrheum.2014.164
8. Kim JM, Stavre ZN, Matthew B, Greenblatt, Shim JH. Osteoblast-osteoclast communication and bone homeostasis. Cells. (2020) 9:2073. doi: 10.3390/cells9092073
9. Kiernan CH, Brama P, Farrell E. The immune response to allogeneic differentiated mesenchymal stem cells in the context of bone tissue engineering. Tissue Eng: Part B. (2018) 24:75–83. doi: 10.1089/ten.teb.2017.0175
10. Kovach T, Lobo P, Cui QJ. Interactions between MSCs and immune cells: implications for bone healing. J Immunol Res. (2015) 2015:752510. doi: 10.1155/2015/752510
11. Kizil C, Brand M. Effects of inflammation on stem cells: together they strive? EMBO Rep. (2015) 16:416–26. doi: 10.15252/embr.201439702
12. Evrard M, Chong SZ, Teng KW, Becht E, Chen JM, Sieow JJ, et al. Developmental analysis of bone marrow neutrophils reveals populations specialized in expansion, trafficking, and effector functions. Immunity. (2018) 48:364–79. doi: 10.1016/j.immuni.2018.02.002
13. Broughton G, Attinger CE. Wound healing: an overview. Plast Reconstr Surg. (2006) 117:1e–S−32e–S. doi: 10.1097/01.prs.0000222562.60260.f9
14. Kolaczkowska E. Neutrophil recruitment and function in health and inflammation. Nat Rev Immunol. (2013) 13, 159–175. doi: 10.1038/nri3399
15. Ahn MH, Chwae YG, Jung JH, Suh CH, Kwon JE, Kim HA. Neutrophil extracellular traps may contribute to the pathogenesis in adult-onset still disease. J Rheumatol. (2019) 2019:12. doi: 10.3899/jrheum.181058
16. Dinarello. The IL-1 family of cytokines and receptors in rheumatic diseases. Nat Rev Rheumatol. (2019) 15:612–32. doi: 10.1038/s41584-019-0277-8
17. Hajishengallis G, Hajishengallis E, Lambris J. Neutrophil homeostasis and inflammation: novel paradigms from studying periodontitis. J Leukoc Biol. (2015) 98:539–48. doi: 10.1189/jlb.3VMR1014-468R
18. Hajishengallis. Periodontitis: from microbial immune subversion to systemic inflammation. Nat Rev Immunol. (2015) 15:30–44. doi: 10.1038/nri3785
19. Cooper PR, Chapple IL. Neutrophil extracellular traps as a new paradigm in innate immunity: friend or foe? Periodontology. (2013) 63:165–97. doi: 10.1111/prd.12025
20. Nussbaum G. How has neutrophil research improved our understanding of periodontal pathogenesis? J Clin Periodontol. (2011)38 49–59 doi: 10.1111/j.1600-051X.2010.01678.x
21. Carcuac O. Composition of human peri-implantitis and periodontitis lesions. J Dental Res. (2014) 93:1083–8. doi: 10.1177/0022034514551754
22. Hajishengallis G. New developments in neutrophil biology and periodontitis. Periodontology. (2020) 822:78–92. doi: 10.1111/prd.12313
23. Abe T, Benakanakere MR, Maekawa T, Kinane DF, Cancro MP, Korostoff JM, et al. The B cell-stimulatory cytokines BLyS and APRIL are elevated in human periodontitis and are required for B cell-dependent bone loss in experimental murine periodontitis. J Immunol. (2015) 195:1427–35. doi: 10.4049/jimmunol.1500496
24. Allaeys I, Picard S, Pouliot M, Borgeat P, Poubelle PE. Osteoblast retraction induced by adherent neutrophils promotes osteoclast bone resorption: implication for altered bone remodeling in chronic gout. Lab Invest. (2011) 91:905–20. doi: 10.1038/labinvest.2011.46
25. Lewkowicz P.rzygodzka P., Cwiklińska H., Cichalewska M, Matysiak M., Selmaj K., Lewkowicz P. Induction of human IL-10-producing neutrophils by LPS-stimulated Treg cells and IL-10. Mucosal Immunol. (2016) 9, 364–378. doi: 10.1038/mi.2015.66
26. Hagelauer N, Pabst AM, Ziebart T, Ulbrich H, Walter C. In vitro effects of bisphosphonates on chemotaxis, phagocytosis, and oxidative burst of neutrophil granulocytes. Clinical Oral Invest. (2015) 19:139–48. doi: 10.1007/s00784-014-1219-0
27. Sinder BP, Pettit AR, McCauley LK. Macrophages: their emerging roles in bone. J Bone Miner Res. (2015) 30:2140–9. doi: 10.1002/jbmr.2735
28. Murray PJ, Wynn TA. Protective and pathogenic functions of macrophage subsets. Nauret Rev Immunol. (2011) 11:723–37. doi: 10.1038/nri3073
29. Schlundt C, El Khassawna T, Serra A, Dienelt A, Wendler S, Schell H, et al. Macrophages in bone fracture healing: their essential role in endochondral ossification. Bone. (2018) 106:78–89. doi: 10.1016/j.bone.2015.10.019
30. Pajarinen J, Gibon E, Kohno Y, Maruyama M, Nathan K, Lu L, et al. Mesenchymal stem cell-macrophage crosstalk and bone healing. Biomaterials. (2019) 196:80–9. doi: 10.1016/j.biomaterials.2017.12.025
31. Odgren PR, Reyes-Gutierrez P. The cast of clasts: catabolism and vascular invasion during bone growth, repair, and disease by osteoclasts, chondroclasts, and septoclasts. Connect Tissue Res. (2016) 57:161–74. doi: 10.3109/03008207.2016.1140752
32. Jiang N, Chen M, Zheng Y, Zhou J, Kim SG, Embree MC, et al. Periodontal ligament and alveolar bone in health and adaptation: tooth movement. Front Oral Biol. (2016) 18:1–8. doi: 10.1159/000351894
33. Dutzan N, Greenwell-Wild T, Moutsopoulos NM. Characterization of the human immune cell network at the gingival barrier. Mucosal Immunol. (2016) 9:1163–72. doi: 10.1038/mi.2015.136
34. Hasturk H, Dyke TE. Oral inflammatory diseases and systemic inflammation: role of the macrophage. Front Immunol. (2012) 3:118. doi: 10.3389/fimmu.2012.00118
35. Lavin Y, Rahman A, Merad M. Regulation of macrophage development and function in peripheral tissues. Nat Rev Immunol. (2015) 15:731–44. doi: 10.1038/nri3920
36. Lin WM, Zhang DT, Zhang XH, Qi XY, Wang Q, Chen YQ, et al. Mapping the immune microenvironment for mandibular alveolar bone homeostasis at single-cell resolution. Bone Reseach. (2021) 9:17. doi: 10.1038/s41413-021-00141-5
37. Song L, Guo LJ, Graves DT. The function of dendritic cells in modulating the host response. Mol Oral Microbiol. (2018) 33:13–21. doi: 10.1111/omi.12195
38. Dai JT, Reinert S, Alexander D. Jaw periosteal cells seeded in beta-tricalcium phosphate inhibit dendritic cell maturation. Biomolecules. (2020) 10:887. doi: 10.3390/biom10060887
39. Xiao WM, Pacios S, Alnammary M, Barger LA, Wang Y, Wu YY, et al. FOXO1 deletion reduces dendritic cell function and enhances susceptibility to periodontitis. Am J Pathol. (2015) 185:1085–93. doi: 10.1016/j.ajpath.2014.12.006
40. Lapérine O, Guicheux J, Beck-Cormier S, Lesclous P. Dendritic-cell-derived osteoclasts: a new game changer in bone-resorption-associated diseases. Drug Discov Today. (2016) 21:1345–54. doi: 10.1016/j.drudis.2016.04.022
41. Rivollier A, Tebib J, Piperno M, Aitsiselmi T, Rabourdin-Combe C, Jurdic P, et al. Immature dendritic cell transdifferentiation into osteoclasts: a novel pathway sustained by the rheumatoid arthritis microenvironment. Blood. (2004) 104:4029–37. doi: 10.1182/blood-2004-01-0041
42. Oliveira SD, Candel S, Renshaw SA, Mulero V, Calado A. Cxcl8 (IL-8) mediates neutrophil recruitment and behavior in the zebrafish inflammatory response. J Immunol. (2013) 190:4349–59. doi: 10.4049/jimmunol.1203266
43. Khosla S. A crisis in the treatment of osteoporosis. J Bone Miner Res. (2016) 31:1485–7. doi: 10.1002/jbmr.2888
44. Constantino J, Falcão A, Neves BM, Cruz MT. Dendritic cell-based immunotherapy: a basic review and recent advances. Immunol Res. (2017) 65:798–810. doi: 10.1007/s12026-017-8931-1
45. Souto GR, Abreu M, Costa FO, Mesquita RA. Pro-inflammatory, Th1, Th2. Th17 cytokines and dendritic cells: a cross-sectional study in chronic periodontitis. PLoS ONE. (2014) 9:e91636 doi: 10.1371/journal.pone.0091636
46. Elsayed R, Cutler CW, Arce R, Gerber J, Celis E, Sultan H, et al. Role of dendritic cell-mediated immune response in oral homeostasis: a new mechanism of osteonecrosis of the jaw. FASEB J. (2020) 34:2595–608. doi: 10.1096/fj.201901819RR
47. Nudel I, Furmanov K, Arizon M, Clausen BE, Wilensky A, Hovav AH. Dendritic cells in distinct oral mucosal tissues engage different mechanisms to prime CD8+ T cells. J Immunol. (2011) 186:891–900. doi: 10.4049/jimmunol.1002943
48. Pushalkar S, Kurago Z, Ramanathapuram LV, Matsumura S, Fleisher KE, Glickman R, et al. Oral microbiota and host innate immune response in bisphosphonate-related osteonecrosis of the jaw. Int J Oral Sci. (2014) 6:219–26. doi: 10.1038/ijos.2014.46
49. Abbasi J. Amid osteoporosis treatment crisis, experts suggest addressing patients' bisphosphonate concerns. JAMA. (2018) 319:2464–6. doi: 10.1001/jama.2018.7097
50. Srivastava RK, Mishra PK. Immunoporosis: immunology of osteoporosis-role of T cells. Front Immunol. (2018) 9:657. doi: 10.3389/fimmu.2018.00657
51. Titanji K, Foster A, Sheth AN, Lennox JA, Knezevic A, Shenvi N, et al. T-cell receptor activator of nuclear factor-kappaB ligand/osteoprotegerin imbalance is associated with HIV-induced bone loss in patients with higher CD4+ T-cell counts. AIDS. (2018) 32:885–94. doi: 10.1097/QAD.0000000000001764
52. Kawai M, Khosla S, Rosen CJ. Emerging therapeutic opportunities for skeletal restoration. Nat Rev Drug Discov. (2011) 10:141–56. doi: 10.1038/nrd3299
53. Tang MJ, Luo GJ, Yu XJ. Interferon-gamma-mediated osteoimmunology. Front Immunol. (2018) 9:1508. doi: 10.3389/fimmu.2018.01508
54. Buchwald ZS, DiPaolo R, Pagadala MS, Aurora R. Osteoclast activated FoxP3+ CD8+ T-cells suppress bone resorption in vitro. PLoS ONE. (2012) 7:e38199. doi: 10.1371/journal.pone.0038199
55. Ono T, Nakashima T, Nitta T, Hori S, Iwakura Y, Takayanagi H. IL-17-producing γδ T cells enhance bone regeneration. Nat Commun. (2016) 7:10928. doi: 10.1038/ncomms10928
56. Marc. Roles of non-canonical wnt signalling pathways in bone biology. Int J Mol Sci. (2021) 19:10840. doi: 10.3390/ijms221910840
57. Figueredo CM, Love RM. T and B Cells in periodontal disease: new functions in a complex scenario. Int J Mol Sci. (2019) 20:3949. doi: 10.3390/ijms20163949
58. Rajakumar SA, Lee KK, Grandal I, Merico D, Liu CC, Allo B, et al. B cell acute lymphoblastic leukemia cells mediate RANK-RANKL–dependent bone destruction. Sci Transl Med. (2020) 12:eaba5942 doi: 10.1126/scitranslmed.aba5942
59. Zouali M. The emerging roles of B cells as partners and targets in periodontitis. Autoimmunity. (2017) 50:61–70. doi: 10.1080/08916934.2016.1261841
60. Manilay JO. Tight relationships between B lymphocytes and the skeletal system. Trends Mol Medicine. (2014) 20:405–12. doi: 10.1016/j.molmed.2014.03.003
61. Choi Y. B cells activated in the presence of Th1 cytokines inhibit osteoclastogenesis. Exp Mol Med. (2003) 35:385–92. doi: 10.1038/emm.2003.51
62. Mahanonda R, Subbalekha K, Sa-Ard-Iam N, Rattanathammatada W, Thawanaphong S, Rerkyen P, et al. Human memory B cells in healthy gingiva, gingivitis, and periodontitis. J Immunol. (2016) 197:715–25. doi: 10.4049/jimmunol.1600540
63. Han YJ, Miao YB, Shi T, Lin XP. Improved RANKL production by memory B cells: a way for B cells promote alveolar bone destruction during periodontitis. Int Immunopharmacol. (2018) 64:232–7. doi: 10.1016/j.intimp.2018.08.033
64. Kawai T, Hosokawa Y, Makihira S, Seki M, Karimbux NY, Goncalves RB, et al. B and T lymphocytes are the primary sources of RANKL in the bone resorptive lesion of periodontal disease. Am J Pathol. (2006) 169:987–98. doi: 10.2353/ajpath.2006.060180
65. Oliver-Bell MJ, MacLeod MK, Planell A, Campbell L, Nibbs RJ, Garside P, et al. Periodontitis in the absence of B cells and specific anti-bacterial antibody. Mol Oral Microbiol. (2015) 30:160–9. doi: 10.1111/omi.12082
66. Baker PJ, Tiffany M, Roopenian DC. B cell IgD deletion prevents alveolar bone loss following murine oral infection. Interdiscipl Perspect Infect Dis. (2009) 2009:864359. doi: 10.1155/2009/864359
67. Zundler S. Interleukin-12: functional activities and implications for disease. Cytokine Growth Factor Rev. (2015) 26:559–68. doi: 10.1016/j.cytogfr.2015.07.003
68. Jagannathan M, Liang YM, Shin HJ, Hetzel JT, Kantarci A, Rubin D, et al. TLR cross-talk specifically regulates cytokine production by B cells from chronic inflammatory disease patients. J Immunol. (2009) 183:7461–70. doi: 10.4049/jimmunol.0901517
69. Luster AD. The chemokine system in innate immunity. Cold Spring Harbors Perspect Biol. (2015) 7:a016303. doi: 10.1101/cshperspect.a016303
70. Liskmann S, Vihalemm T, Salum O, Zilmer K, Fischer K, Zilmer M. Correlations between clinical parameters and interleukin-6 and interleukin-10 levels in saliva from totally edentulous patients with peri-implant disease. Int J Oral Maxillofac Implants. (2006) 21:543–50. doi: 10.1034/j.1600-0501.1994.050401.x
71. Schierano G, Cassarino E, Pagano M, Preti G, Emanuelli G. Transforming growth factor-beta and interleukin 10 in oral implant sites in humans. J Dental Res. (2003) 82:428–32. doi: 10.1177/154405910308200605
72. Lorenzo. Cytokines and bone: osteoimmunology. Handjournal Exp Pharmacol. (2020) 262:177–230. doi: 10.1007/164_2019_346
73. Lee SK, Kim HJ, Park J, Son SH, Kim KR, Chung WY. Human antigen R-regulated CCL20 contributes to osteolytic breast cancer bone metastasis. Sci Rep. (2017) 7:9610. doi: 10.1038/s41598-017-09040-4
74. Yao ZQ, Duan R, Li YY, Luo L, Boyce BF. RANKL cytokine enhances TNF-induced osteoclastogenesis independently of TNF receptor associated factor (TRAF) 6 by degrading TRAF3 in osteoclast precursors. J Biol Chem. (2017) 292:10169–79. doi: 10.1074/jbc.M116.771816
75. Ji H, Yang YL, Zhang Y, Iwamoto H, Lim S, Nakamura M, et al. TNFR1 mediates TNF-alpha-induced tumour lymphangiogenesis and metastasis by modulating VEGF-C-VEGFR3 signalling. Nat Commun. (2014) 5:4944. doi: 10.1038/ncomms5944
76. Deyama Y, Yoshimura Y, Izumiyama Y, Takeyama S, Hatta M, Totsuka Y, et al. Oral squamous cell carcinomas stimulate osteoclast differentiation. Oncol Rep. (2008) 20:663–8.
77. Quan JJ, Zhou GB, Parsons PG, Boyle GM, Gao J. Potential molecular targets for inhibiting bone invasion by oral squamous cell carcinoma: a review of mechanisms. Cancer Metastasis Rev. (2012) 31:209–19. doi: 10.1007/s10555-011-9335-7
78. Canalis. Effects of tumor necrosis factor on bone formation in vitro. Endocrinology. (1987) 121:1596–604. doi: 10.1210/endo-121-5-1596
79. Kaneki H, Chen D, Yao ZQ, Schwarz EM, Zhang YE, Boyce BF, et al. Tumor necrosis factor promotes Runx2 degradation through up-regulation of Smurf1 and Smurf2 in osteoblasts. J Biol Chem. (2006) 281:4326–33. doi: 10.1074/jbc.M509430200
80. Zuo CJ, Shi Y, Wu W, Zhang N, Xu JK, Wang CD, et al. TNF-α inhibits SATB2 expression and osteoblast differentiation through NF-κB and MAPK pathways. Oncotarget. (2017) 9:4833–50. doi: 10.18632/oncotarget.23373
81. Gilbert L, Farmer P, Boden S, Kozlowski M, Rubin J, Nanes MS. Inhibition of osteoblast differentiation by tumor necrosis factor-alpha. Endocrinology. (2000) 141:3956–64. doi: 10.1210/endo.141.11.7739
82. Lu XH, He XF, Rubin J, Nanes MS. Transcriptional regulation of the osterix (Osx, Sp7) promoter by tumor necrosis factor identifies disparate effects of mitogen-activated protein kinase and NF kappa B pathways. J Biol Chem. (2006) 281:6297–306. doi: 10.1074/jbc.M507804200
83. Kim N, Takami M, Lee JW, Lee SH, Okada F, Kim JH, et al. Osteoclast differentiation independent of the TRANCE-RANK-TRAF6 axis. Journal of Experiment Medicine. (2005) 202:589–95. doi: 10.1084/jem.20050978
84. Noguchi T, Hirao M, Kawase R, Ohama T, Yamashita S, Morimoto T, et al. Progranulin plays crucial roles in preserving bone mass by inhibiting TNF-α-induced osteoclastogenesis and promoting osteoblastic differentiation in mice. Biochem Biophys Res Commun. (2015) 465:638–43. doi: 10.1016/j.bbrc.2015.08.077
85. Graves DC. The contribution of interleukin-1 and tumor necrosis factor to periodontal tissue destruction. J Periodontol. (2003) 74:391–401. doi: 10.1902/jop.2003.74.3.391
86. Tan J, Xue P, An Y, Luo LK, Zhang R, Wu GS, et al. Tumor necrosis factor-α attenuates the osteogenic differentiation capacity of periodontal ligament stem cells by activating PERK signaling. J Periodontol. (2016) 87:e159–171. doi: 10.1902/jop.2016.150718
87. Garlanda C, Mantovani A. The interleukin-1 family: back to the future. Immunity. (2013) 39:1003–18. doi: 10.1016/j.immuni.2013.11.010
88. Banerjee M. Interleukin-1 (IL-1) family of cytokines: role in type 2 diabetes. Clinica Chimica Acta. (2012) 413:1163–70. doi: 10.1016/j.cca.2012.03.021
89. Schett G. Effects of inflammatory and anti-inflammatory cytokines on the bone. Eur J Clin Invest. (2011) 41:1361–6. doi: 10.1111/j.1365-2362.2011.02545.x
90. Braun T. Positive regulators of osteoclastogenesis and bone resorption in rheumatoid arthritis. Arthritis Res Ther. (2011) 13:235. doi: 10.1186/ar3380
91. Wei S, Zhou P, Ross FP, Teitelbaum SL. IL-1 mediates TNF-induced osteoclastogenesis. J Clin Invest. (2005) 115:282–90. doi: 10.1172/JCI200523394
92. Lader. Prostaglandin E2, interleukin 1alpha, and tumor necrosis factor-alpha increase human osteoclast formation and bone resorption in vitro. Endocrinology. (1998) 139:3157–64. doi: 10.1210/endo.139.7.6085
93. Liu SY, Pan F, Wise GE. Chronology and regulation of gene expression of RANKL in the rat dental follicle. Eur J Oral Sci. (2005) 113:404–9. doi: 10.1111/j.1600-0722.2005.00245.x
94. Kim YS, Lee HD, Oh HW, Kim EC. Effect of cytosolic phospholipase A2 on proinflammatory cytokine-induced bone resorptive genes including receptor activator of nuclear factor kappa B ligand in human dental pulp cells. J Endod. (2010) 36:636–41. doi: 10.1016/j.joen.2009.12.009
95. Graves DT, Garlet GP. Review of osteoimmunology and the host response in endodontic and periodontal lesions. J Oral Microbiol. (2011) 17:3. doi: 10.3402/jom.v3i0.5304
96. Liu XY, Wang MM, Gao Y, Zhang T, Hu G, Duan HQ, et al. IL-1alpha-induced microvascular endothelial cells promote neutrophil killing by increasing MMP-9 concentration and lysozyme activity. Immunol Res. (2016) 64:133–42. doi: 10.1007/s12026-015-8731-4
97. Sullivan CB, Evans SH, Ritter T, Shaw G, Barry F, Murphy JM. TNFα and IL-1β influence the differentiation and migration of murine MSCs independently of the NF-κB pathway. Stem Cell Res Ther. (2014) 5:104. doi: 10.1186/scrt492
98. Voss JO, Bara JJ, Fussinger MA, Duttenhoefer F, Alini M, Stoddart MJ. Effect of short-term stimulation with interleukin-1. Biomed Res Int. (2015) 2015:714230. doi: 10.1155/2015/714230
99. Chen YY, Lv CH, Chen Y, Zhao WH, Li WL, Chen HY, et al. NLRP3 regulates alveolar bone loss in ligature-induced periodontitis by promoting osteoclastic differentiation. Cell Prolif. (2021) 54:e12973. doi: 10.1111/cpr.12973
100. Bendre M, Nicholas RW, Suva LJ. Breast cancer metastasis to bone: it is not all about PTHrP. Clin Orthopeadics Relat Res. (2003) 415:S39–45 doi: 10.1097/01.blo.0000093844.72468.f4
101. Kudo AS, Pocock A, Itonaga I, Fujikawa Y, Athanasou NA. Interleukin-6 and interleukin-11 support human osteoclast formation by a RANKL-independent mechanism. Bone. (2003) 32:1–7. doi: 10.1016/S8756-3282(02)00915-8
102. Rose-John S, Scheller J, Grötzinger J, Seegert D. The IL-6/sIL-6R complex as a novel target for therapeutic approaches. Expert Opin Ther Targets. (2007) 11:613–24. doi: 10.1517/14728222.11.5.613
103. John MA, Zhou XF, Denny P, Ho CM, Montemagno C, Shi WY, et al. Interleukin 6 and interleukin 8 as potential biomarkers for oral cavity and oropharyngeal squamous cell carcinoma. Arch Otolaryngol Head Neck Surg. (2004) 130:929–35. doi: 10.1001/archotol.130.8.929
104. Gao IM, Maruo N, Kubota T, Murota S, Aso T. Expression of IL-6 receptor and GP130 in mouse bone marrow cells during osteoclast differentiation. Bone. (1998) 22:487–93. doi: 10.1016/S8756-3282(98)00040-4
105. Ishimi CM, Jin CH, Akatsu T, Abe E, Nakamura Y, Yamaguchi A, et al. IL-6 is produced by osteoblasts and induces bone resorption. J Immunol. (1990) 145:3297–303.
106. Huh J. IL-6 is produced by adipose-derived stromal cells and promotes osteogenesis. Biochim Biophys Acta. (2013) 1833:2608–16. doi: 10.1016/j.bbamcr.2013.06.025
107. Konttinen YT, Laine P, Kitti U, Santavirta S, Teronen O. Immunohistochemical evaluation of inflammatory mediators in failing implants. Int J Periodontics Restorative Dent. (2006) 26:135–41. doi: 10.1016/S0003-2670(99)00118-X
108. Tsukasaki M, Nagashima K, Nitta T, Pluemsakunthai W, Shukunami C, Iwakura Y, et al. Host defense against oral microbiota by bone-damaging T cells. Nature Commun. (2018) 9:701. doi: 10.1038/s41467-018-03147-6
109. Jimi E, Futura H, Matsuo K, Tominaga K, Takahashi T, Nakanishi O. The cellular and molecular mechanisms of bone invasion by oral squamous cell carcinoma. Oral Diseases. (2011) 17:462–8. doi: 10.1111/j.1601-0825.2010.01781.x
110. Kayamori K, Nakashima T, Takayanagi H, Morita KI, Omura K, Nguyen ST, et al. Roles of interleukin-6 and parathyroid hormone-related peptide in osteoclast formation associated with oral cancers: significance of interleukin-6 synthesized by stromal cells in response to cancer cells. Am J Pathol. (2010) 176:968–80. doi: 10.2353/ajpath.2010.090299
111. Karakasheva TA, Tang QS, Qiao E, Waldron TJ, Soni M, Klein-Szanto AJ, et al. IL-6 mediates cross-talk between tumor cells and activated fibroblasts in the tumor microenvironment. Cancer Res. (2018) 78:4957–70. doi: 10.1158/0008-5472.CAN-17-2268
112. Gu CF, Li XX. IL-17 family: cytokines, receptors and signaling. Cytokine. (2013) 64:10. doi: 10.1016/j.cyto.2013.07.022
113. Zhao RQ, Feng FF. Upregulated cellular expression of IL-17 by CD4+ T-cells in osteoporotic postmenopausal women. Ann Nutr Metab. (2016) 68:113–8. doi: 10.1159/000443531
114. Tyagi AM, Mansoori MN, Trivedi R, Chattopadhyay N, Singh D. Estrogen deficiency induces the differentiation of IL-17 secreting Th17 cells: a new candidate in the pathogenesis of osteoporosis. PLoS ONE. (2012) 7:e44552. doi: 10.1371/journal.pone.0044552
115. Huang Z, Graves DT. The interrelationship between diabetes, IL-17 and bone loss. Curr Osteoporos Rep. (2020) 18:23–31. doi: 10.1007/s11914-020-00559-6
116. Graves JD, Silva TA. The oral microbiota is modified by systemic diseases. J Dental Res. (2019) 98:148–56. doi: 10.1177/0022034518805739
117. Abusleme. IL-17: overview and role in oral immunity and microbiome. Oral Dis. (2017) 23:854–65. doi: 10.1111/odi.12598
118. Smit MJ, Brouwer E, Janssen K, Vissink A, Winkelhoff A. Periodontitis and rheumatoid arthritis: what do we know?. J Periodontol. (2015) 86:1013–9. doi: 10.1902/jop.2015.150088
119. Ginaldi L. Osteoimmunology and beyond. Curr Med Chem. (2016) 23:3754–74. doi: 10.2174/0929867323666160907162546
120. Queiroz-Junior C, Coelho FM, Costa VV, Bessoni RL, Sousa L, Garlet GP, et al. Experimental arthritis triggers periodontal disease in mice: involvement of TNF-α and the oral microbiota. J Immunol. (2011) 187:3821–30. doi: 10.4049/jimmunol.1101195
121. Corrêa JD, Ferreira GA, Mendonça SM, Fernandes GR, Xiao E, Teixeira AL, et al. Subgingival microbiota dysbiosis in systemic lupus erythematosus: association with periodontal status. Microbiome. (2017) 5:34. doi: 10.1186/s40168-017-0252-z
122. Daniel Cruceriu OB, Ovidiu Balacescu, Ioana Berindan-Neagoe. The dual role of tumor necrosis factor-alpha (TNF-á) in breast cancer: molecular insights and therapeutic approaches. Cell Oncol. (2020) 43:1–18. doi: 10.1007/s13402-019-00489-1
123. Huang W, Schwarz EM. Exposure to receptor-activator of NFkappaB ligand renders pre-osteoclasts resistant to IFN-gamma by inducing terminal differentiation. Arthritis Res Ther. (2003) 5:R49–59. doi: 10.1186/ar612
124. Gao YH, Ryan MR, Terauchi M, Page K, Yang XY, Weitzmann MN, et al. IFN-gamma stimulates osteoclast formation and bone loss in vivo via antigen-driven T cell activation. J Clin Invest. (2007) 117:122–32. doi: 10.1172/JCI30074
125. Kohara H, Fujimura Y, Yoshimatsu M, Morita Y, Eguchi T, Masuyama R, et al. IFN-γ directly inhibits TNF-α-induced osteoclastogenesis in vitro and in vivo and induces apoptosis mediated by Fas/Fas ligand interactions. Immunol Lett. (2011) 137:53–61. doi: 10.1016/j.imlet.2011.02.017
126. Sato TS, Shizume K, Yamakawa Y, Ono Y, Demura H, Akatsu T, et al. Prolonged decrease of serum calcium concentration by murine gamma-interferon in hypercalcemic, human tumor (EC-GI)-bearing nude mice. Cancer Res. (1992) 52:444–9.
127. Shashkova E, Cline-Smith A, Ferris C, Buchwald Z, Gibbs J, Novack D, et al. Osteoclast primed FoxP3+ CD8 T-cells induce T-bet and eomesodermin and IFN-γ to regulate bone resorption. J Immunol. (2016) 197:726–35. doi: 10.4049/jimmunol.1600253
Keywords: immune microenvironment, bone homeostasis, osteoimmunology, cytokines, immune cells (ICs)
Citation: Li N, Fu L, Li Z, Ke Y, Wang Y, Wu J and Yu J (2021) The Role of Immune Microenvironment in Maxillofacial Bone Homeostasis. Front. Dent. Med. 2:780973. doi: 10.3389/fdmed.2021.780973
Received: 22 September 2021; Accepted: 02 November 2021;
Published: 29 November 2021.
Edited by:
Zhipeng Fan, Capital Medical University, ChinaCopyright © 2021 Li, Fu, Li, Ke, Wang, Wu and Yu. This is an open-access article distributed under the terms of the Creative Commons Attribution License (CC BY). The use, distribution or reproduction in other forums is permitted, provided the original author(s) and the copyright owner(s) are credited and that the original publication in this journal is cited, in accordance with accepted academic practice. No use, distribution or reproduction is permitted which does not comply with these terms.
*Correspondence: Jinhua Yu, eXVqaW5odWFAbmptdS5lZHUuY24=
Disclaimer: All claims expressed in this article are solely those of the authors and do not necessarily represent those of their affiliated organizations, or those of the publisher, the editors and the reviewers. Any product that may be evaluated in this article or claim that may be made by its manufacturer is not guaranteed or endorsed by the publisher.
Research integrity at Frontiers
Learn more about the work of our research integrity team to safeguard the quality of each article we publish.